-
PDF
- Split View
-
Views
-
Cite
Cite
Kelvin K T Goh, Aiqian Ye, Nicola Dale, Characterisation of ice cream containing flaxseed oil, International Journal of Food Science and Technology, Volume 41, Issue 8, October 2006, Pages 946–953, https://doi.org/10.1111/j.1365-2621.2006.01151.x
- Share Icon Share
Abstract
Five batches of a 12% (w/w) fat ice cream formulation were prepared using different amounts of flaxseed oil (0%, 3%, 6%, 9% and 12% w/w) to replace milkfat. Meltdown rates, texture of ice cream, particle size of fat globules and microstructure of the ice cream were determined. Increasing the proportion of flaxseed oil in ice cream resulted in an increase in the meltdown rate and a decrease in the ice cream hardness. These were attributed to the low melting temperature of flaxseed oil and the varying extent of fat flocculation. In ice cream containing a high proportion of milkfat, the fat globules formed large particles largely due to flocculation rather than partial-coalescence. The extent of fat flocculation decreased as the proportion of flaxseed oil was increased. Evidence of fat coalescence was observed in ice cream containing 12% flaxseed oil. The fat flocculates stabilised the air cells and strengthened the foam structure of the ice cream.
Introduction
Alpha-linolenic acids (18:3n-3; ALA) have been reported to play a major role in the prevention and treatment of chronic diseases such as hypertension, coronary heart disease, diabetes, arthritis, cancer, autoimmune diseases and other inflammatory diseases (Simopoulos, 1999, Larsson et al., 2004). In today's western diet, there is a low intake of n-3 fatty acids compared with linoleic acids (18:2n-6; LA) (James et al., 2000), with a ratio of c. 20–30:1 instead of the traditional range of c. 1–2:1 (Simopoulos, 1999). Although it is recognised that ALA from plant source is not equivalent in terms of the biologically active eicosapentaenoic acid (EPA) and decosahexaenoic acid (DHA) found in marine oils (Francois et al., 2003), the role of ALA remains important for long-term dietary intake as well as for curbing the problems of vitamin E deficiency in diets (Simopoulos, 1999). Flaxseed oil obtained from the seed of flax (Linum usitatissimum) is an excellent source of alpha-linoleic acid and present in c. 60% of total fatty acids. An effective avenue to increase the intake of n-3 fatty acids is through the incorporation of flaxseed oil in food products (Oomah, 2001). Unfortunately, the high degree of unsaturation of n-3 fatty acids means that they can become highly susceptible to rancidity, due to factors such as heat, light and oxygen, during processing and storage. A food system that is deemed to be ideal for incorporating flaxseed oil is ice cream because of its low storage temperature (−18 °C). However, the very low melting temperature of flaxseed oil (≤12 °C) may result in the formation of a poor ice cream structure and hence, affect its textural and functional properties. The concept of incorporating flaxseed oil in ice cream has been cited in a relatively recent article (Pszczola, 2002). However, research on the physicochemical properties of ice cream incorporated with flaxseed oil has not been reported to date. The aim of this study was to characterise the changes that occur in a premium ice cream formulation (containing 12% w/w fat) made with different proportions of milkfat and flaxseed oil.
Materials and methods
Ice cream processing
The ice cream mix consisted of 12% (w/w) fat (milkfat from Fonterra, New Zealand and flaxseed oil from Functional Whole Foods, New Zealand), 11.5% (w/w) skim milk powder (Fonterra, Auckland, New Zealand), 11% (w/w) sucrose (standard granulated 1A sugar, New Zealand Sugar Company, Auckland, New Zealand), 5.2% (w/w) Avonsweet 62DE glucose syrup (Penford, Auckland, New Zealand), 0.3% emulsifier/stabiliser mix (Cremodan SE 30; Danisco, Auckland, New Zealand) and 60% (w/w) water. Six batches of 20 kg ice cream mixes were prepared based on the above formulation except that the fat portion consisted of different proportion of milkfat and flaxseed oil to achieve a 12% fat ice cream. The combination of the different proportion of milkfat and flaxseed oil are given as % milkfat:% flaxseed oil in the following order; 12:0, 10:2, 8:4, 6:6, 3:9 and 0:12. The ingredients were reconstituted at c. 50 °C, homogenised using a two-stage homogeniser (Rannie, Copenhagen, Denmark) with the first stage operating at 14 and 3.5 MPa for the second stage. The mix was then pasteurised at 86 °C for 15 s, cooled to 5 °C and aged at the same temperature for c. 20 h. The mix was pumped through a continuous ice cream freezer (Hoyer KF-80, Tetra Pak Hoyer, Hoejbjerg, Denmark) at rate of 30 L h−1 with a dasher speed and cylinder pressure set at 700 rpm and 0.4 MPa, respectively. A target overrun of 75 ± 2% was achieved for all the ice cream mixes. The extruded ice cream samples were collected in 105 mm × 44 mm × 52 mm laminated cardboard boxes and hardened at −30 °C for at least 24 h before transferring to a −18 °C freezer.
