-
PDF
- Split View
-
Views
-
Cite
Cite
A Christodoulaki, H He, M Zhou, A Cardona Barberán, C De Roo, S M Chuva De Sousa Lopes, M Baetens, B Menten, A Van Soom, P De Sutter, S Weyers, A Boel, D Stoop, B Heindryckx, Characterization of ovarian tissue oocytes from transgender men reveals poor calcium release and embryo development, which might be overcome by spindle transfer, Human Reproduction, Volume 38, Issue 6, June 2023, Pages 1135–1150, https://doi.org/10.1093/humrep/dead068
- Share Icon Share
Abstract
Can spindle transfer (ST) overcome inferior embryonic development of in vitro matured ovarian tissue oocytes (OTO-IVM) originating from testosterone-treated transgender men?
ST shows some potential to overcome the embryo developmental arrest observed in OTO-IVM oocytes from transgender men.
OTO-IVM is being applied as a complementary approach to increase the number of oocytes/embryos available for fertility preservation during ovarian tissue cryopreservation in cancer patients. OTO-IVM has also been proposed for transgender men, although the potential of their oocytes remains poorly investigated. Currently, only one study has examined the ability of OTO-IVM oocytes originating from transgender men to support embryo development, and that study has shown that they exhibit poor potential.
Both ovaries from 18 transgender men undergoing oophorectomy were collected for the purposes of this study, from November 2020 to September 2022. The patients did not wish to cryopreserve their tissue for fertility preservation and donated their ovaries for research. All patients were having testosterone treatment at the time of oophorectomy and some of them were also having menses inhibition treatment.
Sibling ovaries were collected in either cold or warm medium, to identify the most optimal collection temperature. Cumulus oocyte complexes (COCs) from each condition were isolated from the ovarian tissue and matured in vitro for 48 h. The quality of OTO-IVM oocytes was assessed by calcium pattern releasing ability, embryo developmental competence following ICSI, and staining for mitochondrial membrane potential. In vitro matured metaphase I (MI) oocytes, germinal vesicle (GV) oocytes, and in vivo matured oocytes with aggregates of smooth endoplasmic reticulum (SERa) were donated from ovarian stimulated women undergoing infertility treatment and these served as Control oocytes for the study groups. ST was applied to overcome poor oocyte quality. Specifically, enucleated mature Control oocytes served as cytoplasmic recipients of the OTO-IVM spindles from the transgender men. Embryos derived from the different groups were scored and analysed by shallow whole genome sequencing for copy number variations (CNVs).
In total, 331 COCs were collected in the cold condition (OTO-Cold) and 282 were collected in the warm condition (OTO-Warm) from transgender men. The maturation rate was close to 54% for OTO-Cold and 57% for OTO-Warm oocytes. Control oocytes showed a calcium releasing ability of 2.30 AU (n = 39), significantly higher than OTO-Cold (1.47 AU, P = 0.046) oocytes (n = 33) and OTO-Warm (1.03 AU, P = 0.036) oocytes (n = 31); both values of calcium release were similar between the two collection temperatures. Mitochondrial membrane potential did not reveal major differences between Control, OTO-Warm, and OTO-Cold oocytes (P = 0.417). Following ICSI, 59/70 (84.2%) of Control oocytes were fertilized, which was significantly higher compared to 19/47 (40.4%) of OTO-Cold (P < 0.01) and 24/48 (50%) of OTO-Warm oocytes (P < 0.01). In total, 15/59 (25.4%) blastocysts were formed on Day 5 in the Control group, significantly higher than 0/19 (0%) from the OTO-Cold (P = 0.014) and 1/24 (4.1%) in OTO-Warm oocytes (P = 0.026). Application of ST rescued the poor embryo development, by increasing the Day 5 blastocyst rate from 0% (0/19) to 20.6% (6/29) (P = 0.034), similar to that in the ICSI-Control group (25.4%, 15/59). A normal genetic profile was observed in 72.7% (8/11) of OTO-Cold, 72.7% (8/11) of OTO-Warm and 64.7% (11/17) of Control Day 3–Day 5 embryos. After ST was applied for OTO-IVM oocytes, 41.1% (7/17) of the embryos displayed normal genetic patterns, compared to 57.1% (4/7) among ST-Control Day 3–Day 5 embryos.
N/A.
Due to the limited access to human oocytes and ovarian tissue, our results should be interpreted with some caution, as only a limited number of human oocytes and embryos could be investigated.
The results of this study, clearly indicate that OTO-IVM oocytes originating from transgender patients are of inferior quality, which questions their use for fertility preservation. The poor quality is likely to be related to cytoplasmic factors, supported by the increased blastocyst numbers following application of ST. Future research on OTO-IVM from transgender men should focus on the cytoplasmic content of oocytes or supplementation of media with factors that promote cytoplasmic maturation. A more detailed study on the effect of the length of testosterone treatment is also currently missing for more concrete guidelines and guidance on the fertility options of transgender men. Furthermore, our study suggests a potentially beneficial role of experimental ST in overcoming poor embryo development related to cytoplasmic quality.
A.C. is a holder of FWO grants (1S80220N and 1S80222N). A.B. is a holder of an FWO grant (1298722N). B.H. and A.V.S. have been awarded with a special BOF (Bijzonder Onderzoeksfonds), GOA (Geconcerteerde onderzoeksacties) and 2018000504 (GOA030-18 BOF) funding. B.H. has additional grants from FWO-Vlaanderen (Flemish Fund for Scientific Research, G051516N and G1507816N) and Ghent University Special Research Fund (Bijzonder Onderzoeksfonds, BOF funding (BOF/STA/202109/005)), and has been receiving unrestricted educational funding from Ferring Pharmaceuticals (Aalst, Belgium). The authors declare that they have no conflict of interest.
N/A.
Introduction
Gender affirming therapies for transgender men aim at enhancing masculine characteristics and overcoming gender dysphoria (T’Sjoen et al., 2019). Nevertheless, medical interventions such as hormonal and especially surgical treatments, might impair normal ovarian function, resulting in subfertility or infertility (De Roo et al., 2016; Hembree et al., 2017). Conclusive research on the parental wish among transgender men is currently lacking. Studies have demonstrated that about 50% of transgender individuals wish to have children (Wierckx et al., 2012a,b; De Roo et al., 2016). For this reason, fertility guidance should be offered to transgender men before initiation of their treatment (Mayhew et al., 2020).
Current fertility preservation strategies for transgender men include oocyte or embryo cryopreservation (Cheng et al., 2019; Leung et al., 2019). However, this approach requires ovarian stimulation, leading to elevated oestrogen levels. Testosterone supplementation is ceased for several months before oocyte recovery and vaginal ultrasounds are needed to follow up the ovarian stimulation prior to oocyte retrieval. These interventions potentially enhance gender dysphoria and discomfort in transgender men (Moravek, 2018; Mayhew and Gomez-Lobo, 2020).
Ovarian tissue cryopreservation (OTC) is another fertility preservation technique considered for transgender men (Bearelly et al., 2020). In contrast to oocyte or embryo cryopreservation, no prior ovarian stimulation or withdrawal of testosterone treatment is required and ovariectomy can take place during gender affirming surgery (Moravek, 2018). Ovarian tissue cryopreservation is a common fertility preservation strategy for cancer patients as ovarian tissue transplanted following successful cancer treatment can restore hormonal cyclicity and even lead to live births (Andersen et al., 2019; Dolmans et al., 2021). Nonetheless, transplantation of ovarian tissue in transgender men would lead to restoration of elevated oestrogen levels and interfere with the desired masculine characteristics (Sterling and Garcia, 2020).
