-
PDF
- Split View
-
Views
-
Cite
Cite
Wen Han, Sen Ma, Li Li, Xueling Zheng, Xiaoxi Wang, Rheological properties of gluten and gluten-starch model doughs containing wheat bran dietary fibre, International Journal of Food Science and Technology, Volume 53, Issue 12, December 2018, Pages 2650–2656, https://doi.org/10.1111/ijfs.13861
- Share Icon Share
Abstract
The effect of wheat bran dietary fibre (WBDF) on the texture and extensional properties of gluten (G) as well as gluten-starch (G+S) doughs were investigated. Texture analysis revealed an increasing hardness of WBDF-enriched doughs, ascribed to the gradual formation of intermolecular hydrogen bonds and weakened aggregation behaviour of gluten proteins. Extension results showed that G and G+S systems exhibited growing extension resistance thanks to the strengthened strain-hardening capacity induced by WBDF. On the other hand, the WBDF accelerated the rupture of the gluten network and led to a downtrend of dough extensibility by increasing the friction with gluten branches and impairing the uniformity of the network structure. The findings indicate the possible impairment of the dough texture properties upon the addition of WBDF. Accordingly, for bran-enriched flour products, the pretreatment with bran-like particles leading to size reduction, softening or solubility improvement may be advantageous.
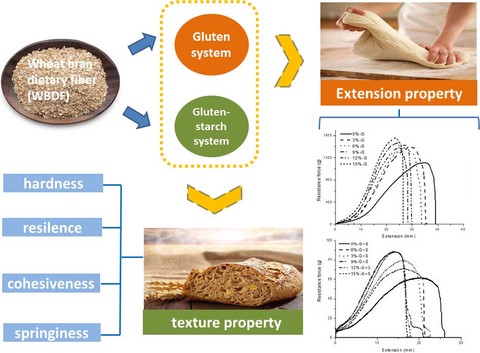
Introduction
Increased daily consumption of whole grain products is believed to have broad health benefits, as most of the nutrients are concentrated in the outer layers of the grain which constitute wheat bran (Betoret et al., 2011; Li et al., 2016; Lv et al., 2017). However, low consumer acceptance of whole grain products was observed when compared to the fine flour products due to the high content of dietary fibres (DFs) in the wheat bran (Liu et al., 2015; Grigor et al., 2016). Various studies have revealed that wheat bran-enriched foods with undesirable texture and taste associated with the dark colour, rough crumb texture and small loaf volume were responsible for the limited consumption of whole grain (Bakke & Vickers, 2007; Collar et al., 2007). Therefore, to address the consumption problem of whole grain products, the adverse effects of wheat bran dietary fibre (WBDF) on dough should be studied in depth.
The dough texture properties play an important role in determining the overall acceptance of flour products, such as bread (McCann et al., 2016). During the dough-kneading stage, the dough undergoes different mechanical deformations, which mainly include compression and extension. The extensional property of dough is primarily governed by the polymeric network of gluten proteins, which is influenced by the content and composition characteristics of gluten, such as molecular dimension, the entanglement and aggregation of chains, as well as the ratio of glutenin to gliadin (Singh & MacRitchie, 2001). Regarding the effect of DFs on dough extensibility, different conclusions have been reached. Generally, there are three patterns: the dough extensibility decreases with DF addition, the dough extensibility increases with DF addition, and the dough extensibility first increases and then decreases with DF addition (Skendi et al., 2010; Ahmed, 2015). The discrepancies may be attributed to the flour quality as well as the variety and level of added DF.
Additionally, texture properties, such as hardness, cohesiveness, springiness and resilience have also been closely associated with the end product quality. Numerous studies have revealed that the addition of DFs is more likely to produce doughs with harder texture and less springiness (Bleis et al., 2015; Wang et al., 2017a,b). Studies have indicated that the interactions among gluten, starch and DFs may be one of the major factors responsible for the quality of the flour product (Abang Zaidel et al., 2008; Chen et al., 2011; Fan et al., 2016). It has also been established that the bread quality is directly linked with the gluten conformation and aggregation properties, as significant correlations have been found between gluten macropolymer quantity and the rheological properties of dough (Wang et al., 2007; Nawrocka et al., 2017). In addition, one of the secondary structures of gluten, namely β-spiral, is considered to impart dough elasticity (Tatham et al., 1985). Upon wheat bran addition, such unique structure may turn into β-sheets, which can result in the impaired dough springiness (Bock & Damodaran, 2013). Thus, it is plausible that the effects of DF on the dough rheological properties may be complex and intertwined.