Procedure for heat-shock
Ice cream samples were subjected to temperature abuse according to the following daily regime over a 3-day period: 20 °C for 30 min, −18 °C for 7 h, 20 °C for 30 min, −18 °C overnight (16 h).
Relative viscosity of ice cream mixes
The relative viscosity (ηr) of the mix was measured using a Cannon Fenske capillary viscometer (Viscometer no. 200, F188, Cannon Instrument Co., PA, USA) at 20 °C with water as a reference sample. Each sample (5 mL) was loaded into the capillary viscometer and allowed to equilibrate for c. 5 min before measuring the efflux time. The density of the ice cream mix was determined using a pycnometer. The relative viscosity was obtained using the equation:

where ρs and ts are the density and efflux time of the ice cream mix; and ρw and tw are the density and efflux time of water. All measurements were carried out in triplicate.
Rate of meltdown
All ice cream blocks from the −18 °C freezer with similar weights (158 ± 5 g) were placed on a 1 cm wire mesh screen wire rack at 20 ± 0.5 °C and the weight of the melt was recorded at every 10 min interval over 60 min. The meltdown test was performed in triplicate. The above procedure for meltdown test was also carried out on heat-shock ice cream.
Determination of average particle size and size distribution
The ice cream samples (both non-heat-shock and heat-shock) were melted at 20 °C. The melted ice cream samples and ice cream mixes were each dispersed in Milli-Q water (Millipore, Milford, MA, USA), and a 2% (w/v) Sodium dodecyl sulphate (SDS) (Merck, Darmstadt, Germany) and 50 mm ethylenediaminetetracetic acid (EDTA) (Merck) solution. The latter was to dissociate any flocculated fat globules prior to fat globule size measurements. The samples (including the melted ice cream without SDS and EDTA treatment) were analysed for their fat droplet size distribution. A Malvern MasterSizer MSE (Malvern Instruments Ltd, Malvern, Worcestershire, UK) was used to determine the average diameter (d43) of the ice cream fat globules and/or oil droplets. The parameters that were used to analyse the particle size distribution were defined by the presentation code 2NAD. The relative refractive index (N), i.e. the ratio of the refractive index of the emulsion droplets (1.456) to that of the dispersion medium (1.33), was 1.095. The absorbance value of the fat globules and oil droplets was 0.001. Droplet size measurements are reported as weight-average mean diameters, d43. The d43 is defined as ∑nidi4/∑nidi3, where ni is the number of particles with diameter di. Mean particle diameters were calculated from measurements.
Confocal laser scanning microscopy
A Leica (Heidelberg, Germany) confocal laser scanning microscope (CLSM) with a chosen objective lens and an Ar/Kr laser with an excitation line of 488 nm (in such a way that only the fluorescent wavelength band could reach the detector) were used to examine the ice cream emulsions. The melted ice cream samples were prepared as described above. Approximately 3 mL sample was mixed with the fluorescent dye, Nile Blue (Merck) in a test tube. The mixture was then placed on a slide covered with a cover slip and observed under the microscope.
Viscosity of melted ice cream
Viscosity measurements of the melted ice cream samples were performed in a steady state flow using a controlled stress rheometer (Rheometrics SR-5000, Rheometrics Instruments, Piscataway, NJ, USA) equipped with cone and plate geometry. Cone diameter was 40 mm and cone angle 4°. All measurements were performed in a steady stress sweep mode at a temperature of 20 ± 0.1 °C about 30 min after the samples were melted. The data were plotted in the logarithmic scale based on viscosity as a function of shear rate.