In vitro maturation of ovarian tissue oocytes (OTO-IVM) following oophorectomy represents a possible alternative treatment method. This approach is considered for fertility preservation of cancer patients, complementary to OTC, or in cases where transplantation of ovarian tissue could potentially lead to cancer recurrence (De Roo and Tilleman, 2021). In this strategy, cumulus oocyte complexes (COCs) containing immature oocytes are collected following oophorectomy (Kirillova et al., 2021). Cryopreserved oocytes from OTO-IVM can be thawed when a child wish is expressed, and live births have been reported in cancer patients making use of this approach (Prasath et al., 2014; Uzelac et al., 2015; Segers et al., 2020).
For transgender patients, extensive knowledge on the quality and safety of OTO-IVM oocytes as a strategy for fertility preservation is currently lacking. Recent studies for transgender men display the potential of OTO-IVM oocytes to mature at a rate of 23.8–39% (De Roo et al., 2017; Lierman et al., 2017, 2021). In the most recent study on OTO-IVM in transgender men (Lierman et al., 2021), the ability of these oocytes to be fertilized was demonstrated. Nevertheless, from the 1903 harvested COCs, only 453 matured (23.8%). In total, 139 mature oocytes were injected with sperm and only one Day 5 blastocyst could be established, due to the combination of low fertilization and embryo development rates. Genetic analysis of the arrested embryos demonstrated that genetic abnormalities are probably not the reason for the impaired embryonic development (Lierman et al., 2021). These results might therefore rather be attributed to the collection protocol or to the lack of cytoplasmic maturity in OTO-IVM oocytes from transgender men.
The oocytes of transgender men are exposed to testosterone treatment and originate from small antral follicles (De Roo et al., 2017). Furthermore, despite the significant improvements in assisted reproductive technologies (ART), IVM results in general remain variable. This is mostly attributed to the large variety of protocols and applications of IVM, with the most challenging aspect being synchronization of nuclear and cytoplasmic maturation (Coticchio et al., 2015). Thus, inferior cytoplasmic maturation could also be a factor affecting embryo development in the above-mentioned study.
Another factor which could affect the rate of embryo development is that, prior to OTC, exposure of the tissue to ice is sometimes necessary, in order to avoid tissue damage and to slow down the cell metabolic rate, especially when ovaries are transported from distant hospitals (Pors et al., 2021). Nevertheless, extensive exposure of the tissue to ice could potentially affect the COCs enclosed in the antral follicles (Wilken-Jensen et al., 2014; Fasano et al., 2017; Nikiforov et al., 2020).
The aim of the current study was to evaluate the quality of OTO-IVM oocytes from transgender men and overcome the poor embryonic development reported previously for these patients. With this aim, we first investigated the effect of tissue collection temperature on oocyte maturation and embryo development, by collecting ovarian tissue from transgender mean and placing the samples on ice or in prewarmed collection medium. The maturation rate, the oocyte diameter, and the embryo development process were examined for both conditions. Furthermore, the calcium releasing ability was investigated as indicator of oocyte cytoplasmic quality. Calcium plays an important role in oocyte fertilization and embryo development (Yoon et al., 2008; Zafar et al., 2021), with both oocyte and sperm factors contributing to the calcium release; irregularities in the calcium releasing machinery have been linked to fertilization failure (Yeste et al., 2016; Ferrer-Buitrago et al., 2019).
Moreover, we investigated mitochondrial membrane potential. Mitochondria are important organelles for oocyte function and are solely derived from the mother (Otten and Smeets, 2015). Although there are only about 100 mitochondrial DNA copies in sperm cells, it is known that oocytes have about 100 000–600 000 (Craven et al., 2017). Since mitochondrial replication does not occur earlier than the blastocyst stage in embryos, the initial numbers and functionality of available mitochondria in the oocyte will determine the energy needed for the events of fertilization and embryo development (Craven et al., 2017).
In order to overcome poor embryo development of OTO-IVM oocytes originating from transgender patients, we applied spindle transfer (ST). This technique is a type of nuclear transfer (NT) approach, which involves the transfer of the genetic material of a certain oocyte or zygote to an enucleated counterpart (Craven and Turnbull, 2019). The technology was initially developed to overcome the transmission of mitochondrial DNA diseases from mothers to offspring (Craven et al., 2016). NT has also been considered as a solution to poor oocyte quality, as it involves the transfer of the genetic material to a completely new, suitable cytoplasm (Craven et al., 2017). There have been promising animal and human studies on the application of this technique for certain indications, such as advanced maternal age in a mouse model (Tang et al., 2020), failed fertilization in humans (Tang et al., 2022), and embryo developmental arrest in mice (Costa-Borges et al., 2020; Tang et al., 2020). Thus, cytoplasmic factors seem to contribute to failed fertilization or inferior embryonic development. In our study, we applied ST by transferring the spindle of OTO-IVM oocytes from transgender men to the cytoplasm of enucleated oocytes from patients who have undergone ovarian stimulation. Finally, we evaluated chromosomal integrity by copy number analysis in the derived embryos from OTO-IVM oocytes of transgender men, control oocytes, and ST oocytes.
Materials and methods
Participants and ethical approval
In total, 18 transgender men with planned operations in the Department for Reproductive Medicine, Ghent University Hospital were recruited for the study from November 2020 to September 2022. Only patients between the age of 18–35 years were considered for inclusion. Patients with no wish for fertility preservation signed a written informed consent form to donate both of their ovaries for this research.
Transgender men had a mean age of 20.5 years (min 18, max 24). All patients had undergone testosterone treatment for a mean of 25.5 months (min 12, max 52). Nine patients had undergone menses inhibition therapy (using additional progesterone) at the time of the surgery for an average of 32.1 months (min 1, max 60), four patients had discontinued menses inhibition before surgery, and five patients had never received menses inhibition. Detailed patient characteristics can be found in Supplementary Table SI.
The use of human ovaries, oocytes, and sperm was approved by the Ghent University Hospital Ethical Committee (EC 2019/1270) and approval for the creation of the embryos was obtained from the Belgian Federal Commission for medical and scientific research on embryos in vitro (FCE-ADV_083_UZGent). All participants agreed by signing an informed consent specific for this study.
Collection of ovaries
Following bilateral oophorectomy of transgender men, sibling ovaries were collected in prewarmed (37°C) or cold collection (on ice) medium (L-15 Leibovitz medium, Sigma) supplemented with 0.45% human serum albumin (Red Cross, Belgium). Ovaries were collected immediately after isolation from the surgical room of Ghent University Hospital and transferred within 10 min to the laboratory, where ovarian weight was recorded (Supplementary Table SII). Ovaries collected in warm medium were manipulated first, whilst ovaries collected in cold medium stayed on ice for 2–3 h following collection. For seven of the patients, both ovaries were collected on ice.
Collection of COCs
Following arrival in the lab, the ovaries were examined on their surface for visible follicles. Follicles were immediately aspirated with 18-gauge needles (VWR). Aspirated follicular fluid was examined under the stereomicroscope for the presence of COCs. Ovaries were cut in half and medulla tissue was separated from the cortex. Residual pieces of medulla and spent medium were investigated, under a heat-stage (37°C) Olympus SZ40 stereo microscope for the presence of COCs. COCs were collected in prewarmed Sydney IVF gamete buffer (Cook Medical) and subsequently cultured in in-house IVM medium. IVM medium consisted of tissue culture medium (TCM 199, Sigma), supplemented with 10 mIU/ml recombinant FSH (Puregon), 0.5 IU/ml HCG (Pregnyl), 10 ng/ml epidermal growth factor (Sigma), 1 μg/ml estradiol (Sigma), 0.8% human serum albumin (Red Cross, Belgium), 100 IU/ml penicillin G and 100 μg/ml streptomycin (Sigma), 0.3 mM sodium pyruvate (Sigma), and 1 mM l-glutamine (Sigma). COCs were cultured for 48 h in groups of 10, in 4-well dishes under mineral oil and standard culture conditions (37°C, 6% CO2, 5% O2).
