-
PDF
- Split View
-
Views
-
Cite
Cite
Elif Çavdaroğlu, Stefano Farris, Ahmet Yemenicioğlu, Development of pectin–eugenol emulsion coatings for inhibition of Listeria on webbed-rind melons: a comparative study with fig and citrus pectins, International Journal of Food Science and Technology, Volume 55, Issue 4, April 2020, Pages 1448–1457, https://doi.org/10.1111/ijfs.14458
- Share Icon Share
Abstract
The objective of this study was to employ pectin-based antimicrobial coatings for inhibition of Listeria on surfaces of whole webbed-rind melons that cause frequent outbreaks of listeriosis. For this purpose, emulsion-based coatings were developed using citrus pectin (CPEC) or pectin extracted from processing wastes of sun-dried figs (FPEC) and eugenol (EUG). The emulsions of FPEC and CPEC with EUG (droplet size range: 1.99–11.22 µm) were highly stable for minimum 10 days at 10 °C. The FPEC-EUG films showed higher flexibility and degree of wettability than CPEC-EUG films. In contrast, CPEC-EUG films had a higher gas barrier performance against oxygen at 50% relative humidity than FPEC-EUG films. The zone inhibition tests showed that FPEC-EUG films are more effective against Listeria innocua than CPEC-EUG films. However, FPEC and CPEC coatings with 2% EUG caused 2.2 and 2.7 decimal inactivation of Listeria on Galia melons within 1 week, respectively. The pectin coatings with EUG could reduce the risk of listeriosis from webbed-rind melons.
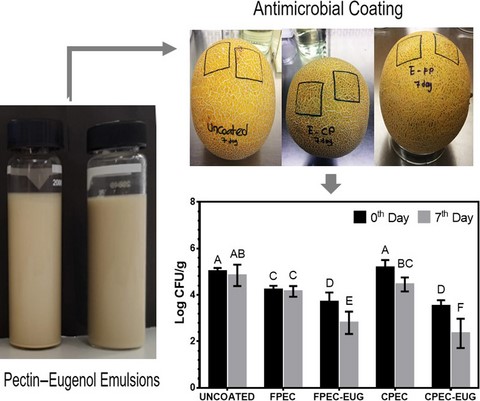
Introduction
The microbial outbreaks originating from fruits that grow on the ground are observed very frequently, as they are in direct contact with potential microbial contaminants such as irrigation water, sewage, manure or fertiliser, and animals (Sapers & Sites, 2003; Chen et al., 2012; Ma et al., 2016; De Corato, 2019). The melons are among the most important risk fruits since their stem scar and rough peel provide a unique protective environment for pathogenic bacteria such as Listeria monocytogenes, Salmonella enterica and Escherichia coli O157:H7 (Sapers & Sites, 2003; Chen et al., 2012) that could easily contaminate the inner edible parts of fruit during processes such as cutting and slicing (Ma et al., 2016). The cantaloupe (muskmelon, mushmelon, rockmelon or sweet melon) is a particularly risky melon cultivar since it has a complex webbed-rind surface that provides a protective growth medium for the Listeria spp. (Behrsing et al., 2003). An outbreak of listeriosis in the United States linked to cantaloupe melons in 2011 clearly proved the great risk of webbed-rind melons since it caused thirty three deaths, one miscarriage and 143 hospitalisations (CDC, 2012). In 2018, consumption of L. monocytogenes-contaminated cantaloupes caused seven deaths, one miscarriage with a total of twenty two confirmed cases in Australia (NSW DPI, 2018).
Active edible coatings incorporated with antimicrobials have been increasingly employed to inhibit microbial pathogens and to increase the quality of fresh-cut fruits (Rojas-Graü et al., 2009). Edible films of chitosan, alginate and zein incorporated with different natural and chemical agents (e.g. cinnamon oil, allyl isothiocyanate, eugenol, nisin, lauric arginate, ethylenediaminetetraacetic acid) have been applied for coating of whole melons (Chen et al., 2012; Ma et al., 2016; Boyacı et al., 2019). However, studies related to the application of emulsion-based pectin coatings incorporated with natural antimicrobials for coating of whole melons are scarce.
The objective of the current work is to employ antimicrobial emulsion-based coatings of pectin from different sources with EUG to eliminate Listeria contaminated on Galia melons (hybrids of cantaloupe and honeydew melons), which have a complex webbed-rind surface (as cantaloupe) and a sweet, creamy textured, light yellow to green flesh (as honeydew). Due to its inherent bio-adhesive properties (Farris et al., 2011), pectin might be a suitable coating material to deliver antimicrobials onto fruit surface contaminated with pathogens. The main original feature of this study is that, for the first time, it proposed the development of edible coatings using pectin extracted from wastes arising from the industry of sun-dried fig processing. The fig fruit has long been known for its high soluble dietary fibre content that is formed mainly by pectin (Trad et al., 2014). Turkey, with 78.2 metric tons of production in the 2017/18 season, is the largest producer and exporter of sun-dried figs in the world (Anonymous, 2018). The quality of fruits obtained by the classical sun-drying process that starts on trees and continues in fields until reaching the targeted moisture content (18%–22% moisture) is highly variable and depends strongly on climate, environmental factors and agricultural practices. Therefore, a considerable amount of the production is represented by low quality and defected sun-dried figs that, for this reason, can be processed to produce marmalade, molasses, animal feed or ethanol, depending on their quality and severity of their defects. This work opens new perspectives to fruit industry by employing sun-dried fig processing wastes in production of a value-added product such as pectin, and by characterisation and application of obtained pectin films for coating of webbed-rind melons that cause challenging safety problems. The coating procedure developed in this work could be applied to melons at the post-harvest period in packaging houses following classical washing procedures. This kind of an antimicrobial coating procedure may be an additional measure against remaining pathogens at the fruit surface.
Materials and methods
Materials
Citrus pectin (P9135, galacturonic acid ≥79%, methoxy content ≥8%) and eugenol (E51791) were obtained from Sigma-Aldrich (St. Louis, MO, USA). All other chemicals were reagent grade. Purees of sun-dried fig processing wastes (a mixture of highly defected fruits, fruit residues from processing, fruits routinely separated for quality control), all passed from UV inspection for luminescence that indicates aflatoxins, were kindly supplied by KFC Gida A.S. (Izmir, Turkey). The purees were mixed, divided into small portions, and kept at −20 °C until used for pectin extraction. The Listeria innocua NRRL-B 33314 (ATCC 1915) was from the culture collection of the microbiology laboratory of the Department of Food Engineering at Izmir Institute of Technology. The Galia melons (Cucumis melo var. reticulatus) were purchased from a local market in Izmir, Turkey.
