-
PDF
- Split View
-
Views
-
Cite
Cite
Dorota Witrowa-Rajchert, Piotr P Lewicki, Rehydration properties of dried plant tissues, International Journal of Food Science and Technology, Volume 41, Issue 9, November 2006, Pages 1040–1046, https://doi.org/10.1111/j.1365-2621.2006.01164.x
- Share Icon Share
Abstract
Apples, carrots and potatoes cut in cubes were dried by convection and then subjected to rehydration in water at room temperature. Mass and volume changes were followed during rehydration. A minimal displacement gauge was used to record changes of height of individual cubes during wetting. Porosity of the dried material was calculated and its changes during rehydration were observed. Comparing volume increase with mass gain during rehydration it was found that the mechanism of water imbibing is strongly influenced by porosity and chemical composition of the dried material. Dried apple was very porous, hence its mass gain was much faster than the volume increase. On the other hand, gelatinised starch in dried potato strongly absorbed water and the volume increase outpaced the mass gain. Recording changes of height of the dried cubes upon rehydration evidenced phase changes and injury incurred to the tissue structure by dehydration. In apple a collapse of structure was observed, while potato cubes swelled from the very beginning of the wetting process. In carrot instantaneous changes of height of the cube were observed, which could be due to relaxation of shrinkage stresses and displacements of injured tissue structures.
Introduction
Drying causes many changes in structure and properties of plant material. The changes consist of physical alterations, chemical reactions and biochemical effects. Physical alterations include shrinkage, increased or decreased porosity, and decreased ability to imbibe water and damage to microscopic structure.
Drying air either replaces water evaporated from the material undergoing drying or the volume of the sample decreases. Usually both processes occur but the ratio between them depends on the kind of material and the extent of drying. Many authors report (Mazza & LeMaguer, 1980; Crapiste et al., 1985; Suarez & Viollaz, 1991; Karathanos et al., 1993) that the change in volume of drying material is equivalent to the volume of evaporated water. However, in some publications (Lozano et al., 1983; Lewicki & Witrowa, 1992; Lewicki et al., 1994; Madamba et al., 1994; McMinn & Magee, 1997) it is shown that the shrinkage does not follow amount of evaporated water during the whole drying process. Drying of surface layers increases their mechanical resistance and the material shrinkage is hindered. The shrinkage stresses that are created can tear apart cell walls and damage to internal structure is observed. This would be expressed by increased porosity of dried material, and that was observed by many authors (Lozano et al., 1980, 1983; Madamba et al., 1994; Zogzas et al., 1994; Wang & Brennan, 1995; Karathanos et al., 1996).
Concentration of cell sap increases due to evaporation of water. This leads to changes in pH, redox potential and ionic strength, which affect tertiary and quaternary structure of biopolymers. Degradation of pectins (Levi et al., 1988), crosslinking of proteins and changes in structure of lipoproteins are observed (Cole, 1962; Chapman, 1994). Sterling & Shimazu (1961) reported increased crystallinity of cellulose. Concentration of cell sap can promote crystallisation of low molecular weight constituents but, on the other hand, increased viscosity may prevent crystallisation, hence an amorphous state can be formed in dried material.
Chemical reactions may be initiated during drying and they can affect the stability of the dry material. Nonenzymatic browning changes not only the colour of the material, but also affects proteins, which are constituents of plasmalemma and cytoplasm. As a result water-binding properties can be affected. Oxidation reactions are accompanied by free radical formation, which interacting with biopolymers promote crosslinking and affect properties of the material.
Plant tissue subjected to drying can exert some biochemical activity until the material is sufficiently wet (Lewicki et al., 2001). Moreover, native enzymes can be preserved during drying and their activity can be observed upon rehydration. Wijaya et al. (1991) showed that the enzymatic activity of dried garlic is dependent on the method of dehydration. Enzymatic activity in dried onion is much higher in freeze-dried material than that in similar product dried by convection (Freeman & Whenham, 1975). Haas et al. (1974) showed that some enzymatic activity is preserved in dried vegetables.
