-
PDF
- Split View
-
Views
-
Cite
Cite
M Karthikeyan, A O Dileep, B A Shamasundar, Effect of water washing on the functional and rheological properties of proteins from threadfin bream (Nemipterus japonicus) meat, International Journal of Food Science and Technology, Volume 41, Issue 9, November 2006, Pages 1002–1010, https://doi.org/10.1111/j.1365-2621.2006.01122.x
- Share Icon Share
Abstract
The effect of the number of water washing cycles on the proximate composition, physicochemical and functional properties of proteins from threadfin bream meat was studied. Contents of proteins, fat, ash and non-protein nitrogen showed a significant (P < 0.05) decrease with the number of washing cycles. The removal of low molecular weight components and increase in the concentration of high molecular weight components was confirmed by gel filtration profile and sodium dodecyl sulphate polyacrylamide gel electrophoresis. The water absorption, fat absorption and emulsion capacity parameters showed a positive correlation with the number of washing cycles. The gel-forming ability showed a marked improvement with the number of washing cycles. The dynamic viscoelastic behaviour of unwashed and washed meat showed a structure build-up reaction and was more pronounced in once washed meat. The flow behaviour of total protein solution from unwashed and washed meat showed a pseudoplastic behaviour.
Introduction
Surimi is a wet myofibrillar protein concentrate obtained from mechanically deboned fish flesh that is water washed, blended with cryoprotectants, frozen and stored frozen (Park et al., 2004). Washing the minced meat is a critical step in the production of surimi, which not only removes fat, pigments, amines and sarcoplasmic proteins but also concentrates myofibrillar proteins (Toyoda et al., 1992). The rate at which these undesirable soluble components are removed from the minced meat is a function of several factors, including water temperature, the degree of agitation and the contact time between water and meat particles (Green, 1989). The number of washing cycles and water/meat ratios used for washing vary among surimi processors. A water/meat ratio of 3:1 to 8:1 is often used by on-shore processors (Park & Morrissey, 2000). This washing process is repeated three to four times to ensure sufficient removal of sarcoplasmic protein fractions. The majority of the soluble components are freely and rapidly removed in the first washing cycle primarily by dilution of the free soluble components (Toyoda et al., 1992). Long periods of washing would result in higher hydration of mince and degradation of myofibrillar proteins, making the subsequent dehydration process more difficult and could reduce gel-forming ability (Pacheo-Aguilar et al., 1989; Lin & Park, 1996).
The surimi industry in Japan, America and Europe mainly depends on Alaska Pollock as raw material for surimi production. Nearly 50–70% of total surimi produced comes from Alaska Pollock (Park & Morrissey, 2000). However, this proportion is continuously reducing with the decline in stocks of Alaska Pollock and has paved the way for utilisation of other fisheries resources for surimi production. The threadfin bream (Nemipterus spp) belong to the family Nemipteridae and nearly ten species are commonly found in the Indo-Pacific region of tropical and subtropical waters. The main species caught along the Indian coast includes Nemipterus japonicus, which has a light meat colour, a low fat content and is available in abundance. With the increase in demand for surimi and surimi-based products, many of the Southeast Asian countries, including India, have stepped up production using locally available resources. The process line for Alaska Pollock surimi production is adopted in most of the countries including India and it involves the use of a large amount of fresh water ranging from ten to twenty times the weight of deboned meat (Lee, 1984). It is important that the effect of the number of washings and other processing conditions on the physicochemical and functional properties of proteins from different fish suitable for surimi production should be standardised. The need for standardisation of the number of washing cycles, especially in batch production of surimi from different fish species, is high, in order to minimise the consumption of water and to avoid environmental pollution. With this in view, the present investigation was carried out to determine the effect of the number of washing cycles on the physicochemical, rheological and functional properties of proteins from threadfin bream meat.
Materials and methods
Material
Fresh threadfin bream (N. japonicus) caught by trawl net, off the coast of Mangalore (Southwest coast of India), was used for the present study. The fish were iced with an equal weight of ice on board the fishing vessel, landed at Mangalore landing centre and quickly transported to the laboratory in iced conditions (3.0 ± 1.0 °C). The length and weight of the fish ranged from 15 to 20 cm and from 100 to150 g, respectively. The fish were washed thoroughly using chilled water (3 °C) and dressed to remove scales, gills and viscera. The dressed fish were filleted, the meat picked manually and then macerated using a pestle and mortar under chilled conditions. The macerated meat was used for water washing in chilled potable water (3 °C). The meat and chilled water were mixed manually in a stainless steel vessel and stirred gently using a glass rod. One portion of the meat was washed once and the other portion of the meat was washed thrice, the unwashed meat being used as the control. During each wash, the meat was mixed thoroughly with chilled water in the ratio of 1:3 (meat:water) for 2 min in a 10-L stainless steel vessel and the slurry was allowed to settle for 5 min. The supernatant water was decanted and the washing cycle repeated as required. The removal of excess water was accomplished by squeezing the meat manually using a muslin cloth and this process was standardised by trial and error method so as to have consistent moisture content in the washed meat. The partially dehydrated meat was used for further analysis. The experiments were conducted in three different batches and from each batch representative samples were taken for analysis.
