-
PDF
- Split View
-
Views
-
Cite
Cite
Véronique Zuliani, Isabelle Lebert, Pascal Garry, Jean-Luc Vendeuvre, Jean-Christophe Augustin, André Lebert, Effects of heat-processing regime, pH, water activity and their interactions on the behaviour of Listeria monocytogenes in ground pork. Modelling the boundary of the growth/no-growth areas as a function of pH, water activity and temperature, International Journal of Food Science and Technology, Volume 41, Issue 10, December 2006, Pages 1197–1206, https://doi.org/10.1111/j.1365-2621.2006.01185.x
- Share Icon Share
Abstract
The influence of four heat-processing regimes and a storage phase on the behaviour of Listeria monocytogenes in ground pork was studied. The effects of pH and water activity (aw) were also tested. During the heat process phase, aw, the heat-processing regime and its interactions with pH or aw, had a significant effect on the behaviour of L. monocytogenes. During the storage phase, all parameters tested and their interactions had significant effects. Nevertheless, the area in which the growth of L. monocytogenes was observed at the end of the experiment was not influenced by the heat-processing regime tested. On the contrary, pH, aw and their interactions had significant effects on Listeria behaviour. The boundary of the growth area delimited by environmental conditions where growth was higher than 1.0 Log CFU g−1 from those where growth was lower than this limit was correctly predicted by Augustin's model.
Introduction
Bacterial food diseases frequently occur in developed countries with significant health and economic consequences. In 1999, 660 clusters were reported in France (Haeghebaert et al., 2002). Most of these diseases involved Salmonella, Staphylococcus aureus or Clostridium perfringens. Other bacteria, such as Listeria monocytogenes, were involved as well (Buisson & Teysou, 2002). This bacteria is problematic because of its ability to grow over a wide range of temperatures, pH and water activity (aw) values (Augustin, 1999), enabling it to survive in many foods. Listeria monocytogenes has often been involved in meat disease outbreaks: in France in 1992, 279 people became ill after consumption of pork tongue in aspic; a year later, potted minced pork was responsible for thirty-nine cases. In 1999 and 2000, potted minced pork and pork tongue in aspic were once again involved in listeriosis outbreaks in France (Folio, 2003). In 2002, a sausage mixture was incriminated in the last French outbreak because of the consumption of delicatessen products (Thévenot et al., 2005).
During the process of delicatessen products, physicochemical properties of the meat are modified: pH is decreasing because of the use of starter culture or addition of organic acid salt in the brine and water activity (aw) is decreasing because of the addition of salts or water evaporation during drying (Durand, 1999). Temperature is also modified; indeed, it first increases during the heat process phase and then decreases during the storage at refrigeration temperature.
In this study, the effects of pH, aw and heat-processing regime on the behaviour of L. monocytogenes inoculated in ground pork were studied during the heat process phase and the storage phase at 20 °C. Our aim was to determine the parameters and their interactions that had an influence on the evolution of contamination during a process composed of formulation, a mild heat treatment and a storage phase. We also studied the boundary between the growth and the no-growth areas, as a function of pH and aw for a given processing regime. An attempt was then made to predict such a boundary.
Materials and methods
The organism used and preparation of inoculum
Listeria monocytogenes 14, serotype 4b, is a fast growing strain (Bégot et al., 1997) isolated from an industrial pork batter. Before being used, it was stored on glass beads at −20 °C.
Stationary phase inoculum was obtained after three subcultures in 10 mL Brain Heath Infusion (Oxoid, Dardilly, France), incubated at 37 °C in a rotary shaker (Novotron, Infors, Massy, France) at 150 r.p.m. This provided a 3–4 h stationary phase inoculum containing about 109 CFU mL−1 (Leporq, 2001).
Media and reagents
NaCl (Normapur, Prolabo, Fontenay-sous-Bois, France) was added to adjust the aw of the meat. HCl (Rectapur, Prolabo), 1 N or NaOH (Normapur, Prolabo) 1 N were used to adjust the pH. NaCl powder, HCl and NaOH solutions were sterilised by autoclaving for 15 min at 121 °C. Palcam Agar (Biokar, Beauvais, France) was used for enumeration of L. monocytogenes and Plate Count Agar (Biokar) for total counts. Tryptone (0.1% w/v, Biokar)–NaCl (0.85% w/v, Prolabo) water was used for all serial dilutions and for dilution of the inoculum. All media and diluents were autoclaved at 121 °C for 15 min.
Experimental design
In order to study the influence of the heat-processing regime and the formulation (aw, pH) on the behaviour of L. monocytogenes, a complete factorial design (four × five × five) was used.
The effects of the heat-processing regime (4 h at 42 °C, referred to as 4 h/42 °C; 1 h at 48 °C: 1 h/48 °C; 1 h at 53 °C: 1 h/53 °C; and a control treatment, 24 h at 20 °C: 24 h/20 °C i.e., at storage temperature), pH (5.0, 5.3, 5.6, 5.9 and 6.2) and aw (0.93, 0.94, 0.95, 0.96 and 0.97) were studied.
The four processing regimes were chosen in order to reproduce as closely as possible the actual practices used by the delicatessen industry. Indeed, heat-processing phase takes place between 50 and 55 °C (during 1–1.5 h) for high-temperature processes or between 30 and 45 °C (during 12–24 h) for low-temperature processes (Frentz & Juillard, 2003). Length of the phase was adapted to the geometry of the meat sample: it was chosen in order to avoid roasting of the meat sample. Indeed, after this phase of the process, in the industry, bacon is still raw. High values of pH and aw tested were close to the average ones found for commercial diced bacon. The low values corresponded to those frequently found for other typical raw meat products (Cole et al., 1990; Pidcock et al., 2002).
Experimental procedure
Mincing and freezing of meat
First, the fat was removed from shank-free pork shoulders. The meat was then minced and meat rectangles (length: 20 cm; width: 15 cm and thickness: 1.3 cm) of about 400 g were vacuum packed and frozen at −20 °C. Fifty-five meat rectangles where obtained from the 22 kg homogeneous meat batter and were used all along the study.
Meat decontamination
In order to work with meat that was equally contaminated and had low initial contamination, it was irradiated using high electron energies (AERIAL, Illkirch, France). Frozen vacuum-packed meat rectangles were irradiated with a dose of 15 kGy. This dose was chosen because it was the minimum dose that allowed the decrease of the bacterial population without forming too many oxidation compounds (Farkas, 1998). The dose rate during irradiation was 2 kGy s−1. The dose absorbed by the samples was checked using a quantimeter FWT 60 00 calibrated with standards of the Henri Becquerel National Laboratory (Gif sur Yvette, France).
Adjusting awand pH
After thawing the meat at 4 °C, the aw was adjusted. NaCl 3.6–8.6% (w/w) was manually mixed with meat. The aw was measured at 20 °C after adjustment of the pH with an electric hygrometer (aw-sprint TH500, Novasina, Roucaire, Nice, France).
After adjusting the aw, meat pH was adjusted: NaOH or HCl solutions were gradually added to the meat and mixed to obtain the desired pH that was measured using a MP230 pH meter (Mettler Toledo, Viroflay, France) and an Inlab 427 penetration probe (Mettler Toledo). These two phases were carried out in a laminar airflow cabinet, NU 425-400 (NuAire, Saint Ouen l'Aumone, France) to prevent the contamination of the meat. They were performed 24 h before inoculation of the meat. Samples were then stored overnight at 4 °C before use.
Meat inoculation
Meat samples were inoculated at a rate of 105 cells g−1 of meat. According to the threshold detection of the technique used, this inoculum size made it possible to observe the growth, inhibition and decrease of L. monocytogenes. The subculture was added to the meat in a closed stomacher bag. The contents of the bag were then mixed by hand in order to obtain a homogeneous concentration of bacteria.