Multiple changes imparted to the material by drying affect its ability to imbibe water and rehydrate. During rehydration three processes can be distinguished, namely the imbibing of water by the dried material, the swelling of biopolymers and leaching of solubles into surrounding water (Witrowa-Rajchert, 1999). The processes proceed simultaneously, but the extent and kinetics of changes observed during rehydration can provide evidence concerning the damage done to the tissue by the drying. Such interpretation of the rehydration is possible, because the process is affected by factors dependent either on lattice forces inside the plant tissue or on the interactions between the matrix and the surrounding medium.
The aim of this work was to assess changes incurred to plant tissue by convective drying expressed by the rehydration properties of dried material. Rehydration properties of dried apple, carrot and potato were assayed and related to macroscopic indices of changes in internal structure of materials subjected to convective drying.
Materials and methods
Plant material
The experiments were carried out with apples (var. Idared), carrots (var. Kazan) and potatoes (var. Irga). Fruits and vegetables were stored for 3 months after harvest at 4–6 °C and at 90% air humidity. Apples, carrots and potatoes with uniform size and weight were selected for this experiment and used within 1 month.
Pretreatment
All investigated materials were cut into 1-cm cubes. Some 200 cubes were cut for each drying experiment in order to have a representative sample for each investigated material. Apple cubes were soaked in 0.5% ascorbic acid solution to limit enzymatic browning. Carrot and potato cubes were blanched in water at 96–98 °C (carrots for 3 min and potatoes for 2 min). The ratio of material to water was 1:10 (w/w). The blanched material was cooled with water to room temperature. Applied parameters of blanching were those recommended by technology (Fellows, 1988) and applied in industry.
Drying
Cubes blotted with filter paper were spread on the dryer screen in a single layer with a tray load of 6 kg m−2. Convection drying was carried out at 70 °C (apples and carrots) and 60 °C (potatoes) and air velocity 2 m s−1. Drying parameters were those recommended by technology.
Rehydration
Dried materials were rehydrated in distilled water at room temperature for 0.5, 1, 2, 3, 4 and 5 h. The ratio of material to water was 1:50 (w/w), and liquid was stationary. Rehydrated material was strained, blotted with filter paper and weighted.
Analytical procedures
Dry matter content in raw, dried and rehydrated materials was measured according to Polish Standard PN-90/A-75101/03. Volume of raw and dried cubes and material undergoing rehydration was measured by volumetric method (Mazza, 1983).
Change in height of a single dried cube during rehydration was followed in the following way. A cube of dried material was placed in a vessel and immobilised with two pins. A plate of 1 cm2 surface and 0.09 g mass was put on the upper cube surface. Vertically from the top, a displacement gauge was placed on the plate and zeroed. Mass of the moving part of the gauge was 3.2 g. Hence a total pressure exerted on the cube was 32.9 Pa. Water at room temperature was added to the vessel and the change in cube height during rehydration was continuously registered in micrometres by computer.
The density was defined as the ratio of mass to volume:

Porosity of rehydrating material was expressed by the following equation:
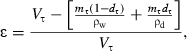
where V is volume (m3), m is mass (kg), d is dry matter (fraction), ρ is density (kg m−3), ɛ is porosity (fraction), and subscripts w is for wet, τ at time τ, and d is for dry.
Kinetics of the mass increase of dried materials during rehydration was described by the following equation:
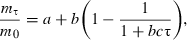
where a, b, c are constants, τ is time (h), and subscript 0 is for initial.
Statistical analysis
Drying experiments were done in triplicates and dry cubes from each drying were combined together and stored in hermetic container for several days in order to equilibrate water content. Rehydration of dry material was done in triplicates and for each time of rehydration twelve cubes chosen randomly were used. Hence the rehydration experiment for each material was done on 216 cubes out of 600.