Methods
Extraction of fig pectin from waste material
Pectin was extracted by the hot acidic extraction method (Yuliarti et al., 2015) with slight modifications. For this purpose, 50 g of sun-dried fig puree was mixed with 150 mL of a solution of citric acid (CA) at different concentrations (1.0%, 3.0% or 6.0% (w/v)). The mixture was then homogenised for 1 min at high speed using a Waring laboratory blender (31BL91, Torrington, CT, USA) equipped with a stainless steel jar. The homogenate was first heated to 95 °C using a hotplate under continuous stirring (for 1 or 3 h) and then cooled to room temperature in an ice bath, followed by centrifugation at 5000 g at 4 °C for 20 min. The supernatant was collected (extract-1), and the precipitate obtained was extracted again (sample to acid ratio 1:1, CA concentration 1.0%, 3.0% or 6.0% (w/v), extraction at 95 °C for 30 min) to collect the residual pectin. The mixture obtained from the second extraction was centrifuged (extract-2), and it was combined with the extract-1. For the precipitation of pectin in the total extract, 96% ethanol (extract to ethanol ratio 1:2) was mixed with the extract at room temperature, and the mixture was stirred for 30 min. The extract–ethanol mixture was then left at 4 °C for 24 h to precipitate the pectin. The precipitated pectin was collected by centrifugation at 9600 g for 10 min. The collected precipitate was then washed twice with ethanol to remove impurities and water as well as to reduce brown pigments. The obtained purified pectin was then dried for 12–18 h in an oven at 40 °C to remove residual ethanol, and then, it was brought to ≤5% moisture content by lyophilisation (Labconco, FreeZone, 6 L, Kansas City, MO, USA).
Molecular properties and composition of extracted pectin
The degree of esterification of the fig pectin was determined by the titrimetric method described by Nazaruddin et al. (2013). The results were expressed as % from the average of three measurements.
The galacturonic acid (GA) content was determined by the classical meta-hydroxydiphenyl method spectrophotometrically at 520 nm (Cemeroğlu, 2010). The standard curve was obtained using GA. The average of three measurements was used to calculate the results as % (g GA per 100 g of pectin).
The soluble protein content of extracted pectin was determined by the Bradford method (1976) using BSA as a standard. The average of triplicate measurements was expressed as g protein per 100 g of pectin.
The phenolic content of extracted pectin was determined spectrophotometrically at 760 nm using the Folin–Ciocalteu's reagent as a reactive compound and gallic acid (GAE) as a standard (Singleton & Rossi, 1965). The average of triplicate measurements was expressed as mg GAE per 100 g of pectin.
Preparation of pectin–EUG emulsion-based films and coatings
For this purpose, 3 g of FPEC or CPEC was suspended in 100 mL of distilled water. The suspension was then heated on a hotplate under continuous stirring at 60 °C for 30 min. After cooling to room temperature, the solution was homogenised at 10 000 r.p.m. for 1 min using a homogeniser (Heidolph, Germany, rotor ɸ = 6.6 mm tip). The pH of the mixture was adjusted to 8.0 using 4 m NaOH, and it was stirred for 30 min to cause de-esterification of pectin. This process intended increasing pectins’ negatively charged exposed –COO− groups that are important to create repulsive forces among oil droplets and increase emulsion stability (Ngouémazong et al., 2015). The pH of the mixture was then slowly lowered to a final value between 3 and 4 with 1 m HCl. After that, 0.9 g of glycerol (30% of pectin) was added as a plasticiser of emulsion films, and the mixture was stirred for 15 min. Finally, to prepare FPEC-EUG and CPEC-EUG emulsions, EUG was added into the mixture at different concentrations (0.25%, 0.5%, 1%, 2% or 3% (w/w)), and the mixtures were then homogenised at 10 000 r.p.m. for 4 min. The freshly prepared emulsions with desired amounts of EUG were used directly in melon coating studies as described in Section 2.2.6 while films used in characterisation studies and zone inhibition tests on the agar surface were obtained by the casting method. To obtain pre-cast films, 20 g of the emulsion was cast onto a glass Petri dish (inner diameter 10 cm), which was dried in a controlled test cabinet at 25 °C and 50% RH for 24 h.
Stability of pectin–EUG emulsions
The stability of FPEC-EUG and CPEC-EUG emulsions was determined by monitoring the turbidity evolution for 10 days at 10 °C. The turbidity values were expressed in nephelometric turbidity units (NTU) using a HACH turbidity meter (2100 AN, USA) and in absorbance units by measuring the absorbance of emulsions at 600 nm. Tests were conducted using two replicates. Zeta potential and particle size of the emulsions were also determined using a NanoPlus DLS Particulate Systems (Micromeritics Instrument Corporation, GA, USA).
Antimicrobial activity of films on inoculated agar surface
The antimicrobial activity of freshly prepared pre-cast FPEC-EUG and CPEC-EUG films containing EUG between 0.25% and 2% (w/w) was tested under aseptic conditions by the agar diffusion method as reported by Boyacı et al. (2019) using L. innocua as test microorganism. The discs (diameter: 1.3 cm) of pre-cast and dried films were formed at aseptic conditions by a cork borer. Antimicrobial activity test was conducted as two replicates. Nine discs (1 disc was placed per Petri dish) from each film were tested at each replicate. The diameters of the clear zones formed around the discs were measured by a micrometer (Chronos®, UK), and the average zone areas were expressed in cm2.
Antimicrobial activity of coatings on inoculated webbed-rind melons
The antimicrobial activity of FPEC-EUG and CPEC-EUG coatings with 2% EUG was also tested on inoculated melons. Melons were first washed extensively in tap water, followed by ethanol (70%, w/w) and sterile distilled water. The cleaned melons were then left to dry under the laminar flow hood overnight at room temperature. For the preparation of the inoculum, overnight cultures of L. innocua grown in nutrient broth under aerobic conditions at 37 °C were prepared. One mL of this active culture was transferred to 9 mL of nutrient broth in a tube and incubated at 10 °C for 24 h to promote the adaptation of the culture to the cold-storage conditions. Two separate zones (4 cm × 4 cm) on each melon's surface were then inoculated by spreading 150 µL of the L. innocua culture (108 CFU mL−1). The inoculated melons were kept under aseptic conditions for 20 min to promote the absorption and drying of the inoculum on the melon surface. Freshly prepared solutions of FPEC-EUG or CPEC-EUG containing EUG at 2% (w/w) and control FPEC or CPEC pectin film solutions (150 µL) were then pipetted onto the inoculated areas (4 cm × 4 cm) of melons and spread homogeneously using a sterile plastic rod. Inoculated melons without film treatment were used as control. Melons were kept for 30 min under laminar flow hood to dry pectin film solutions on their surface (0th day). After that, melons were stored at 10 °C and 50% RH for 7 days and enumerated for their L. innocua counts.
Microbiological tests were carried out on the 0th and 7th days. A ten g portion of treated areas (4 cm × 4 cm area framed previously with a marker) of melon rind were excised using a sterile knife. The cut rind pieces were placed into stomacher bags (Thermo Fisher Scientific, Inc., Waltham, MA, USA) containing 90 mL sterile 0.1% (w/w) peptone water and homogenised for 150 s using a stomacher (BagMixer® 400, Interscience, France). The homogenates were then serially diluted with 0.1% w/v peptone water and surface plated on Oxford Listeria Selective Agar (Merck, Darmstadt, Germany) with Oxford Listeria Selective Supplement (Merck, Darmstadt, Germany). Counting of colonies was carried out after 48-h incubation at 37 °C. The counts were performed in triplicate plates for two inoculated areas (4 cm × 4 cm) for each treatment (uncoated, FPEC- or CPEC-coated, and FPEC-EUG- or CPEC-EUG-coated samples). Microbiological counts were expressed as logarithm (Log) of colony-forming unit per gram (Log CFU g−1) for each treatment.