Accordingly, the determination of the WBDF-induced tensile and texture properties of doughs is of great importance to study and evaluate the quality of the final products. Moreover, additional underlying interactions between DF and gluten matrix should be investigated to further understand the possible factors that induce the rheological properties changes. The present study is based on earlier studies and continues the in-depth study of the textural and extensional properties of WBDF-enriched doughs as these two indices are good indicators of the end product quality. Moreover, the mechanism underlying the influence of the gluten secondary structure ion the dough rheological behaviour has not been widely studied. Thus, based on the results, the plausible mechanisms for the effects of WBDF on the gluten network structure with and without starch were also considered together to further understand the possible relationship between gluten structural changes and the dough properties. Our ultimate aim was to improve the utilisation of wheat bran and promote the acceptability of DF-enriched products.
Material and methods
Materials
Commercial wheat bran (15.32% protein, 10.17% starch, 6.64% ash, 14.21% moisture), wheat starch (0.319% protein, 90.11% starch, 0.213% ash, 14.48% moisture) and wheat gluten (73.64% protein, 8.48% starch, 1.26% ash, 12.07% moisture,) were purchased from a local market.
Wheat bran dietary fibre preparation
Wheat bran was pulverised to particles of about 420 μm using a sieve. Then, 80 g of wheat bran powder was blended with 800 mL of distilled water in a colloid mill. The suspension was washed under running water to remove the starch fraction and the water soluble dietary fibre. Next, 800 mL of distilled water was added and the suspension was heated at 95 °C for 30 min, followed by adjustment of the pH to 5.6. Afterwards, 1.5% (w/w) of thermostable α-amylase (40 000 U g−1) was added to the slurry and incubated for 30 min at 95 °C. Subsequently, 3% (w/w) of alkaline protease (≥200 000 U g−1) was added to the slurry and incubated for 2 h after cooling the it to 50 °C and adjusting the pH to 9.0. The supernatant was discarded, and the residues were washed repeatedly under running water until the solution was no longer turbid. Ultimately, the solid residues were dried for 24 h in an oven at 60 °C. The prepared WBDF was obtained after pulverising the mixture to 150 μm in particle size. The purity of the WBDF was assessed using a total dietary fiber assay kit (K-TDFR, Megazyme, Bray Co., Wicklow, Ireland). The total dietary fibre content was 90.43%, including 5.76% soluble dietary fibre and 84.67% insoluble dietary fibre. The impurities consisted mainly of protein (4.72%) and starch (4.12%), measured according to Kjeldahl method using Kjeltec 8400 (Foss, Hoganas, Sweden) and AACC Method 76-11.
Wheat gluten preparation
The G system is composed of only wheat gluten, while the G+S system is a mixture of wheat gluten and wheat starch with a constant weight proportion 15:80 (on the same moisture basis) (Nawrocka et al., 2016). The two systems were prepared with intention of figuring out the distinctions between WBDF-gluten dough and WBDF-starch-gluten dough. The WBDF contents were 0, 3, 6, 9, 12 and 15% (w/w) in relation to gluten weight (on the same moisture basis). The ingredients were mixed and kneaded into a dough with 50% moisture using a pin mixer for 3 min. Samples of the obtained dough of the G and G+S systems were used in the subsequent tests.
Texture analysis
The texture profile analysis (TPA) was performed according to previous study, with slight modifications (Gao et al., 2016; Peng et al., 2017). The dough was developed and formed into a cylinder with the height of 15 mm and a diameter of 20 mm using a columniform Teflon mould. Each dough with different levels of added WBDF was prepared for three pieces. The TPA was performed using a TA.XT Plus Analyzer (Stable Micro Systems Ltd., Godalming, Surrey, UK). During the test, the dough was compressed twice up to 70% of the original height with the P/36R probe. The testing parameters were: target mode, strain; pause duration, 5 s; pretest speed, 3.0 mm s−1; test speed, 1.0 mm s−1; posttest speed, 1.0 mm s−1; trigger force, 5 g. The test speed was set as 1 mm s−1 and the trigger force was 5.0 g. A force-time curve was acquired from the program. Three replicates of the twelve dough samples were analysed and averaged. Four indices, including hardness, resilience, cohesiveness and springiness were selected to reflect the texture properties of the dough.