Collection of human control oocytes
Immature oocytes donated from ovarian stimulated women undergoing infertility treatment at the Department for Reproductive Medicine, Ghent University Hospital, were subjected to IVM and these served as Controls. Oocytes retrieved at prophase I, i.e. germinal vesicle stage (GV), were cultured for 24 h in the same IVM medium mentioned above. Collected metaphase I (MI) oocytes were cultured for either 3 or 24 h in Sydney IVF Cleavage Medium (Cook Medical). In vivo matured metaphase II (MII) oocytes containing aggregates of smooth endoplasmic reticulum (SERa) were also used as Control oocytes, and were collected at a minimum of 8 h after oocyte retrieval. Successfully matured oocytes were used as Control oocytes for ICSI, calcium imaging or mitochondrial staining, or as cytoplasmic recipients for ST.
Oocyte diameter
Diameters of mature oocytes were measured with Image J software, by calculating the average of two perpendicular diameters for each oocyte (excluding zona pellucida).
Sperm samples
Residual donated sperm from two men undergoing IVF in the clinic was used for all experiments. Patient 1 was 30 years old, with proven fertility, and normozoospermic: 7 ml total volume, 19.27×106 spermatozoa/ml, and 63.5% progressive motility (type a + b). Patient 2 was 36 years old, with proven fertility, and normozoospermic: 4.7 ml total volume, 31.75×106 spermatozoa/ml, and 50.8% progressive motility. Sperm samples were frozen (SpermFreeze, Fertipro) and thawed for the purposes of the different experiments.
Evaluation of oocyte quality: calcium imaging, mitochondrial staining, and embryo development
Following 48 h of culture in the IVM medium, OTO-IVM oocytes were exposed to hyaluronidase (200 IU/ml, Sigma–Aldrich, Belgium) for 1 min, washed extensively in gamete buffer, and scored for their maturation status under a stereomicroscope. In vitro matured ovarian tissue oocytes from ovaries collected in cold (OTO-Cold) and warm medium (OTO-Warm) were either analysed by calcium imaging or injected with donated sperm by ICSI and monitored up to Day 5 of embryonic development.
Calcium imaging was performed as described previously by our team (Ferrer-Buitrago et al., 2019). Briefly, oocytes were exposed for 30 min in Sydney IVF Cleavage Medium containing fura-PE3-AM (Teflabs, TX, USA) in a concentration of 7.5 μΜ under standard culture conditions. Following 30 min, oocytes were injected with a single sperm via ICSI in an inverted microscope (Olympus IX71). ICSI was performed within 30 min and oocytes were then transferred to an inverted epifluorescence microscope (Olympus IX71, Olympus Soft Imaging Solutions GmbH, Belgium) under standard culture conditions.
Calcium release was recorded for 10 consecutive hours, with a 10× objective and a filter switch (Lambda DG-4 filter switch, Sutter Instrument Company, Novato, CA, USA) to provide excitation alternating between 340 and 380 nm. Calcium data were analysed using Clampfit 10.2 software (Axon Laboratories, Molecular Devices UK Ltd). The total amount of calcium released (in arbitrary units (AU)) was calculated as the product of the mean amplitude (maximum fluorescence intensity per peak) per mean frequency (number of calcium spikes) of all oocytes injected per condition (including the oocytes showing no calcium peaks).
To measure mitochondrial membrane potential, OTO-Cold, OTO-Warm, and Control oocytes were exposed to JC-1 staining (Invitrogen). JC-1 is a dual emission dye, accumulating in the mitochondria. When the mitochondrial membrane potential (ΔΨm) is >140 mV, JC-1 forms aggregates and emits red fluorescence (emission 590 nm). If ΔΨm is <100 mV, JC-1 remains a monomer and emits green fluorescence (emission 529 nm). Specifically, oocytes were exposed to 5 µg/ml JC-1 in Sydney IVF Cleavage Medium for 35 min in standard culture conditions. Following staining, oocytes were imaged in small groups, in a confocal microscope (Zeiss LSM9000). Z-stack images were generated for each oocyte. Images were then merged, and intensity was analysed using Image J software. The ΔΨm value for every oocyte, was calculated as the ratio of the intensity between red and green fluorescence.
For embryo development, oocytes were checked after 18 h for the formation of pronuclei, following ICSI. Only oocytes with two pronuclei were kept in culture, while zygotes with an abnormal number of pronuclei or showing direct cleavage were not kept in culture and were considered unsuitable. Embryos were cultured in Sydney IVF Cleavage Medium up to Day 3 and then transferred to Sydney IVF Blastocyst Medium (Cook Medical). Embryos were scored daily for their cell number and fragmentation until Day 5. Embryo scoring was performed on Days 2, 3, and 5 according to ESHRE guidelines (Balaban et al., 2011), as previously mentioned by our group (Bonte et al., 2019).
Spindle transfer
ST in human oocytes was carried out as previously described (Tang et al., 2022). Briefly, OTO-IVM oocytes from transgender patients and donated spare MII oocytes from infertility patients were placed in 10 μl droplets of gamete buffer, supplemented with 5 μg/ml cytochalasin B in a glass-bottom dish. Droplets were covered with mineral oil and oocytes were left at 37°C for 10 min before manipulation. Recipient oocytes were enucleated, after drilling a hole in the zona pellucida with a laser objective and the spindle was visualized using OosightTM Imaging System in an inverted microscope (Olympus IX71). The spindle-chromosome-complex was removed using a 15 μm enucleation pipette. Oocytes were washed in gamete buffer and let to rest in the incubator for 20 min. Following 20 min, spindles from OTO-IVM oocytes were transferred in the enucleated recipients. The spindle was briefly exposed to haemagglutinating virus of Japan envelope (HVJ-E) (GenomONE-CF EX, Cosmo Bio) and placed in the perivitelline space (PV) of the recipient oocyte. Fusion occurred within 30 min and reconstructed oocytes were fertilized by ICSI, using frozen/thawed sperm, within 2 h following fusion, and then cultured in Sydney IVF Cleavage Medium (Days 1–3) or Sydney IVF Blastocyst Medium (Days 3–5), under mineral oil at standard culture conditions.
Genetic analysis
In order to detect copy number variations (CNVs) in generated embryos, we performed shallow whole genome sequencing as described previously (Popovic et al., 2018). Briefly, whole embryos were collected in phosphate-buffered saline (PBS) and stored at −20°C until genetic analysis. Whole genome amplification (WGA) was performed with the SurePlex DNA amplification kit (Rubicon Genomics Inc., Ann Arbor, MI, USA). DNA was fragmented to ∼200 bp using a M220 Focused-ultrasonicator Instrument (Covaris, Woburn, MA, USA) and subsequent library preparation was performed with the NEXTflex™ Rapid DNA-Seq Library Prep Kit for Illumina Sequencing (Bioo Scientific, Uden, Netherlands). Agencourt AMPure XP beads (Beckman Coulter, Suarlée, Belgium) were used for purification. Template preparation was performed on the cBot™ System (Illumina, San Diego, CA, USA), using 2.5 nM of equimolar pooled libraries, and sequencing was performed on a Hiseq3000 sequencer (Illumina, San Diego, CA, USA). Data analysis for CNV detection was performed using the WisecondorX and Vivar software (Sante et al., 2014; Raman et al., 2019).
Statistical analysis
Categorical variables expressed in percentages (%) were compared using chi-square test (χ2). The average AU values expressing total calcium release and average oocyte diameters were compared using the non-parametric Kruskal–Wallis test. Bonferroni correction was applied before consulting the P value. Correlation between continuous variants was performed with Pearson’s correlation test. Statistical significance was set to P < 0.05. Statistical analysis was performed with the SPSS Statistics program (version 27).