Heat processing
To guarantee the homogeneity of the heat process for all the experiments, a rectangle of meat was formed: its width was the same as that of the 400 mL stomacher bag (InterScience, Saint-Nom-La Bretèche, France), i.e. 16.5 cm with a thickness of 1 cm. The length was determined according to the exact weight of the sample (6.1 cm for 100 g of minced meat). Heat processing took place in a forced air incubator (FC 90, Eurotherm, Dardilly, France). During each heat process, the temperature of the incubator was recorded to control its stability (El-win and Easylog software, Lascar, Wiltshire, England).
Bacterial enumerations
For each conditions included in the experimental design, four meat samples were analysed for L. monocytogenes numbers: at the beginning of the experiment (t0), at the end of the heat process phase (t1), during storage at days 4 (t4) and 6 (t6). Enumeration of total bacterial count was made one time for each experiment just before meat inoculation.
In addition to the kinetics of the experimental design, kinetics with fifteen enumeration times were performed in triplicate at pH 5.6/aw 0.95 for the four heat-processing regimes (including control treatment) and at pH 6.2/aw 0.97 for the control treatment.
The contaminated L. monocytogenes meat was heat processed and finally stored at 20 °C in a refrigerated incubator (KB 240, Binder, Tuttlingen, Germany). This storage temperature, made it possible to reduce the experiment time. The use-by-date of commercial delicatessen products such as diced bacon is generally about 45 days when it is stored at 4 °C. Six days of storage at 20 °C were chosen in our experiments as the first signs of microbiological degradation of commercial diced bacon (expansion of the packaging tray, greenness of meat) were observed under these conditions.
For each enumeration, 20 g of meat were aseptically removed from the packages and placed in stomacher bags with 180 mL of tryptone–salt water. Samples were then stomached in a Model 400 Lab Stomacher (InterScience) for 1 min and serial dilutions were carried out. All platings were made in duplicate using a spiral Plater (InterScience). Plates were incubated (incubator type BE 500, Memmert, Schwabach, Germany) for 24–48 h at 37 °C for Palcam, 72 h at 30 °C for Plate Count Agar, and then enumerated.
Data analysis
The 100 conditions included in the experimental design were divided into three phases:
- 1
between t0 and t1, corresponded to the heat process phase.
- 2
between t1 and t6, corresponded to the storage phase.
- 3
between t0 and t6, corresponded to the global phase.
Study of the growth capacity
We calculated a growth capacity (GC) that characterises the evolution of the population during each phase as described below:
- 1
for the heat process phase: GCt1/t0 = Log (N at t1) – Log (N at t0)
- 2
for the storage phase: GCt6/t1 = Log (N at t6) – Log (N at t1)
- 3
for the global experiment: GCt6/t0 = Log (N at t6) – Log (N at t0)
where N is the L. monocytogenes concentration in CFU per gram of meat.
GC data were used to perform multifactorial analysis of variance (Anova) using Statistica 6.0 software (Statsoft, Maisons-Alfort, France). As all meat samples came from the same batter, we pooled all the data for the statistical analysis.
Modelling
Two models were needed to model bacterial growth
- 1A primary model for describing the evolution of the bacterial concentration as a function of time. The logistic growth model with delay (Rosso et al., 1996) was used. The curves were then characterised by maximal growth rate (μmax), lag time (lag), initial bacterial concentration (N0) and maximal bacterial concentration (Nmax). The differential and integrated forms of the primary model are, respectively, represented by eqns 1, 2 and 3. Using experimental values measured for N0 for each experiment and Nmax of 1.65 × 108 CFU g−1, we fitted the curves in order to optimise μmax and lag values with the method of Rosenbrock, followed by the method of Quasi-Newton (Statistica 6.0).(1)
where t is the time (h), N the bacterial population at time t (CFU g−1), μmax the maximal growth rate, N0 the initial bacterial concentration (CFU g−1), lag the lag time (h) and Nmax the maximal bacterial concentration (CFU g−1).
- 2A secondary model for describing the influence of aw, pH and heat-processing regimes on μmax and lag. The cardinal model with interactions developed by Augustin (Augustin & Carlier, 2000) was used (eqn 4 and 5). Its parameters (optimal growth rate, μopt, and K, which links lag with μmax) were optimised using the method of Newton (Excel, Microsoft, Courtabœuf, France) based on the results of the kinetics established from fifteen enumeration times. Other parameters used were those proposed by Augustin (1999) (Table 1).where μopt is the maximum growth rate (h−1) obtained in standard medium at optimum growth conditions, K a constant, CM the cardinal model function presented in eqn 3(2)(3)
where X is an environmental factor (T for temperature, pH or aw), Xmin, Xopt and Xmax are the minimal, optimal and maximal values for X, n = 2 for CM(T) and CM(aw), n = 1 for CM(pH).
Cardinal values for Listeria monocytogenes, n, form parameter of the cardinal model functions (CMn) (Augustin, 1999)
Tmin = −3.0 °C . | Topt = 37 °C . | Tmax = 45.5 °C . | n = 2 . |
---|---|---|---|
pHmin = 4.38 | pHopt = 7.10 | pHmax = 9.61 | n = 1 |
aw min = 0.913 | aw opt = 0.997 | aw max = 1.000 | n = 2 |
Tmin = −3.0 °C . | Topt = 37 °C . | Tmax = 45.5 °C . | n = 2 . |
---|---|---|---|
pHmin = 4.38 | pHopt = 7.10 | pHmax = 9.61 | n = 1 |
aw min = 0.913 | aw opt = 0.997 | aw max = 1.000 | n = 2 |
Cardinal values for Listeria monocytogenes, n, form parameter of the cardinal model functions (CMn) (Augustin, 1999)
Tmin = −3.0 °C . | Topt = 37 °C . | Tmax = 45.5 °C . | n = 2 . |
---|---|---|---|
pHmin = 4.38 | pHopt = 7.10 | pHmax = 9.61 | n = 1 |
aw min = 0.913 | aw opt = 0.997 | aw max = 1.000 | n = 2 |
Tmin = −3.0 °C . | Topt = 37 °C . | Tmax = 45.5 °C . | n = 2 . |
---|---|---|---|
pHmin = 4.38 | pHopt = 7.10 | pHmax = 9.61 | n = 1 |
aw min = 0.913 | aw opt = 0.997 | aw max = 1.000 | n = 2 |
Results
Experimental variations
Water activity (aw)
Homogeneity of the aw in meat samples was checked in the condition where 8.6% of NaCl were added. We obtained an aw equal to 0.931 ± 0.002 for three aliquots taken from the same sample adjusted to aw 0.93.
pH
After adjustment of the aw, the pH was adjusted. After this step, maximal difference between three measures made in the same meat sample was 0.1 pH unit.
For some samples, pH was also controlled at the end of the experiments, no significant difference was observed between initial and final pH.
Bacterial concentration
Four replicates of the central point of the experimental design (one replicate was part of the experimental design + three kinetics made with fifteen enumerations time), pH 5.6/aw 0.95, were performed for the three heat-processing regimes and the control treatment (Table 2).
Growth capacity (GC in Log CFU g−1) as a function of the phase and the heat processing regime (including control treatment)
. | Control . | Heat-processing regime . | ||
---|---|---|---|---|
24 h/20 °C . | 4 h/42 °C . | 1 h/48 °C . | 1 h/53 °C . | |
GCt1/t0 | ||||
Mean | 0.1 | −0.8 | −0.6 | −0.9 |
SD | 0.01 | 0.14 | 0.12 | 0.10 |
GCt6/t1 | ||||
Mean | 2.8 | 3.5 | 3.3 | 3.3 |
SD | 0.19 | 0.15 | 0.18 | 0.15 |
GCt6/t0 | ||||
Mean | 2.9 | 2.7 | 2.7 | 2.4 |
SD | 0.19 | 0.08 | 0.17 | 0.11 |
. | Control . | Heat-processing regime . | ||
---|---|---|---|---|
24 h/20 °C . | 4 h/42 °C . | 1 h/48 °C . | 1 h/53 °C . | |
GCt1/t0 | ||||
Mean | 0.1 | −0.8 | −0.6 | −0.9 |
SD | 0.01 | 0.14 | 0.12 | 0.10 |
GCt6/t1 | ||||
Mean | 2.8 | 3.5 | 3.3 | 3.3 |
SD | 0.19 | 0.15 | 0.18 | 0.15 |
GCt6/t0 | ||||
Mean | 2.9 | 2.7 | 2.7 | 2.4 |
SD | 0.19 | 0.08 | 0.17 | 0.11 |
Mean and standard deviation (SD) calculated from four replicates. GCt1/t0 = GC during heat process phase, GCt6/t1 = GC during storage phase and GCt6/t0 = GC during global experiment.