Tensile properties of films
Tensile strength at break, elongation at break, and Young's modulus were determined using a Texture Analyzer TA-XT2 (Stable Microsystems, Godalming, UK) according to ASTM Standard Method D 882-02 (ASTM, 2002). The dried films were cut into 5 mm wide and 80 mm long strips. The initial grip distance was 50 mm, and the crosshead speed was 50 mm min−1. At least eight replicates of each film were tested. The thickness of the films was measured by using a micrometer (Chronos®, UK).
Scanning Electron Microscopy (SEM) of films
The cross-sectional morphologies of pectin film samples with and without eugenol were examined by using SEM (250 Quanta FEG, FEI Company, USA). The films were prepared by crushing after freezing in liquid nitrogen. Specimens were gold-coated with a sputter coater (Emitech K550X, Quorum Technologies Inc., UK) under 15 mA for 60 s.
Surface wettability
Water contact angle measurements were performed on both the pristine (control) pectin films (FPEC and CPEC) and the EUG-containing pectin films (FPEC-EUG and CPEC-EUG) using an optical contact angle apparatus (OCA 15 Plus, Data Physics Instruments GmbH, Filderstadt, Germany) equipped with a high-resolution CCD camera and a high-performance digitising adapter. SCA20 software (Data Physics Instruments GmbH, Filderstadt, Germany) was used for the image capturing and contact angle determination. Rectangular specimens (3 × 1.5 cm2) were kept flat throughout the analysis, and the contact angle of water in air (θ, °) was measured by gently dropping a droplet of 4.0 ± 0.5 mL of Milli-Q water (18.3 MV cm) onto the substrate at 23 ± 1 °C and 50 ± 2% relative humidity (RH).
Oxygen Transmission Rate (OTR)
The oxygen barrier properties of the films were assessed using a Multiperm permeability analyzer (Extrasolution Srl, Capannori, Italy) equipped with an electrochemical sensor. Pectin films were sandwiched between two aluminium-tape masks, allowing a surface of 2.5 cm2 to be exposed to the permeation of oxygen. The oxygen transmission rate (OTR, mL m‒2 24 h‒1 cm3 m‒2 24 h‒1) was determined according to the standard method of ASTM F1927, with a carrier flow (N2) of 10 mL min‒1 at 23 °C and 50% relative humidity (RH), and at 1 atm pressure difference on the two sides of the specimen. Each OTR value was from three replicates.
Statistical analysis
Results were analysed for significance by using variance analysis (one way-Anova) and Fisher post-test using Minitab (ver.16.2.0.0, Minitab Inc., UK). The differences were considered significant if P ≤ 0.05.
Results and discussions
Extraction yield and some characteristics of fig pectin from waste material
The extraction yields and some molecular properties of pectin obtained from sun-dried fig processing wastes at different extraction conditions are given in Table 1. The yield of pectin ranged between 6.70% and 9.12% was increased by the increase of CA concentration. In contrast, the increase of extraction time from 1 to 3 h at a given CA concentration did not cause a significant increase in the pectin yield (P > 0.05). Thus, the application of 3 h extraction is not beneficial to improve extraction yield. The basic molecular properties, GA and DE, of fig pectin ranged between 18.6%–24.6% and 57.4%–79.3% depending on the severity of extraction, respectively. The most effective factor on the molecular properties was CA that caused a concentration-dependent reduction in GA and DE of pectin. The increase of the extraction period from 1 to 3 h at a certain concentration of CA had no significant effect on GA of pectin (P > 0.05). The increase of the extraction period did not also affect DE in presence of 1% and 3% CA while this caused a significant reduction in DE in the presence of CA at 6% (P < 0.05). The overall results suggested that extraction at 95 °C with 3% or 6% CA for 1 h could be employed to obtain fig pectin yield between 8.0% and 9.0%. In the current work, to minimise modifications in pectin chain length, mild extraction at 3% CA for 1 h was applied rather than extraction at 6% CA for 1 h.
Yield, galacturonic acid content and degree of esterification of pectins extracted from sun-dried fig processing waste at different conditions
CA conc. (%) . | Time (h) . | Yield (%) . | GA (%) . | DE (%) . |
---|---|---|---|---|
1 | 1 | 7.00 ± 0.14CD | 24.56 ± 2.43A | 76.48 ± 2.69A |
3 | 1 | 8.21 ± 0.35B | 20.16 ± 1.51BC | 67.08 ± 0.42B |
6 | 1 | 9.12 ± 0.49A | 18.56 ± 2.94C | 60.76 ± 2.23C |
1 | 3 | 6.70 ± 0.62D | 24.17 ± 0.77A | 79.25 ± 1.68A |
3 | 3 | 7.74 ± 0.40BC | 22.90 ± 1.50AB | 68.03 ± 1.29B |
6 | 3 | 8.58 ± 0.64AB | 18.87 ± 1.19C | 57.44 ± 1.26D |
CA conc. (%) . | Time (h) . | Yield (%) . | GA (%) . | DE (%) . |
---|---|---|---|---|
1 | 1 | 7.00 ± 0.14CD | 24.56 ± 2.43A | 76.48 ± 2.69A |
3 | 1 | 8.21 ± 0.35B | 20.16 ± 1.51BC | 67.08 ± 0.42B |
6 | 1 | 9.12 ± 0.49A | 18.56 ± 2.94C | 60.76 ± 2.23C |
1 | 3 | 6.70 ± 0.62D | 24.17 ± 0.77A | 79.25 ± 1.68A |
3 | 3 | 7.74 ± 0.40BC | 22.90 ± 1.50AB | 68.03 ± 1.29B |
6 | 3 | 8.58 ± 0.64AB | 18.87 ± 1.19C | 57.44 ± 1.26D |
Data at each column indicated by different letters are significantly different (P ≤ 0.05).
Yield, galacturonic acid content and degree of esterification of pectins extracted from sun-dried fig processing waste at different conditions
CA conc. (%) . | Time (h) . | Yield (%) . | GA (%) . | DE (%) . |
---|---|---|---|---|
1 | 1 | 7.00 ± 0.14CD | 24.56 ± 2.43A | 76.48 ± 2.69A |
3 | 1 | 8.21 ± 0.35B | 20.16 ± 1.51BC | 67.08 ± 0.42B |
6 | 1 | 9.12 ± 0.49A | 18.56 ± 2.94C | 60.76 ± 2.23C |
1 | 3 | 6.70 ± 0.62D | 24.17 ± 0.77A | 79.25 ± 1.68A |
3 | 3 | 7.74 ± 0.40BC | 22.90 ± 1.50AB | 68.03 ± 1.29B |
6 | 3 | 8.58 ± 0.64AB | 18.87 ± 1.19C | 57.44 ± 1.26D |
CA conc. (%) . | Time (h) . | Yield (%) . | GA (%) . | DE (%) . |
---|---|---|---|---|
1 | 1 | 7.00 ± 0.14CD | 24.56 ± 2.43A | 76.48 ± 2.69A |
3 | 1 | 8.21 ± 0.35B | 20.16 ± 1.51BC | 67.08 ± 0.42B |
6 | 1 | 9.12 ± 0.49A | 18.56 ± 2.94C | 60.76 ± 2.23C |
1 | 3 | 6.70 ± 0.62D | 24.17 ± 0.77A | 79.25 ± 1.68A |
3 | 3 | 7.74 ± 0.40BC | 22.90 ± 1.50AB | 68.03 ± 1.29B |
6 | 3 | 8.58 ± 0.64AB | 18.87 ± 1.19C | 57.44 ± 1.26D |
Data at each column indicated by different letters are significantly different (P ≤ 0.05).