Results
Collection temperature of ovarian tissue did not affect maturation rate or oocyte diameter in OTO-IVM oocytes of transgender men
A total of 12 transgender patients (Patients 1–9, Patients 14, 17, and 18 Supplementary Table SI) were recruited for the comparison between cold and warm collection medium. In total, 613 COCs were collected, with a mean of approximately 47 COCs per patient (minimum 27, maximum 72 COCs). Thirteen ovaries were collected in cold medium, while eleven ovaries were collected in warm medium. In total, 331 COCs were collected in the cold condition (OTO-Cold) and 282 were collected in the warm condition (OTO-Warm). Only healthy-looking COCs were collected for IVM (Fig. 1A). Following 48 h in the IVM medium, some oocytes degenerated (Fig. 1B), some matured (Fig. 1C), while others remained at the GV stage (Fig. 1D) or progressed only to the MI stage (Fig. 1E). In total, significantly more OTO-Cold oocytes, 264/331 (79.7%) survived following IVM culture compared to 196/282 (69.5%) OTO-Warm oocytes (P = 0.003). From the 264 oocytes surviving in the OTO-Cold group, 143 matured (54.1%), which was similar to that in the OTO-Warm group, with 112/196 (57.1%) reaching the MII stage (P = 0.525).
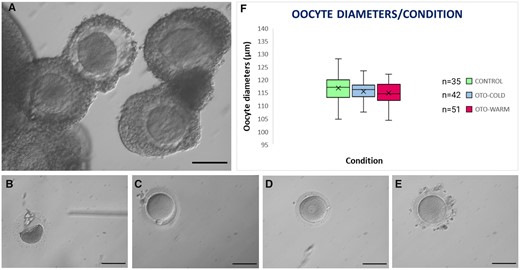
Type of oocytes classified following IVM for 48 h and oocyte diameters. (A) Appearance of healthy COCs collected for IVM. (B) Degenerated, (C) Mature, (D) GV, and (E) MI oocytes following 48 h of IVM. Scale bar: 100 μm. (F) Box plot representing the oocyte diameters of mature Control oocytes and OTO-IVM oocytes of transgender men collected in cold or warm medium. COCs, cumulus oocyte complexes; IVM, in vitro maturation; GV, germinal vesicle; MI, metaphase I; OTO-IVM, in vitro matured ovarian tissue oocytes.
Considering the length of testosterone treatment, we performed Pearson’s correlation to detect any correlation with the number of oocytes retrieved or matured. The correlation coefficient was 0.405 (P = 0.096) and thus, the months of testosterone treatment did not seem to affect the number of COCs collected. Also no significant correlation was detected between the length of the treatment and the maturation rate following 48 h in IVM medium (r = 0.319, P = 0.197).
Diameters of mature oocytes were calculated by measuring two perpendicular diameters on the oocyte and calculating the average of the two measurements. In total, we measured the diameters of 35 Control, 42 OTO-Cold, and 51 OTO-Warm oocytes (Fig. 1F). The average oocyte diameter for Control oocytes was 116.86 μm, which was similar for OTO-Cold (115.57 μm) and OTO-Warm (114.98 μm) oocytes (P = 0.236). Following maturation, oocytes were randomly distributed to the different groups and assessed for embryo development, calcium imaging, and mitochondrial membrane potential.
Total calcium release is impaired in OTO-IVM oocytes from transgender men
In total, 33 OTO-Cold, 31 OTO-Warm, and 39 Control oocytes were analysed for the total calcium released. To further describe the calcium patterns observed in each group, the number of oocytes in each condition showing 0 (Fig. 2A), 1–2 (Fig. 2B), or more than 3 peaks (Fig. 2C) was also determined.
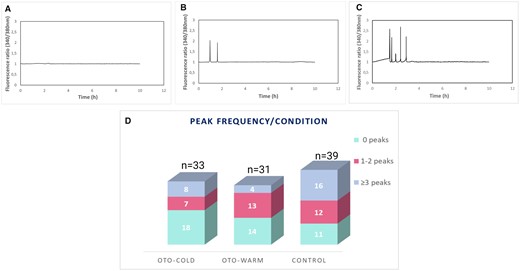
Calcium imaging analysis of OTO-IVM and Control oocytes. Generated graphs for (A) an oocyte with 0, (B) 2, and (C) 5 peaks. (D) Graph representing the peak frequency for every condition. The total number of analysed oocytes for every condition is indicated at the top of each column. OTO-COLD, in vitro matured ovarian tissue oocytes from transgender men collected from ovaries transferred in cold medium; OTO-WARM, in vitro matured ovarian tissue oocytes from transgender men collected from ovaries transferred in prewarmed medium; OTO-IVM, in vitro matured ovarian tissue oocytes.
In total, 15/33 OTO-Cold, 17/31 OTO-Warm, and 28/39 Control oocytes showed peaks, indicating calcium release. A higher number of oocytes exhibiting >3 peaks was present in the Control group. In total, 18, 14, and 11 oocytes did not show any peaks in the OTO-Cold, OTO-Warm, and Control groups, respectively (Fig. 2D). The average of AxF (total calcium released) was calculated, including the oocytes showing no peaks for every group, resulting in 1.47 AU, 1.03 AU, and 2.30 AU for the OTO-Cold, OTO-Warm, and Control groups, respectively (Table I). Calcium release was significantly higher in Control oocytes when compared to OTO-Cold (P = 0.046) and OTO-Warm oocytes (P = 0.036, Table I). No statistically significant difference was observed between the OTO-Cold and OTO-Warm oocytes. These results indicate a superior oocyte quality of the Control oocytes, when compared to OTO-IVM oocytes derived from transgender men.
. | OTO-Cold . | OTO-Warm . | ICSI-Control . |
---|---|---|---|
Analysed oocytes | 33 | 31 | 39 |
Average A | 1.21 | 0.97 | 1.02 |
Average F | 1.21 | 1.06 | 2.25 |
AxF (AU) | 1.47*a | 1.03*b | 2.30 |
. | OTO-Cold . | OTO-Warm . | ICSI-Control . |
---|---|---|---|
Analysed oocytes | 33 | 31 | 39 |
Average A | 1.21 | 0.97 | 1.02 |
Average F | 1.21 | 1.06 | 2.25 |
AxF (AU) | 1.47*a | 1.03*b | 2.30 |
OTO, ovarian tissue oocytes collected (in cold or warm medium) from transgender men and matured in vitro; control oocytes, immature oocytes or mature oocytes (with aggregates of smooth endoplasmic reticulum) collected from ovarian stimulated women undergoing IVF.
Fertilization was performed using ICSI with a single sperm cell. The average amplitude (A) value and average frequency (F) for each group are displayed. The product of AxF represents the Calcium release. Values of AxF were compared with Kruskal–Wallis statistical test. Differences with a P value <0.05 were considered significant. Asterisks represent a P value <0.05 between respective groups and ICSI-Control oocytes.
P = 0.046.
P = 0.036.
. | OTO-Cold . | OTO-Warm . | ICSI-Control . |
---|---|---|---|
Analysed oocytes | 33 | 31 | 39 |
Average A | 1.21 | 0.97 | 1.02 |
Average F | 1.21 | 1.06 | 2.25 |
AxF (AU) | 1.47*a | 1.03*b | 2.30 |
. | OTO-Cold . | OTO-Warm . | ICSI-Control . |
---|---|---|---|
Analysed oocytes | 33 | 31 | 39 |
Average A | 1.21 | 0.97 | 1.02 |
Average F | 1.21 | 1.06 | 2.25 |
AxF (AU) | 1.47*a | 1.03*b | 2.30 |
OTO, ovarian tissue oocytes collected (in cold or warm medium) from transgender men and matured in vitro; control oocytes, immature oocytes or mature oocytes (with aggregates of smooth endoplasmic reticulum) collected from ovarian stimulated women undergoing IVF.