Growth capacity (GC in Log CFU g−1) as a function of the phase and the heat processing regime (including control treatment)
. | Control . | Heat-processing regime . | ||
---|---|---|---|---|
24 h/20 °C . | 4 h/42 °C . | 1 h/48 °C . | 1 h/53 °C . | |
GCt1/t0 | ||||
Mean | 0.1 | −0.8 | −0.6 | −0.9 |
SD | 0.01 | 0.14 | 0.12 | 0.10 |
GCt6/t1 | ||||
Mean | 2.8 | 3.5 | 3.3 | 3.3 |
SD | 0.19 | 0.15 | 0.18 | 0.15 |
GCt6/t0 | ||||
Mean | 2.9 | 2.7 | 2.7 | 2.4 |
SD | 0.19 | 0.08 | 0.17 | 0.11 |
. | Control . | Heat-processing regime . | ||
---|---|---|---|---|
24 h/20 °C . | 4 h/42 °C . | 1 h/48 °C . | 1 h/53 °C . | |
GCt1/t0 | ||||
Mean | 0.1 | −0.8 | −0.6 | −0.9 |
SD | 0.01 | 0.14 | 0.12 | 0.10 |
GCt6/t1 | ||||
Mean | 2.8 | 3.5 | 3.3 | 3.3 |
SD | 0.19 | 0.15 | 0.18 | 0.15 |
GCt6/t0 | ||||
Mean | 2.9 | 2.7 | 2.7 | 2.4 |
SD | 0.19 | 0.08 | 0.17 | 0.11 |
Mean and standard deviation (SD) calculated from four replicates. GCt1/t0 = GC during heat process phase, GCt6/t1 = GC during storage phase and GCt6/t0 = GC during global experiment.
Whatever is the heat-processing regime or the phase studied, standard deviations (SDs) were always lower than 0.20. In the same way, regardless of the phase studied, the variation coefficient (absolute value of the ratio of the SD to the mean value) was lower than 0.20 for the three heat-processing regimes and the control treatment. For the GCt6/t1 and GCt6/t0, the variation coefficient was between 0.03 and 0.07.
The heat process phase
Table 3 compares the GCt1/t0 under the 100 conditions tested (three heat-processing regimes and the control treatment × five pH × five aw). We decided that at a GC value lower than −1.0 Log CFU g−1, the combination of pH, aw and the heat-processing regime resulted in a decrease in the number of L. monocytogenes (inactivation). When the GC value was equal to or higher than −1.0 Log CFU g−1 and equal to or lower than 1.0 Log CFU g−1, L. monocytogenes was inhibited. When the GC value was higher than 1.0 Log CFU g−1, the environmental conditions were favourable to L. monocytogenes growth. We thus concluded that during the heat process phase, the L. monocytogenes population survived in 88 of the 100 conditions tested. Of the twelve other conditions, three resulted in L. monocytogenes growth and occurred only in the control treatment 24 h/20 °C: aw 0.97/pH 6.2, aw 0.97/pH 5.9 and aw 0.96/pH 6.2, that is, for conditions that combined high pH and high aw. These results are indicative of the considerable influence of pH and aw on the GCt1/t0.
Growth capacity for Listeria in ground pork during heat process phase (GCt1/t0) as a function of aw, pH and heat-processing regime compared with control treatment, with N0 = 105 CFU g−1
aw . | pH . | Control . | Heat-processing regime . | ||
---|---|---|---|---|---|
24 h/20 °C . | 4 h/42 °C . | 1 h/48 °C . | 1 h/53 °C . | ||
0.97 | 5.0 | 0.1 | −0.1 | −0.1 | −0.6 |
5.3 | 0.3 | −0.3 | −0.5 | −1.1 | |
5.6 | 0.5 | −0.4 | −0.8 | −0.9 | |
5.9 | 1.8 | −0.6 | −0.5 | −1.3 | |
6.2 | 2.6 | 0.0 | −0.7 | −1.3 | |
0.96 | 5.1 | −0.1 | −0.2 | −0.4 | −0.3 |
5.3 | −0.1 | −0.4 | −0.4 | −0.5 | |
5.6 | 0.9 | −0.2 | −0.7 | −0.8 | |
5.9 | 0.9 | −0.2 | −0.3 | −1.1 | |
6.2 | 1.7 | −0.1 | −0.6 | −0.9 | |
0.95 | 5.0 | 0.0 | −0.1 | −0.3 | 0.1 |
5.3 | −0.2 | −0.5 | −0.1 | −0.5 | |
5.6 | 0.1 | −0.9 | −0.7 | −1.0 | |
5.9 | 0.5 | −0.8 | −0.7 | −1.2 | |
6.2 | 0.1 | −1.1 | −0.4 | −1.7 | |
0.94 | 5.0 | −0.4 | −0.2 | −0.2 | −0.1 |
5.3 | −0.2 | −0.8 | −0.1 | −0.5 | |
5.6 | −0.1 | −0.3 | −0.5 | −0.7 | |
5.9 | −0.2 | −0.8 | −0.5 | −0.7 | |
6.2 | −0.3 | −1.9 | −0.7 | −0.3 | |
0.93 | 5.0 | −0.2 | −0.3 | −0.2 | −0.2 |
5.3 | −0.1 | −0.5 | −0.3 | −0.4 | |
5.6 | −0.1 | −0.6 | −0.3 | −0.2 | |
5.9 | −0.6 | −0.9 | −0.1 | −0.6 | |
6.2 | −0.3 | −0.7 | −0.3 | −1.7 |
aw . | pH . | Control . | Heat-processing regime . | ||
---|---|---|---|---|---|
24 h/20 °C . | 4 h/42 °C . | 1 h/48 °C . | 1 h/53 °C . | ||
0.97 | 5.0 | 0.1 | −0.1 | −0.1 | −0.6 |
5.3 | 0.3 | −0.3 | −0.5 | −1.1 | |
5.6 | 0.5 | −0.4 | −0.8 | −0.9 | |
5.9 | 1.8 | −0.6 | −0.5 | −1.3 | |
6.2 | 2.6 | 0.0 | −0.7 | −1.3 | |
0.96 | 5.1 | −0.1 | −0.2 | −0.4 | −0.3 |
5.3 | −0.1 | −0.4 | −0.4 | −0.5 | |
5.6 | 0.9 | −0.2 | −0.7 | −0.8 | |
5.9 | 0.9 | −0.2 | −0.3 | −1.1 | |
6.2 | 1.7 | −0.1 | −0.6 | −0.9 | |
0.95 | 5.0 | 0.0 | −0.1 | −0.3 | 0.1 |
5.3 | −0.2 | −0.5 | −0.1 | −0.5 | |
5.6 | 0.1 | −0.9 | −0.7 | −1.0 | |
5.9 | 0.5 | −0.8 | −0.7 | −1.2 | |
6.2 | 0.1 | −1.1 | −0.4 | −1.7 | |
0.94 | 5.0 | −0.4 | −0.2 | −0.2 | −0.1 |
5.3 | −0.2 | −0.8 | −0.1 | −0.5 | |
5.6 | −0.1 | −0.3 | −0.5 | −0.7 | |
5.9 | −0.2 | −0.8 | −0.5 | −0.7 | |
6.2 | −0.3 | −1.9 | −0.7 | −0.3 | |
0.93 | 5.0 | −0.2 | −0.3 | −0.2 | −0.2 |
5.3 | −0.1 | −0.5 | −0.3 | −0.4 | |
5.6 | −0.1 | −0.6 | −0.3 | −0.2 | |
5.9 | −0.6 | −0.9 | −0.1 | −0.6 | |
6.2 | −0.3 | −0.7 | −0.3 | −1.7 |
boldface: GCt1/t0 > 1.0 Log CFU g−1; italics: GCt1/t0 < −1.0 Log CFU g−1; other: −1.0 Log CFU g−1 ≤ GCt1/t0 ≤ 1.0 Log CFU g−1.