Extension test
For the micro-scale extension test, the G and G+S dough with different levels of added WBDF were prepared. All the dough samples were shaped manually into a roll according to Scherf & Koehler (2018). The dough extension test was conducted on a TA-XT2 Texture Analyzer (Stable Micro Systems, Ltd.). The resistance to extension (g) and extensibility (mm) were determined in tension mode by recording the peak force and the distance at the maximum and the extension limit. The test settings were as follows: pretest speed: 2.0 mm s−1; test speed: 3.3 mm s−1; posttest speed: 10 mm s−1, distance: 60 mm; trigger force: 5 g. All measurements were performed in triplicate for twelve independently prepared doughs.
Results and discussions
Texture profile analysis of dough
The effect of WBDF on the textural properties of the G and G+S doughs was examined and is presented in Table 1. In general, the hardness of both G and G+S systems increased with the increase of the level of WBDF. The texture properties are more likely to be determined by the secondary changes induced by the WBDF. Based on the findings of the gluten secondary structure changes as a result of wheat bran addition from 0 to 10%, wheat bran assisted in promoting a more ordered structure as evidenced by the increase in the content of β-sheet structure (Bock & Damodaran, 2013). Other studies also added to the growing evidence by proving the formation of intermolecular hydrogen bonds that lead to a more rigid structure (Tatham et al., 1985). It is well known that the β-sheet is formed via intermolecular hydrogen bonds. Therefore, it is plausible that the increasingly dominant β-sheet conformation strengthened the hardness of the WBDF-enriched dough samples.
WBDF content (%) . | G . | G+S . | ||||||
---|---|---|---|---|---|---|---|---|
Hardness (g) . | Resilence (%) . | Cohesiveness . | Springiness (%) . | Hardness (g) . | Resilence (%) . | Cohesiveness . | Springiness (%) . | |
0 | 3914.64 ± 50.85a | 17.60 ± 0.42a | 0.81 ± 0.01bc | 62.94 ± 1.63a | 1193.66 ± 54.43a | 4.24 ± 0.05a | 0.50 ± 0.06a | 51.71 ± 3.06a |
3 | 4082.60 ± 37.03ab | 17.34 ± 0.78a | 0.83 ± 0.01cd | 70.00 ± 0.96a | 1236.94 ± 39.69a | 4.22 ± 0.30a | 0.48 ± 0.22a | 48.22 ± 2.03ab |
6 | 4422.17 ± 51.57ab | 17.09 ± 0.01a | 0.86 ± 0.01d | 78.69 ± 4.25a | 1260.93 ± 17.9ab | 4.40 ± 0.27ab | 0.36 ± 0.00a | 44.86 ± 0.16ab |
9 | 5025.55 ± 167.72b | 17.16 ± 0.29a | 0.78 ± 0.02b | 69.37 ± 0.35a | 1277.12 ± 53.54ab | 4.67 ± 0.09ab | 0.38 ± 0.01a | 39.23 ± 2.28b |
12 | 6457.43 ± 95.91c | 16.75 ± 0.04a | 0.72 ± 0.00a | 63.86 ± 2.35a | 1543.07 ± 4.67b | 4.62 ± 0.01ab | 0.30 ± 0.03a | 23.60 ± 2.45c |
15 | 7308.72 ± 104.57c | 16.84 ± 0.11a | 0.70 ± 0.02a | 64.56 ± 4.26a | 2333.50 ± 13.09c | 4.85 ± 0.25b | 0.27 ± 0.02a | 19.69 ± 0.66c |
WBDF content (%) . | G . | G+S . | ||||||
---|---|---|---|---|---|---|---|---|
Hardness (g) . | Resilence (%) . | Cohesiveness . | Springiness (%) . | Hardness (g) . | Resilence (%) . | Cohesiveness . | Springiness (%) . | |
0 | 3914.64 ± 50.85a | 17.60 ± 0.42a | 0.81 ± 0.01bc | 62.94 ± 1.63a | 1193.66 ± 54.43a | 4.24 ± 0.05a | 0.50 ± 0.06a | 51.71 ± 3.06a |
3 | 4082.60 ± 37.03ab | 17.34 ± 0.78a | 0.83 ± 0.01cd | 70.00 ± 0.96a | 1236.94 ± 39.69a | 4.22 ± 0.30a | 0.48 ± 0.22a | 48.22 ± 2.03ab |
6 | 4422.17 ± 51.57ab | 17.09 ± 0.01a | 0.86 ± 0.01d | 78.69 ± 4.25a | 1260.93 ± 17.9ab | 4.40 ± 0.27ab | 0.36 ± 0.00a | 44.86 ± 0.16ab |
9 | 5025.55 ± 167.72b | 17.16 ± 0.29a | 0.78 ± 0.02b | 69.37 ± 0.35a | 1277.12 ± 53.54ab | 4.67 ± 0.09ab | 0.38 ± 0.01a | 39.23 ± 2.28b |
12 | 6457.43 ± 95.91c | 16.75 ± 0.04a | 0.72 ± 0.00a | 63.86 ± 2.35a | 1543.07 ± 4.67b | 4.62 ± 0.01ab | 0.30 ± 0.03a | 23.60 ± 2.45c |
15 | 7308.72 ± 104.57c | 16.84 ± 0.11a | 0.70 ± 0.02a | 64.56 ± 4.26a | 2333.50 ± 13.09c | 4.85 ± 0.25b | 0.27 ± 0.02a | 19.69 ± 0.66c |
WBDF: wheat bran dietary fibre; Values for a particular column followed by different letters differ superscript significantly (P < 0.05).
