-
PDF
- Split View
-
Views
-
Cite
Cite
Yuanming Wu, Xiaohua Chen, Li Wang, Lunjie Wu, Lu Lin, Wenwu Ding, Zhenming Che, Jianfeng Wang, Jianzhou Li, Yi Liu, Weifeng Sun, Safe preparation of beefy meaty peptide with Bacillus subtilis, International Journal of Food Science and Technology, Volume 55, Issue 4, April 2020, Pages 1825–1832, https://doi.org/10.1111/ijfs.14468
- Share Icon Share
Abstract
Preparation of flavour peptides with microorganisms is an attractive choice for its large-scale production. However, beefy meaty peptide (BMP), as a research hotspot in the field of umami peptide, has been studied in few microorganisms, and furthermore, the safe preparation of BMP has not yet been reported. In this study, multi-copy BMP (8BMP) was successfully expressed and produced in the ‘generally recognized as safe’ Bacillus subtilis (B. subtilis). Firstly, 8BMP with the potentially intense umami was screened out based on molecular docking analysis. Then, it was successfully expressed in the B. subtilis 168 and verified through sensory evaluation. Finally, the scaling capacity of 8BMP was evaluated in a 5-L fermenter, with the highest yield (0.84 g L−1) 6.4 times than that of shake flask, which is conductive for industrial production of BMP and other food peptides.
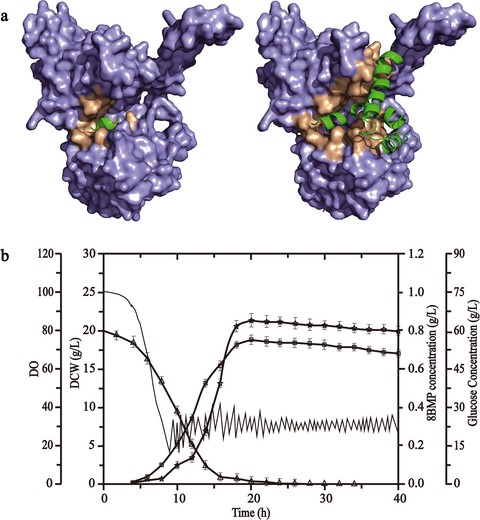
Introduction
Umami, one of the five basic tastes, perceived by the heterodimer T1R1/T1R3 of the G protein-coupled receptors in humans (Roper & Chaudhari, 2017), has been deeply enjoyed for its delightful taste (Kurihara, 2015). Umami peptide, as a natural umami ingredient after monosodium glutamate (MSG), guanosine monophosphate (GMP) and inosine monophosphate (IMP), owns advantages in nutrition and safety, which plays an essential role in food seasoning and healthy diet. Among numerous umami peptides, beefy meaty peptide (BMP), initially isolated from beef hydrolysate digested by papain (Yamasaki & Maekawa, 1978), was a heavily researched umami octapeptide with the primary amino acid sequence as Lys-Gly-Asp-Glu-Glu-Ser-Leu-Ala (KGDEESLA). It has attracted more and more attention in recent years (Wang et al., 1996; Iwaniak et al., 2016; Zhang et al., 2017).
Currently, BMP could be obtained by three methods: enzymatic extraction (Yamasaki & Maekawa, 1978), chemical synthesis (Yamasaki & Maekawa, 1980) and biological expression (Zeng et al., 2010; Zhang et al., 2017). The first two methods are not suitable for industrial production due to their low yields or high costs, whereas the last is an attractive approach to produce BMP (Li, 2012; Wu et al., 2013; Xia et al., 2013). Nevertheless, up to now, there are few reports about preparing BMP in microorganisms, except the biological expression of BMP in Pichia pastoris (P. pastoris) and in Escherichia coli (E. coli) (Zeng et al., 2010; Wang et al., 2011; Zhang et al., 2017). Furthermore, the industrial production of BMP was nearly limited by the relatively complicated fermentation of P. pastoris and the potential insecurity of E. coli (Hussein & Omaye, 2003; Vital et al., 2017). Therefore, another alternative biological expression is expected for safe and efficient preparation of BMP.