Measurement of volume of cubes of raw, dry and undergoing rehydration material was repeated three times. In each measurement five cubes were used. In total volume of 120 cubes was measured. Designing experiment this way it was assumed that an average information on the influence of drying on rehydration properties of apple, carrot and potato will be obtained, and the heterogeneity effects, arising from the nature of plant material, will be minimised.
The results from rehydration experiment were subjected to mathematical interpretation using software Table Curve 2D (SPSS Inc., Chicago, IL, USA). Determination coefficients for the relationship between relative mass increase and time were in the range 0.9895–0.9972, and for volume increase the coefficient varied from 0.9934 to 0.9963.
As changes in height were measured on a single cube the measurement was repeated on twenty samples. In this measurement heterogeneity of cubes was very evident and calculation of averages was not justified. Hence, the most typical results are presented in this study. More detailed description of the changes in dry cube height during rehydration is presented in Lewicki & Wiczkowska (2006).
Results and discussion
Relative mass increases of dried materials subjected to rehydration in water are presented in Fig. 1. The largest rehydration ability was shown by carrot, in which the mass increased over sixfold during 5 h soaking in water. Dried apple imbibed water not as good as carrot and its mass increased about fourfold. Dried potato cubes showed the least ability to imbibe water and its mass increased during 5 h rehydration about threefold.
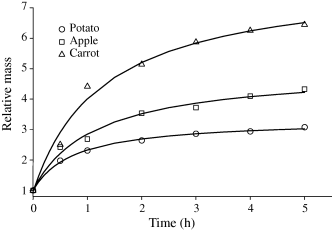
Relative increase in mass of dried material during rehydration in water.
Analysis of eqn 3 shows that at τ → ∞ the equilibrium relative mass increase is equal to (a + b). Calculated values are shown in Table 1 which shows that after 5 h of rehydration, the potato cubes were close to reach equilibrium while other investigated materials were far from the equilibrium state. Calculated equilibrium mass depended on the kind of rehydrated material, but in any case it did not match the mass of the raw material. Equilibrium mass of rehydrated apple was equal to 68% of its mass before drying. For carrot and potato it was 57% and 54%, respectively. On the basis of these data it could be inferred that pre-treatment and drying strongly affected the ability of the dried material to imbibe and bind water. The influence was dependent on the kind of material subjected to drying and was more pronounced for carrot and potato than for apple.
Material . | Equilibrium relative mass . | Standard error of estimation . | Equilibrium relative volume . | Standard error of estimation . |
---|---|---|---|---|
Apple | 4.96 | 0.14 | 0.69 | 0.01 |
Carrot | 7.95 | 0.26 | 0.73 | 0.02 |
Potato | 3.33 | 0.05 | 0.66 | 0.02 |
Material . | Equilibrium relative mass . | Standard error of estimation . | Equilibrium relative volume . | Standard error of estimation . |
---|---|---|---|---|
Apple | 4.96 | 0.14 | 0.69 | 0.01 |
Carrot | 7.95 | 0.26 | 0.73 | 0.02 |
Potato | 3.33 | 0.05 | 0.66 | 0.02 |
Material . | Equilibrium relative mass . | Standard error of estimation . | Equilibrium relative volume . | Standard error of estimation . |
---|---|---|---|---|
Apple | 4.96 | 0.14 | 0.69 | 0.01 |
Carrot | 7.95 | 0.26 | 0.73 | 0.02 |
Potato | 3.33 | 0.05 | 0.66 | 0.02 |
Material . | Equilibrium relative mass . | Standard error of estimation . | Equilibrium relative volume . | Standard error of estimation . |
---|---|---|---|---|
Apple | 4.96 | 0.14 | 0.69 | 0.01 |
Carrot | 7.95 | 0.26 | 0.73 | 0.02 |
Potato | 3.33 | 0.05 | 0.66 | 0.02 |
Both gain in material mass and an increase in material volume occurred during rehydration (Fig. 2). Theoretically the increase in volume of the cube should correspond to the amount of water absorbed. However, the increase in volume should also depend on porosity and chemical composition of the material. If the material was characterised by a considerable porosity water should fill the pores and a gain in its volume should be small or none. In the case of low porosity, and the presence of biopolymers water penetrating into the matrix of a solid body caused swelling and increase of the volume of the material.