Texture analysis
The texture of the ice cream samples was analysed using a TA.XT2 Texture Analyser (Stable Micro Systems, Godalming, UK), fitted with a 11 mm diameter stainless steel. Hardness was measured as the maximum force (N) required to penetrate the sample to a depth of 20 mm at a test speed of 0.5 mm s−1 at ambient temperature (c. 20 °C).
Statistical analysis
Statistical analysis of the data was carried by Analysis of Variance (Anova) using Minitab statistical software (Release 14) where appropriate.
Results and discussion
Particle size distribution and viscosity of ice cream mix
The size distribution of the fat globules/oil droplets in all the ice cream mixes showed monomodal distributions with no evidence of flocculation (Fig. 1). The average diameters of the fat globules (d43) in the aged ice cream mixes containing different proportions of milkfat and flaxseed oil were not significantly different from each other (P-value = 0.124 and were c. 1 μm in size; Table 1). The results suggested that the fat globules/oil droplets formed after homogenisation were effectively stabilised by the proteins and the emulsifiers (mono- and diglycerides). During ageing, it is known that the added emulsifiers competitively displace proteins from the fat globule at the interfacial layer (Goff, 2000, Krog, 1998).
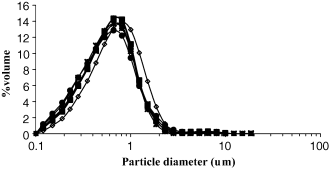
Particle size distribution of fat globules/agglomerates in ice cream mixes containing different milkfat to flaxseed oil ratios measured at room temperature (c. 20 °C). The six batches of ice cream mixes containing different combinations of % milkfat:% flaxseed oil in a 12% w/w fat ice cream are denoted as 12:0 (), 12:2 (◼), 8:4 (▲), 6:6 (×), 3:9 (*) and 0:12 (•).
Average particle size (d43) of fat globules/oil droplets in ice cream mixes containing different ratios of milkfat to flaxseed oil (±SE, n = 2)
Milkfat:flaxseed ratio (μm) . | Average particle size (d43) . |
---|---|
12:0 | 1.00 ± 0.03 |
10:2 | 1.04 ± 0.20 |
8:4 | 0.85 ± 0.00 |
6:6 | 1.08 ± 0.01 |
3:9 | 0.74 ± 0.005 |
0:12 | 0.80 ± 0.04 |
Milkfat:flaxseed ratio (μm) . | Average particle size (d43) . |
---|---|
12:0 | 1.00 ± 0.03 |
10:2 | 1.04 ± 0.20 |
8:4 | 0.85 ± 0.00 |
6:6 | 1.08 ± 0.01 |
3:9 | 0.74 ± 0.005 |
0:12 | 0.80 ± 0.04 |
Average particle size (d43) of fat globules/oil droplets in ice cream mixes containing different ratios of milkfat to flaxseed oil (±SE, n = 2)
Milkfat:flaxseed ratio (μm) . | Average particle size (d43) . |
---|---|
12:0 | 1.00 ± 0.03 |
10:2 | 1.04 ± 0.20 |
8:4 | 0.85 ± 0.00 |
6:6 | 1.08 ± 0.01 |
3:9 | 0.74 ± 0.005 |
0:12 | 0.80 ± 0.04 |
Milkfat:flaxseed ratio (μm) . | Average particle size (d43) . |
---|---|
12:0 | 1.00 ± 0.03 |
10:2 | 1.04 ± 0.20 |
8:4 | 0.85 ± 0.00 |
6:6 | 1.08 ± 0.01 |
3:9 | 0.74 ± 0.005 |
0:12 | 0.80 ± 0.04 |
The data from the viscometric measurement of the ice cream mixes showed a decrease in the relative viscosity as the proportion of flaxseed oil was increased (Fig. 2). This may be explained by the different degree of fat crystallisation occurring in the milkfat and the flaxseed oil globules at the refrigeration temperature (c. 5 °C). The flaxseed oil has a high percentage of unsaturated fat (c. 80% w/v) consisting of 24% linoleic acid (18:2) and 76% is alpha linolenic acid (18:3). In the 18-carbon fatty acid series, the melting temperatures of linoleic acid and alpha linolenic acid are at 5 °C and 11 °C, respectively (Wood et al., 2004). Therefore, it can be expected that an ice cream mix which had a higher amount of milkfat would have more fat crystals formed during the ageing process when compared with an ice cream mix which had a higher proportion of flaxseed oil and vice versa. Milkfat globule which has a higher amount of crystallised fat can be described to behave like a hard sphere (hence, the ability to resist deformation during shear) than the flaxseed oil droplet which is likely to occur as a fluid and is described as a ‘soft’ sphere (hence, likely to be deformed under shear). This explains why a higher relative viscosity was obtained in the ice cream containing a higher proportion of milkfat than that containing largely of flaxseed oil.