Analyses
Composition
Total protein (N × 6.25), moisture, ash and fat content were determined according to the method as described in AOAC (1995). The non-protein nitrogen (NPN) content in the trichloroacetic acid extract (20%) of minced meat was determined by the method described by Velankar & Govindan (1958).
pH
The pH of the meat samples was measured using a Systronic 324 pH meter (Ahmedabad, India). Five grams of meat were ground with 45 mL distilled water and the pH was measured.
Viscosity
The apparent reduced viscosity of total proteins from threadfin bream meat was measured using Oswald's viscometer at 25 ± 1 °C using extraction buffer (EB: phosphate buffer 0.05 m, pH 7.5, containing 1.0 m NaCl) as solvent. The reduced viscosity at different protein concentrations was calculated by the method described by Yang (1961). The reduced viscosity at a single-protein concentration (3 mg mL−1) was derived from the plot of protein concentration vs. reduced viscosity (ηRed).
Gel filtration profile
The gel filtration profile of total proteins extracted from threadfin bream meat was obtained using a Sepharose 6B gel packed in a column of 1.5 × 80 cm (diameter × height) using EB as solvent at room temperature. The bed volume of the column was 154 mL and the void volume as determined by using blue dextran was found to be 50 mL. A protein concentration of 3.5–4.0 mg mL−1 was loaded on to the column and eluted with EB at a flow rate of 30 mL h−1. Fractions of 3 mL were collected manually and the concentration of the proteins in the eluent was monitored by measuring the absorbance at 280 nm using a Bausch and Lomb UV/Vis spectrophotometer (Model 21-UVD, Austin, TX, USA). A plot of elution volume vs. absorbance at 280 nm for each fraction was obtained.
Sodium dodecyl sulphate polyacrylamide gel electrophoresis
Sodium dodecyl sulphate poly acrylamide gel electrophoresis (SDS-PAGE) of total proteins from unwashed and washed threadfin bream meat was carried out under reducing conditions according to the method of Laemmli (1970). Electrophoresis was carried out using polyacrylamide gel slabs of 10 × 8 cm (length × width) in a vertical gel electrophoresis unit (model Mighty small II, SE 250, Hoefer-Pharmacia Biotech, San Francisco, CA, USA). A discontinuous gel of acrylamide concentration of T% = 10, C% = 2.5 (for resolving gel) and T% = 4, C% = 2.5 (for stacking gel) was used:


The thickness of the gel was 0.75 mm. The run was carried out in a constant current mode at 2 mA per well using a Hoefer-Pharmacia Biotech, power pack (model PS 3000). A clear protein solution of 25–30 μg was loaded into the well. The standard molecular weight markers of wide range (205–14.2 kD) procured from Sigma (St Louis, MO, USA) were loaded separately. After the run, the gels were stained using Coomassie brilliant blue R-250 overnight. The gels were destained using an acetic acid–methanol mixture until the protein bands were clearly visible. The molecular weight of the bands obtained in the sample was approximated by measuring the relative mobility of the standard proteins in the molecular weight markers.
Water absorption capacity, fat absorption capacity and emulsion capacity
Water absorption capacity (WAC) was determined by the method described by Sosulki (1962) and expressed as g water g−1 dried material. Fat absorption capacity (FAC) was estimated by following the method described by Lin et al. (1974) and expressed as g oil g−1 dried material. Emulsion capacity (EC) of total proteins was determined according to the method of Swift et al. (1961) and expressed as mL oil mg−1 protein.
Preparation of heat-induced gel and gel strength measurement
The minced meat (100 g) was ground manually with 2.5% of salt in a pre-chilled pestle and mortar for 10 min. The resulting paste was packed in KrehalonTM (Kureha Chemical Industry Co. Ltd., Japan) casing (260 mm length, 48 mm diameter and 200 gauge thickness) without air pockets, using a hand stuffer. Prior to the stuffing of paste into the casing, one end of the casing was sealed and after stuffing the other end was sealed. The sealing was done with aluminium wire using a clipping machine. The sealed casings were heated at 90 ± 2 °C for 45 min in a water bath (Haake, model K10, Karlsruhe, Germany) and cooled in chilled water (5–6 °C) for 20 min. The gel was kept in a refrigerator (6–8 °C) overnight. The gel strength and expressible content of the gels were measured after keeping at ambient temperature for 1 h.
The gel strength of heat-induced gel was determined by using an Okada gellometer (Saitama Keki Seisa Kuso Co. Ltd., Tokyo, Japan) as described by Suzuki (1981). The expressible water content of the heat-induced gel was measured according to the method of Okada (1963).