WBDF content (%) . | G . | G+S . | ||||||
---|---|---|---|---|---|---|---|---|
Hardness (g) . | Resilence (%) . | Cohesiveness . | Springiness (%) . | Hardness (g) . | Resilence (%) . | Cohesiveness . | Springiness (%) . | |
0 | 3914.64 ± 50.85a | 17.60 ± 0.42a | 0.81 ± 0.01bc | 62.94 ± 1.63a | 1193.66 ± 54.43a | 4.24 ± 0.05a | 0.50 ± 0.06a | 51.71 ± 3.06a |
3 | 4082.60 ± 37.03ab | 17.34 ± 0.78a | 0.83 ± 0.01cd | 70.00 ± 0.96a | 1236.94 ± 39.69a | 4.22 ± 0.30a | 0.48 ± 0.22a | 48.22 ± 2.03ab |
6 | 4422.17 ± 51.57ab | 17.09 ± 0.01a | 0.86 ± 0.01d | 78.69 ± 4.25a | 1260.93 ± 17.9ab | 4.40 ± 0.27ab | 0.36 ± 0.00a | 44.86 ± 0.16ab |
9 | 5025.55 ± 167.72b | 17.16 ± 0.29a | 0.78 ± 0.02b | 69.37 ± 0.35a | 1277.12 ± 53.54ab | 4.67 ± 0.09ab | 0.38 ± 0.01a | 39.23 ± 2.28b |
12 | 6457.43 ± 95.91c | 16.75 ± 0.04a | 0.72 ± 0.00a | 63.86 ± 2.35a | 1543.07 ± 4.67b | 4.62 ± 0.01ab | 0.30 ± 0.03a | 23.60 ± 2.45c |
15 | 7308.72 ± 104.57c | 16.84 ± 0.11a | 0.70 ± 0.02a | 64.56 ± 4.26a | 2333.50 ± 13.09c | 4.85 ± 0.25b | 0.27 ± 0.02a | 19.69 ± 0.66c |
WBDF content (%) . | G . | G+S . | ||||||
---|---|---|---|---|---|---|---|---|
Hardness (g) . | Resilence (%) . | Cohesiveness . | Springiness (%) . | Hardness (g) . | Resilence (%) . | Cohesiveness . | Springiness (%) . | |
0 | 3914.64 ± 50.85a | 17.60 ± 0.42a | 0.81 ± 0.01bc | 62.94 ± 1.63a | 1193.66 ± 54.43a | 4.24 ± 0.05a | 0.50 ± 0.06a | 51.71 ± 3.06a |
3 | 4082.60 ± 37.03ab | 17.34 ± 0.78a | 0.83 ± 0.01cd | 70.00 ± 0.96a | 1236.94 ± 39.69a | 4.22 ± 0.30a | 0.48 ± 0.22a | 48.22 ± 2.03ab |
6 | 4422.17 ± 51.57ab | 17.09 ± 0.01a | 0.86 ± 0.01d | 78.69 ± 4.25a | 1260.93 ± 17.9ab | 4.40 ± 0.27ab | 0.36 ± 0.00a | 44.86 ± 0.16ab |
9 | 5025.55 ± 167.72b | 17.16 ± 0.29a | 0.78 ± 0.02b | 69.37 ± 0.35a | 1277.12 ± 53.54ab | 4.67 ± 0.09ab | 0.38 ± 0.01a | 39.23 ± 2.28b |
12 | 6457.43 ± 95.91c | 16.75 ± 0.04a | 0.72 ± 0.00a | 63.86 ± 2.35a | 1543.07 ± 4.67b | 4.62 ± 0.01ab | 0.30 ± 0.03a | 23.60 ± 2.45c |
15 | 7308.72 ± 104.57c | 16.84 ± 0.11a | 0.70 ± 0.02a | 64.56 ± 4.26a | 2333.50 ± 13.09c | 4.85 ± 0.25b | 0.27 ± 0.02a | 19.69 ± 0.66c |
WBDF: wheat bran dietary fibre; Values for a particular column followed by different letters differ superscript significantly (P < 0.05).