Molecular docking is a process of combining a ligand with its acceptor and uses the principles of geometric interaction and energy interaction to evaluate the binding effect to obtain the best docking model (Morris & Marguerita, 2008). Umami peptides also have their acceptors, such as the T1R1/T1R3 of the G protein-coupled receptors in humans (Toda et al., 2013; Roper & Chaudhari, 2017). Recently, molecular docking has been shown to contribute to the research of flavour peptides, which was considered to have the capacity of promoting the excavation of potential umami peptides (Toda et al., 2013; Roper & Chaudhari, 2017).
Bacillus subtilis (B. subtilis) has been regarded as a ‘generally recognised as safe’ (GRAS) microbe (Guan et al., 2015; Guan et al., 2016). Since it does not secret any exotoxin or endotoxin, B. subtilis owns natural advantages in food safety including the biological expression and industrial production of recombinant food proteins, such as amylase (Chen et al., 2015), lipase (Lu et al., 2010) and proinsulin (Jorge & Rosalia, 2003). Therefore, it would be a potentially feasible expression host for the safe and industrial production of BMP.
The aim of this work was to realise the safe and efficient production of BMP with intense umami. Firstly, the molecular docking was employed to screen the optimal copy number of BMP (8BMP). Then, the optimised 8BMP gene was inserted into pMA09 vector and transformed into B. subtilis 168 for efficient expression. The umami of 8BMP was verified and characterised by sensory evaluation. Finally, based on the optimisation of culture conditions in shake flask, the batch fermentation for 8BMP production in a 5-L fermenter was conducted using B. subtilis 168, which would provide a reference for the safe preparation of other umami peptides with microorganisms.
Materials and methods
Molecular docking
Homology modelling of the Venus flytrap domain of human umami receptor subunit T1R1 (VFTD/hT1R1) was performed by the Modeller software (Fiser & Šali, 2003). The best homologous structural template used for modelling was obtained by aligning the amino acid sequence of VFTD/hT1R1 (NCBI accession: DAA00012.1) using PSI-BLAST tool in PDB database (Altschul et al., 1997).
Since the amino acid number of multi-copy BMP (kBMP, k = 1, 2, 4, 8, 10 and 12) was no more than 100 amino acids, the de novo structure prediction for BMP, 2BMP and 4BMP were carried out using PEP-FOLD3 (suiting for five to fifty amino acids) (Lamiable et al., 2016) and for 8BMP, 10BMP and 12BMP using I-TASSER Suite (suiting for 10 to 1500 amino acids) (Yang et al., 2015), based on the length of the peptide chain. Molecular docking of each kBMP with the umami receptor VFTD/hT1R1 was executed using pyDockWeb server (Brian et al., 2013), which was evaluated by pyDock scoring function (Tammy et al., 2010). Subsequently, the lowest energy of kBMP-VFTD/hT1R1 (k = 1, 2, 4, 8, 10 and 12) complexes was screened by comparing their binding affinity ΔG (kcal mol−1) using PRODIGY (Xue et al., 2016). All interactions between the ligand kBMP and receptor VFTD/hT1R1 were analysed using PyMOL.
Strains, plasmids and reagents
The synthesis of 8BMP coding gene with 6 × His tag at C-terminus was performed in the Beijing Genomics Institute (Beijing, China). The B. subtilis-E. coli shuttle plasmid pMA09 was used for the expression of 8BMP in the B. subtilis 168. The NdeI, EcoRI and T4 DNA ligase were purchased from Takara (Dalian, China). Other chemicals (AR) and biochemicals (BR) were obtained commercially.
Plasmids construction
The codon optimisation of 8BMP gene (Table S1), with a C-terminal 6 × His tag and the recognition sites NdeI (CA↓TATG) and EcoRI (G↓AATTC) in 5′ end and 3′ end, respectively, was executed based on the preference of the host strain B. subtilis 168. The synthesised 8BMP gene and the vector pMA09 were separately digested by NdeI/EcoRI (Takara, Chongqing, China), and the digested 8BMP gene was inserted into the linear pMA09 for the pMA09-8BMP construction by T4 DNA ligase. The recombinant plasmid pMA09-8BMP was transferred to the empty strain B. subtilis 168 by chemical transformation, and then, the recombinant strain was extracted and cultured at 37 °C for 8-12 h on the solid medium with 50 μg mL−1 Kanamycin for positive clone screening. Finally, the host strain B. subtilis 168/pMA09-8BMP was obtained.