Fertilization was performed using ICSI with a single sperm cell. The average amplitude (A) value and average frequency (F) for each group are displayed. The product of AxF represents the Calcium release. Values of AxF were compared with Kruskal–Wallis statistical test. Differences with a P value <0.05 were considered significant. Asterisks represent a P value <0.05 between respective groups and ICSI-Control oocytes.
P = 0.046.
P = 0.036.
Mitochondrial membrane potential is not impaired in OTO-IVM oocytes from transgender men
Using JC-1 staining, we examined the inner mitochondrial membrane potential of OTO-IVM oocytes from transgender men and Control oocytes. Following exposure to JC-1, oocytes emitted green (indicating compromised membranes) (Fig. 3A) and/or red light (indicating intact membranes). The merged images were used to calculate the intensity ratio between red and green fluorescence signal. In total, we analysed 29 OTO-Cold, 29 OTO-Warm, and 23 Control oocytes. The ratio of ΔΨm for the OTO-Cold oocytes was 1.24, which was higher than for the OTO-Warm group (0.79) and similar to the Control group (1.03). Nevertheless, no statistically significant difference was detected between the three groups (Kruskal Wallis test, P = 0.417). A box plot representing the intensity ratio between red/green fluorescence for all groups, can be found in Fig. 3B.
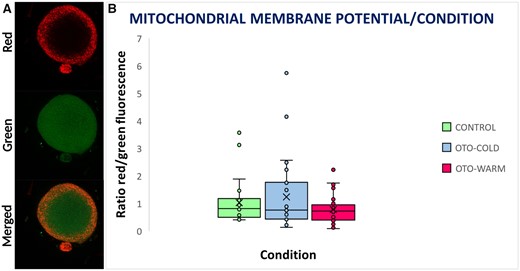
Mitochondrial staining and membrane potential in Control, OTO-Cold, and OTO-Warm oocytes. (A) Mitochondrial staining of a mature oocyte. In the upper panel, mitochondria with high membrane potential emit in red fluorescence. In the middle panel, mitochondria with compromised membrane potential emit in green fluorescence. In the lower panel, is a merged image from red and green channels. (B) Box plot representing the intensity of red/green fluorescence in Control, OTO-Cold, and OTO-Warm oocytes. OTO-COLD, in vitro matured ovarian tissue oocytes from transgender men collected from ovaries transferred in cold medium; OTO-WARM, in vitro matured ovarian tissue oocytes from transgender men collected from ovaries transferred in prewarmed medium.
Embryo developmental arrest is evident in OTO-IVM oocytes from transgender men
All remaining matured oocytes were used to assess subsequent embryonic development after fertilization. In total, 70 Control, 47 OTO-Cold, and 48 OTO-Warm oocytes underwent ICSI to monitor embryo development. In the Control group, 59/70 (84.2%) oocytes were normally fertilized (indicated by the presence of two pronuclei (PN)), which was significantly higher than 19/47 (40.4%) for OTO-Cold oocytes (P < 0.001) and 24/48 (50.0%) for OTO-Warm oocytes (P < 0.001) (Table II). No statistically significant difference was detected between the OTO-Cold and OTO-Warm groups (P = 0.349). Abnormal fertilization (3PN, 1PN, and direct cleavage) did not differ significantly between the Control and OTO-Cold groups (P = 0.148), with only 1/70 (1.4%) injected control oocytes exhibiting 3PN, 1/47 (2.1%), and 2/47 (4.2%) of the OTO-Cold zygotes exhibiting direct cleavage and 3PN, respectively (Table II). On the contrary, a significantly higher number of zygotes showed abnormal fertilization in the OTO-Warm group, with 2/48 (4.1%) exhibiting 1PN and 3/48 (6.2%) exhibiting 3PN, compared with the Control group (P = 0.029) (Table II). This rate did not differ significantly between OTO-Cold and OTO-Warm zygotes (P = 0.479).
. | OTO-Cold . | OTO-Warm . | ICSI-Control . | |
---|---|---|---|---|
Injected oocytes | 47 | 48 | 70 | |
Normally fertilized (2PN)—Day 1 | 19/47 (40.4%)*a | 24/48 (50.0%)*b | 59/70 (84.2%) | |
Abnormally fertilized | 1PN | – | 2/48 (4.1%) | – |
3PN | 2/47 (4.2%) | 3/48 (6.2%)*c | 1/70 (1.4%) | |
Direct cleavage | 1/47 (2.1%) | – | – | |
2-cells stage—Day 2 | 15/19 (78.9%) | 21/24 (87.5%) | 47/59 (79.6%) | |
4–8 cells stage—Day 3 | 7/19 (36.8%)*d | 14/24 (58.3%) | 42/59 (71.1%) | |
Blastocyst stage—Day 5 | –*e | 1/24 (4.1%)*f | 15/59 (25.4%) | |
Blastocyst stage—Day 6 | 1/19 (5.2%) | 1/24 (4.1%) | – |
. | OTO-Cold . | OTO-Warm . | ICSI-Control . | |
---|---|---|---|---|
Injected oocytes | 47 | 48 | 70 | |
Normally fertilized (2PN)—Day 1 | 19/47 (40.4%)*a | 24/48 (50.0%)*b | 59/70 (84.2%) | |
Abnormally fertilized | 1PN | – | 2/48 (4.1%) | – |
3PN | 2/47 (4.2%) | 3/48 (6.2%)*c | 1/70 (1.4%) | |
Direct cleavage | 1/47 (2.1%) | – | – | |
2-cells stage—Day 2 | 15/19 (78.9%) | 21/24 (87.5%) | 47/59 (79.6%) | |
4–8 cells stage—Day 3 | 7/19 (36.8%)*d | 14/24 (58.3%) | 42/59 (71.1%) | |
Blastocyst stage—Day 5 | –*e | 1/24 (4.1%)*f | 15/59 (25.4%) | |
Blastocyst stage—Day 6 | 1/19 (5.2%) | 1/24 (4.1%) | – |
OTO, ovarian tissue oocytes collected (in cold or warm medium) from transgender men and matured in vitro; control oocytes, immature oocytes or mature oocytes (with aggregates of smooth endoplasmic reticulum) collected from ovarian stimulated women undergoing IVF; PN, pronuclei.
Fertilization was performed using ICSI with a single sperm cell. Blastocyst rates were calculated based on the number of normally fertilized zygotes. Percentages were compared using the chi-square test and P < 0.05 was considered significant. Asterisks represent a P value <0.05 between respective groups and ICSI-Control oocytes.
P < 0.001.
P < 0.001.
P = 0.029.
P = 0.007.
P = 0.014.
P = 0.026.