In the literature, studies related to pectin extraction from sun-dried fig processing wastes are scarce. However, the extraction yield obtained at 95 °C with 3% or 6% CA for 1 h from sun-dried fig waste in the current work was higher than the pectin yield of 6% obtained by Garibzahedi et al. (2019), who extracted peels of fresh figs with CA-acidified hot water at 90 °C for 1 h. Moreover, the extraction yield of Garibzahedi et al. (2019) from ultrasound–microwave-assisted extraction (11.7%) was higher than those obtained in the current work with hot acidic extraction. These results clearly showed that the processing wastes of fresh or dried figs could be evaluated as a source of pectin.
Stability of pectin–EUG emulsions
The 3% solutions of FPEC or CPEC formed highly turbid and stable emulsions with EUG at concentrations between 0.25% and 2%. This was demonstrated by the stable spectrophotometric absorbance measurements at 600 nm for both FPEC-EUG and CPEC-EUG emulsions that cold-stored at 10 °C for 10 days (see Table S1). The turbidity of all FPEC-EUG and most of CPEC-EUG emulsions was also not measurable (>10 000 NTU). The only measurable NTUs were those obtained from CPEC at 0.25% EUG and 1% EUG, with values that remained stable during 10 days of cold storage in the ranges 4000–5000 NTU and 8000–10 000 NTU, respectively (see Table S1). The stability of FPEC-EUG and CPEC-EUG emulsions was also proved by their limited changes in droplet size and zeta potential values during 10 days of cold storage (Table 2). The initial droplet size of FPEC-EUG emulsions was significantly lower than that of CPEC-EUG emulsions. This result seems to indicate a better emulsifying capability of FPEC than CPEC, which could be ascribed to the high protein content of FPEC coming from the seeds of fig (FPEC and CPEC contain 15.0 and 6.2 g protein per 100 g pectin, respectively). No significant differences were detected in the droplet size (3.46–10.08 µm) for both types of emulsions between 3 and 10 days of cold storage, which suggests that FPEC and CPEC behaved in a similar way in terms of emulsion stability. It is also important to note that both emulsions remained stable and they did not show any visually detectable phase separation minimum 6 weeks at 10 °C. Moreover, FPEC-EUG emulsions showed slightly to moderately lower zeta potential values than CPEC-EUG emulsions, possibly due to a lower GA content (means less –COO-), but higher protein content (might mask negative charges) of FPEC than CPEC. Further long-term storage stability tests are needed to understand the feasibility of commercialising ready-to-use emulsion preparations. However, it should be noted that the best performance (homogeneity and effectiveness) from antimicrobial emulsion-based coatings with volatile essential oils is obtained when their emulsions are prepared freshly before each application.
Droplet sizes and zeta potentials of different FPEC-EUG and CPEC-EUG emulsions during cold storage at 10 °C
EUG (%, w/w) . | D[3,2], µm . | Zeta Potential, mV . | ||||||
---|---|---|---|---|---|---|---|---|
0th Day . | 3rd Day . | 7th Day . | 10th Day . | 0th Day . | 3rd Day . | 7th Day . | 10th Day . | |
FPEC-EUG emulsions | ||||||||
0.25% | 1.99 ± 0.28c,C | 3.46 ± 0.07d,B | 3.52 ± 0.83c,B | 4.65 ± 0.32c,A | −9.48 ± 1.22c,A | −9.57 ± 0.80bc,A | −10.93 ± 5.40b,AB | −14.96 ± 1.19b,B |
1% | 2.02 ± 0.29c,B | 3.89 ± 0.09d,A | 3.91 ± 0.72c,A | 4.52 ± 0.87c,A | −5.64 ± 0.77b,A | −6.17 ± 0ab,A | −5.65 ± 0a,A | −5.35 ± 0.89a,A |
2% | 2.31 ± 0.64c,B | 4.19 ± 0.38d,A | 3.96 ± 0.44c,A | 4.55 ± 0.51c,A | −2.12 ± 0.49a,A | −3.34 ± 0.57a,A | −6.51 ± 1.21ab,B | −7.50 ± 1.67a,B |
CPEC-EUG emulsions | ||||||||
0.25% | 6.93 ± 0.4b,AB | 7.15 ± 0.36c,A | 6.60 ± 0.82b,AB | 6.11 ± 0.98b,B | −17.34 ± 0.28d,B | −16.23 ± 0.21d,A | −16.43 ± 0.27c,A | −16.44 ± 0.24b,A |
1% | 8.41 ± 3.08b,A | 8.96 ± 1.87b,A | 6.92 ± 1.12b,A | 7.18 ± 1.19ab,A | −19.06 ± 2.79d,A | −20.07 ± 3.80e,A | −19.24 ± 2.73c,A | −20.26 ± 4.10c,A |
2% | 11.22 ± 2.76a,A | 10.08 ± 0.75a,AB | 8.99 ± 1.61a,AB | 8.70 ± 1.70a,B | −10.29 ± 2.51c,A | −13.02 ± 2.85cd,AB | −16.30 ± 1.15c,BC | −18.27 ± 2.42bc,C |
EUG (%, w/w) . | D[3,2], µm . | Zeta Potential, mV . | ||||||
---|---|---|---|---|---|---|---|---|
0th Day . | 3rd Day . | 7th Day . | 10th Day . | 0th Day . | 3rd Day . | 7th Day . | 10th Day . | |
FPEC-EUG emulsions | ||||||||
0.25% | 1.99 ± 0.28c,C | 3.46 ± 0.07d,B | 3.52 ± 0.83c,B | 4.65 ± 0.32c,A | −9.48 ± 1.22c,A | −9.57 ± 0.80bc,A | −10.93 ± 5.40b,AB | −14.96 ± 1.19b,B |
1% | 2.02 ± 0.29c,B | 3.89 ± 0.09d,A | 3.91 ± 0.72c,A | 4.52 ± 0.87c,A | −5.64 ± 0.77b,A | −6.17 ± 0ab,A | −5.65 ± 0a,A | −5.35 ± 0.89a,A |
2% | 2.31 ± 0.64c,B | 4.19 ± 0.38d,A | 3.96 ± 0.44c,A | 4.55 ± 0.51c,A | −2.12 ± 0.49a,A | −3.34 ± 0.57a,A | −6.51 ± 1.21ab,B | −7.50 ± 1.67a,B |
CPEC-EUG emulsions | ||||||||
0.25% | 6.93 ± 0.4b,AB | 7.15 ± 0.36c,A | 6.60 ± 0.82b,AB | 6.11 ± 0.98b,B | −17.34 ± 0.28d,B | −16.23 ± 0.21d,A | −16.43 ± 0.27c,A | −16.44 ± 0.24b,A |
1% | 8.41 ± 3.08b,A | 8.96 ± 1.87b,A | 6.92 ± 1.12b,A | 7.18 ± 1.19ab,A | −19.06 ± 2.79d,A | −20.07 ± 3.80e,A | −19.24 ± 2.73c,A | −20.26 ± 4.10c,A |
2% | 11.22 ± 2.76a,A | 10.08 ± 0.75a,AB | 8.99 ± 1.61a,AB | 8.70 ± 1.70a,B | −10.29 ± 2.51c,A | −13.02 ± 2.85cd,AB | −16.30 ± 1.15c,BC | −18.27 ± 2.42bc,C |
a–e and A–C values followed by different letters at each column and row indicate statistically significant differences (P ≤ 0.05), respectively.