8BMP heterologous expression
The recombinant B. subtilis 168 carrying pMA09-8BMP was cultured in 5 mL Luria-Bertani (LB) medium with 50 μg mL−1 Kanamycin overnight (8 h). Next, 1% bacteria solution was transferred into 50 mL fresh liquid LB (containing Kanamycin 50 μg mL−1) for 30 h. All of the cultures were grown at 37 °C and 200 r.p.m. The umami peptide 8BMP was revealed by sodium dodecyl sulphate-polyacrylamide gel electrophoresis (SDS-PAGE) (Banga, 1998).
Purification of 8BMP
The purifier ÄKTA instrument (GE Healthcare UK Ltd.) was used to purify 8BMP by affinity chromatography. All the purification was kept in the 10 mm (pH 7.5) potassium phosphate buffer at 4 °C. The cell extracts were centrifuged at 13 000 g by centrifuge (Eppendorf 5427R) for 20 min and disrupted by sonication for 30 min. A HisTrap column (GE Healthcare UK Ltd.) was applied to purify 8BMP from cell disruption, and target fractions with 6 × His tag were eluted off through a series of gradient concentrations of imidazoles from 50 to 300 mm (Hochuli et al., 1988). Then, the purified umami peptide segments were dialysed in ultrapure water overnight for sensory evaluation, and it was revealed and examined by SDS-PAGE before the determination of protein concentration.
Sensory evaluation
The sensory evaluation group consisted of ten trained members (five women and five men). Five sensory attributes including basic sour, sweet, bitter, salty and umami were chosen to evaluate the taste of 8BMP. The standards of these five tastes are as follows: 0.8 g L−1 citric acid for the sour standard, 10 g L−1 sucrose for the sweet standard, 0.8 g L−1 quinine for the bitter standard, 3.5 g L−1 salt for the salty standard and 3.5 g L−1 MSG for the umami standard. Additionally, 0.2 g 8BMP was dissolved in 100 mL NaCl-MSG solution (0.42–0.18%, W/V, umami enhancing standard) to evaluate its umami enhancing effect. Before the sensory evaluation, the pH of the sample solution was adjusted to 6.5 by adding trace amounts of HCl or NaOH (0.01 mol L−1) to achieve the best taste (Liu et al., 2015). The five basic taste properties of sample were tested through putting 5 mL sample solution (0.2 g L−1 8BMP) in the mouth, followed by swirling around in the oral cavity for 10 s. The taste intensity of the all standard solutions was defined as ten points, and the umami peptide sample was scored in comparison with the standard solutions. The evaluation was performed at room temperature (25 °C).
Statistical analysis
The one-way ANOVA was performed by the SPSS 21.0 (SPSS Inc., Chicago, IL). The mean values were assessed by the paired t test and considered to have a significant difference (P < 0.05). Error bars represent the standard deviation for at least three independent experiments.
Shake flask optimisation
The basal medium of shake flask contained (g L−1): carbon sources (optimised) 20, yeast extract 20, K2HPO4·3H2O 12.5, KH2PO4 2.5 and MgSO4·7H2O 3. The optimisation experiment of culture conditions was investigated in shake flask, containing the effects of carbon source, inoculum size and culture temperature on the expression of umami peptides in recombinant B. subtilis 168/pMA09-8BMP. The factor levels were set as follows: the carbon sources (lactose, fructose, sucrose, glycerol and glucose), inoculum sizes (1%, 2%, 3%, 6% and 9%) and culture temperatures (24, 28, 32, 37 and 42 °C). Other culture conditions were consistent with those of heterologous expression. Finally, the optimal culture conditions were determined by dry cell weight and 8BMP concentration.