. | OTO-Cold . | OTO-Warm . | ICSI-Control . | |
---|---|---|---|---|
Injected oocytes | 47 | 48 | 70 | |
Normally fertilized (2PN)—Day 1 | 19/47 (40.4%)*a | 24/48 (50.0%)*b | 59/70 (84.2%) | |
Abnormally fertilized | 1PN | – | 2/48 (4.1%) | – |
3PN | 2/47 (4.2%) | 3/48 (6.2%)*c | 1/70 (1.4%) | |
Direct cleavage | 1/47 (2.1%) | – | – | |
2-cells stage—Day 2 | 15/19 (78.9%) | 21/24 (87.5%) | 47/59 (79.6%) | |
4–8 cells stage—Day 3 | 7/19 (36.8%)*d | 14/24 (58.3%) | 42/59 (71.1%) | |
Blastocyst stage—Day 5 | –*e | 1/24 (4.1%)*f | 15/59 (25.4%) | |
Blastocyst stage—Day 6 | 1/19 (5.2%) | 1/24 (4.1%) | – |
. | OTO-Cold . | OTO-Warm . | ICSI-Control . | |
---|---|---|---|---|
Injected oocytes | 47 | 48 | 70 | |
Normally fertilized (2PN)—Day 1 | 19/47 (40.4%)*a | 24/48 (50.0%)*b | 59/70 (84.2%) | |
Abnormally fertilized | 1PN | – | 2/48 (4.1%) | – |
3PN | 2/47 (4.2%) | 3/48 (6.2%)*c | 1/70 (1.4%) | |
Direct cleavage | 1/47 (2.1%) | – | – | |
2-cells stage—Day 2 | 15/19 (78.9%) | 21/24 (87.5%) | 47/59 (79.6%) | |
4–8 cells stage—Day 3 | 7/19 (36.8%)*d | 14/24 (58.3%) | 42/59 (71.1%) | |
Blastocyst stage—Day 5 | –*e | 1/24 (4.1%)*f | 15/59 (25.4%) | |
Blastocyst stage—Day 6 | 1/19 (5.2%) | 1/24 (4.1%) | – |
OTO, ovarian tissue oocytes collected (in cold or warm medium) from transgender men and matured in vitro; control oocytes, immature oocytes or mature oocytes (with aggregates of smooth endoplasmic reticulum) collected from ovarian stimulated women undergoing IVF; PN, pronuclei.
Fertilization was performed using ICSI with a single sperm cell. Blastocyst rates were calculated based on the number of normally fertilized zygotes. Percentages were compared using the chi-square test and P < 0.05 was considered significant. Asterisks represent a P value <0.05 between respective groups and ICSI-Control oocytes.
P < 0.001.
P < 0.001.
P = 0.029.
P = 0.007.
P = 0.014.
P = 0.026.
In the Control group, 47/59 (79.6%) of the normally fertilized oocytes (zygotes) cleaved to two cells. This rate was similar (P = 0.947) in the OTO-Cold 15/19 (78.9%) and OTO-Warm groups (P = 0.400), where 21/24 (87.5%) of normally fertilized zygotes cleaved to two cells (Table II). The 2 cell rate was also not significantly different between the two groups of oocytes originating from transgender men (P = 0.451). On Day 3, 42/59 (71.1%) embryos progressed to the 4–8 cell state in the Control group, whilst only 7/19 (36.8%) and 14/24 (58.3%) progressed in the OTO-Cold (P = 0.007) and OTO-Warm group (P = 0.257), respectively (Table II). However, no statistically significant difference was observed between the two groups OTO-IVM oocytes of transgender men (P = 0.161). Blastocyst development was severely compromised in the OTO-IVM oocytes. In the OTO-Cold condition, no blastocysts were obtained on Day 5, but one blastocyst (1/19, 5.2%) was formed on Day 6. The Day 5 blastocyst rate was significantly lower (P = 0.014) when compared to Control oocytes, as there were 15/59 (25.4%) Control blastocysts formed by Day 5 (Table II). In the OTO-Warm group, 1/24 (4.1%) embryos developed to the blastocyst stage, which was also significantly lower than the Control group (P = 0.026), but similar to that for the OTO-Cold oocytes (P = 0.368) (Table II). One additional blastocyst was formed in the OTO-Warm group on Day 6 (Table II). The quality of the generated embryos can be found in Supplementary Table SIII and is based on Supplementary Table SIV.
Spindle transfer rescues blastocyst development of OTO-IVM oocytes
As poor embryonic development was observed both in OTO-Cold and OTO-Warm derived embryos, we aimed to investigate whether an inferior cytoplasmic quality was the reason for the poor developmental capacity. Since no prominent difference was noticed between development and calcium releasing ability in OTO-Cold and OTO-Warm oocytes, we decided to collect the next ovaries only on ice. In total, seven more patients were recruited for ST experiments. Fifteen ovaries from patients 7, 10, 11, 12, 13, 14, 15, and 16 were collected in cold medium and COCs were retrieved as mentioned above. We recovered 416 COCs from the 15 ovaries, with an average of 27.7 COCs per ovary (min 8, max 61). Following maturation, 349/416 oocytes survived (83.8%) and 210/349 (60.1%) matured. Only mature oocytes were used for ST. Oocytes were distributed in different ST groups. In the ST-OTO group, control oocytes were used as cytoplasmic recipients and OTO-Cold oocytes were used as nuclear donors (Fig. 4A). The spindle of OTO-Cold oocytes (Fig. 4B) was transferred to control oocytes following enucleation (Fig. 4C). Reconstructed oocytes (Fig. 4D) were fertilized with ICSI, and embryo development was monitored. In the ST-Control group, fresh Control oocytes were used as cytoplasmic recipients and Control oocytes were used as spindle donors (Fig. 4E). This group served as the control for the ST technique.
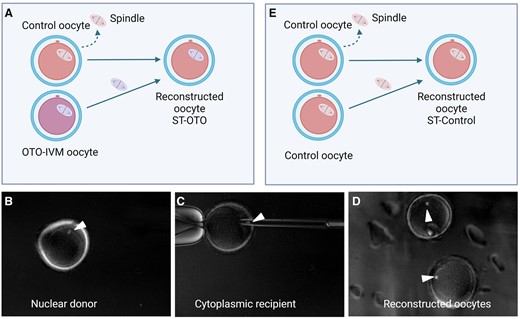
Representation of spindle transfer (ST). (A) ST-OTO group: the spindle of OTO-IVM oocytes from transgender men is transferred into enucleated Control oocytes. (B) Visible spindle of a nuclear donor (OTO-IVM) oocyte right before enucleation. (C) Transfer of an OTO-IVM spindle in an enucleated Control oocyte, serving as the cytoplasmic recipient. (D) Reconstructed oocytes. (E) ST-control group: the spindle of Control oocytes is transferred into enucleated Control oocytes. White arrows in (B), (C), and (D) indicate the visible spindle. OTO-IVM, in vitro matured ovarian tissue oocytes.
Following ICSI, fertilization was significantly lower in the ST-OTO (29/46 (63%)) and ST-Control (24/39 (61.5%)) groups when compared to ICSI-Control oocytes (59/70 (84.2%)) (P = 0.009 and P = 0.008, respectively) (Table III). Furthermore, more abnormally fertilized oocytes were observed in the ST-Control group (8/39 (20.5%)) compared to ICSI-Controls (1/70 (1.4%)) (P < 0.01). On Day 5, no important differences could be noted on the number of generated blastocysts between ST-OTO (6/29 (20.6%)), ST-Control (7/24 (29.1%)), and ICSI-Control (15/59 (25.4%)) oocytes (Table III). When compared to OTO-Cold oocytes, ST-OTO significantly improved the fertilization rate from 40.4% to 63.0% (Tables II, III, and IV). Embryonic development did not differ significantly between the two groups for Day 2 and Day 3 but blastocyst development was restored following ST from 0% on Day 5 to 20.6% (P = 0.034) (Table IV). However, the quality of ST-OTO embryos on Day 5 was poor (Supplementary Table SIII).