Growth capacity for Listeria in ground pork during heat process phase (GCt1/t0) as a function of aw, pH and heat-processing regime compared with control treatment, with N0 = 105 CFU g−1
aw . | pH . | Control . | Heat-processing regime . | ||
---|---|---|---|---|---|
24 h/20 °C . | 4 h/42 °C . | 1 h/48 °C . | 1 h/53 °C . | ||
0.97 | 5.0 | 0.1 | −0.1 | −0.1 | −0.6 |
5.3 | 0.3 | −0.3 | −0.5 | −1.1 | |
5.6 | 0.5 | −0.4 | −0.8 | −0.9 | |
5.9 | 1.8 | −0.6 | −0.5 | −1.3 | |
6.2 | 2.6 | 0.0 | −0.7 | −1.3 | |
0.96 | 5.1 | −0.1 | −0.2 | −0.4 | −0.3 |
5.3 | −0.1 | −0.4 | −0.4 | −0.5 | |
5.6 | 0.9 | −0.2 | −0.7 | −0.8 | |
5.9 | 0.9 | −0.2 | −0.3 | −1.1 | |
6.2 | 1.7 | −0.1 | −0.6 | −0.9 | |
0.95 | 5.0 | 0.0 | −0.1 | −0.3 | 0.1 |
5.3 | −0.2 | −0.5 | −0.1 | −0.5 | |
5.6 | 0.1 | −0.9 | −0.7 | −1.0 | |
5.9 | 0.5 | −0.8 | −0.7 | −1.2 | |
6.2 | 0.1 | −1.1 | −0.4 | −1.7 | |
0.94 | 5.0 | −0.4 | −0.2 | −0.2 | −0.1 |
5.3 | −0.2 | −0.8 | −0.1 | −0.5 | |
5.6 | −0.1 | −0.3 | −0.5 | −0.7 | |
5.9 | −0.2 | −0.8 | −0.5 | −0.7 | |
6.2 | −0.3 | −1.9 | −0.7 | −0.3 | |
0.93 | 5.0 | −0.2 | −0.3 | −0.2 | −0.2 |
5.3 | −0.1 | −0.5 | −0.3 | −0.4 | |
5.6 | −0.1 | −0.6 | −0.3 | −0.2 | |
5.9 | −0.6 | −0.9 | −0.1 | −0.6 | |
6.2 | −0.3 | −0.7 | −0.3 | −1.7 |
aw . | pH . | Control . | Heat-processing regime . | ||
---|---|---|---|---|---|
24 h/20 °C . | 4 h/42 °C . | 1 h/48 °C . | 1 h/53 °C . | ||
0.97 | 5.0 | 0.1 | −0.1 | −0.1 | −0.6 |
5.3 | 0.3 | −0.3 | −0.5 | −1.1 | |
5.6 | 0.5 | −0.4 | −0.8 | −0.9 | |
5.9 | 1.8 | −0.6 | −0.5 | −1.3 | |
6.2 | 2.6 | 0.0 | −0.7 | −1.3 | |
0.96 | 5.1 | −0.1 | −0.2 | −0.4 | −0.3 |
5.3 | −0.1 | −0.4 | −0.4 | −0.5 | |
5.6 | 0.9 | −0.2 | −0.7 | −0.8 | |
5.9 | 0.9 | −0.2 | −0.3 | −1.1 | |
6.2 | 1.7 | −0.1 | −0.6 | −0.9 | |
0.95 | 5.0 | 0.0 | −0.1 | −0.3 | 0.1 |
5.3 | −0.2 | −0.5 | −0.1 | −0.5 | |
5.6 | 0.1 | −0.9 | −0.7 | −1.0 | |
5.9 | 0.5 | −0.8 | −0.7 | −1.2 | |
6.2 | 0.1 | −1.1 | −0.4 | −1.7 | |
0.94 | 5.0 | −0.4 | −0.2 | −0.2 | −0.1 |
5.3 | −0.2 | −0.8 | −0.1 | −0.5 | |
5.6 | −0.1 | −0.3 | −0.5 | −0.7 | |
5.9 | −0.2 | −0.8 | −0.5 | −0.7 | |
6.2 | −0.3 | −1.9 | −0.7 | −0.3 | |
0.93 | 5.0 | −0.2 | −0.3 | −0.2 | −0.2 |
5.3 | −0.1 | −0.5 | −0.3 | −0.4 | |
5.6 | −0.1 | −0.6 | −0.3 | −0.2 | |
5.9 | −0.6 | −0.9 | −0.1 | −0.6 | |
6.2 | −0.3 | −0.7 | −0.3 | −1.7 |
boldface: GCt1/t0 > 1.0 Log CFU g−1; italics: GCt1/t0 < −1.0 Log CFU g−1; other: −1.0 Log CFU g−1 ≤ GCt1/t0 ≤ 1.0 Log CFU g−1.
The most effective heat-processing regime to reduce the L. monocytogenes population during the heat process phase was the 1 h/53 °C treatment. Indeed, of the nine conditions where the L. monocytogenes population decreased, seven took place during the 1 h/53 °C treatment.
In order to statistically determine whether the heat-processing regime, the aw and the pH influenced the GCt1/t0, an Anova was carried out (Table 4). Results showed that aw had a significant effect on the behaviour of L. monocytogenes during the heat process phase. Three others factors had a highly significant effect: heat-processing regime, interactions between heat-processing regime and pH (heat-processing regime × pH) and interactions between heat-processing regime and aw (heat-processing regime × aw). These results emphasised that the use of drastic time/temperature conditions during this process is an effective method for reducing the L. monocytogenes population.
Anova, effect of heat-processing regimes (including control treatment), pH, aw and their interactions on the GCt1/t0
Parameter . | SS . | df . | MS . | F-value . | P . |
---|---|---|---|---|---|
Intercept | 12.35 | 1 | 12.35 | 95.71 | 0.000 |
Heat-processing regime | 14.79 | 3 | 4.93 | 38.20 | 0.000 |
aw | 1.54 | 4 | 0.38 | 2.98 | 0.028 |
pH | 0.94 | 4 | 0.24 | 1.82 | 0.140 |
Heat-processing regime × aw | 7.57 | 12 | 0.63 | 4.89 | 0.000 |
Heat-processing regime × pH | 5.64 | 12 | 0.47 | 3.64 | 0.000 |
aw × pH | 3.38 | 16 | 0.21 | 1.64 | 0.094 |
Error | 6.19 | 48 | 0.13 |
Parameter . | SS . | df . | MS . | F-value . | P . |
---|---|---|---|---|---|
Intercept | 12.35 | 1 | 12.35 | 95.71 | 0.000 |
Heat-processing regime | 14.79 | 3 | 4.93 | 38.20 | 0.000 |
aw | 1.54 | 4 | 0.38 | 2.98 | 0.028 |
pH | 0.94 | 4 | 0.24 | 1.82 | 0.140 |
Heat-processing regime × aw | 7.57 | 12 | 0.63 | 4.89 | 0.000 |
Heat-processing regime × pH | 5.64 | 12 | 0.47 | 3.64 | 0.000 |
aw × pH | 3.38 | 16 | 0.21 | 1.64 | 0.094 |
Error | 6.19 | 48 | 0.13 |
No significant effect: P > 0.05; significant effect: 0.01 < P ≤ 0.05; very significant effect: 0.001 < P ≤ 0.01; highly significant effect: P ≤ 0.001.
SS = sums of the squares, df = degree of freedom, MS = mean squares, F = Fisher and P = probability.