Batch fermentation
Fermentation medium contained (g L−1): corn steep liquor 50, yeast extract 20, K2HPO4·3H2O 12.5, KH2PO4 2.5, MgSO4·7H2O 3, CaCO3 5, glucose 60 and 15 mL of rare element solution. The rare metal solution contained (g L−1): FeSO4·7H2O 4.0, CaCl2 4.0, MnSO4·5H2O 1.0, CoCl2·6H2O 0.4, NaMoO4·2H2O 0.2, ZnSO4·7H2O 0.2, AlCl3·6H2O 0.1, CuCl2·H2O 0.1 and H3BO4 0.05 (Zhu et al., 2015).
Batch fermentation was conducted in a 5-L fermenter (Winpact Bench-Top, Major Science Inc., New Taipei City, Taiwan) containing 2.5 L fermentation medium. A single colony of the recombinant B. subtilis 168 carrying pMA09-8BMP from LB agar plate was inoculated in 5 mL LB liquid medium and cultivated at 37 °C for at least 12 h. Afterwards, 1% of the pre-culture was transferred into 500 mL shake flask with 50 mL working volume of LB liquid medium. After 8 h of cultivation with shaking, the bacterial culture was transferred into the fermenter at an inoculum size of 6%. Dissolved oxygen (DO) was monitored using a polarographic DO sensor and maintained above 30% saturation by controlling both the inlet air and the agitation rate between 200 and 800 r.p.m. The fermentation temperature was controlled at 37 °C through the automatic temperature control system, and the pH was maintained at 7 by associating pH detector with acid–base pump through adding 25% aqueous ammonia solution. Foaming was controlled by the addition of an anti-foaming agent (a mixture of organic polyether dispersions, Sigma). About 50 mL bacterial solution was sampled every 2 h during the whole fermentation process to detect dry cell weight and the concentration of 8BMP by the Bradford method (Hammond & Kruger, 1988).
Dry cell weight analysis
To determine the dry cell weight (DCW), 5 mL culture broth was centrifuged at 13 000 g for 20 min after a low-speed centrifugation to remove impurities. The pellet was washed with sterilised water, centrifuged at 13 000 g for 20 min and dried to a constant weight at 80 °C. The above washing steps were repeated three times.
Result and discussion
Selection of the 8BMP
Tandem repeat expression of short peptides has been confirmed to be an efficient strategy to avoid proteolytic degradation and improve production, such as antimicrobial peptides LL37 and PGLa-H (Rončević et al., 2017; Xiao et al., 2017). Therefore, BMP as a short peptide consisting of only eight amino acids (Yamasaki & Maekawa, 1978), was suitable for tandem repeat (copy number) expression.
The human’s umami taste perception is caused by the interaction between the umami substance and the umami receptor located in the taste bud. The strength of the interaction between the peptide and umami receptor could reflect the umami intensity perceived by human to some extent. In order to pre-determine the optimal copy number of BMP with the best umami intensity, molecular docking was performed between the ligand BMP and umami receptor hT1R1 by determining the strength of their interaction. Firstly, the structure of each kBMP (k = 1, 2, 4, 8, 10 and 12) was successfully predicted and assessed with PEP-FOLD3 and I-TASSER. Then, the VFTD/hT1R1 was chosen as the major binding domain of umami substance in the receptor (Toda et al., 2013; Ahn et al., 2018), because of its importance in the umami substance recognition (Li, 2009; Toda et al., 2013). Its structure was modelled using the crystal structure of VFTD of human CaSR (VFTD/hCaSR, PDB: 5K5T) as template based on the result of sequence alignment (Figure S1), and its homology modelling structure was considered to be reasonable based on PROCHECK calculation (Figure S2). Then, a series of docking models of each kBMP with VFTD/hT1R1 were obtained based on the pyDockWeb server, and the optimal ligand-receptor complex of each kBMP-VFTD/hT1R1 with the lowest energy was screened out according to the pyDock scoring results. Finally, the best kBMP (k = 1, 2, 4, 8, 10 and 12) was determined after comparing the binding affinity (ΔG, kcal mol−1) of each kBMP-VFTD/hT1R1 complex calculated by PRODIGY. The result indicated the ΔG absolute value of 8BMP-VFTD/hT1R1 was the highest (Table 1). Therefore, the 8BMP was regarded as the best one with potentially strongest umami intensity.