Dynamic viscoelastic behaviour
The dynamic viscoelastic behaviour (DVB) of unwashed and washed threadfin bream meat in the temperature range of 30–90 °C was measured using a Carri-Med Controlled Stress Rheometer (CSR Carri-Med model CSL 500, Dorking, Surrey, UK) under oscillatory mode using 4 cm parallel plate measuring geometry. Four grams of meat ground with 2.5% of sodium chloride were used for DVB measurements. The gap between measuring geometry and Peltier plate was adjusted to 2000 μm. The temperature sweep was carried out by applying a small amplitude oscillation (0.0005 Rad) with a frequency of 1 Hz, which was in the linear viscoelastic region. A heating rate of 1 °C min−1 was achieved through the Peltier plate system during the heating regime. The applied sinusoidal stress was compared with the resultant sinusoidal strain. The two sine waves had a phase difference δ, which was used to give the elastic (storage modulus G′) and viscous (loss modulus G′′) elements of the gel. These two values along with tan δ (G′′/G′) were recorded simultaneously by the instrument. The average of three measurements is used for plotting.
Flow profile measurements (shear stress sweep)
The flow properties of protein solutions from unwashed and washed threadfin bream (thrice washed) meat were measured as a function of temperature using a Controlled Stress Rheometer (CSR Carri-Med model CSL 500) with flow software. Extraction buffer was used as the solvent for protein extraction. The concentration of protein in the samples was 10 mg mL−1 and the measurements were made at 30 and 40 °C. The sample was equilibrated for 5 min before the shearing experiment was started. The measuring geometry used was a 4-cm cone and plate with a truncation of 59 μm. The range of stress applied varied between 2 and 5 Pa depending on the angular velocity in the pre-shear experiment. The ascent and descent times were 2 min each, with a peak hold time of 1 min. Shear stress sweeps of the protein solutions at 30 and 40 °C were performed in triplicate and average values were taken for plotting. A flow curve was obtained by plotting log10 viscosity and log10 shear rate value.
Statistical analysis
All the results expressed are the mean of three measurements. Each measurement from a single batch and mean values were reported. Simple correlation was used to relate the number of washings with WAC, FAC, EC, gel strength and expressible water. The Kruskal–Wallis test was used to find out the significance of difference because of washing.
Results and discussion
The composition of unwashed threadfin bream meat showed a moisture content of 77.14% and total protein content of 18.95%. On washing three times, the moisture content increased, whereas the protein, fat and ash content decreased (Table 1). The increase in moisture content can be attributed to hydration of myofibrillar proteins (Suvanich et al., 2000). The reduction in ash content is attributed to the removal of water-soluble mineral constituents from the meat (Sijo et al., 2002).
Changes in total nitrogen, moisture, fat and ash during different washing cycles of pink perch meat
Number of washings . | Total protein (N × 6.25) [g (100 g)−1] . | Moisture [g (100 g)−1] . | Crude fat [g (100 g)−1] (wet weight) . | Ash [g (100 g)−1] (wet weight) . |
---|---|---|---|---|
Unwashed | 3.03 (0.005) | 77.14 (0.01) | 3.45 (0.03) | 1.13 (0.05) |
Once washed | 2.45 (0.01) | 81.25 (0.10) | 2.10 (0.01) | 0.36 (0.05) |
Thrice washed | 2.06 (0.01) | 84.24 (0.03) | 1.23 (0.01) | 0.15 (0.01) |
Number of washings . | Total protein (N × 6.25) [g (100 g)−1] . | Moisture [g (100 g)−1] . | Crude fat [g (100 g)−1] (wet weight) . | Ash [g (100 g)−1] (wet weight) . |
---|---|---|---|---|
Unwashed | 3.03 (0.005) | 77.14 (0.01) | 3.45 (0.03) | 1.13 (0.05) |
Once washed | 2.45 (0.01) | 81.25 (0.10) | 2.10 (0.01) | 0.36 (0.05) |
Thrice washed | 2.06 (0.01) | 84.24 (0.03) | 1.23 (0.01) | 0.15 (0.01) |
n = 3; values in parenthesis indicate standard deviation.
Changes in total nitrogen, moisture, fat and ash during different washing cycles of pink perch meat
Number of washings . | Total protein (N × 6.25) [g (100 g)−1] . | Moisture [g (100 g)−1] . | Crude fat [g (100 g)−1] (wet weight) . | Ash [g (100 g)−1] (wet weight) . |
---|---|---|---|---|
Unwashed | 3.03 (0.005) | 77.14 (0.01) | 3.45 (0.03) | 1.13 (0.05) |
Once washed | 2.45 (0.01) | 81.25 (0.10) | 2.10 (0.01) | 0.36 (0.05) |
Thrice washed | 2.06 (0.01) | 84.24 (0.03) | 1.23 (0.01) | 0.15 (0.01) |
Number of washings . | Total protein (N × 6.25) [g (100 g)−1] . | Moisture [g (100 g)−1] . | Crude fat [g (100 g)−1] (wet weight) . | Ash [g (100 g)−1] (wet weight) . |
---|---|---|---|---|
Unwashed | 3.03 (0.005) | 77.14 (0.01) | 3.45 (0.03) | 1.13 (0.05) |
Once washed | 2.45 (0.01) | 81.25 (0.10) | 2.10 (0.01) | 0.36 (0.05) |
Thrice washed | 2.06 (0.01) | 84.24 (0.03) | 1.23 (0.01) | 0.15 (0.01) |
n = 3; values in parenthesis indicate standard deviation.