Subtle changes were observed in the resilience of both G and G+S systems. The viscosity property of elastomeric materials usually increases with increasing molecular weight. Apparently, the cohesiveness of doughs with the G formula was much larger than that of G+S dough. According to Singh and MacRitchie, (2001), it was believed that there was another force associated with widely spaced points along the chain, referred to as ‘entanglements’. For very large molecules, there may be many ‘entanglements’, thus contributing strength to the polymers. In the case of the G system, the entangled peptide chains might promote more interactions through entanglement coupling, thus yields higher cohesiveness than the G+S system. It was also observed that adding WBDF weakened the cohesiveness of all the dough samples. According to previous study, the high molecular weight (HMW) aggregates generate higher elastic property for both gluten and dough (Singh & Singh, 2013). Upon fibre addition, the weakened aggregation behaviour and decreased HMW aggregates were observed in many studies (Zhou et al., 2014; Nawrocka et al., 2016). Consequently, the reduced cohesiveness of the dough samples may be attributed to the impaired intermolecular interactions. During large-scale deformation, such structure is likely to show feeble resistance, thus producing smaller cohesiveness.
The data presented in Table. 1 reveal that the G system exhibited almost a three-fold higher hardness than that in the G+S system, whereas for springiness, this ratio dropped to nearly one. This finding indicates that the texture of the G+S dough is softer than that of the G dough. Two factors have been described that could produce a high elasticity. The first is a longer polymer chains to generate greater extensibility. The other factor is a lower covalent crosslinking to generate greater flexibility (Tatham et al., 1985). Considering the above two factors, we deduced that the high springiness of the G system is closely related to the long polymer chains, whereas the G+S system is more involved with the high mobility of the chains. After compression, the molecular chains reintegrate and then result in the springiness characteristic. The data shown in Table 1 indicate that the two kinds of dough samples exhibited notably different trends upon the addition of WBDF. The springiness of the G dough initially increased to 78.69 ± 4.25 and then decreased to 64.56 ± 4.26 with the increase in WBDF content, while the springiness of the G+S dough showed a dramatic downtrend from 51.71 ± 3.06 to 19.69 ± 0.66. According to our assumption about the cause of the springiness of the two systems, a small amount of WBDF disrupts the compact gluten network and increases the mobility of the molecular chains, thus leading to the enhancement of the springiness of the G system. However, a high level of WBDF reduces the springiness by inhibiting the aggregation behaviour of gluten proteins. The contribution of gluten proteins to the springiness property is much larger than that of the WBDF, thus, as was observed the springiness of the G dough was not significantly affected below a peculiar threshold weight concentration of 15% WBDF. As for the G+S system, due to the dilution effect of large amounts of starch, the mobility of the gluten molecular chains is strong enough. The reason for the remarkable reduction in the springiness of the G+S dough samples could be that the interactions among the molecular chains were further weakened by the dilution effect of the WBDF. Besides, the depolymerisation of HMW gluten proteins could also be an important factor for the significant decrease in the springiness value. The HMW subunits were thought to be the major elastic component because they have a β-turn-rich repetitive domain (Tatham et al., 1985). The repetitive β-turn structure was reported to form a β-spiral, which can extend to 130% of its original length (Urry et al., 1982). The β-spiral conformation represents an energetically favourable state that would be affected by external forces. Upon the removal of stress, the stable conformation would reform, resulting in an elastic recoil. Hydrogen bonds and hydrophobic interaction are the main forces that assist in maintaining such structure. However, not all the β-spiral structures are elastic. This largely depends on the formation of hydrogen bonding between the turns (Tatham et al., 1985). In other words, anything that disturbs the favourable formation of the two kinds of secondary bonds would impair such elastic structure. According to our previous findings, the addition of WBDF exerted a significant influence on the surface hydrophobicity and hydrogen bonds of gluten (Han et al., 2018). Therefore, the formation of the β-spiral structure may be one of the factors directly responsible for the changes of the dough springiness.