Binding affinity of the ligand multi-copy BMP and receptor VFTD/hT1R1 complex (kBMP-VFTD/hT1R1)
Complexes . | Binding affinity ΔG (kcal mol−1) . |
---|---|
BMP-VFTD/hT1R1 complex | −12.1 |
2BMP-VFTD/hT1R1 complex | −12.7 |
4BMP-VFTD/hT1R1 complex | −11.8 |
8BMP-VFTD/hT1R1 complex | −19.0 |
10BMP-VFTD/hT1R1 complex | −10.6 |
12BMP-VFTD/hT1R1 complex | −11.0 |
Complexes . | Binding affinity ΔG (kcal mol−1) . |
---|---|
BMP-VFTD/hT1R1 complex | −12.1 |
2BMP-VFTD/hT1R1 complex | −12.7 |
4BMP-VFTD/hT1R1 complex | −11.8 |
8BMP-VFTD/hT1R1 complex | −19.0 |
10BMP-VFTD/hT1R1 complex | −10.6 |
12BMP-VFTD/hT1R1 complex | −11.0 |
Binding affinity of the ligand multi-copy BMP and receptor VFTD/hT1R1 complex (kBMP-VFTD/hT1R1)
Complexes . | Binding affinity ΔG (kcal mol−1) . |
---|---|
BMP-VFTD/hT1R1 complex | −12.1 |
2BMP-VFTD/hT1R1 complex | −12.7 |
4BMP-VFTD/hT1R1 complex | −11.8 |
8BMP-VFTD/hT1R1 complex | −19.0 |
10BMP-VFTD/hT1R1 complex | −10.6 |
12BMP-VFTD/hT1R1 complex | −11.0 |
Complexes . | Binding affinity ΔG (kcal mol−1) . |
---|---|
BMP-VFTD/hT1R1 complex | −12.1 |
2BMP-VFTD/hT1R1 complex | −12.7 |
4BMP-VFTD/hT1R1 complex | −11.8 |
8BMP-VFTD/hT1R1 complex | −19.0 |
10BMP-VFTD/hT1R1 complex | −10.6 |
12BMP-VFTD/hT1R1 complex | −11.0 |
To further analyse the potential umami intensity of 8BMP, the structural comparison between 8BMP-VFTD/hT1R1 and BMP-VFTD/hT1R1 was performed. As shown in Fig. 1, both 8BMP and BMP could interact with the umami receptor VFTD/hT1R1. In detail, the small BMP was completely wrapped in this gap (Fig. 1a), while the bigger 8BMP might be more matchable for the whole active pocket of umami receptor VFTD/hT1R1 (Fig. 1b). It revealed that both 8BMP and BMP had strong interactions with the VFTD/hT1R1 and the 8BMP might be more effective to activate umami perception. Therefore, 8BMP was considered as the candidate with the most potentially intense umami taste.
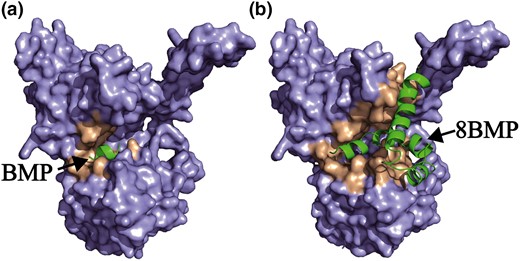
General interface view of molecular docking models of BMP-VFTD/hT1R1 complex (a) and 8BMPVFTD/hT1R1 complex (b).
Expression of 8BMP in B. subtilis 168
The optimised 8BMP gene was inserted into the vector pMA09 by T4 DNA ligase to construct the recombinant plasmid pMA09-8BMP (Figure S3). Two clear DNA segments obtained by NdeI/EcoRI double digestion of pMA09-8BMP were observed, the one with a size of about 7100 bps and the other with a size of about 210 bps (Figure S4). The validated plasmid pMA09-8BMP was then transferred into the host strain B. subtilis 168 for 8BMP expression. As a result, compared with the control expression (Fig. 2 lane 1), the target 8BMP in the recombined strain was clearly detected by SDS-PAGE (Fig. 2 lane 2), which was consistent with its theoretical molecular mass 7.5 kDa. Furthermore, the cell extracts with 8BMP were purified by His-tag affinity chromatography and detected at the same position (Fig. 2 lane 3). These results indicated that 8BMP could be successfully expressed in B. subtilis 168.