A considerable reduction in the NPN content of meat was observed after three washings (Table 2). Washing is a necessary operation to prevent myofibrillar protein denaturation during frozen storage and the gel strength continues to improve as the number of leaching cycles increases (Matsumoto & Noguchi, 1992). The NPN content of thrice washed meat was found to be 83.33 mg 100 g−1. Hassan & Mathew (1999) reported an NPN content of 220 mg 100 g−1 for thrice washed threadfin bream meat. The NPN comprises free amino acids and trimethylamine oxide, which are water soluble, and hence a reduction could be observed. Joseph & Perigreen (1986) reported a 50% loss in NPN content during the washing of catfish mince. Other substances removed during washing include blood, pigments, odours, enzymes, mucus and some water-soluble proteins (Chou, 1993; Hoke, 1993).
Changes in non-protein nitrogen, pH and functional properties of proteins from pink perch meat during different water washing cycles
Number of washings . | Unwashed . | Once washed . | Thrice washed . |
---|---|---|---|
Non-protein nitrogen (mg 100 g−1 of meat) | 315.97 (3.47) | 170.14 (3.48) | 83.33 (2.28) |
pH | 7.00 (0.05) | 7.10 (0.10) | 7.15 (0.05) |
Reduced viscosity at zero protein concentration (dL g−1) | 0.59 | 0.59 | 0.74 |
Water absorption capacity (g water g−1dried material) | 4.12 (0.05) | 7.83 (0.62) | 9.51 (0.04) |
Fat absorption capacity (g oil g−1dried material) | 4.58 (0.10) | 5.51 (0.40) | 6.43 (0.22) |
Emulsion capacity (mL of oil mg−1 protein) | 0.26 (0.001) | 0.36 (0.002) | 0.46 (0.001) |
Gel strength (g cm) | 304.93 (12.61) | 511.85 (15.84) | 604.47 (18.61) |
Expressible water (%) | 29.47 (0.02) | 21.12 (0.40) | 19.73 (0.18) |
Number of washings . | Unwashed . | Once washed . | Thrice washed . |
---|---|---|---|
Non-protein nitrogen (mg 100 g−1 of meat) | 315.97 (3.47) | 170.14 (3.48) | 83.33 (2.28) |
pH | 7.00 (0.05) | 7.10 (0.10) | 7.15 (0.05) |
Reduced viscosity at zero protein concentration (dL g−1) | 0.59 | 0.59 | 0.74 |
Water absorption capacity (g water g−1dried material) | 4.12 (0.05) | 7.83 (0.62) | 9.51 (0.04) |
Fat absorption capacity (g oil g−1dried material) | 4.58 (0.10) | 5.51 (0.40) | 6.43 (0.22) |
Emulsion capacity (mL of oil mg−1 protein) | 0.26 (0.001) | 0.36 (0.002) | 0.46 (0.001) |
Gel strength (g cm) | 304.93 (12.61) | 511.85 (15.84) | 604.47 (18.61) |
Expressible water (%) | 29.47 (0.02) | 21.12 (0.40) | 19.73 (0.18) |
n = 3; values in parenthesis indicate standard deviation.
Changes in non-protein nitrogen, pH and functional properties of proteins from pink perch meat during different water washing cycles
Number of washings . | Unwashed . | Once washed . | Thrice washed . |
---|---|---|---|
Non-protein nitrogen (mg 100 g−1 of meat) | 315.97 (3.47) | 170.14 (3.48) | 83.33 (2.28) |
pH | 7.00 (0.05) | 7.10 (0.10) | 7.15 (0.05) |
Reduced viscosity at zero protein concentration (dL g−1) | 0.59 | 0.59 | 0.74 |
Water absorption capacity (g water g−1dried material) | 4.12 (0.05) | 7.83 (0.62) | 9.51 (0.04) |
Fat absorption capacity (g oil g−1dried material) | 4.58 (0.10) | 5.51 (0.40) | 6.43 (0.22) |
Emulsion capacity (mL of oil mg−1 protein) | 0.26 (0.001) | 0.36 (0.002) | 0.46 (0.001) |
Gel strength (g cm) | 304.93 (12.61) | 511.85 (15.84) | 604.47 (18.61) |
Expressible water (%) | 29.47 (0.02) | 21.12 (0.40) | 19.73 (0.18) |
Number of washings . | Unwashed . | Once washed . | Thrice washed . |
---|---|---|---|
Non-protein nitrogen (mg 100 g−1 of meat) | 315.97 (3.47) | 170.14 (3.48) | 83.33 (2.28) |
pH | 7.00 (0.05) | 7.10 (0.10) | 7.15 (0.05) |
Reduced viscosity at zero protein concentration (dL g−1) | 0.59 | 0.59 | 0.74 |
Water absorption capacity (g water g−1dried material) | 4.12 (0.05) | 7.83 (0.62) | 9.51 (0.04) |
Fat absorption capacity (g oil g−1dried material) | 4.58 (0.10) | 5.51 (0.40) | 6.43 (0.22) |
Emulsion capacity (mL of oil mg−1 protein) | 0.26 (0.001) | 0.36 (0.002) | 0.46 (0.001) |
Gel strength (g cm) | 304.93 (12.61) | 511.85 (15.84) | 604.47 (18.61) |
Expressible water (%) | 29.47 (0.02) | 21.12 (0.40) | 19.73 (0.18) |
n = 3; values in parenthesis indicate standard deviation.