Extensional properties of the dough
The resistance to extension and extensibility of the WBDF-fortified G and G+S systems are shown in Table 2. The extension resistance of G and G+S systems are 1,068.74 ± 56.05 and 61.98 ± 0.33 g, respectively, indicating that the gluten ratio significantly affects the tensile resistance of the dough. Based on the findings about the tensile viscosity of gluten-starch blends with different gluten content, the larger the gluten ratio, the higher the force required to fracture the dough system (Uthayakumaran et al., 2002). However, this tendency reached to the maximum with 60% gluten content, and higher gluten ratio even reduced the dough tensile viscosity, suggesting the absence of a strict positive correlation between gluten content and tensile resistance (Uthayakumaran et al., 2002). Additionally, the extension resistance values of the G and G+S samples were much lower than those found in a previous work in which the gluten dough and flour dough exhibited higher resistance at lower strain rate (Boita et al., 2016). Accordingly, the reduction in tensile resistance should be attributed to the different strain rate (Uthayakumaran et al., 2002).
WBDF content (%) . | G . | G+S . | ||
---|---|---|---|---|
Resistance to extension (g) . | Extensibility (mm) . | Resistance to extension (g) . | Extensibility (mm) . | |
0 | 1068.74 ± 56.05a | 38.73 ± 0.61ab | 61.98 ± 0.33a | 27.22 ± 0.09a |
3 | 1377.27 ± 27.05b | 39.46 ± 0.37a | 72.12 ± 1.19b | 22.64 ± 1.30b |
6 | 1406.85 ± 10.97b | 33.40 ± 0.36abc | 80.99 ± 0.71c | 20.84 ± 1.34bc |
9 | 1410.83 ± 87.44b | 32.63 ± 1.21bc | 88.67 ± 0.27d | 21.27 ± 0.01b |
12 | 1470.35 ± 31.28bc | 29.39 ± 0.79c | 88.56 ± 0.43d | 18.73 ± 0.72c |
15 | 1548.58 ± 9.72c | 27.23 ± 1.05c | 89.44 ± 1.14d | 18.68 ± 1.18c |
WBDF content (%) . | G . | G+S . | ||
---|---|---|---|---|
Resistance to extension (g) . | Extensibility (mm) . | Resistance to extension (g) . | Extensibility (mm) . | |
0 | 1068.74 ± 56.05a | 38.73 ± 0.61ab | 61.98 ± 0.33a | 27.22 ± 0.09a |
3 | 1377.27 ± 27.05b | 39.46 ± 0.37a | 72.12 ± 1.19b | 22.64 ± 1.30b |
6 | 1406.85 ± 10.97b | 33.40 ± 0.36abc | 80.99 ± 0.71c | 20.84 ± 1.34bc |
9 | 1410.83 ± 87.44b | 32.63 ± 1.21bc | 88.67 ± 0.27d | 21.27 ± 0.01b |
12 | 1470.35 ± 31.28bc | 29.39 ± 0.79c | 88.56 ± 0.43d | 18.73 ± 0.72c |
15 | 1548.58 ± 9.72c | 27.23 ± 1.05c | 89.44 ± 1.14d | 18.68 ± 1.18c |
WBDF: wheat bran dietary fibre; Values for a particular column followed by different letters differ superscript significantly (P < 0.05).