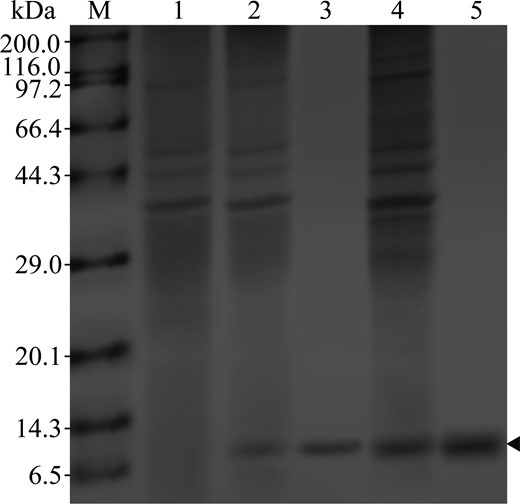
SDS-PAGE analysis of 8BMP collected from cell extracts of shake flask, affinity purification and cell extracts of fermenter. M: protein molecular weight marker, 1: B. subtilis 168/pMA09 extracts, 2: cell extracts with 8BMP of shake flask, 3: purified 8BMP, 4: cell extracts with 8BMP of fermenter and 5: purified 8BMP of fermenter.
Sensory evaluation of 8BMP
The taste profile analysis of successfully expressed 8BMP was conducted using sensory evaluation (Fig. 3). As shown in this taste profile, umami taste got the highest rating (8.6) among the five basic tastes, followed by sweet and salty, and the sour and bitter with the lower scores. Interestedly, the addition of 0.2 g 8BMP to 100 mL NaCl-MSG solution (0.42–0.18%, W/V) enhanced the intensity of umami effectively (score 12.8) (Fig. 3). These results indicated that 8BMP owns certain umami taste, possibly because the ligand binding space of umami receptor is large enough to accommodate the larger 8BMP, which was consistent with the previous analysis of molecular docking (Fig. 1).
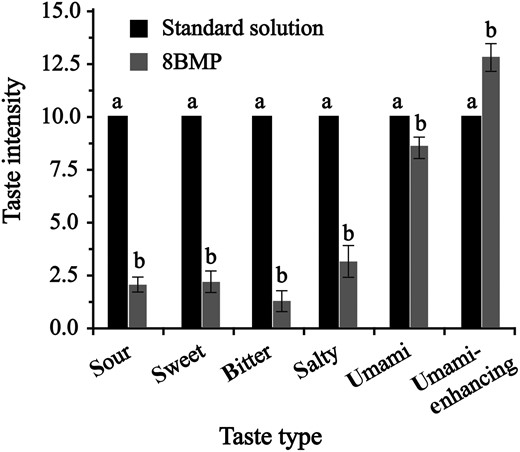
Sensory evaluation of 8BMP. Different letters indicate significant difference (P < 0.05).
Effect of culture conditions on cell growth and 8BMP expression in shake flask
Effect of culture medium compositions
The carbon source, as the most important energy source, could affect cell growth and protein heterogeneous expression (Khushoo et al., 2004). Therefore, B. subtilis 168 harbouring the plasmid pMA09-8BMP was cultured in the medium (supplemented with Kanamycin) with various carbon sources (lactose, fructose, sucrose, glycerol and glucose) for 40 h to determine the optimal carbon source. As shown in Fig. 4a, various carbon sources had a great influence on the cell growth and 8BMP expression, and the variance analysis showed significant differences among the carbon sources. Intuitively, glycerol (DCW 2.51 g L−1, 8BMP yield 0.117 mg mL−1) and glucose (DCW 2.62 g L−1, 8BMP yield 0.119 mg mL−1) could promote more cell growth and 8BMP expression than that of others, and the differences between them were not significant, indicating both glucose and glycerol were effective carbon sources for the 8BMP yield. However, considering that glucose was an effective fast-acting carbon source and would be more conducive for the cell growth of B. subtilis (Singh et al., 2008), it was chosen as the optimal carbon source.