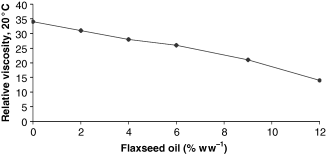
Relative viscosity of ice cream mixes containing different milkfat:flaxseed oil ratios measured by capillary viscometer at 20 °C with water regarded as the solvent.
Ice cream meltdown properties
The weights of the melted ice cream obtained from the non-heat-shock and heat-shock samples, recorded at every 10 min interval over a 60 min period in a 20 °C room, are shown in Fig. 3. For non-heat-shock ice cream (Fig. 3a), the meltdown profiles among the ice cream samples containing ≤4% (w/w) flaxseed oil (in a 12% fat ice cream formulation) appeared relatively similar. However, the rate of meltdown increased markedly when the amount of flaxseed oil was increased to 6% (w/w). Further increases in the meltdown rate were observed in the 9% (w/w) and 12% (w/w) flaxseed oil ice cream, respectively. As for the heat-shock samples (Fig. 3b), the meltdown profiles were very similar to those of the non-heat-shock samples.
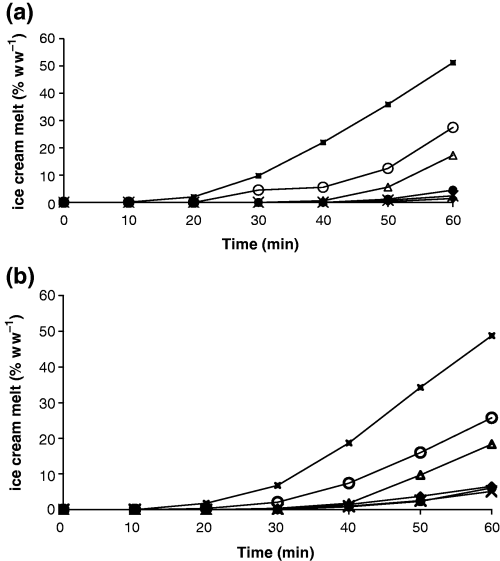
(a) Meltdown characteristics of non-heat-shock ice cream over 60 min at 20 °C. Each data point is an average value obtained from three samples. The six batches of ice cream samples containing different combinations of % milkfat:% flaxseed oil in a 12% w/w fat ice cream are denoted as 12:0 (◆), 12:2 (×), 8:4 (•), 6:6 (△), 3:9 (○) and 0:12 (■). (b) Meltdown characteristics of heat-shock ice cream over 60 min at 20 °C. Each data point is an average value obtained from three samples. The ice cream samples containing different combinations of % milkfat:% flaxseed oil in a 12% w/w fat ice cream are denoted as 12:0 (◆), 12:2 (×), 8:4 (•), 6:6 (◇), 3:9 (○) and 0:12 (■).
The graph in Fig. 4 consolidates the weights of the melted ice cream at 60 min for both heat-shock and non-heat-shock samples. The non-heat-shock ice cream samples show no significant difference (P-value > 0.05) in the meltdown rates when the amount of flaxseed oil was incorporated at ≤4% (w/w) in a 12% (w/w) fat ice cream formulation. However, a significant increase (P-value ≤ 0.05) in the meltdown rate was observed when flaxseed oil was incorporated at 6% (w/w). The meltdown rate continued to increase significantly when the incorporated flaxseed oil was at 9% (w/w). Another significant increase in the meltdown rate was recorded in ice cream containing 12% flaxseed oil (P-value ≤ 0.05). It was also found that the meltdown rates between heat-shock and non-heat-shock samples within each ice cream formulation were not significantly different at 60 min (P-value > 0.05) (Fig. 4). However, the results do not imply that the ice cream structure was unaffected after heat-shock treatment. Obviously, heat-shock could cause ice recrystallisation to occur within the ice cream structure. The effect of heat-shock on the ice cream texture was demonstrated by measuring the ice cream hardness using the texture analyser.