Anova, effect of heat-processing regimes (including control treatment), pH, aw and their interactions on the GCt1/t0
Parameter . | SS . | df . | MS . | F-value . | P . |
---|---|---|---|---|---|
Intercept | 12.35 | 1 | 12.35 | 95.71 | 0.000 |
Heat-processing regime | 14.79 | 3 | 4.93 | 38.20 | 0.000 |
aw | 1.54 | 4 | 0.38 | 2.98 | 0.028 |
pH | 0.94 | 4 | 0.24 | 1.82 | 0.140 |
Heat-processing regime × aw | 7.57 | 12 | 0.63 | 4.89 | 0.000 |
Heat-processing regime × pH | 5.64 | 12 | 0.47 | 3.64 | 0.000 |
aw × pH | 3.38 | 16 | 0.21 | 1.64 | 0.094 |
Error | 6.19 | 48 | 0.13 |
Parameter . | SS . | df . | MS . | F-value . | P . |
---|---|---|---|---|---|
Intercept | 12.35 | 1 | 12.35 | 95.71 | 0.000 |
Heat-processing regime | 14.79 | 3 | 4.93 | 38.20 | 0.000 |
aw | 1.54 | 4 | 0.38 | 2.98 | 0.028 |
pH | 0.94 | 4 | 0.24 | 1.82 | 0.140 |
Heat-processing regime × aw | 7.57 | 12 | 0.63 | 4.89 | 0.000 |
Heat-processing regime × pH | 5.64 | 12 | 0.47 | 3.64 | 0.000 |
aw × pH | 3.38 | 16 | 0.21 | 1.64 | 0.094 |
Error | 6.19 | 48 | 0.13 |
No significant effect: P > 0.05; significant effect: 0.01 < P ≤ 0.05; very significant effect: 0.001 < P ≤ 0.01; highly significant effect: P ≤ 0.001.
SS = sums of the squares, df = degree of freedom, MS = mean squares, F = Fisher and P = probability.
The storage phase
Following the heat process, meat samples were stored at 20 °C for 6 days. For the 100 conditions tested, the GCt6/t1 were calculated. Data are not shown but result from the subtraction of GCt1/t0 to GCt6/t0 (Tables 3 and 5). Growth was observed for seventy-one conditions. Inhibition of the L. monocytogenes population was observed for twenty-nine conditions and no inactivation was observed. Moreover, contrary to the heat process phase, the influence of pH and aw on the GCt6/t1 was very pronounced for the four treatments (three heat processes and the control).
Growth capacity of Listeria at 20 °C in ground pork during the global experiment (GCt6/t0) as a function of aw, pH and heat-processing regime compared with the control treatment with N0 = 105 CFU g−1
aw . | pH . | Control . | Heat-processing regime . | ||
---|---|---|---|---|---|
24 h/20 °C . | 4 h/42 °C . | 1 h/48 °C . | 1 h/53 °C . | ||
0.97 | 5.0 | 2.5 | 3.0 | 3.1 | 2.7 |
5.3 | 3.3 | 3.0 | 3.5 | 3.0 | |
5.6 | 3.2 | 3.1 | 3.3 | 3.1 | |
5.9 | 3.3 | 3.1 | 3.4 | 3.1 | |
6.2 | 3.4 | 3.1 | 3.5 | 3.0 | |
0.96 | 5.1 | 1.3 | 1.8 | 0.3 | 1.2 |
5.3 | 2.2 | 2.8 | 2.5 | 3.2 | |
5.6 | 2.8 | 2.8 | 2.9 | 3.2 | |
5.9 | 2.9 | 2.6 | 2.9 | 2.9 | |
6.2 | 2.9 | 2.5 | 3.0 | 3.1 | |
0.95 | 5.0 | 0.1 | 0.3 | 0.4 | −0.9 |
5.3 | 1.5 | 2.7 | 2.8 | 2.7 | |
5.6 | 2.7 | 2.8 | 2.8 | 2.5 | |
5.9 | 3.0 | 2.9 | 2.9 | 2.4 | |
6.2 | 2.8 | 3.0 | 3.0 | 2.4 | |
0.94 | 5.0 | −0.2 | −0.2 | −0.3 | −0.4 |
5.3 | −0.4 | 0.2 | −0.2 | −0.1 | |
5.6 | 0.9 | 1.8 | 1.7 | 1.9 | |
5.9 | 2.2 | 2.4 | 2.8 | 2.9 | |
6.2 | 2.4 | 2.4 | 2.8 | 2.9 | |
0.93 | 5.0 | −0.3 | −0.5 | −0.5 | −0.2 |
5.3 | −0.3 | −0.7 | −0.4 | −0.4 | |
5.6 | −0.6 | −0.6 | −0.3 | −0.5 | |
5.9 | −0.6 | 0.7 | 0.0 | 0.5 | |
6.2 | 0.4 | 1.2 | 2.0 | 2.2 |
aw . | pH . | Control . | Heat-processing regime . | ||
---|---|---|---|---|---|
24 h/20 °C . | 4 h/42 °C . | 1 h/48 °C . | 1 h/53 °C . | ||
0.97 | 5.0 | 2.5 | 3.0 | 3.1 | 2.7 |
5.3 | 3.3 | 3.0 | 3.5 | 3.0 | |
5.6 | 3.2 | 3.1 | 3.3 | 3.1 | |
5.9 | 3.3 | 3.1 | 3.4 | 3.1 | |
6.2 | 3.4 | 3.1 | 3.5 | 3.0 | |
0.96 | 5.1 | 1.3 | 1.8 | 0.3 | 1.2 |
5.3 | 2.2 | 2.8 | 2.5 | 3.2 | |
5.6 | 2.8 | 2.8 | 2.9 | 3.2 | |
5.9 | 2.9 | 2.6 | 2.9 | 2.9 | |
6.2 | 2.9 | 2.5 | 3.0 | 3.1 | |
0.95 | 5.0 | 0.1 | 0.3 | 0.4 | −0.9 |
5.3 | 1.5 | 2.7 | 2.8 | 2.7 | |
5.6 | 2.7 | 2.8 | 2.8 | 2.5 | |
5.9 | 3.0 | 2.9 | 2.9 | 2.4 | |
6.2 | 2.8 | 3.0 | 3.0 | 2.4 | |
0.94 | 5.0 | −0.2 | −0.2 | −0.3 | −0.4 |
5.3 | −0.4 | 0.2 | −0.2 | −0.1 | |
5.6 | 0.9 | 1.8 | 1.7 | 1.9 | |
5.9 | 2.2 | 2.4 | 2.8 | 2.9 | |
6.2 | 2.4 | 2.4 | 2.8 | 2.9 | |
0.93 | 5.0 | −0.3 | −0.5 | −0.5 | −0.2 |
5.3 | −0.3 | −0.7 | −0.4 | −0.4 | |
5.6 | −0.6 | −0.6 | −0.3 | −0.5 | |
5.9 | −0.6 | 0.7 | 0.0 | 0.5 | |
6.2 | 0.4 | 1.2 | 2.0 | 2.2 |
boldface: GCt1/t0 > 1.0 Log CFU g−1; other: −1.0 Log CFU g−1 ≤ GCt6/t0≤ 1.0 Log CFU g−1.