Droplet sizes and zeta potentials of different FPEC-EUG and CPEC-EUG emulsions during cold storage at 10 °C
EUG (%, w/w) . | D[3,2], µm . | Zeta Potential, mV . | ||||||
---|---|---|---|---|---|---|---|---|
0th Day . | 3rd Day . | 7th Day . | 10th Day . | 0th Day . | 3rd Day . | 7th Day . | 10th Day . | |
FPEC-EUG emulsions | ||||||||
0.25% | 1.99 ± 0.28c,C | 3.46 ± 0.07d,B | 3.52 ± 0.83c,B | 4.65 ± 0.32c,A | −9.48 ± 1.22c,A | −9.57 ± 0.80bc,A | −10.93 ± 5.40b,AB | −14.96 ± 1.19b,B |
1% | 2.02 ± 0.29c,B | 3.89 ± 0.09d,A | 3.91 ± 0.72c,A | 4.52 ± 0.87c,A | −5.64 ± 0.77b,A | −6.17 ± 0ab,A | −5.65 ± 0a,A | −5.35 ± 0.89a,A |
2% | 2.31 ± 0.64c,B | 4.19 ± 0.38d,A | 3.96 ± 0.44c,A | 4.55 ± 0.51c,A | −2.12 ± 0.49a,A | −3.34 ± 0.57a,A | −6.51 ± 1.21ab,B | −7.50 ± 1.67a,B |
CPEC-EUG emulsions | ||||||||
0.25% | 6.93 ± 0.4b,AB | 7.15 ± 0.36c,A | 6.60 ± 0.82b,AB | 6.11 ± 0.98b,B | −17.34 ± 0.28d,B | −16.23 ± 0.21d,A | −16.43 ± 0.27c,A | −16.44 ± 0.24b,A |
1% | 8.41 ± 3.08b,A | 8.96 ± 1.87b,A | 6.92 ± 1.12b,A | 7.18 ± 1.19ab,A | −19.06 ± 2.79d,A | −20.07 ± 3.80e,A | −19.24 ± 2.73c,A | −20.26 ± 4.10c,A |
2% | 11.22 ± 2.76a,A | 10.08 ± 0.75a,AB | 8.99 ± 1.61a,AB | 8.70 ± 1.70a,B | −10.29 ± 2.51c,A | −13.02 ± 2.85cd,AB | −16.30 ± 1.15c,BC | −18.27 ± 2.42bc,C |
EUG (%, w/w) . | D[3,2], µm . | Zeta Potential, mV . | ||||||
---|---|---|---|---|---|---|---|---|
0th Day . | 3rd Day . | 7th Day . | 10th Day . | 0th Day . | 3rd Day . | 7th Day . | 10th Day . | |
FPEC-EUG emulsions | ||||||||
0.25% | 1.99 ± 0.28c,C | 3.46 ± 0.07d,B | 3.52 ± 0.83c,B | 4.65 ± 0.32c,A | −9.48 ± 1.22c,A | −9.57 ± 0.80bc,A | −10.93 ± 5.40b,AB | −14.96 ± 1.19b,B |
1% | 2.02 ± 0.29c,B | 3.89 ± 0.09d,A | 3.91 ± 0.72c,A | 4.52 ± 0.87c,A | −5.64 ± 0.77b,A | −6.17 ± 0ab,A | −5.65 ± 0a,A | −5.35 ± 0.89a,A |
2% | 2.31 ± 0.64c,B | 4.19 ± 0.38d,A | 3.96 ± 0.44c,A | 4.55 ± 0.51c,A | −2.12 ± 0.49a,A | −3.34 ± 0.57a,A | −6.51 ± 1.21ab,B | −7.50 ± 1.67a,B |
CPEC-EUG emulsions | ||||||||
0.25% | 6.93 ± 0.4b,AB | 7.15 ± 0.36c,A | 6.60 ± 0.82b,AB | 6.11 ± 0.98b,B | −17.34 ± 0.28d,B | −16.23 ± 0.21d,A | −16.43 ± 0.27c,A | −16.44 ± 0.24b,A |
1% | 8.41 ± 3.08b,A | 8.96 ± 1.87b,A | 6.92 ± 1.12b,A | 7.18 ± 1.19ab,A | −19.06 ± 2.79d,A | −20.07 ± 3.80e,A | −19.24 ± 2.73c,A | −20.26 ± 4.10c,A |
2% | 11.22 ± 2.76a,A | 10.08 ± 0.75a,AB | 8.99 ± 1.61a,AB | 8.70 ± 1.70a,B | −10.29 ± 2.51c,A | −13.02 ± 2.85cd,AB | −16.30 ± 1.15c,BC | −18.27 ± 2.42bc,C |
a–e and A–C values followed by different letters at each column and row indicate statistically significant differences (P ≤ 0.05), respectively.
Antilisterial activity of films in zone inhibition test
The results of the zone inhibition tests with films containing EUG between 0.25% and 2% against L. innocua are displayed in Figure 1. The minimum amounts of EUG in films that yielded clear zones around the discs of FPEC and CPEC films were 0.25% and 0.5%, respectively. However, the clear zones at the indicated EUG concentrations were observed only in 8 out of 18, and 14 out of 18 discs tested for FPEC and CPEC films, respectively, due to the fact that the inhibitory concentration was not reached in a homogeneous manner all around the discs. However, the ‘no zone discs’ were eliminated when EUG concentrations were increased. The FPEC-EUG films formed significantly greater zone areas (5 and 1.6 fold) than CPEC-EUG films at 0.5% and 1% EUG concentrations, respectively. Thus, it is clear that FPEC-EUG emulsion-based films performed better than CPEC-EUG films in terms of EUG release at the test conditions used in the experiment. However, both CPEC-EUG and FPEC-EUG films showed similar antimicrobial activities when the concentration of the essential oil was increased to 2%, a critical concentration that provided an excessive amount of EUG in both films for effective inactivation of Listeria. Thus, the optimal EUG concentration of 2% was used in films tested on inoculated melons.