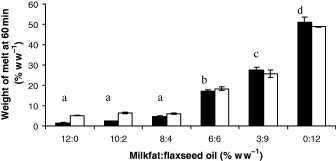
Weight of non-heat-shock (■) and heat-shock (□) melted ice cream samples at 20 °C over a 60 min period. Error bars represent means ± SE, n = 3. Different alphabets indicate significant difference at 95% confidence interval of non-heat-shock ice cream.
Particle size distribution of melted ice cream
The definition of coalescence is given as ‘an irreversible increase in the size of the fat globules and a loss of identity of the coalescing globules’ and partial coalescence as ‘an irreversible agglomeration/clustering of fat globules, held together by a combination of fat crystals and liquid fat, and a retention of identity of individual globules as long as the crystal structure is maintained’ (Goff, 2000). It is widely known that the formation of a good ice cream structure is dependent on the extent of fat destabilisation which in turn stabilise the air cells at the air interface (Goff et al., 1999). However, it has not been clear whether the model for fat destabilisation is one of partial coalescence or flocculation (clustering of fat globules) or both (Goff, 2000).
It has been shown, in this study, that different proportions of flaxseed oil and milkfat did affect the rates of ice cream meltdown (Fig. 4). The differences in the meltdown rates could be explained by the size distribution of the fat globules/oil droplets of the melted ice cream. The average particle size (d43) of fat globules in the melted ice cream samples and the ice cream samples in SDS/EDTA solution are shown in Fig. 5.
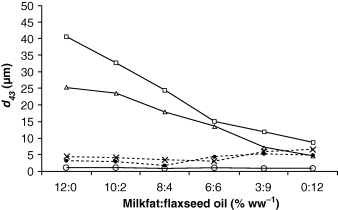
Average volume-weighted diameters (d43) of fat globules/agglomerates in mixes and melted ice cream containing different proportion of milkfat and flaxseed oil in a 12% (w/w) fat ice cream. The samples consist of non-heat-shock melted ice cream with SDS/EDTA (•) and without SDS/EDTA (△) heat-shock melted ice cream with SDS/EDTA (×) and without SDS/EDTA (□); ice cream mix after ageing with SDS/EDTA (○).
The average particle size of the fat globules (d43) obtained from the melted ice cream samples without SDS/EDTA treatment decreased almost linearly as the proportion of flaxseed oil increases (Fig. 5). As for the melted ice cream samples treated with SDS/EDTA solution, the corresponding values of d43 decreased to an approximately constant value for all samples. The results indicated that the larger particle size observed in the ice cream formulation with a higher proportion of milkfat was mainly because of flocculation of the fat globules. On the other hand, a decrease in the average particle size (because of a lower degree of fat agglomeration) was observed in ice cream containing higher proportion of flaxseed oil. If the extent of flocculation is due to the interactions between interfacial layers of the fat/oil globules, it could suggest that the molecular composition at the interfacial layer of milkfat globules was different from that of the flaxseed oil globules.
It was also observed that the d43 of the melted ice cream (with SDS/EDTA treatment) increased only slightly when comparison was made with d43 values of the ice cream mixes (with SDS/EDTA treatment). This slight increase was attributed to coalescence and/or partial coalescence of the fat globules or the oil droplets during the concomitant churning and freezing process.
The results from this study showed that the partial fat destabilisation of milkfat globules is dominated by flocculation rather that coalescence or partial coalescence. This occurrence of fat flocculation appeared to be most prominent in the ice cream formulation containing completely milkfat. The formation of large fat flocculates could be promoted by protein–protein interaction at the interfaces of the fat globules. Possible explanations may be given to the low occurrence of coalescence and/or partial coalescence. One of these includes the thickening effects of the non-adsorbing polysaccharides (which reduce the rate of fat globule collision because of Brownian movement and shear). Another reason may be attributed to the adsorption of proteins and anionic polysaccharides at the fat globule interface, which may then form a protective layer, retarding fat coalescence (Gelin et al., 1994, Dickinson & Galazka, 1991, Dalgleish & Hollocou, 1997).