A marginal increase in pH of the threadfin bream meat as a function of the number of washings was recorded (Table 2). The pH of unwashed and washed catfish mince indicated a higher value for washed mince (Suvanich et al., 2000). The higher pH of washed meat compared to that of unwashed meat may be because of the removal of free acidic amino acids and other water-soluble acidic substances (Suvanich & Marshall, 1998).
The measurement of viscosity of protein solutions provides information on size, shape and gross conformation (Van Holde, 1985). The reduced viscosity as a function of protein concentration showed an increase in value with an increase in protein concentration (Table 2). The extrapolation to zero protein concentration showed higher intrinsic viscosity values for thrice washed meat (0.74 dL g−1) compared to those of once washed and unwashed meat (0.59 dL g−1). The variation in the reduced viscosity value at any given concentration of protein solution is influenced by various factors such as nature of protein and other composite substances. As the viscosity measurements in the present study were made in total protein solution, the interpretation in terms of size, shape and denaturation becomes difficult.
The gel filtration profile of total proteins from unwashed meat indicated three fractions (Fig. 1a). The concentration of fraction I was higher than the other two fractions. The removal of water-soluble constituents (fraction III) in meat was confirmed by the gel filtration profiles of washed meat (Fig. 1b and c). The fraction eluting at an elution volume of 137 mL was not present in the gel filtration profile of once washed and thrice washed meat. This removal of low molecular weight components will concentrate the myofibrillar proteins and the properties will be altered because of concentration (Lin & Park, 1996; Chen et al., 1997).
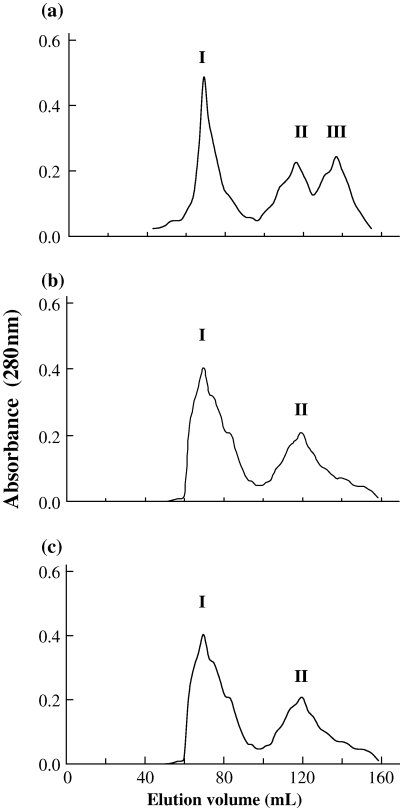
Gel filtration profile of total proteins from: (a) unwashed, (b) once washed and (c) thrice washed threadfin bream meat (peaks I, II and III represent fractions I, II and III, respectively).
The SDS-PAGE pattern of total proteins from fresh unwashed meat revealed multiple bands in the molecular weight range of 205–14.2 kDa (Fig. 2). The intensity and concentration of these bands varied. The thick band in the 205 kDa range is the myosin heavy chain. The 45 and 36 kDa components were also predominant compared to other bands. The electrophoretic pattern of washed meat clearly indicated concentration of the myosin heavy chain and the concentration increased with the number of washings. The pattern of washed meat also showed a reduction in concentration of the 55 kDa (Fig. 2, lane c) component compared to that of unwashed meat. Under reduced conditions, the appearance of multiple bands is a common feature of many protein fractions. In the present study, although the gel filtration profile indicated the removal of low molecular weight fractions, the same could not be confirmed by the SDS-PAGE pattern possibly because of the appearance of a number of subunits of different protein fractions.
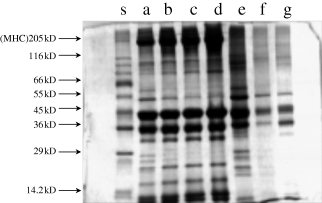
SDS-PAGE pattern of total proteins from unwashed and washed threadfin bream meat. Lane s, standard markers; a, unwashed meat; b, once washed meat; c, twice washed meat; d, thrice washed meat; e, first wash water; f, second wash water; g, third wash water (standard markers include 205 kDa myosin heavy chain from rabbit muscle; 116 kDa β-galactosidase; 97 kDa phosphorylase; 66 kDa albumin bovine serum; 55 kDa glutamic dehydrogenase; 45 kDa ovalbumin; 36 kDa gluceraldehyde-3-phosphate dehydrogenase).
The hydration ability of freeze-dried threadfin bream meat (unwashed and washed) has been assessed (Table 2). The hydration of protein molecules is influenced by the number of large residues it possesses and their conformational status. The water absorption capacity of the unwashed sample was found to be 4.12 g water g−1 dried material and it increased more than twofold after three washings. The water absorption capacity values from unwashed threadfin bream meat were much higher than those reported for shark meat (Sijo, 2000) and lower than those for prawn meat (Shamasundar & Prakash, 1994a). The higher value for washed meat is related to the concentration of myofibrillar proteins and number of polar residues available for hydration.