Relative volume increase of dried material during rehydration in water.
In order to investigate the correlation between water penetration and volume increase the rates of mass and volume gains were calculated. It was found that the rate of the relative volume gain was proportional to the relative mass gain and a straight line (Fig. 3) presented the relationship. Moreover, it showed that during 5 h rehydration there was no change in the mechanism of water absorption and both processes, i.e. volume increase and mass gain were strictly interrelated.
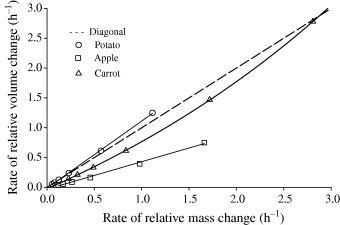
Relationship between rate of relative mass increase and rate of relative volume increase during rehydration.
The relative mass gains of apple and carrot were found more rapid than the relative volume gains (Fig. 3). In those dried materials, water penetrating first into pores did not evoke an equivalent volume gain. The smallest slope of a straight line was obtained for apple, which was characterised by the largest porosity (Table 2). In the case of potato, rehydration proceeded in a different manner. The relative volume of potato increased more rapidly than its relative mass and the slope of a straight line became larger than one. Water penetrated first the material through the solid matrix, caused swelling of biopolymers and a more rapid increase in the volume than that in the mass of the material.
Material . | Dry matter content (%) . | Shrinkage (%) . | Porosity (%) . | ||
---|---|---|---|---|---|
Raw material . | Dried material . | Raw material . | Dried material . | ||
Carrot | 8.98 ± 0.58 | 93.28 ± 1.11 | 89.5 | 5.1 ± 0.9 | 17.1 ± 0.2 |
Apple | 12.10 ± 0.49 | 93.22 ± 0.30 | 73.3 | 23.5 ± 1.4 | 71.2 ± 0.2 |
Potato | 17.74 ± 0.28 | 92.87 ± 0.30 | 81.5 | 1.7 ± 0.6 | 10.7 ± 2.6 |
Material . | Dry matter content (%) . | Shrinkage (%) . | Porosity (%) . | ||
---|---|---|---|---|---|
Raw material . | Dried material . | Raw material . | Dried material . | ||
Carrot | 8.98 ± 0.58 | 93.28 ± 1.11 | 89.5 | 5.1 ± 0.9 | 17.1 ± 0.2 |
Apple | 12.10 ± 0.49 | 93.22 ± 0.30 | 73.3 | 23.5 ± 1.4 | 71.2 ± 0.2 |
Potato | 17.74 ± 0.28 | 92.87 ± 0.30 | 81.5 | 1.7 ± 0.6 | 10.7 ± 2.6 |
Material . | Dry matter content (%) . | Shrinkage (%) . | Porosity (%) . | ||
---|---|---|---|---|---|
Raw material . | Dried material . | Raw material . | Dried material . | ||
Carrot | 8.98 ± 0.58 | 93.28 ± 1.11 | 89.5 | 5.1 ± 0.9 | 17.1 ± 0.2 |
Apple | 12.10 ± 0.49 | 93.22 ± 0.30 | 73.3 | 23.5 ± 1.4 | 71.2 ± 0.2 |
Potato | 17.74 ± 0.28 | 92.87 ± 0.30 | 81.5 | 1.7 ± 0.6 | 10.7 ± 2.6 |
Material . | Dry matter content (%) . | Shrinkage (%) . | Porosity (%) . | ||
---|---|---|---|---|---|
Raw material . | Dried material . | Raw material . | Dried material . | ||
Carrot | 8.98 ± 0.58 | 93.28 ± 1.11 | 89.5 | 5.1 ± 0.9 | 17.1 ± 0.2 |
Apple | 12.10 ± 0.49 | 93.22 ± 0.30 | 73.3 | 23.5 ± 1.4 | 71.2 ± 0.2 |
Potato | 17.74 ± 0.28 | 92.87 ± 0.30 | 81.5 | 1.7 ± 0.6 | 10.7 ± 2.6 |
The aforementioned results indicated that the process of water absorption during rehydration consisted in filling the air spaces formed upon drying or in penetrating into the matrix of a solid body, depending on the type of the material used, namely its chemical composition.