WBDF content (%) . | G . | G+S . | ||
---|---|---|---|---|
Resistance to extension (g) . | Extensibility (mm) . | Resistance to extension (g) . | Extensibility (mm) . | |
0 | 1068.74 ± 56.05a | 38.73 ± 0.61ab | 61.98 ± 0.33a | 27.22 ± 0.09a |
3 | 1377.27 ± 27.05b | 39.46 ± 0.37a | 72.12 ± 1.19b | 22.64 ± 1.30b |
6 | 1406.85 ± 10.97b | 33.40 ± 0.36abc | 80.99 ± 0.71c | 20.84 ± 1.34bc |
9 | 1410.83 ± 87.44b | 32.63 ± 1.21bc | 88.67 ± 0.27d | 21.27 ± 0.01b |
12 | 1470.35 ± 31.28bc | 29.39 ± 0.79c | 88.56 ± 0.43d | 18.73 ± 0.72c |
15 | 1548.58 ± 9.72c | 27.23 ± 1.05c | 89.44 ± 1.14d | 18.68 ± 1.18c |
WBDF content (%) . | G . | G+S . | ||
---|---|---|---|---|
Resistance to extension (g) . | Extensibility (mm) . | Resistance to extension (g) . | Extensibility (mm) . | |
0 | 1068.74 ± 56.05a | 38.73 ± 0.61ab | 61.98 ± 0.33a | 27.22 ± 0.09a |
3 | 1377.27 ± 27.05b | 39.46 ± 0.37a | 72.12 ± 1.19b | 22.64 ± 1.30b |
6 | 1406.85 ± 10.97b | 33.40 ± 0.36abc | 80.99 ± 0.71c | 20.84 ± 1.34bc |
9 | 1410.83 ± 87.44b | 32.63 ± 1.21bc | 88.67 ± 0.27d | 21.27 ± 0.01b |
12 | 1470.35 ± 31.28bc | 29.39 ± 0.79c | 88.56 ± 0.43d | 18.73 ± 0.72c |
15 | 1548.58 ± 9.72c | 27.23 ± 1.05c | 89.44 ± 1.14d | 18.68 ± 1.18c |
WBDF: wheat bran dietary fibre; Values for a particular column followed by different letters differ superscript significantly (P < 0.05).
The addition of WBDF to G and G+S samples caused distinct effects on the dough resistance to extension and extensibility values. Usually, the trends of the two indicators tend to be consistent, in other words, the more extensile the sample, the better the extension resistance. Indeed, Boita et al. (2016) found that the increase in the content of wheat bran in dough resulted in similar downward trend of the extension and extension resistance. It was believed that the dilution effect induced by the wheat bran affected the dough extension property. However, different trends were observed in our study. The dough extension resistance increased with the addition of WBDF, whereas the extensibility exhibited the opposite trend.
When the dough sample is undergoing an axial extension, the elongational viscosity remains relatively constant within a low strain range. Then, the elongational viscosity starts to increase rapidly, as long as the strain exceeds a threshold. Such a rapid increase in viscosity at higher strain is recognised as a strain-hardening response (also called mechanical hardening) (Sliwinski et al., 2004a,b). The increase of the gluten content in dough was reported to produce a more remarkable strain-hardening response and stretching stiffness (Sliwinski et al., 2004a,b). However, starch addition could lead to a reduction in both strain-hardening ability and elongational viscosity.
The resistance-extension curves of dough prepared from the G and G+S systems enhanced with WBDF are shown in Fig. 1. These curves reveal that adding WBDF to doughs remarkably improved the strain-hardening capacity of both the G and G+S systems, as indicated by the steeper curves. The strain rate of the bread during fermentation has been estimated as ≈10−4 s−1 in an early study (Bloksma & Bushuk, 1988). Thus, it can be hypothesised that the greater degree of strain-hardening at lower strain rates may be responsible for the limited expansion of fibre-fortified bread during baking. Therefore, the prolonged proofing time and increased level of added yeast should be adopted to obtain a bread with suitable volume.
![Resistance force vs. extension curves of dough prepared from G and G+S system enhanced with WBDF. [Colour figure can be viewed at wileyonlinelibrary.com]](https://oup.silverchair-cdn.com/oup/backfile/Content_public/Journal/ijfst/53/12/10.1111_ijfs.13861/1/m_ijfs13861-fig-0001-m.jpeg?Expires=1750253774&Signature=qzIz4ipxrplfXZhWZQ6X74zIhFvhDkpl8raJlJv2vDrFyEsSDsoYze6PSJjeeuGvYMceiy0pTGITMei3~q6KfDwX4cblDN2SdzU~TXF~PZzFmSSyxu2Ja2q3-RE5Ebne6T1qTYeJdxZFsv7Ardddg8bDX7uBWjy33T-J58UtUroY9qSSfkJ8KZdH~uNKhiFwxhlCqbV55iYsOE8UsvvsD3HTgdOHtQDE64XNlynb-00XvnKFJHgj7Mf8OGe4UKsvOxL0smnqXUX2r9-CxPAPwrpe79I6Hu0ikB25xlG5FSQShRC1zuYLxqOdfg~TRoNptKtCPzyftzT6CP2wRz5ybw__&Key-Pair-Id=APKAIE5G5CRDK6RD3PGA)
Resistance force vs. extension curves of dough prepared from G and G+S system enhanced with WBDF. [Colour figure can be viewed at wileyonlinelibrary.com]
The strengthened strain-hardening capacity induced by WBDF may be the direct cause of the notably increased extension resistance of doughs. For all the dough samples, the weakened extensibility suggested a weaker gluten matrix. As the presence of WBDF increased the frictional force between the gluten protein chains, the gluten fibril appeared to be thinner during extensional deformation and resulted in easier fracture of the gluten network. Besides, the rigid WBDF was more likely to pierce the uniform gluten network and resulted in the impairment of the extensibility.