The fat absorption capacity of unwashed threadfin bream meat was found to be 4.58 g oil g−1dried material, which increased to a value of 6.43 g oil g−1 dried material for thrice washed meat (Table 2). Fat absorption capacity is purely a physical phenomenon wherein oil becomes entrapped by the dried protein matrix. FAC mainly depends on the size of the meat particle used and the experimental conditions. The increase in fat absorption capacity of washed meat is mainly because of the concentration of myofibrillar proteins, which are large molecules. The fat absorption capacity of threadfin bream meat was higher than shark meat (Sijo, 2000) and other vegetable proteins (Akubor & Obiegbuna, 1999).
The emulsion capacity of total proteins from unwashed threadfin bream meat was found to be 0.26 mL oil mg−1 protein (Table 2). The emulsion capacity of total proteins as affected by washing showed an increasing trend, reaching a value of 0.46 mL oil mg−1 protein after three washings. An emulsion is a three-phase system, where protein acts as an emulsifier reducing the interfacial tension between the water and oil components (Dickinson & Stainsby, 1982). The ability of protein molecules to emulsify a given volume of oil mainly depends on the surface hydrophobic groups, which can orient readily in the aqueous phase thus lowering the interfacial energy (Kato & Nakai, 1980). The emulsion capacity of proteins from unwashed meat was higher than the value reported for shrimp (Shamasundar & Prakash, 1994b). It is well documented that myofibrillar proteins are the major contributor to emulsification properties (Gordon & Barbut, 1992). Repeated washing of threadfin bream meat could concentrate myofibrillar proteins and hence increase the emulsion capacity value for proteins from washed meat. It appears that sarcoplasmic proteins in unwashed meat had a lower ability to emulsify the oil.
The strength of gels prepared from fresh unwashed meat was found to be 305 g cm. Subjecting the meat to one washing cycle increased the gel strength to 512 g cm. Further washing up to three cycles increased the gel strength to 604 g cm (Table 2) and there existed a significant positive correlation between the number of washings and the gel strength (Table 3). The gel strength indicates the ability of protein molecules to undergo ordered aggregation with entrapment of water. The myofibrillar proteins, especially myosin and actomyosin, are known to play a dominant role in gelation of fish meat and shows variation with species (Shimizu et al., 1981). Myofibrillar protein fractions are capable of forming gels with different rheological properties (Foegeding et al., 1991). The increase in gel strength of the meat because of washing can be related to the concentration of myofibrillar proteins (Chen et al., 1997). With continued washing, the gel strength increased up to two washing cycles and thereafter levelled off (Lee, 1986).
Correlation coefficient of different parameters analysed from unwashed and washed pink perch meat
Parameters compared . | r-value . |
---|---|
Washing vs. water absorption capacity | 0.9194* |
Washing vs. fat absorption capacity | 0.9814** |
Washing vs. emulsion capacity | 0.9820** |
Washing vs. gel strength | 0.9183 |
Washing vs. expressible water | −0.8357 |
Water absorption capacity vs. fat absorption capacity | 0.9778** |
Water absorption capacity vs. emulsion capacity | 0.9772** |
Water absorption capacity vs. gel strength | 0.9999*** |
Water absorption capacity vs. expressible water | −0.9843** |
Fat absorption capacity vs. emulsion capacity | 0.9999*** |
Fat absorption capacity vs. gel strength | 0.9772** |
Fat absorption capacity vs. expressible water | −0.9256* |
Emulsion capacity vs. gel strength | 0.9766** |
Emulsion capacity vs. expressible water | −0.9244* |
Gel strength vs. expressible water | −0.9848** |
Parameters compared . | r-value . |
---|---|
Washing vs. water absorption capacity | 0.9194* |
Washing vs. fat absorption capacity | 0.9814** |
Washing vs. emulsion capacity | 0.9820** |
Washing vs. gel strength | 0.9183 |
Washing vs. expressible water | −0.8357 |
Water absorption capacity vs. fat absorption capacity | 0.9778** |
Water absorption capacity vs. emulsion capacity | 0.9772** |
Water absorption capacity vs. gel strength | 0.9999*** |
Water absorption capacity vs. expressible water | −0.9843** |
Fat absorption capacity vs. emulsion capacity | 0.9999*** |
Fat absorption capacity vs. gel strength | 0.9772** |
Fat absorption capacity vs. expressible water | −0.9256* |
Emulsion capacity vs. gel strength | 0.9766** |
Emulsion capacity vs. expressible water | −0.9244* |
Gel strength vs. expressible water | −0.9848** |
*P < 0.05, **P < 0.01 and ***P < 0.001.