Growth capacity of Listeria at 20 °C in ground pork during the global experiment (GCt6/t0) as a function of aw, pH and heat-processing regime compared with the control treatment with N0 = 105 CFU g−1
aw . | pH . | Control . | Heat-processing regime . | ||
---|---|---|---|---|---|
24 h/20 °C . | 4 h/42 °C . | 1 h/48 °C . | 1 h/53 °C . | ||
0.97 | 5.0 | 2.5 | 3.0 | 3.1 | 2.7 |
5.3 | 3.3 | 3.0 | 3.5 | 3.0 | |
5.6 | 3.2 | 3.1 | 3.3 | 3.1 | |
5.9 | 3.3 | 3.1 | 3.4 | 3.1 | |
6.2 | 3.4 | 3.1 | 3.5 | 3.0 | |
0.96 | 5.1 | 1.3 | 1.8 | 0.3 | 1.2 |
5.3 | 2.2 | 2.8 | 2.5 | 3.2 | |
5.6 | 2.8 | 2.8 | 2.9 | 3.2 | |
5.9 | 2.9 | 2.6 | 2.9 | 2.9 | |
6.2 | 2.9 | 2.5 | 3.0 | 3.1 | |
0.95 | 5.0 | 0.1 | 0.3 | 0.4 | −0.9 |
5.3 | 1.5 | 2.7 | 2.8 | 2.7 | |
5.6 | 2.7 | 2.8 | 2.8 | 2.5 | |
5.9 | 3.0 | 2.9 | 2.9 | 2.4 | |
6.2 | 2.8 | 3.0 | 3.0 | 2.4 | |
0.94 | 5.0 | −0.2 | −0.2 | −0.3 | −0.4 |
5.3 | −0.4 | 0.2 | −0.2 | −0.1 | |
5.6 | 0.9 | 1.8 | 1.7 | 1.9 | |
5.9 | 2.2 | 2.4 | 2.8 | 2.9 | |
6.2 | 2.4 | 2.4 | 2.8 | 2.9 | |
0.93 | 5.0 | −0.3 | −0.5 | −0.5 | −0.2 |
5.3 | −0.3 | −0.7 | −0.4 | −0.4 | |
5.6 | −0.6 | −0.6 | −0.3 | −0.5 | |
5.9 | −0.6 | 0.7 | 0.0 | 0.5 | |
6.2 | 0.4 | 1.2 | 2.0 | 2.2 |
aw . | pH . | Control . | Heat-processing regime . | ||
---|---|---|---|---|---|
24 h/20 °C . | 4 h/42 °C . | 1 h/48 °C . | 1 h/53 °C . | ||
0.97 | 5.0 | 2.5 | 3.0 | 3.1 | 2.7 |
5.3 | 3.3 | 3.0 | 3.5 | 3.0 | |
5.6 | 3.2 | 3.1 | 3.3 | 3.1 | |
5.9 | 3.3 | 3.1 | 3.4 | 3.1 | |
6.2 | 3.4 | 3.1 | 3.5 | 3.0 | |
0.96 | 5.1 | 1.3 | 1.8 | 0.3 | 1.2 |
5.3 | 2.2 | 2.8 | 2.5 | 3.2 | |
5.6 | 2.8 | 2.8 | 2.9 | 3.2 | |
5.9 | 2.9 | 2.6 | 2.9 | 2.9 | |
6.2 | 2.9 | 2.5 | 3.0 | 3.1 | |
0.95 | 5.0 | 0.1 | 0.3 | 0.4 | −0.9 |
5.3 | 1.5 | 2.7 | 2.8 | 2.7 | |
5.6 | 2.7 | 2.8 | 2.8 | 2.5 | |
5.9 | 3.0 | 2.9 | 2.9 | 2.4 | |
6.2 | 2.8 | 3.0 | 3.0 | 2.4 | |
0.94 | 5.0 | −0.2 | −0.2 | −0.3 | −0.4 |
5.3 | −0.4 | 0.2 | −0.2 | −0.1 | |
5.6 | 0.9 | 1.8 | 1.7 | 1.9 | |
5.9 | 2.2 | 2.4 | 2.8 | 2.9 | |
6.2 | 2.4 | 2.4 | 2.8 | 2.9 | |
0.93 | 5.0 | −0.3 | −0.5 | −0.5 | −0.2 |
5.3 | −0.3 | −0.7 | −0.4 | −0.4 | |
5.6 | −0.6 | −0.6 | −0.3 | −0.5 | |
5.9 | −0.6 | 0.7 | 0.0 | 0.5 | |
6.2 | 0.4 | 1.2 | 2.0 | 2.2 |
boldface: GCt1/t0 > 1.0 Log CFU g−1; other: −1.0 Log CFU g−1 ≤ GCt6/t0≤ 1.0 Log CFU g−1.
Most of the conditions where the L. monocytogenes population remained constant were those for which the pH was lower than 5.9 and the aw lower than 0.95. The contamination level in meat can thus be reduced during storage when low pH and low aw act as important hurdles (Leistner, 1999). On the contrary, the increase of those parameters resulted in an increase of the L. monocytogenes population, except for 24 h/20 °C. Indeed, after the control treatment, growth actually only occurred in sixteen out of the twenty-five conditions. For some conditions of the other nine combinations (when pH and aw were high), growth had already begun during the control treatment phase (Table 3, GCt1/t0). Six aw/pH formulations did not result in L. monocytogenes growth after 1 h/53 °C, eight after 1 h/48 °C and six after 4 h/42 °C. Anova (Table 6) carried out on the GCt6/t1 confirms our observations. Indeed, pH, aw, heat-processing regime (including control treatment) and heat-processing regime × pH and aw × pH, as well, have a highly significant effect on the growth of L. monocytogenes during the storage phase. Heat-processing regime × aw is also significant.
Anova, effect of heat-processing regimes (including control treatment), pH, aw and their interactions on the GCt6/t0
Parameter . | SS . | df . | MS . | F-value . | P . |
---|---|---|---|---|---|
Intercept | 491.95 | 1 | 491.95 | 2487.61 | 0.000 |
Heat-processing regime | 20.15 | 3 | 6.72 | 33.96 | 0.000 |
aw | 101.71 | 4 | 25.43 | 128.58 | 0.000 |
pH | 50.00 | 4 | 11.75 | 59.40 | 0.000 |
Heat-processing regime × aw | 5.10 | 12 | 0.42 | 2.15 | 0.031 |
Heat-processing regime × pH | 10.60 | 12 | 0.88 | 4.47 | 0.000 |
aw × pH | 35.62 | 16 | 2.23 | 11.26 | 0.000 |
Error | 9.49 | 48 | 0.20 |
Parameter . | SS . | df . | MS . | F-value . | P . |
---|---|---|---|---|---|
Intercept | 491.95 | 1 | 491.95 | 2487.61 | 0.000 |
Heat-processing regime | 20.15 | 3 | 6.72 | 33.96 | 0.000 |
aw | 101.71 | 4 | 25.43 | 128.58 | 0.000 |
pH | 50.00 | 4 | 11.75 | 59.40 | 0.000 |
Heat-processing regime × aw | 5.10 | 12 | 0.42 | 2.15 | 0.031 |
Heat-processing regime × pH | 10.60 | 12 | 0.88 | 4.47 | 0.000 |
aw × pH | 35.62 | 16 | 2.23 | 11.26 | 0.000 |
Error | 9.49 | 48 | 0.20 |
No significant effect: P > 0.05; significant effect: 0.01 < P ≤ 0.05; very significant effect: 0.001 < P ≤ 0.01; highly significant effect: P ≤ 0.001.
SS = sums of the squares, df = degree of freedom, MS = mean squares, F = Fisher and P = probability.
Anova, effect of heat-processing regimes (including control treatment), pH, aw and their interactions on the GCt6/t0
Parameter . | SS . | df . | MS . | F-value . | P . |
---|---|---|---|---|---|
Intercept | 491.95 | 1 | 491.95 | 2487.61 | 0.000 |
Heat-processing regime | 20.15 | 3 | 6.72 | 33.96 | 0.000 |
aw | 101.71 | 4 | 25.43 | 128.58 | 0.000 |
pH | 50.00 | 4 | 11.75 | 59.40 | 0.000 |
Heat-processing regime × aw | 5.10 | 12 | 0.42 | 2.15 | 0.031 |
Heat-processing regime × pH | 10.60 | 12 | 0.88 | 4.47 | 0.000 |
aw × pH | 35.62 | 16 | 2.23 | 11.26 | 0.000 |
Error | 9.49 | 48 | 0.20 |
Parameter . | SS . | df . | MS . | F-value . | P . |
---|---|---|---|---|---|
Intercept | 491.95 | 1 | 491.95 | 2487.61 | 0.000 |
Heat-processing regime | 20.15 | 3 | 6.72 | 33.96 | 0.000 |
aw | 101.71 | 4 | 25.43 | 128.58 | 0.000 |
pH | 50.00 | 4 | 11.75 | 59.40 | 0.000 |
Heat-processing regime × aw | 5.10 | 12 | 0.42 | 2.15 | 0.031 |
Heat-processing regime × pH | 10.60 | 12 | 0.88 | 4.47 | 0.000 |
aw × pH | 35.62 | 16 | 2.23 | 11.26 | 0.000 |
Error | 9.49 | 48 | 0.20 |
No significant effect: P > 0.05; significant effect: 0.01 < P ≤ 0.05; very significant effect: 0.001 < P ≤ 0.01; highly significant effect: P ≤ 0.001.