The porosity of rehydrated apple, carrot and potato was calculated on the basis of eqn 2. The correlation between porosity and water content is presented in Fig. 4. During hydration process, a decrease in porosity was observed, which ranged from 0.71 to 0.28 for apple, from 0.17 to 0.05 for carrot, and from 0.11 to 0.05 for potato. In addition, a substantial decline in porosity was observed for apple in the first stages of rehydration. It confirmed previous observations that water penetrated first the pores of the dried apple causing a higher gain of mass than the volume and simultaneous considerable decrease in porosity. At the beginning of hydration, the porosity of carrot and potato was maintained on the level characteristic for the dried material. Further rehydration caused penetration of water into solid matrix of the material causing its swelling. In the subsequent stages of the process, water filled the pores and decreased porosity of the material.
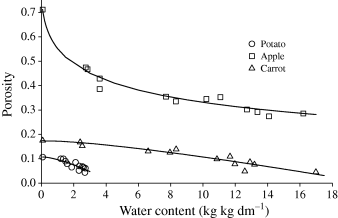
The porosity of carrot after 5 h of rehydration corresponded to that of the material before drying, whereas the porosity of apple and potato was still larger than the initial values. The increased porosity could be due to either ‘closing’ of air bubbles present in the micropores, the phenomenon described in the literature (Luyet, 1962), or injury to the tissue structure and its loosening (Lewicki & Pawlak, 2003).
Although raw food materials are characterised by various structures and composition, their main components are always carbohydrates, lipids, proteins and water. The composition of carbohydrate fraction in food varies from low-molecular water-soluble sugars to cell wall polymers, which may be either plasticised or softened by water, or even water soluble. On the other hand, the protein fraction is entirely of polymer structure. Those compounds are acknowledged as biomaterials compatible with water and capable of existing in molecularly unordered amorphous state that may be formed in dried materials at the final stages of dewatering (Roos et al., 1996). During drying, water may be simultaneously removed from the structures of some polymers, which in turn increases the degree of their crystallinity (Sterling & Shimazu, 1961; Chapman, 1994). It is known that amorphous regions of both low-molecular compounds and polymers enhance rehydration, but crystalline regions inhibit its course (Lewicki, 1998).
If drying-induced changes, consisting in phase transitions of the components, occurred in the investigated raw materials containing low-molecular substances and polymers, then they would affect the hydration process and reverting of the cubes to the size prior to drying.
The change of height of a single dry cube during rehydration was followed with a minimal displacement gauge and expressed as the ratio of the cube height at a specified time to the height of dry material before hydration (L/L). Figure 5 presents changes of the relative height of cubes of investigated materials. An initial reduction in the size of dried apple upon rehydration was observed in all experiments. After having reached its minimum, the height of the cube returned to its size after drying and further increase of height was observed. The minimal value of the L/L ratio ranged from 0.93 to 0.96, and the time taken accounted from a few to ten or more minutes.
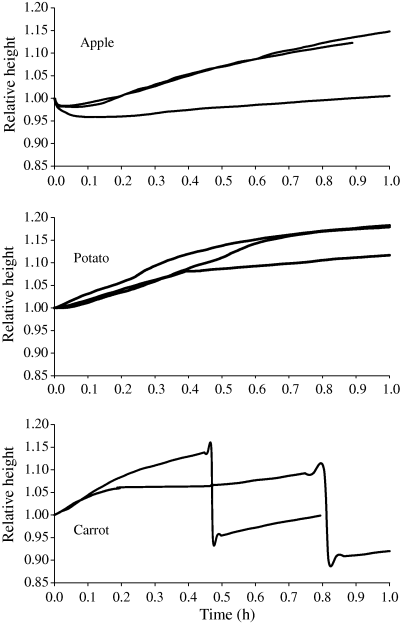
Relationship between relative cube height and time of rehydration.