Correlation coefficient of different parameters analysed from unwashed and washed pink perch meat
Parameters compared . | r-value . |
---|---|
Washing vs. water absorption capacity | 0.9194* |
Washing vs. fat absorption capacity | 0.9814** |
Washing vs. emulsion capacity | 0.9820** |
Washing vs. gel strength | 0.9183 |
Washing vs. expressible water | −0.8357 |
Water absorption capacity vs. fat absorption capacity | 0.9778** |
Water absorption capacity vs. emulsion capacity | 0.9772** |
Water absorption capacity vs. gel strength | 0.9999*** |
Water absorption capacity vs. expressible water | −0.9843** |
Fat absorption capacity vs. emulsion capacity | 0.9999*** |
Fat absorption capacity vs. gel strength | 0.9772** |
Fat absorption capacity vs. expressible water | −0.9256* |
Emulsion capacity vs. gel strength | 0.9766** |
Emulsion capacity vs. expressible water | −0.9244* |
Gel strength vs. expressible water | −0.9848** |
Parameters compared . | r-value . |
---|---|
Washing vs. water absorption capacity | 0.9194* |
Washing vs. fat absorption capacity | 0.9814** |
Washing vs. emulsion capacity | 0.9820** |
Washing vs. gel strength | 0.9183 |
Washing vs. expressible water | −0.8357 |
Water absorption capacity vs. fat absorption capacity | 0.9778** |
Water absorption capacity vs. emulsion capacity | 0.9772** |
Water absorption capacity vs. gel strength | 0.9999*** |
Water absorption capacity vs. expressible water | −0.9843** |
Fat absorption capacity vs. emulsion capacity | 0.9999*** |
Fat absorption capacity vs. gel strength | 0.9772** |
Fat absorption capacity vs. expressible water | −0.9256* |
Emulsion capacity vs. gel strength | 0.9766** |
Emulsion capacity vs. expressible water | −0.9244* |
Gel strength vs. expressible water | −0.9848** |
*P < 0.05, **P < 0.01 and ***P < 0.001.
The expressible water content of the prepared gels showed an inverse correlation with gel strength in all the samples (Table 3). Expressible water content is an index of water holding capacity and is a sensitive indicator of changes in the electrical charge and structure of muscle proteins in which pH plays an important role. The expressible water content of gels prepared from unwashed meat was 29.47% and decreased to 19.73% after three washings (Table 2). A close relationship between gel texture and water holding capacity of cooked gels has been reported (Lee, 1984). Gel strength indicates the protein per unit weight available to form a gel, whereas expressible water is a reflection of the physical properties of proteins that are related to their electrical charge and structure (Pacheo-Aguilar et al., 1989). The unwashed meat had a higher expressible water content than washed meat indicating the superior water holding capacity of washed meat.
Gelation of muscle proteins results from the transformation of an amorphous viscous solution to a three-dimensional elastic network. Hence, changes in stress–strain relationships during the gelation process could be monitored by rheological parameters (Egelandsdal et al., 1995). The dynamic rheological test or small strain gel rigidity test has been widely used to study the heat-induced gelation of myofibrillar proteins (Visessanguan et al., 2000). The storage modulus (G′) is a good index for the gel-forming ability of food proteins. Gelation of food proteins is a function of the nature of the proteins and processing condition such as pH, ionic strength, binding agent and heating regime (Wang & Xiong, 1998).
The dynamic viscoelastic behaviour of unwashed and washed threadfin bream meat revealed that the storage modulus value (G′) increased with increasing temperature up to 70 °C in all the samples with a maximum value between 60 and 80 °C, indicating structure build-up or network formation (Fig. 3). The high rate of increase in the G′ value below 60 °C is likely to involve protein unfolding and formation of disulphide and hydrophobic interaction (Niwa, 1992). The increase in storage modulus values is an indication of a structure build-up reaction attributed to ordered aggregation by the application of thermal energy (Ziegler & Foegeding, 1990). The viscous element (G′′) followed the same trend as that of G′ but the intensity of ordered aggregation was much higher leading to lower G′′ values.
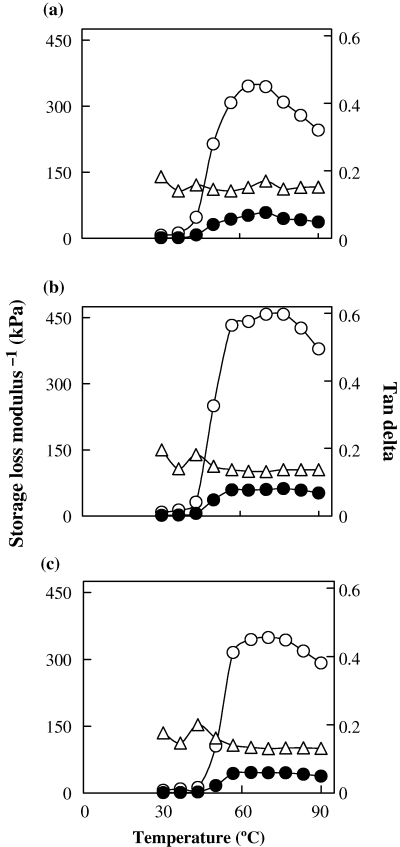
Dynamic viscoelastic behaviour of fresh threadfin bream meat in the temperature range of 30–90 °C: (a) unwashed, (b) once washed, (c) thrice washed. -○- g′, -●- g′′, -Δ- Tan delta
The gelation profile of once washed meat indicated a higher G′ value as compared to unwashed and thrice washed meat. The maximum G′ value of 458 kPa in the case of once washed meat is an indication that myofibrillar proteins from threadfin bream have the ability to form a highly ordered network leading to higher gel strength. The maximum G′ value for thrice washed meat was found to be marginally higher (by 4 kPa) than unwashed meat. Theoretically, one would have expected a higher G′ maximum value for thrice washed meat. The reason for not observing this could be because of higher moisture content in the meat sample arising from increased hydration because of the more prolonged contact with water.