According to the observed secondary structural changes of the bran-enriched gluten samples, more β-sheet conformations were adopted (Bock & Damodaran, 2013). Such conformation may assist peptide chains in developing a layer structure rather than a round structure. It was reported that the dough extensibility was mainly determined by the entangled gluten network (Singh & MacRitchie, 2001). Thus, the entanglement and aggregation behaviour of peptide chains could significantly affect the dough extensibility. The β-sheet structure is formed by intermolecular hydrogen bonding among molecular chains. Such structure is stiffer enough to impart the dough with enhanced resistance to extension. According to McCann et al. (2016), the extension resistance of gluten increased as higher strain rate was applied. Evidence from the macromolecular properties of gluten suggested that the slips among molecular chains become dominant at a low extension rate, whereas the peptide chains tend to intertwine to a form a branch structure and increase the extension resistance at higher extension rate (Singh & MacRitchie, 2001). The β-sheet structure could be regarded as the mentioned ‘branch structure’ to some extent, which was induced by the interaction between WBDF and gluten rather the extension behaviour. Such molecular beam composed by aggregated protein branches could greatly improve the extension resistance behaviour of dough during extension deformation. The above findings could explain the extension behaviour of both the G and G+S doughs. However, the presence of starch in the G+S system produced a more complex deformation mechanism. According to the confocal laser scanning microscopy (CLSM) images, the friction of starch granules with protein branches resulted in a weakened and fractured gluten network upon extensional deformation (McCann et al., 2016). Another study pointed out that the stiffness of the gluten network relied heavily on the large starch granules (Tanner et al., 2011). Accordingly, the WBDF particles with coarser surface and higher mechanical strength, was more likely to accelerate the rupture of the gluten network by increasing the friction with the gluten branches.
Conclusion
This study investigated the effect of WBDF on the textural and extensional properties of gluten (G) as well as gluten-starch (G+S) doughs. Upon WBDF addition, the hardness of both G and G+S doughs increased by 86.70% and 95.49%, respectively, probably due to the increasing abundance of gluten proteins with β-sheet conformation. However, the cohesiveness values decreased by 13.58% for the G system and 0.46% for the G+S system due to the impaired intermolecular interactions and weakened aggregation behaviour of WBDF-enriched doughs. The addition of WBDF exert significantly different influences on the springiness of the G and G+S systems, which were considered to be associated with the formation of β-spiral conformation and the discrepancy of their properties. Considering the extension property, the two kinds of doughs exhibited similar trend as the WBDF content increased. The markedly increased extension resistance from 1068.74 ± 56.05 to 1548.58 ± 9.72 for G doughs and 61.98 ± 0.33 to 89.44 ± 1.14 for the G+S system may be ascribed to the strengthened strain-hardening capacity induced by WBDF and the formation of more β-sheet structures. It was assumed that the greater degree of strain-hardening at lower strain rates may be responsible for the limited expansion of fibre-fortified bread during baking. Additionally, WBDF also accelerated the rupture of the gluten network as evidenced by the notable reduction of the dough extensibility by increasing the friction with gluten chains and impairing the uniformity of the network structure. Therefore, for bran-enriched flour products, the pretreatment with bran-like particles leading to size reduction, softening or solubility improvement may be advantageous. This study advances our understanding of the textural changes of WBDF-enriched doughs, however, the study of the mechanism of the quality changes induced by WBDF should be further explored.
Acknowledgments
This work was supported by the State key research and development plan ‘modern food processing and food storage and transportation technology and equipment’ (No. 2017YFD0400200), Henan province colleges and universities young backbone teacher plan (No.2016GGJS-070), Key scientific and technological project of Henan Province (No. 172102110008), National Natural Science Foundation of China (No. 31571873) and Talent Projects from Henan University of Technology (NO. 2018RCJH08).