. | ST-OTO . | ST-Control . | ICSI-Control . | |
---|---|---|---|---|
Injected oocytes | 46 | 39 | 70 | |
Normally fertilized (2PN)—Day 1 | 29/46 (63%)*a | 24/39 (61.5%)*b | 59/70 (84.2%) | |
Abnormally fertilized | 3PN | 1/46 (2.1%) | 4/39 (10.2%) | 1/70 (1.4%) |
4PN | – | 1/39 (2.5%)*c | – | |
Direct cleavage | 3/46 (6.5%) | 3/39 (7.6%) | – | |
2-cells stage—Day 2 | 19/29 (65.5%) | 17/24 (70.8%) | 47/59 (79.6%) | |
4–8 cells stage—Day 3 | 13/29 (44.8%)*d | 16/24 (66.6%) | 42/59 (71.1%) | |
Blastocyst stage—Day 5 | 6/29 (20.6%) | 7/24 (29.1%) | 15/59 (25.4%) |
. | ST-OTO . | ST-Control . | ICSI-Control . | |
---|---|---|---|---|
Injected oocytes | 46 | 39 | 70 | |
Normally fertilized (2PN)—Day 1 | 29/46 (63%)*a | 24/39 (61.5%)*b | 59/70 (84.2%) | |
Abnormally fertilized | 3PN | 1/46 (2.1%) | 4/39 (10.2%) | 1/70 (1.4%) |
4PN | – | 1/39 (2.5%)*c | – | |
Direct cleavage | 3/46 (6.5%) | 3/39 (7.6%) | – | |
2-cells stage—Day 2 | 19/29 (65.5%) | 17/24 (70.8%) | 47/59 (79.6%) | |
4–8 cells stage—Day 3 | 13/29 (44.8%)*d | 16/24 (66.6%) | 42/59 (71.1%) | |
Blastocyst stage—Day 5 | 6/29 (20.6%) | 7/24 (29.1%) | 15/59 (25.4%) |
OTO, ovarian tissue oocytes collected (in cold medium) from transgender men and matured in vitro; control oocytes, immature oocytes or mature oocytes (with aggregates of smooth endoplasmic reticulum) collected from ovarian stimulated women undergoing IVF; ST, spindle transfer; PN, pronuclei.
Fertilization was performed using ICSI with a single sperm cell. Blastocyst rates were calculated based on the number of normally fertilized zygotes. Percentages were compared using the chi-square test and P < 0.05 was considered significant. Asterisks represent a P value <0.05 between ST-OTO, Control-ST, and ICSI-Control oocytes.
P = 0.009.
P = 0.008.
P < 0.01.
P = 0.016.
. | ST-OTO . | ST-Control . | ICSI-Control . | |
---|---|---|---|---|
Injected oocytes | 46 | 39 | 70 | |
Normally fertilized (2PN)—Day 1 | 29/46 (63%)*a | 24/39 (61.5%)*b | 59/70 (84.2%) | |
Abnormally fertilized | 3PN | 1/46 (2.1%) | 4/39 (10.2%) | 1/70 (1.4%) |
4PN | – | 1/39 (2.5%)*c | – | |
Direct cleavage | 3/46 (6.5%) | 3/39 (7.6%) | – | |
2-cells stage—Day 2 | 19/29 (65.5%) | 17/24 (70.8%) | 47/59 (79.6%) | |
4–8 cells stage—Day 3 | 13/29 (44.8%)*d | 16/24 (66.6%) | 42/59 (71.1%) | |
Blastocyst stage—Day 5 | 6/29 (20.6%) | 7/24 (29.1%) | 15/59 (25.4%) |
. | ST-OTO . | ST-Control . | ICSI-Control . | |
---|---|---|---|---|
Injected oocytes | 46 | 39 | 70 | |
Normally fertilized (2PN)—Day 1 | 29/46 (63%)*a | 24/39 (61.5%)*b | 59/70 (84.2%) | |
Abnormally fertilized | 3PN | 1/46 (2.1%) | 4/39 (10.2%) | 1/70 (1.4%) |
4PN | – | 1/39 (2.5%)*c | – | |
Direct cleavage | 3/46 (6.5%) | 3/39 (7.6%) | – | |
2-cells stage—Day 2 | 19/29 (65.5%) | 17/24 (70.8%) | 47/59 (79.6%) | |
4–8 cells stage—Day 3 | 13/29 (44.8%)*d | 16/24 (66.6%) | 42/59 (71.1%) | |
Blastocyst stage—Day 5 | 6/29 (20.6%) | 7/24 (29.1%) | 15/59 (25.4%) |
OTO, ovarian tissue oocytes collected (in cold medium) from transgender men and matured in vitro; control oocytes, immature oocytes or mature oocytes (with aggregates of smooth endoplasmic reticulum) collected from ovarian stimulated women undergoing IVF; ST, spindle transfer; PN, pronuclei.
Fertilization was performed using ICSI with a single sperm cell. Blastocyst rates were calculated based on the number of normally fertilized zygotes. Percentages were compared using the chi-square test and P < 0.05 was considered significant. Asterisks represent a P value <0.05 between ST-OTO, Control-ST, and ICSI-Control oocytes.
P = 0.009.
P = 0.008.
P < 0.01.
P = 0.016.
. | ST-OTO . | OTO-Cold . | |
---|---|---|---|
Injected oocytes | 46 | 47 | |
Normally fertilized (2PN)—Day 1 | 29/46 (63%) | 19/47 (40.4%)*a | |
Abnormally fertilized | 3PN | 1/46 (2.1%) | 2/47 (4.2%) |
Direct cleavage | 3/46 (6.5%) | 1/47 (2.1%) | |
2-cells stage—Day 2 | 19/29 (65.5%) | 15/19 (78.9%) | |
4–8 cells stage—Day 3 | 13/29 (44.8%) | 7/19 (36.8%) | |
Blastocyst stage—Day 5 | 6/29 (20.6%) | –*b | |
Blastocyst stage—Day 6 | – | 1/19 (5.2%) |
. | ST-OTO . | OTO-Cold . | |
---|---|---|---|
Injected oocytes | 46 | 47 | |
Normally fertilized (2PN)—Day 1 | 29/46 (63%) | 19/47 (40.4%)*a | |
Abnormally fertilized | 3PN | 1/46 (2.1%) | 2/47 (4.2%) |
Direct cleavage | 3/46 (6.5%) | 1/47 (2.1%) | |
2-cells stage—Day 2 | 19/29 (65.5%) | 15/19 (78.9%) | |
4–8 cells stage—Day 3 | 13/29 (44.8%) | 7/19 (36.8%) | |
Blastocyst stage—Day 5 | 6/29 (20.6%) | –*b | |
Blastocyst stage—Day 6 | – | 1/19 (5.2%) |
OTO, ovarian tissue oocytes collected (in cold medium) from transgender men and matured in vitro; control oocytes, immature oocytes or mature oocytes (with aggregates of smooth endoplasmic reticulum) collected from ovarian stimulated women undergoing IVF; PN, pronuclei.
Fertilization was performed using ICSI with a single sperm cell. Blastocyst rates were calculated based on the number of normally fertilized zygotes. Percentages were compared using the chi-square test and P < 0.05 was considered significant. Asterisks represent a P value <0.05 between ST-OTO and OTO-Cold oocytes.
P = 0.029.
P = 0.034.
. | ST-OTO . | OTO-Cold . | |
---|---|---|---|
Injected oocytes | 46 | 47 | |
Normally fertilized (2PN)—Day 1 | 29/46 (63%) | 19/47 (40.4%)*a | |
Abnormally fertilized | 3PN | 1/46 (2.1%) | 2/47 (4.2%) |
Direct cleavage | 3/46 (6.5%) | 1/47 (2.1%) | |
2-cells stage—Day 2 | 19/29 (65.5%) | 15/19 (78.9%) | |
4–8 cells stage—Day 3 | 13/29 (44.8%) | 7/19 (36.8%) | |
Blastocyst stage—Day 5 | 6/29 (20.6%) | –*b | |
Blastocyst stage—Day 6 | – | 1/19 (5.2%) |
. | ST-OTO . | OTO-Cold . | |
---|---|---|---|
Injected oocytes | 46 | 47 | |
Normally fertilized (2PN)—Day 1 | 29/46 (63%) | 19/47 (40.4%)*a | |
Abnormally fertilized | 3PN | 1/46 (2.1%) | 2/47 (4.2%) |
Direct cleavage | 3/46 (6.5%) | 1/47 (2.1%) | |
2-cells stage—Day 2 | 19/29 (65.5%) | 15/19 (78.9%) | |
4–8 cells stage—Day 3 | 13/29 (44.8%) | 7/19 (36.8%) | |
Blastocyst stage—Day 5 | 6/29 (20.6%) | –*b | |
Blastocyst stage—Day 6 | – | 1/19 (5.2%) |
OTO, ovarian tissue oocytes collected (in cold medium) from transgender men and matured in vitro; control oocytes, immature oocytes or mature oocytes (with aggregates of smooth endoplasmic reticulum) collected from ovarian stimulated women undergoing IVF; PN, pronuclei.