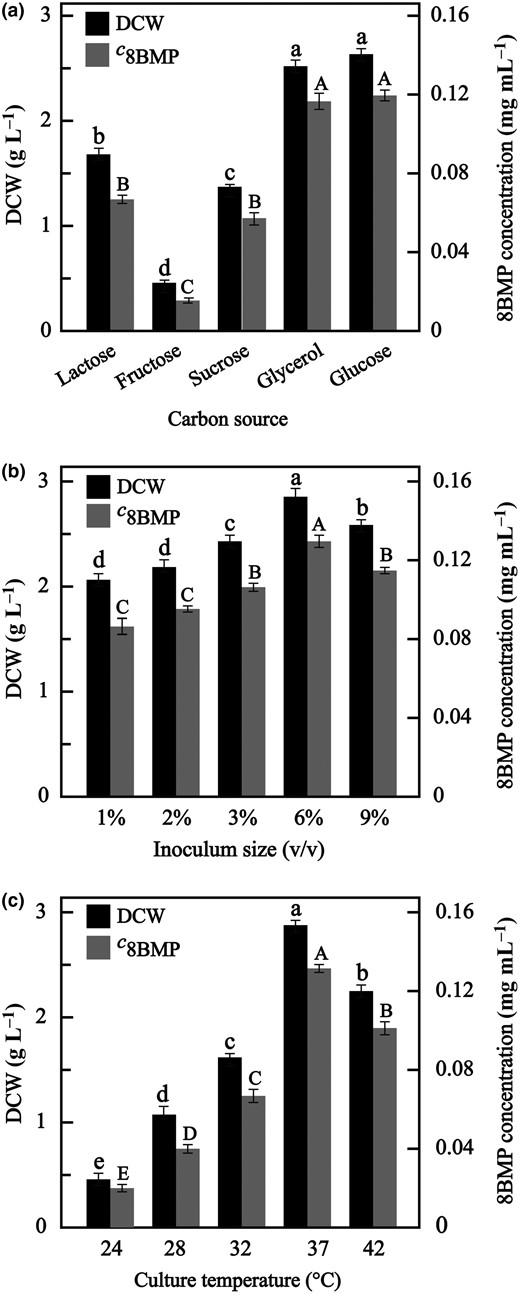
Comparison of cell growth and 8BMP expression in different culture conditions: (a) carbon source, (b) inoculum size and (c) culture temperature. c8BMP represents the concentration of 8BMP. Different letters indicate significant difference (P < 0.05). The letters among DCW were marked with lowercase letters, and those among the 8BMP concentration were marked with uppercase letters.
Effect of inoculum size
The inoculum size could affect the maintenance time of bacteria lag phase. Excessive inoculum size would speed up the growth of bacteria, causing low intensity of protein expression, while low inoculum size would prolong the lag time and is unconducive to protein expression (Khushoo et al., 2004). Therefore, the inoculum sizes of 1%, 2%, 3%, 6% and 9% were chosen to investigate the effect on cell growth and 8BMP expression based on the optimal carbon source. As shown in Fig. 4b, as the inoculum sizes rising from 1% to 6%, the DCW and 8BMP yield were increased synchronously and reached a maximum of 2.85 g L−1 and 0.129 mg mL−1, respectively, at 6%, which were significantly higher than others. However, the DCW and 8BMP yield decreased with the continuous rise of inoculum size, possibly because large inoculum accelerated cell growth rate and then resulted in premature cell ageing. These results indicated that various inoculum sizes could influence cell growth and 8BMP expression, and the optimal inoculum size was 6%.