In the case of ice cream incorporated with flaxseed oil, the degree of flocculation decreased as the proportion of flaxseed oil was increased. In the ice cream formulation containing ≤3% (w/w) milkfat [or ≥9% (w/w) flaxseed oil], the degree of fat flocculation seemed to be negligible. Instead, the increase in the oil droplets size was mainly due to coalescence and/or partial coalescence. This could be explained by the low melting temperature of flaxseed oil, which was likely to remain largely as a liquid oil (rather than a partially crystallised fat) at the ageing temperature of 5 °C. If the oil droplets did remain as a liquid droplet, then they could coalesce especially when the mix was subjected to high shear during the concomitant whipping and freezing process. However, the extent of coalescence observed in ice cream containing a high proportion of flaxseed oil was considered rather limited (compared with the extent of flocculation in the case of milkfat). This could be due to the excellent emulsifying property of the milk proteins and/or emulsifiers. Other factors that may influence either fat flocculation or coalescence may include the chemical composition of the triglycerides, the amount of fat crystals present (Davies et al., 2001) and the type of added surfactants (Bolliger et al., 2000b). It is well-established that the resistance of the ice cream meltdown rate is largely dependent on the degree of fat destabilisation (Bolliger et al., 2000a). Consequently, the low degree of fat flocculation in the ice cream formulation containing high proportions of flaxseed oil could explain why a more rapid melting rate was obtained.
From this study, it was also observed that the particle size of fat/oil increased as a result of heat-shock. Heat-shock ice cream containing a higher proportion of milkfat than flaxseed oil was found to have a slightly higher level of fat flocculation. However, further coalescence of fat globules appeared to be negligible in all cases except for ice cream containing 12% flaxseed oil, which indicated a small increase in the average oil droplet size caused by coalescence.
Microstructure of the melted ice creams
The melted ice cream samples were examined by CLSM. The purpose of this work was to observe the state of fat globules/oil droplets in order to have a visual perspective of the flocculation. The micrographs (Fig. 6) showed that the fat globules of the ice cream sample containing only milkfat (without heat-shock treatment) associated with each other to form large particles, indicating flocculation of the milkfat globules. These flocculated fat particles formed a thick layer on the surface of air bubbles (Fig. 6a). The size of these air bubbles is in the range of c. 20–30 μm. Only a few individual fat globules were observed in the ice cream continuous phase. The structure observed in the present study is in agreement with the previous studies using electron microscopy (Goff, 1997, Kalab, 1985, Boode & Walstra, 1993). The agglomerated fat which formed a thick layer around the air bubbles help strengthen the foam structure (Goff, 2003). In the case of heat-shock samples, the flocculated fat particles appeared to increase in size, but fewer air bubbles were observed in the melted ice cream sample (Fig. 6b).
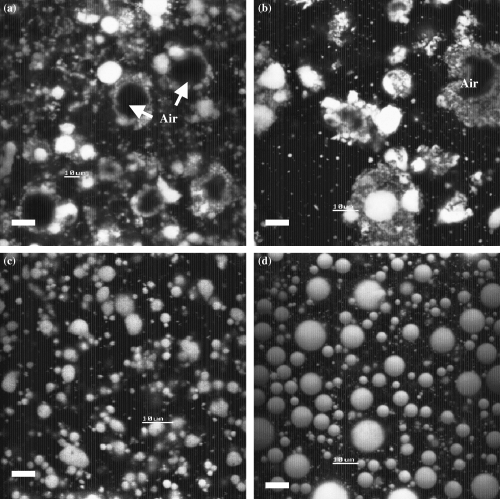
Confocal micrographs of the melted ice cream containing milk fat (a, b) and flaxseed oil (c, d) without heat-shock (a, c) and after heat-shock (b, d). Bars represent 10 μm.
In the non-heat-shock ice cream samples containing only flaxseed oil, some of the oil droplets came together to form clusters of small flocculates (Fig. 6c). It was also observed that some of the oil droplets remained as single droplets. With the heat-shock samples, an increase in the size of some oil droplets was observed. The increase in the oil droplet size appeared to be due to coalescence rather than flocculation (Fig. 6d). In both cases (heat-shock and non-heat-shock), no air bubbles were observed in the melt, which could suggest that the flaxseed oil droplets did not appear to be effective in stabilising the air cells (Fig. 6c and d). The observation of fat globules obtained by CLSM is in good agreement with the results of particle size measurement (Fig. 5).