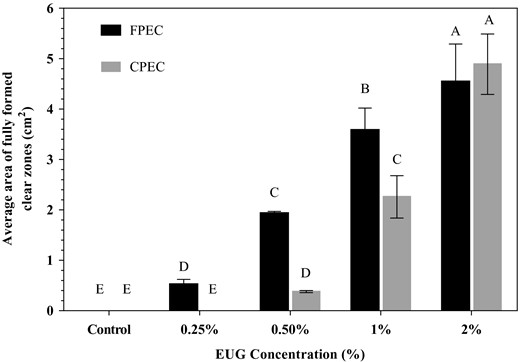
Zone-inhibition-based antimicrobial activity of FPEC-EUG and CPEC-EUG films on Listeria innocua. Data with different letters are significantly different (P ≤ 0.05).
Antilisterial activity of films at inoculated webbed melon surfaces
The results of L. innocua counts conducted at the beginning and at the end of 1-week cold storage (at 10 °C) for uncoated, and FPEC-, CPEC-, FPEC-EUG-, and CPEC-EUG-coated inoculated webbed melons are presented in Figure 2. The listerial counts of uncoated control melons and control CPEC-coated melons were not significantly different at 0th day (P > 0.05) while CPEC-coated melons showed significantly lower listerial counts at 7th day of cold storage (P < 0.05). In contrast, it is important to note that melons coated with control FPEC films gave significantly lower listerial counts than uncoated controls at both 0th and 7th days of cold storage (P < 0.05). This finding suggested that the pectin extracted from sun-dried fig wastes showed an inherent antimicrobial activity that might originate from the antimicrobial activity of polyphenols associated with this hydrocolloid (Amessis-Ouchemoukh et al., 2017). Polysaccharides like pectin interact with polyphenols via their polar groups (e.g. acetal, hydroxyl or carboxyl) and bind phenolic –OH groups with H-bonds and van der Waals forces (Palafox-Carlos et al., 2011). Thus, polyphenols could not be separated effectively from pectin by classical ethanol precipitation and washing cycles applied during pectin purification. Gharibzahedi et al. (2019) reported that pectin from peels of fresh common figs contained 3 g GAE equivalents of total phenols per 100 g of pectin (d.w.). In the current study, the total phenolic content of extracted FPEC was almost twofold higher than that of CPEC (as 1.03 and 0.55 g GAE per 100 g the of pectin (d.w.), respectively). Thus, these results supported that the inherent antimicrobial activity of FPEC control film could be due to its high polyphenol content. Meanwhile, the antimicrobial activity on coated melons against L. innocua increased significantly by the application of pectin–EUG coatings. It is important to note that on the 0th day, the listerial counts of melon surfaces coated with CPEC-EUG and FPEC-EUG films were 1.5 and 1.3 decimals (D) lower than the listerial counts obtained from uncoated melon surfaces, respectively. Moreover, the decimal differences between Listeria counts of uncoated and CPEC-EUG or FPEC-EUG film-coated samples increased to 2.7 and 2.2 D by 1-week cold storage, respectively. Although the listerial counts of melons coated with CPEC-EUG were significantly lower than those coated with FPEC-EUG at the end of 7th day (P ≤ 0.05), the antimicrobial performance on webbed-rind melon surfaces of the pectin coatings from different origin was comparable. After 1-week of storage, the sharp smell of EUG in melons with CPEC-EUG and FPEC-EUG coatings reduced considerably due to the evaporation of essential oil from the pectin films. Thus, it seemed that the increased decimal reduction in Listeria by storage was related mainly to the death of bacteria damaged at the initial stages of the coating when pectin films had contained high concentrations of EUG. Further studies are needed to determine the kinetics of EUG loss from both types of pectin films at different temperatures, and to understand the duration of antimicrobial effectiveness following application of films.
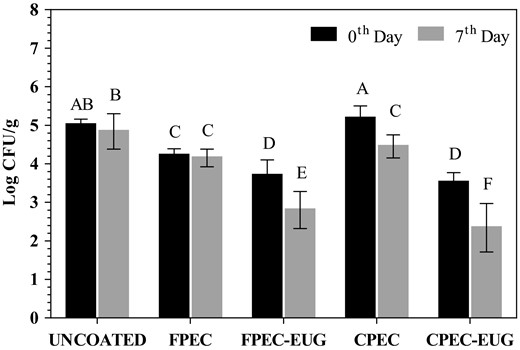
Antimicrobial effects of FPEC-EUG and CPEC-EUG coatings with 2% EUG on Listeria innocua inoculated onto whole Galia melons during cold storage at 10 °C. Data with different letters are significantly different (P ≤ 0.05).
In the literature, studies related to the use of pectin coatings with essential oils on whole webbed-rind melons are scarce. However, Boyacı et al. (2019) reported that zein films with 2% EUG caused almost 3.3 D reduction in listerial counts of some melon cultivars (Santa Claus and Crenshaw) having smooth to slightly rough rind surface within 1 week of cold storage. This result indicated the possibility that the pectin emulsion coatings with EUG are slightly less effective on whole melon surfaces than zein coatings with EUG. However, both films should be compared for the same film thickness on webbed-rind melons that provide a better hiding and growth medium for Listeria than smooth rind melon cultivars (Behrsing et al., 2003). Ma et al. (2016) also obtained >3 log CFU cm−2 reduction of E. coli O157:H7 and Listeria monocytogenes on whole cantaloupes immediately after applying chitosan coating with 0.1% lauric arginate, 0.1% ethylenediaminetetraacetic acid and 1% cinnamon oil on their surface. Moreover, Zhang et al. (2015) also achieved effective inhibition of different pathogens including Listeria monocytogenes on cantaloupes using alginate films with 2% cinnamon bark oil. All these studies clearly showed the good potential of using antimicrobial coatings to increase the safety of whole webbed-rind melons. However, further studies are needed to determine the long-term effects of edible coatings on the quality attributes (e.g. surface colour, texture and sensory properties) of melons.
Mechanical properties of films
Mechanical properties of films obtained from CPEC-EUG and FPEC-EUG emulsions at different EUG concentrations are shown in Table 3. At all conditions, CPEC and CPEC-EUG films showed significantly higher tensile strengths (17.9 to 51-fold) and Young's modulus than FPEC and FPEC-EUG films. However, it is important to note that the FPEC films were much more flexible, and they showed 2.4- to 4.3-fold higher elongation at break than CPEC films. It should also be reported that the increase of EUG concentration between 1% and 3% did not cause a considerable change in the mechanical properties of both types of films. These results clearly showed the different film making characteristics of CPEC and FPEC.