Upon drying at high temperatures, the low-molecular compounds present in apple cells were concentrated and probably formed an amorphous state. The amorphous regions, formed in the material during drying, were readily available to water, the matrix was plasticised, and its viscosity was lowered causing the collapse of the material. In consequence a decrease in material volume was likely to be observed. This was the probable mechanism of structure collapse in dried apple at the beginning of the rehydration process, especially at that stage when water penetrated the pores of the material. During further rehydration polymers (mainly cell walls) absorbed water and the material swelled.
A different course of changes in the relative height was observed for dried potato cubes (Fig. 5). A lack of structure collapse and a continuous increase of the cube size (by c. 12–18%) were observed within the first hour of rehydration.
A lack of the structure collapse during rehydration of dried potato could result from a low content of low-molecular weight substances and presence of large amounts of gelatinised starch, which was caused by blanching. During rehydration water was absorbed by gelatinised starch and swelling of the material was observed from the first moment of the process. The crystalline state of cellulose (Sterling & Shimazu, 1961) as well as drying-induced recrystallisation of gelatinised starch, decreased solubility (Waniska & Gomez, 1992) and reinforced structure of the material. During rehydration, the dried starch characterised by numerous amorphous domains (Lewicki, 1999) became well hydrated and maintained its structure, thus preventing a collapse of the material.
Figure 5 presents the course of the changes of cube height of dried carrot during the rehydration time. At the initial stage of rehydration, the size of the cubes increased rapidly and thereafter an instantaneous collapse of the structure was observed. The collapse occurred at different times and seemed to be a specific property of individual cubes. The collapse was very evident and accounted for more than 20% of the height of the rehydrating cube.
In the dried tissue of carrot, one should expect the amorphous state of low-molecular substances, which could enhance the structure collapse, as early as the rehydration begins. At the same time, the content of cellulose, constituting c. 24% of dry matter (Mattea et al., 1989), could cause reduced water absorption due to substantial crystallinity of that polymer and add strength to the material, as well. As consequence, the rate of volume increase was somewhat slower than the rate of mass gain (Fig. 3). In addition, a characteristic tissue structure of carrot was the reason for a high anisotropic shrinkage during drying and the occurrence of considerable stresses in the material. Addition of water and hydration of polymers could lead to relaxation of those stresses. A rapid collapse in carrot structure after some time of hydration could result from a release of shrinkage stresses remaining in the material after drying. It could also reflect mechanical damage to the structure caused by drying and pronounced upon rehydration.
Conclusions
Rehydration of dried material results in the increase of both the material mass and volume. A strong correlation was found between the mechanisms of water penetration and the ratio of volume increase to mass gain. For the ratio lower than 1 volume increased slower than the mass and it indicated penetration of rehydrating water into porous structure. At the ratios larger than 1 the swelling of rehydrating material was faster than the gain in mass, and absorption of water by polymers was evidenced. A more rapid gain of mass than volume, which was characteristic for dried apple, lead to a rapid dissolution of low-molecular weight compounds, which could be in amorphous state, and to a structure collapse at the initial stages of the rehydration process. In the case when volume rose more rapidly than the mass, characteristic for dried potato, no structure collapse was observed. It is probably affected by crystalline state of high-molecular mass compounds on the one hand and by the presence of amorphous domains in starch on the other.
A collapse of structure observed in the case of dried carrot could not necessarily be due to the phase changes in food constituents. It seemed that shrinkage stresses created during dehydration could undergo relaxation upon wetting and cause displacements in injured tissue. This phenomenon could especially be evident in heterogeneous tissues with anisotropic structures containing parenchyma cells and vascular bundles.