The results of the gel strength experiment and data from the oscillation profile are slightly at variance especially for thrice washed meat. Wood (1979) showed that the consumer assessment of hardness of gel correlated more highly with the rupture strength than with the elastic modulus. Considering the moisture levels of three samples, the unwashed meat had a lower moisture content yet ended up with a lower gel strength and G′ maximum value. On the other hand, once washed meat (where the moisture content was 81.25%) had a higher G′ maximum value and higher gel strength values. The moisture content of thrice washed meat was 84.24%, which resulted in a high gel strength value of 604 g cm, but the G′ maximum value was only slightly higher than unwashed meat. This clearly indicates that the force at rupture will be higher despite a higher moisture content, whereas the small strain test did not give a clear indication. Hamann & Lanier (1986) indicated modulus values for the small strain test were not good predictors of rupture stress because protein degradation may not influence modulus values when strongly influencing rupture values. The relationship of indirect test to the actual expressed properties is not fully understood. It is possible that a surimi sample may display undesirable traits in an indirect test and yet perform quite well in the product application (Lanier, 1992).
The sol–gel transition as indicated by tan δ values revealed three transition points for all the samples (Fig. 3). The transitions occurred at 36.7, 43.3 and 70.0 °C. The transitions at 36.7 and 43.3 °C have been attributed to the tail of the myosin molecule and the transition at the higher temperature to that of the head of the myosin molecule (Sano et al., 1988).
Gelation profile using oscillation software provides useful data on structure build-up reaction and sol–gel transition. The flow profile of the myofibrillar proteins at a specific temperature provides information on resistance to shearing thereby indicating structural impairment, if any. The flow behaviour of total proteins from unwashed and washed threadfin bream meat at 30 °C revealed pseudoplastic behaviour (Fig. 4a and c). As there was a minimal thixotropic area between the up curve and the down curve, there appears to be little damage to the structure owing to shearing. The change in structure could be either a build-up (in which the process is called ‘rheopexy’ or ‘antithixotrophy’) or a breakdown. It could also be the overall result of both processes occurring simultaneously: shearing causes the structure to break down in the sample, but at the same time the natural behaviour of the sample is to build-up structure; hence, the overall picture is dictated by the relative ratio of the two processes (Barnes et al., 1989). The flow profile of proteins at 40 °C from unwashed and washed meat showed a different behaviour (Fig. 4b and d). In both the samples the structure impairment of molecules was evident as the down curve data showed higher viscosity values at low shear rates. The increase in viscosity could be either owing to coagulation or owing to higher protein–protein interactions on thermal treatment and perhaps could be the starting point for the gelation process as evidenced by tan δ values in DVB measurements. The down curve data of proteins from washed meat showed relatively higher viscosity values at low shear rates. This differential behaviour of flow profile could be because of the presence of sarcoplasmic proteins in unwashed meat. The shear stress sweep of acidified shark gel dispersion at a protein concentration of 6.8 mg mL−1 showed large thixotropic areas at 40, 50 and 60 °C (Venugopal et al., 2002). In the present study, flow measurements could not be carried out at 50 and 60 °C as there was extensive coagulation and difficulty in shearing the sample.
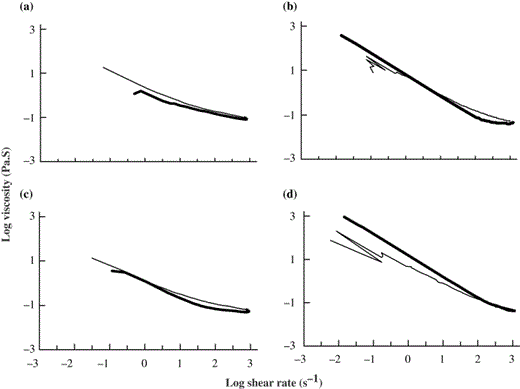
Flow profile of total proteins from fresh threadfin bream meat using EB as solvent: (a) proteins from unwashed meat at 30 °C; (b) proteins from unwashed meat at 40 °C; (c) proteins from washed meat at 30 °C; (d) Proteins from washed meat at 40 °C. The samples were equilibrated for 5 min on the Peltier plate before shearing started. The data represents both up curve and down curve. Bold line represents upcurve.
Conclusion
The present study clearly demonstrates that washing threadfin bream meat removes the sarcoplasmic proteins and low molecular weight components and concentrates the myofibrillar proteins thereby increasing the water absorption capacity, fat absorption capacity and emulsion capacity of proteins. The gel strength can also be enhanced by water washing, thereby improving the textural properties of the final product. The washing cycle can be restricted to one wash as the proteins from once washed meat were found to have better functional properties than those from unwashed and thrice washed meat. The water washing of threadfin bream meat for one washing cycle could not only improve functional proteins, but also could reduce water requirement considerably.