Mechanical properties of FPEC and CPEC films at different EUG concentrations
EUG (%, w/w) . | Tensile strength (MPa) . | Elongation at break (%) . | Young's modulus (MPa) . | Film thickness (µm) . |
---|---|---|---|---|
FPEC films | ||||
– | 0.53 ± 0.10a | 27.44 ± 1.01a | 0.03 ± 0.01a | 83.7 ± 6.30d |
1 | 0.39 ± 0.06a | 24.58 ± 1.93ab | 0.024 ± 0a | 102.3 ± 4.41c |
2 | 0.22 ± 0.01b | 21.43 ± 0.77b | 0.012 ± 0b | 118.0 ± 6.22b |
3 | 0.24 ± 0.02b | 22.15 ± 1.65b | 0.012 ± 0b | 132.6 ± 0.98a |
CPEC films | ||||
– | 9.49 ± 0.93B | 6.35 ± 1.64A | 3.72 ± 0.48BC | 81.08 ± 17.41C |
1 | 14.17 ± 0.86A | 8.87 ± 1.35A | 4.78 ± 0.05A | 102.39 ± 2.76BC |
2 | 8.55 ± 0.86B | 8.87 ± 3.83A | 3.01 ± 0.27C | 122.81 ± 5.50AB |
3 | 12.25 ± 0.66A | 7.11 ± 0.86A | 4.34 ± 0.09AB | 146.90 ± 9.88A |
EUG (%, w/w) . | Tensile strength (MPa) . | Elongation at break (%) . | Young's modulus (MPa) . | Film thickness (µm) . |
---|---|---|---|---|
FPEC films | ||||
– | 0.53 ± 0.10a | 27.44 ± 1.01a | 0.03 ± 0.01a | 83.7 ± 6.30d |
1 | 0.39 ± 0.06a | 24.58 ± 1.93ab | 0.024 ± 0a | 102.3 ± 4.41c |
2 | 0.22 ± 0.01b | 21.43 ± 0.77b | 0.012 ± 0b | 118.0 ± 6.22b |
3 | 0.24 ± 0.02b | 22.15 ± 1.65b | 0.012 ± 0b | 132.6 ± 0.98a |
CPEC films | ||||
– | 9.49 ± 0.93B | 6.35 ± 1.64A | 3.72 ± 0.48BC | 81.08 ± 17.41C |
1 | 14.17 ± 0.86A | 8.87 ± 1.35A | 4.78 ± 0.05A | 102.39 ± 2.76BC |
2 | 8.55 ± 0.86B | 8.87 ± 3.83A | 3.01 ± 0.27C | 122.81 ± 5.50AB |
3 | 12.25 ± 0.66A | 7.11 ± 0.86A | 4.34 ± 0.09AB | 146.90 ± 9.88A |
a–d and A–C at each column denote a statistically significant difference separately for FPEC and CPEC data, respectively (P ≤ 0.05).
Mechanical properties of FPEC and CPEC films at different EUG concentrations
EUG (%, w/w) . | Tensile strength (MPa) . | Elongation at break (%) . | Young's modulus (MPa) . | Film thickness (µm) . |
---|---|---|---|---|
FPEC films | ||||
– | 0.53 ± 0.10a | 27.44 ± 1.01a | 0.03 ± 0.01a | 83.7 ± 6.30d |
1 | 0.39 ± 0.06a | 24.58 ± 1.93ab | 0.024 ± 0a | 102.3 ± 4.41c |
2 | 0.22 ± 0.01b | 21.43 ± 0.77b | 0.012 ± 0b | 118.0 ± 6.22b |
3 | 0.24 ± 0.02b | 22.15 ± 1.65b | 0.012 ± 0b | 132.6 ± 0.98a |
CPEC films | ||||
– | 9.49 ± 0.93B | 6.35 ± 1.64A | 3.72 ± 0.48BC | 81.08 ± 17.41C |
1 | 14.17 ± 0.86A | 8.87 ± 1.35A | 4.78 ± 0.05A | 102.39 ± 2.76BC |
2 | 8.55 ± 0.86B | 8.87 ± 3.83A | 3.01 ± 0.27C | 122.81 ± 5.50AB |
3 | 12.25 ± 0.66A | 7.11 ± 0.86A | 4.34 ± 0.09AB | 146.90 ± 9.88A |
EUG (%, w/w) . | Tensile strength (MPa) . | Elongation at break (%) . | Young's modulus (MPa) . | Film thickness (µm) . |
---|---|---|---|---|
FPEC films | ||||
– | 0.53 ± 0.10a | 27.44 ± 1.01a | 0.03 ± 0.01a | 83.7 ± 6.30d |
1 | 0.39 ± 0.06a | 24.58 ± 1.93ab | 0.024 ± 0a | 102.3 ± 4.41c |
2 | 0.22 ± 0.01b | 21.43 ± 0.77b | 0.012 ± 0b | 118.0 ± 6.22b |
3 | 0.24 ± 0.02b | 22.15 ± 1.65b | 0.012 ± 0b | 132.6 ± 0.98a |
CPEC films | ||||
– | 9.49 ± 0.93B | 6.35 ± 1.64A | 3.72 ± 0.48BC | 81.08 ± 17.41C |
1 | 14.17 ± 0.86A | 8.87 ± 1.35A | 4.78 ± 0.05A | 102.39 ± 2.76BC |
2 | 8.55 ± 0.86B | 8.87 ± 3.83A | 3.01 ± 0.27C | 122.81 ± 5.50AB |
3 | 12.25 ± 0.66A | 7.11 ± 0.86A | 4.34 ± 0.09AB | 146.90 ± 9.88A |
a–d and A–C at each column denote a statistically significant difference separately for FPEC and CPEC data, respectively (P ≤ 0.05).
Morphology of pectin films
Scanning Electron Microscopy micrographs of cross-sectional morphology for FPEC and CPEC films with and without EUG are shown in Figure 3. Control films prepared with FPEC and CPEC both had a dense appearance (Fig. 3a and b). However, control CPEC films exhibited a more uniform morphology than control FPEC films. FPEC films, in particular, contained some aggregates, possibly arising from insoluble proteins originated from seeds of fig fruit. In contrast, FPEC-EUG and CPEC-EUG films revealed a morphology characterised by an extensive distribution of droplets and void pores (Fig. 3c and d). It appeared that part of the EUG remained in the films as emulsion droplets, while some other EUG droplets evaporated to form some voids (pores). Noteworthy, the EUG emulsion droplets were distributed homogenously along the film matrix, with no signs of accumulation close to the film surface. Nisar et al. (2018) observed similar morphologies in citrus pectin films incorporated with clove bud essential oil (CEO). However, these authors observed coalescence of CEO droplets close to the film surface, which was ascribed to phase separation between pectin and the essential oil, as CEO concentration was increased from 0.5% to 1% or 1.5% (Nisar et al., 2018).
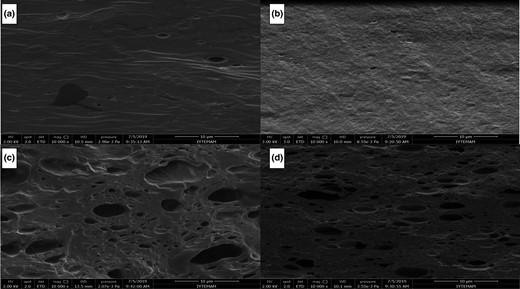
Effect of EUG on the cross-sectional morphology of pectin films: (a) FPEC; (b) CPEC; (c) FPEC-EUG (2%); and (d) CPEC-EUG (2%) (Magnification: 10 000×).