Effect of culture temperature
Since the culture temperature directly affects the speed of growth and metabolism of bacteria (Khushoo et al., 2004), the effect of different temperatures on the cell growth and 8BMP expression was investigated at 24, 28, 32, 37 and 42 °C. As shown in Fig. 4c, the effects of different temperatures on cell growth and 8BMP expression were very significant. In detail, when the culture temperature was at a low level, such as 24, 28 and 32 °C, the final DCW and the 8BMP yield were less owing to the limited activity of metabolic enzyme at these temperatures. Obviously, 37 °C was the most favourable temperature for the cell growth and 8BMP expression, with DCW 2.88 g L−1 and 8BMP yield 0.131 mg mL−1. However, the negative effect of the 8BMP expression was also obvious when culture temperature increased from 37 to 42 °C, possibly because the high temperature affected the cell physiology, such as spore formation and metabolic acceleration (Irene et al., 2010). The significant differences of DCW and 8BMP yield at various temperatures indicated that culture temperature was a critical parameter for 8BMP expression in recombinant B. subtilis 168, and the optimal culture temperature was 37 °C.
In all, at the shake flask level, the optimal conditions were obtained: the carbon source was glucose, the inoculum size was 6%, and the culture temperature was 37 °C.
Scale ability of 8BMP in B. subtilis in a 5-L fermenter
The large-scale production of bioactive peptides through engineering bacteria is an attractive strategy in the industry (Wang et al., 2011; Da et al., 2015), which could effectively facilitate the development and application of related products. Here, based on the above results of cultivation in shake flask, the scale ability of 8BMP was investigated by performing fermentation in a 5-L fermenter. The expression and purification of 8BMP were determined at the protein level by SDS-PAGE analysis (Fig. 2 lane 4, 5). As shown in Fig. 5, the slow decline of DO in the first 5 h indicated a slow cell growth in the long stagnation period, and no target 8BMP was synthesised during this time. Subsequently, the bacteria entered the logarithmic phase, and the target 8BMP was gradually accumulated as the cells rapidly grew. Ultimately, the DCW and 8BMP yield reached maximum values of 19.29 and 0.84 g L−1 at 20 h, respectively, which were 6.7-fold and 6.4-fold higher than that detected in the shake flask. These results demonstrated that the scale ability of 8BMP in B. subtilis was appreciated in the 5-L fermenter, indicating that the safe preparation of 8BMP in B. subtilis would be an effective strategy for the production of BMP and other flavour peptides.
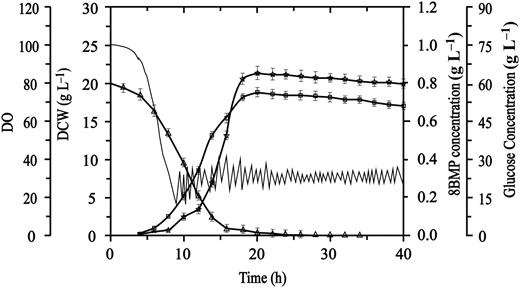
Batch fermentation of 8BMP in B. subtilis 168. DO (—), DCW (□), 8BMP production (☆) and glucose concentration (△).
Conclusion
Bacillus subtilis has been used as a safe and promising biofactory to express heterologous protein, especially for the food peptides. In this study, 8BMP with potentially intense umami was screened out based on the molecular docking analysis. Then, 8BMP was successfully expressed using B. subtilis 168, and its umami taste was verified by sensory evaluation. The scale ability of 8BMP was further examined in a 5-L fermenter. In summary, the safe preparation of 8BMP in B. subtilis could be applied for industrial production of BMP and other flavour peptides.
Acknowledgments
This work was supported by the National Natural Science Foundation of China (31601418, 31601444 and 21506172), the School level project of Hengyang Normal University (18D14), the Applied Basic Research Programs of Science and Technology Department of Sichuan Province (2019YJ0390), the Key Project of Xihua University of China (z1420524) and (z1420525), the Department of Education of Sichuan Province of China (15205445), the Key Laboratory program of Food and Biotechnology of Xihua University of China (szjj2015-013) and (szjj2015-014), Undergraduate Training Program for Innovation (201510623003) and (201510623053).
Conflict of interest
Authors have no conflict of interest to declare.
Ethical guidelines
Ethics approval was not required for this research.
Data availability statement
Research data are not shared.
References
Author notes
These authors contributed to the work equally.