SS = sums of the squares, df = degree of freedom, MS = mean squares, F = Fisher and P = probability.
The global experiment
Study of the bacterial kinetics demonstrates the effect of pH and aw (Fig. 1). For pH 5.0 and heat-processing regime 4 h/42 °C (Fig. 1a), the bacteria survived during the heat process phase. Then, during storage, the growth of L. monocytogenes was only observed at aw 0.97 and 0.96. The stationary phase was not reached for some of the conditions after 6 days at 20 °C.
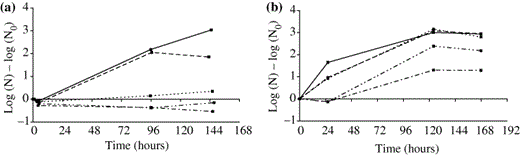
Kinetics of Listeria monocytogenes in ground pork (N0 = 105 CFU g−1) during the global experiment (heat process or control treatment and then storage phase at 20 °C). (a) pH 5.0, heat-processing regime 4 h/42 °C: —— 0.97, ––– 0.96, - - - - - - 0.95, –··–·· 0.94, –·–·– 0.93 and ■ experimental data. (b) aw 0.96, control treatment 24 h/20 °C: —— 6.2, ––– 5.9, - - - - - - 5.6, –··–·· 5.3, –·–·– 5.0 and ■ experimental data.
At aw 0.96 and for the treatment 24 h/20 °C (Fig. 1b), an increase of the initial contamination level was always observed, regardless of the pH. Moreover, as previously observed, pH had a significant influence on the GCt6/t0 (it was equal to about 3.0 Log CFU g−1 at pH 6.2, 5.9 and 5.6, 2.2 for pH 5.3 and only about 1.3 at pH 5.0). Figure 1b also shows that when pH decreased, even if not calculated, the lag time was longer and the maximal growth rate was lower.
Table 5 shows that for the global experiment, sixty-nine conditions of heat process regimes (including control treatment) and formulations (aw × pH) studied resulted in an increase of the initial L. monocytogenes contamination level. Moreover, no decrease was observed after 6 days of storage at 20 °C. For pH under 5.3 and aw under 0.94, no growth occurred, regardless of the heat process regime. On the contrary, growth always occurred when pH was higher than 5.6 and aw was higher than 0.95. There is an intermediate zone between these two areas where both growth and inhibition were observed, depending on the pH/aw combination. This observation attests to interactions between these two parameters. The heat-processing regime did not seem to have an influence on the GCt6/t0. Indeed, the GCt6/t0 was equivalent for the four processing regimes tested (Fig. 2). Even if a decrease took place during the heat process phase when ground pork was heated, the GC during the storage was greater after a heat process than for the control treatment.
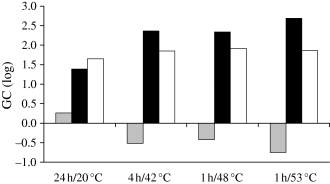
Means of the growth capacity (GC) for the twenty-five conditions of pH/aw as a function of heat-processing regime (including control treatment). GCt1t/0 (heat process phase); ■ GCt6/t1 (storage phase) and □ GCt6/t0 (global experiment).
Modelling
The six kinetics with fifteen sampling times performed in ground pork under constant temperature (control treatment + storage at 20 °C) were fitted to estimate μmax and lag. K (which links μmax and lag) was calculated and was equal to the mean value of 1.944 ± 0.518. Using the Augustin's secondary model, μopt was optimised by non-linear regression. We estimated it to be 1.9 h−1. Other parameters were equal to those proposed by Augustin for the model, taking into account the interactions (Table 1). The primary and the secondary microbial models were then used for predicting bacterial concentrations and thus for predicting the boundary between the growth (GCt6/t0 >1.0 Log CFU g−1) and the no-growth area (GCt6/t0 <1.0 Log CFU g−1) at the end of the storage time. This boundary was defined as the conditions of pH, aw and processing regime that result in a GCt6/t0 equal to 1.0 Log CFU g−1 (i.e. an increase of 1.0 Log CFU g−1 in 168 h). As the GCt6/t0 calculated from the three processing regimes are similar to those from the control treatment (Fig. 2), we only modelled the boundary for the control treatment, that is, in static temperature conditions at 20 °C. For each pH, we determined the aw for which GCt6/t0 was equal to 1.0 Log CFU g−1 at 20 °C. The function target value (Excel, Microsoft) was used. It was repeated for each pH between 4.9 and 6.3 (increment of 0.05). We thus obtained couples of aw/pH for which GCt6/t0 was equal to 1.0 Log CFU g−1. They were used to establish the line representing the boundary between the growth and the no-growth areas (Fig. 3). Comparing experimental data with the prediction shows that the boundary of the growth area was accurately predicted. Indeed, for all the twenty-five conditions tested, the behaviour of Listeria was correctly predicted.
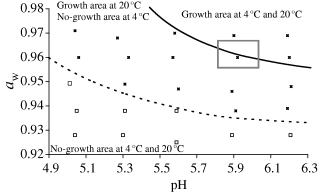
Comparison of GCt6/t0 with predicted boundary (GC = 1.0 Log CFU g−1) of the growth/no-growth areas at 20 °C in ground pork for Listeria monocytogenes as a function of aw and pH. ■ growth (GCt6/t0 > 1.0 Log CFU g−1) and □ no growth (GCt6/t0 < 1.0 Log CFU g−1); —— predicted boundary at 4 °C (GCt45/t0 = 1.0 Log CFU g−1) and ––– predicted boundary at 20 °C (GCt6/t0 = 1.0 Log CFU g−1); □ area of aw/pH measured for commercial diced bacon.
Discussion
In this work, we studied the influence of several formulation scenarios (pH and aw) and heat-processing regimes on the behaviour of L. monocytogenes during common phases of delicatessen product processes. As the structure of the medium has been shown to have a great influence on bacterial development (Robins & Brocklehurst, 1994; Wilson et al., 2002), we worked with ground pork, a solid medium. Moreover, we worked at temperatures (during the heat-processing regime) where a decrease of the population takes place and thermal inactivation kinetics have also been shown to be dependent on the nature of the medium (Doyle et al., 2001). Nevertheless, working with solid medium is more complicated than working with broth: enumeration of bacterial population is more time-consuming and expensive and the homogeneity of pH, aw and bacterial concentration is more difficult to obtain. Thus, we used minced meat in order to guarantee the homogeneity in the meat batter. Moreover, even if we used irradiated meat, the results of this study are also valid for describing the behaviour of L. monocytogenes in delicatessen products. Indeed, it has been shown that microbial interactions only occurred at population densities of 108 CFU mL−1 of protective culture (Malakar et al., 2003), but at this level spoilage is evident, except for fermented foods.
We first analysed the variability of our protocol by repeating the central condition of the experimental design (pH 5.6/aw 0.95) four times for the four processing regimes (including the control). Variations were small, regardless of the phase, so we considered that no replicate was necessary. It must be pointed out that standard deviation are calculated on the overall kinetics. Usually, the variability for lag and μmax are calculated separately. Variation coefficients are about 0.17 for lag and 0.09 for μmax, when experiments are carried out in broth (Bégot et al., 1996). In our case, we took both parameters into account and obtained a variation coefficient that was always lower than 0.20. Thus, we have developed an original meat matrix, in which the physicochemical and microbial parameters could be adjusted homogeneously and which can be used as a meat model to study the behaviour of bacteria in meat products such as delicatessen products (pork sausage, diced bacon, pork tongue, etc.).