Surface wettability of films
The results of the contact angle analysis are summarised in Table 4. The films of pectin exhibited a completely different behaviour depending on the origin. Pristine FPEC films showed a typical wetting behaviour (θ ~ 27°); that is, water molecules rapidly interacted with the film surface due to high chemical affinity. The marked polar character owing to both the properties of the galacturonic skeleton (side chain frequency, sugar profile and chain length) and the protein fraction drove water molecules to spread on the surface as soon as the droplet touched the surface, insomuch as, after a few seconds, θ ~ 10° (Movie S1). As expected, the addition of EUG yielded a significant increase in θ (~54°) due to the hydrophobic character of EUG that made the FPEC film surface more apolar. In turn, this reduced the intensity of the spreading phenomenon of water molecules on the film surface (see Movie S2).
Optical contact angle (OCA) and oxygen transmission rate (OTR) of different pristine and EUG-loaded pectin films
Film . | EUG conc. (%, w/w) . | OCA (θ, °) . | OTR (mL m−2 24 h−1) . |
---|---|---|---|
FPEC | – | 26.8 ± 4.2a | 14.149 ± 1.387a |
FPEC | 2 | 53.9 ± 1.4b | 47.258 ± 5.861b |
CPEC | – | 82.9 ± 5.6A | 2.975 ± 0.341A |
CPEC | 2 | 73.5 ± 4.7B | 12.678 ± 1.504B |
Film . | EUG conc. (%, w/w) . | OCA (θ, °) . | OTR (mL m−2 24 h−1) . |
---|---|---|---|
FPEC | – | 26.8 ± 4.2a | 14.149 ± 1.387a |
FPEC | 2 | 53.9 ± 1.4b | 47.258 ± 5.861b |
CPEC | – | 82.9 ± 5.6A | 2.975 ± 0.341A |
CPEC | 2 | 73.5 ± 4.7B | 12.678 ± 1.504B |
a–b and A–B at each column denote a statistically significant difference separately for FPEC and CPEC data, respectively (P ≤ 0.05).
Optical contact angle (OCA) and oxygen transmission rate (OTR) of different pristine and EUG-loaded pectin films
Film . | EUG conc. (%, w/w) . | OCA (θ, °) . | OTR (mL m−2 24 h−1) . |
---|---|---|---|
FPEC | – | 26.8 ± 4.2a | 14.149 ± 1.387a |
FPEC | 2 | 53.9 ± 1.4b | 47.258 ± 5.861b |
CPEC | – | 82.9 ± 5.6A | 2.975 ± 0.341A |
CPEC | 2 | 73.5 ± 4.7B | 12.678 ± 1.504B |
Film . | EUG conc. (%, w/w) . | OCA (θ, °) . | OTR (mL m−2 24 h−1) . |
---|---|---|---|
FPEC | – | 26.8 ± 4.2a | 14.149 ± 1.387a |
FPEC | 2 | 53.9 ± 1.4b | 47.258 ± 5.861b |
CPEC | – | 82.9 ± 5.6A | 2.975 ± 0.341A |
CPEC | 2 | 73.5 ± 4.7B | 12.678 ± 1.504B |
a–b and A–B at each column denote a statistically significant difference separately for FPEC and CPEC data, respectively (P ≤ 0.05).
A completely different scenario was observed for the films of pectin derived from citrus. Oppositely to the pectin films from fig, CPEC films had an unexpectedly high water contact angle (θ ~ 83°). In addition, absorption rather than spreading of water molecules occurred on the surface (see Figure S1 and Movie S3). A plausible explanation for this behaviour can be found in the composition of this type of pectin. Pectin from citrus had a high GA content (≥79%). It appeared that this played a role in the structural organisation of pectin molecules, with most likely an extensive hydrogen bonding at intermolecular level, which yielded a dense, less permeable network. For this reason, water molecules were less prone to wet the film surface compared to the surface of fig pectin films. In the case of fig pectin, the lower GA content (20.16%), but higher protein content than citrus pectin, probably did not allow the formation of a dense and stable organisation at a molecular level. The addition of EUG (CPEC-EUG) led to a significant decrease of θ (~73°) (see Movie S4). In line with the above considerations, it is plausible that the antimicrobial agent somehow perturbed the original CPEC network organisation working as a pseudo-plasticiser, thereby disrupting (at least partly) the inherent hydrogen-bonding pattern in the pristine samples.
Oxygen permeability characteristics of films
The different chemical composition and physical properties of films obtained from citrus and fig pectins were also reflected in the barrier properties against oxygen. As shown in Table 4, the best barrier performance was observed for the CPEC sample, with an average OTR value slightly below 3 mL m‒2 24 h‒1). This low OTR value can be ascribed to the extensive hydrogen bonds formed by carboxyl and hydroxyl groups of GA units in citrus pectin film. The addition of EUG (CPEC-EUG) impaired the above performance due to the negative effect on the inherent organisation as well as because EUG has a hydrophobic character, leading to a final OTR value of ~13 mL m‒2 24 h‒1.
Films obtained from fig pectin showed a lower barrier performance against oxygen. Pristine FPEC films had an average OTR value of ~14 mL m‒2 24 h‒1, very similar to the OTR value of CPEC films with EUG. The lower OTR performance of pristine films of FPEC compared to CPEC can be explained in consideration of the low GA content and high amount of denatured protein fraction of FPEC. All of these parameters contribute to a lower barrier performance due to a less extensive hydrogen bonding among fig pectin molecules at intra- and intermolecular level. After the addition of EUG, FPEC films exhibited an OTR value more than three times higher than the pristine films. Also, in this case, EUG acted alike a plasticiser; that is, it worsened the original hydrogen-bonding pattern and increased the free volume of the main biopolymer phase, hence allowing a higher oxygen flux across the film thickness. From a practical point of view, coatings obtained from fig pectin might be more suitable for the intended fruit coating application because the deposition of the coating, while slowing down the respiration rate of the fruit, could still allow oxygen to diffuse into the food, thus avoiding initiation of anaerobic respiration of the product.
Conclusions
This study clearly showed the good potential of pectin–EUG emulsion-based edible coatings to reduce the risks associated with contaminated Listeria on webbed-rind melons. The antimicrobial emulsions developed using pectin extracted from sun-dried fig processing wastes and commercial citrus pectin showed similar antilisterial effectiveness on coated webbed-rind melons. However, fig pectin films differed from citrus pectin films in terms of critical EUG concentrations to achieve antimicrobial properties, and different physical, mechanical and gas barrier properties that could be exploited to increase the applicability of the developed films on alternative melon cultivars (or crops) that had variable rind characteristics and physiological properties. This work is an example of the valorisation of agro-industrial wastes to obtain alternative food hydrocolloids with different functional and technological characteristics.
Acknowledgments
The pectin production part of this study was funded by The Scientific and Technical Research Council of Turkey (TÜBİTAK, Project no: 118 O 372). We thank the Materials Research Center and Biotechnology and Bioengineering Research and Applications Center in Izmir Institute of Technology for the generous use of their facilities during the use of SEM, and zeta potential/particle size analysis, respectively. Dr. Stefano Farris is contributed to surface wettability and OTR measurements while other experimental parts were conducted by the Ph.D. student Elif Çavdaroğlu.
Ethical guidelines
Ethics approval was not required for this research.
Data availability statement
Research data are not shared.
Conflict of interest
There are no conflicts of interest in this study.
References
Author notes
The peer review history for this article is available at https://publons.com/publon/10.1111/ijfs.14458