Viscosity flow curves
The flow behaviour of the melted ice cream was consistent with the extent of fat flocculation which occurred in the different ice cream formulations (Fig. 7). Ice cream containing 12% (w/w) milkfat, which had the highest level of fat flocculation, showed the highest viscosity reading and pseudoplasticity index than the ice cream containing 12% (w/w) flaxseed oil. The difference in the viscosity flow curve characteristics is attributed to the different degree of fat flocculation as well as different extent of fat crystallisation. The viscosity of the melted ice cream was also consistent with the relative viscosity obtained from the ice cream mix and appeared to correlate well with the meltdown properties.
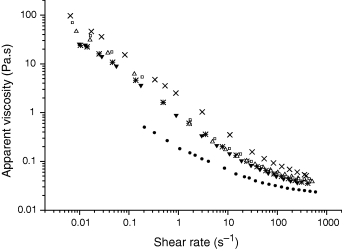
Viscosity flow curves plotted as a function of shear rate obtained from the melted ice cream samples containing different milkfat to flaxseed oil ratios. The six batches of ice cream mixes containing different combinations of % milkfat:% flaxseed oil in a 12% w/w fat ice cream are denoted as 12:0 (×), 12:2 (*), 8:4 (△), 6:6 (□), 3:9 (▼) and 0:12 (•).
Ice cream texture
The hardness of ice cream samples equilibrated at −18 °C was evaluated by performing a penetration test using a texture analyser. The plot of maximum force (N) required to penetrate the ice cream samples is shown in Fig. 8. The different alphabets on the graph indicate significance among the samples (P-value ≤ 0.05). The results indicated that ice cream firmness was significantly reduced by flaxseed oil incorporation. The higher the proportion of flaxseed oil, the lower the firmness. A similar trend was also observed for the heat-shock samples containing different proportion of flaxseed oil. In addition, when comparing the heat-shock and non-heat-shock samples within each ice cream formulation, all the heat-shock samples had a significantly greater degree of firmness than the non-heat-shock samples. This increase in firmness could be explained by the formation of larger ice crystals, which occurred during thawing of the small ice crystals and refreezing of the water molecules. The resultant larger ice crystals formed were likely to contribute to a firmer texture. The melting of the small ice crystals also suggests that the serum phase becomes less viscous, allowing greater mobility of the fat droplets to move about and causing the formation of a slightly larger fat flocculates (as described above).

Peak force (N) required to penetrate the non-heat-shock (■) and heat-shock (□) ice cream samples containing different proportion of milkfat and flaxseed oil, measured using a Texture Analyser. Values represent mean ± SE, n = 3. Different alphabets indicate significant difference at 95% confidence interval of non-heat-shock ice cream.
Conclusions
The incorporation of flaxseed oil in ice cream to replace milkfat could affect the functional characteristics of ice cream, depending on the amount of milkfat being replaced. It is shown, in this study, that the higher the proportion of flaxseed oil used to replace the milkfat, the higher the ice cream meltdown rate and the lower the firmness of the ice cream. The causes of the difference in ice cream functional properties were explained by the different melting temperature of the fat/oil used and the extent of fat flocculation that occurred during the concomitant churning and freezing of the ice cream mix. A greater increase in fat particle size, due to fat flocculation, was observed in ice cream formulation containing a high level of milkfat. The flocculated fat stabilised the air cells which contributed to the structure of ice cream and showed better resistance to meltdown. Ice cream made with a high proportion of flaxseed oil showed minimal fat flocculation, and hence, could not effectively stabilise the air cells resulting in a soft ice cream that has a high meltdown rate. Incorporation of flaxseed oil in a 12% (w/w) ice cream is possible at 2% (w/w) without drastically affecting the ice cream overall functionality. However, sensory evaluation will be needed to determine consumer acceptance in terms of the flavour and the textural attributes it imparts.
Acknowledgments
The authors would like to thank Functional Whole Foods Ltd, New Zealand for the generous supply of flaxseed oil and partial funding of the project.
References
Author notes
†Present address: Sensient Technologies, 5 Doraval Pl Mt Wellington Auckland, New Zealand.
‡Present address: Fonterra Co-operative Group Limited, Private Bag 11 029, Dairy Farm Road, Palmerston North, New Zealand.