During the heat process phase at 4 h/42 °C, 1 h/48 °C and 1 h/53 °C, a bactericidal or bacteriostatic effect was always observed. Some decrease of the population was observed at 42 °C, even if this temperature is lower than the maximal temperature for growth of the strain used (about 45.5 °C). Nevertheless, this temperature was determined in broth, and we can suppose that the growth in meat is an additional stress that contributes to lowering the maximal temperature for growth. We can also suppose that as for minimal temperature for Listeria growth, maximal temperature is also influenced by values of other environmental parameters. Thus maximal temperature for Listeria growth would be lower than 45.5 °C when pH and aw would move away from optimal values (Zuliani, 2005).
For the control treatment (24 h/20 °C), L. monocytogenes growth was observed for high values of pH and aw and no bactericidal effect was observed. We could have obtained a greater bactericidal effect by increasing the temperature of the heat process and/or the time of application. But this option was not applicable because it would have resulted in cooked meat with unsatisfactory organoleptic qualities. Indeed, in previous experimental tests (data not shown), initial changes in meat colour were observed for heat process phases that lasted longer or that were performed at higher temperatures. Therefore, in the range acceptable for the desired organoleptic qualities, the heat-processing regime has to be combined with other hurdles (Leistner, 1999) in order to ensure the microbiological quality of raw delicatessen products. As interactions between pH (or aw) and heat-processing regimes have a highly significant effect on the GC during the heat process phase, it is essential to adjust pH and aw. Beuchat et al. (1986), referred to by Doyle et al., 2001) and Juneja & Eblen (1999), referred to by Doyle et al., 2001) found that the heat resistance of L. monocytogenes was reduced at low pH. For aw, Grau & Vanderline (1993) and Yen et al. (1991) showed that the addition of 3% NaCl to ground pork enhanced the heat resistance of L. monocytogenes. The same results were observed by Passos & Kuaye (2002) in beef hamburgers with 1.5% NaCl. Our study confirms that with the increase of NaCl from 3.6 (aw 0.97) to 8.6% (aw 0.93), L. monocytogenes was more resistant to heat during the process phase. Indeed, higher GCs were observed for 1 h/53 °C when aw was equal to or lower than 0.95 and for 4 h/42 °C when aw was equal to or lower than 0.94.
Immediately after the heat process phase, ground pork was stored at 20 °C, a temperature that is favourable to L. monocytogenes growth. We showed that pH, aw and their interactions are the determining factors as low values could inhibit the increase of the bacterial population. Such interactions have already been observed by Augustin & Carlier (2000), McClure et al. (1989) and Cole et al. (1990) even at lower temperature than 20 °C.
Even if the processing regime influenced the GC of Listeria during the two first phase when analysed separately, it was observed that the population at the end of storage was similar to the one measured for the control treatment after the storage when the inoculum level was equal for all the conditions tested.
In the literature, thermal inactivation is generally studied alone and pronounced effects are shown on the bacterial population (Gaze et al., 1989; Juneja et al., 1997). Our study shows that a single phase of the process must not be taken alone but should be included and studied within the framework of the global process whenever possible. The effect of a single operation can be minimised or emphasised by other steps of the process.
In our study, we defined an area where growth occurred (GCt6/t0 > 1.0 Log CFU g−1) and an area where bactericidal or bacteriostatic effects (GCt6/t0 < 1.0 Log CFU g−1) were observed. The boundary that separates the two areas corresponds to a GC equal to 1.0 Log CFU g−1 (Fig. 3). The study of the boundary of the growth area of Listeria (identical, regardless of the heat-processing regime) confirms the effect of pH, aw and their interactions as described by Leistner (1999), Augustin & Carlier (2000) and Koutsoumanis et al. (2004). Our study showed a minimal pH for L. monocytogenes growth of 5.1 when aw was 0.97 and only 6.2 when aw was 0.93. Comparison with other data is very difficult because relatively few publications consider the effect of multiple factors, especially in food model products (Roberts et al., 1979; Wilson et al., 2002). The boundary of the growth area of Listeria at 20 °C in ground pork is correctly predicted by our method, combining Augustin's and Rosso's models. In most of the cases, the determination of the growth/no-growth interface is based on the logit of the probability P that the organism will grow (Tienungoon et al., 2000). The limit of this approach is that the determination of the probability requires replicates. In their work, Tienungoon et al. (2000) carried out experiments in broth and four replicates were generally made using turbidity methods. Nevertheless, these types of methods cannot be applied to solid food. Augustin (1999) and Le Marc (2001) also developed a method for determining the boundary of the growth/no-growth areas. They defined the boundary as the environmental conditions that make it possible for a μmax to be equal to 0. Our method is original because it takes both lag and μmax values into account and predict directly the population level after a period of time. It is particularly well adapted to industrial manufacturers whose aim is to know the level of contamination that is lower than a limit at the used-by-date of their product. Comparing the growth area of Listeria in ground meat with the average of the values for pH and aw measured for commercial delicatessen products such as diced bacon indicates that this product is compatible with the growth of L. monocytogenes. Indeed, the pH measured in different commercial diced bacons ranged from 5.8 to 6.1 and aw from 0.955 to 0.965. Nevertheless, the minimal aw and minimal pH for growth also depend on the temperature and concentrations of inhibitors (Farber et al., 1989; Cole et al., 1990; Le Marc et al., 2002). Thus, by reducing the storage temperature from 20 °C to refrigeration temperature or by adding nitrite or organic acid salt, frequently used in delicatessen products (Durand, 1999), the boundary of the growth area will be modified and the minimal pH and minimal aw for growth will increase. With our model, we simulated the incidence of a decrease of the storage temperature from 20 to 4 °C on the evolution of the boundary of the growth area (Fig. 3). At 20 °C, we considered that the boundary for GCt6/t0 was equal to 1.0 Log CFU g−1 after 7 days at 20 °C. At 4 °C, we chose the storage time on the basis of the use-by-date of commercial diced bacon, i.e. 45 days. We therefore considered that growth took place when the increase of the population of Listeria was higher than 1.0 Log CFU g−1 in 45 days. The same methodology as presented in the Modelling section was followed, and we obtained the boundary between growth and the no-growth areas at 4 °C. Figure 3 shows that when temperature decreased, minimal pH and aw where a growth was observed increased. The area compatible with the growth of Listeria is thus reduced compared with the one observed when meat is stored at 20 °C. pH and aw domain of diced bacons is located around the boundary of the growth area. This product may result in the development of Listeria, even at low temperatures. Similar predictions can be made by taking other parameters into account, as Augustin's model is a cardinal one for which the influences of environmental parameters on μmax and lag are multiplicative. For example, we could study the influence of additives frequently used in delicatessen products on the evolution of the boundary of the growth area and estimate the areas where Listeria growth is inhibited.
Conclusion
In the industrial raw delicatessen product process, where the heat process is used to guarantee satisfactory organoleptic qualities, we have shown that one treatment considered alone is not adequate to ensure the microbiological quality of the product. Nevertheless, a heat-processing regime at sublethal temperatures can inhibit the growth of Listeria during this phase. According to pH and aw, the heat process can even make it possible to reduce L. monocytogenes contamination, especially at 1 h/53 °C, but this reduction of the contamination level is not extended during the storage phase. Therefore, for the global process, pH and aw have a highly significant effect on the area compatible with growth of Listeria, contrary to the heat-processing regime.
The boundary of the growth area in pork meat is well predicted by the combination of Augustin's and Rosso's models. This novel method could be used by the meat industry to predict the microbial safety of its products. Indeed, in addition to temperature, pH and aw, the effect of additives frequently found in delicatessen products and which have shown an antilisterial effect, such as organic acids (Dubal et al., 2004), phenols (Membréet al., 1997) and nitrite (Buchanan et al., 1989), could be taken into account for determining the boundary of the growth area. The use of such meat model to study the behaviour of bacteria in delicatessen product is encouraging.
Acknowledgments
The authors thank the ANRT (Association Nationale de la Recherche Technique) for its financial assistance and Dr Carole Feurer for the English corrections.