Fertilization was performed using ICSI with a single sperm cell. Blastocyst rates were calculated based on the number of normally fertilized zygotes. Percentages were compared using the chi-square test and P < 0.05 was considered significant. Asterisks represent a P value <0.05 between ST-OTO and OTO-Cold oocytes.
P = 0.029.
P = 0.034.
To prove the cytoplasmic deficiency of ST-OTO oocytes, we subsequently performed ST using enucleated fresh OTO-Cold oocytes as cytoplasmic recipients and Control oocytes as nuclear donors (OTO-ST group) (Supplementary Fig. S1A). For the ease of our experiments, we also investigated the use of vitrified-thawed (V-T) Control oocytes (ST-OTO-V-T) as cytoplasmic recipients for the spindle of OTO-Cold oocytes (Supplementary Fig. S1B). Since embryo development was poor in both groups, this approach was not investigated further. The embryo development can be found in Supplementary Table SV.
Poor embryo development of OTO-IVM oocytes from transgender men is probably not caused by genetic abnormalities
To elucidate whether embryo development was compromised due to chromosomal abnormalities, we analysed the derived embryos for CNVs. Embryos were analysed at the blastocyst stage or on Day 3 of development. Following analysis, we detected embryos with normal genetic profiles (Fig. 5A), embryos with duplications (Fig. 5B) and deletions (Fig. 5C), and embryos with an inconclusive profile (Fig. 5D). In total, 17 embryos from the ICSI-Control, 11 from OTO-Cold, and 11 from OTO-Warm groups were analysed for CNVs. In the ICSI-Control group, 11/17 (64.7%) embryos were euploid, 3/17 (17.6%) embryos exhibited chromosomal deletions and 3/17 (17.6%) duplications.
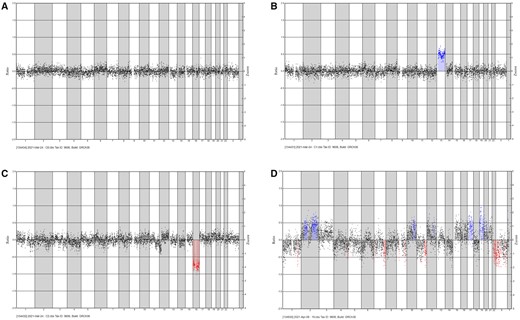
Representative genetic profiles of embryos. Blue indicates duplications and red indicates deletions. (A) An embryo with a normal genetic profile. (B) Embryo with a duplication (trisomy for chromosome 13). (C) Embryo with a deletion (monosomy for chromosome 16). (D) Embryo with an inconclusive genetic profile.
A similar pattern was observed in embryos derived from OTO-IVM oocytes from transgender men. In the OTO-Cold group, 8/11 (72.7%) embryos had a normal chromosomal profile, 2/11 (18.8%) embryos had chromosomal deletions, and 1/11 (9%) embryo had an inconclusive CNV profile. In the OTO-Warm group, 8/11 (72.7%) analysed embryos were normal, whilst 3/11 (27.2%) embryos showed chromosomal deletions.
In total, seven embryos were analysed in the ST-Control group and 17, in the ST-OTO group. Of the ST-Control embryos, 4/7 (57.1%) appeared chromosomally normal, whilst 3/7 (42.9%) exhibited chromosomal deletions. In the ST-OTO group, no CNVs were detected in 7/17 (41.1%) embryos. Deletions and duplications were present in 9/17 (52.9%) embryos, with one embryo showing an inconclusive genetic profile.
Discussion
To our knowledge, this is the first time that ST has been performed to improve embryo development of OTO-IVM oocytes. A limited number of studies on OTO-IVM in transgender men have demonstrated the ability of these oocytes to mature (De Roo et al., 2017; De Roo and Tilleman, 2021). Following maturation, the spindle of OTO-IVM oocytes seems to be intact even after vitrification-thawing cycles (Lierman et al., 2017). Nevertheless, following ICSI, OTO-IVM oocytes of transgender men show a very low fertilization rate and severely compromised embryo development (Lierman et al., 2021), which was also confirmed in this study.
In an attempt to improve maturation rate and embryo development, we compared the quality of OTO-IVM oocytes when tissue is transported on ice or in prewarmed collection medium. The average number of oocytes collected per patient (47 COCs) and the maturation rate in our study was similar to the ones reported in other studies performing OTO-IVM for transgender men (De Roo et al., 2017; Lierman et al., 2017), whilst the maturation efficiency was similar in both OTO-Cold and OTO-Warm oocytes. The degeneration rate was 20.3% in OTO-Cold oocytes, similar to the degeneration rate reported previously (Lierman et al., 2017, 2021). Nevertheless, a higher degeneration rate (30.5%) was observed in oocytes from the OTO-Warm condition. This could possibly be attributed to the fact that collection of COCs was performed by a single operator. Normally, oocyte collection is performed by two individuals, and should be carried out within a maximum timeframe of 1 h (Nikiforov et al., 2020). As the metabolic activity of cells is higher when tissue is collected in warm collection medium, this could potentially have affected the survival rate of oocytes collected in this condition.
Since the maturation and developmental competence of oocytes is associated with the diameter of the follicle (Chian and Cao, 2014; Sánchez et al., 2015), we sought to investigate the diameter of the mature OTO-IVM oocytes, as this can be indicative for embryonic potential. While the diameter of small antral follicles in the tissue is not expected to be more than 10 mm (Yin et al., 2016), the oocyte diameter of OTO-IVM mature oocytes was similar to that detected in the Control oocytes from stimulated infertility patients, where the follicle diameter is about 18–20 mm. These diameters are comparable to in vivo matured oocytes reported in IVF cycles (Romão et al., 2010).
Calcium imaging analysis demonstrated that calcium release in OTO-IVM oocytes was significantly lower when compared to oocytes originating from infertility patients. The increase and patterns of calcium (Ca2+) oscillations are important factors for the exit of the oocyte from meiotic arrest, proper development of male and female pronuclei, recruitment of oocyte mRNAs, embryonic gene expression, and embryo development (Yoon et al., 2008; Chimote and Chimote, 2018; Zafar et al., 2021). For oocyte activation, calcium action is exerted upon gamete fusion, with the release of phospholipase C zeta (PLCζ) from the sperm (Cardona Barberán et al., 2020). PLCζ initiates a number of cascades which will further stimulate Ca2+ discharge from the endoplasmic reticulum of the oocytes, leading to long lasting Ca2+ oscillations (Sun and Yeh, 2021). As a result, both the oocyte and sperm have an important role in Ca2+ release (Yeste et al., 2016; Ferrer-Buitrago et al., 2019). Since the same sperm sample was used for all experiments, we can conclude that the reason for the reduced Ca2+ releasing patterns, is the poor quality of the OTO-IVM oocytes. Certain components in the oocyte calcium releasing machinery could be the reason for the poor oscillations (Yeste et al., 2016; Bonte et al., 2018), although these factors have not been extensively investigated. Currently, there are no reports in literature on calcium releasing ability of oocytes originating from transgender men under testosterone treatment. Nevertheless, it is known that oocytes are sensitive to steroids while still at the GV stage (Chimote and Chimote, 2018). Oestrogens promote calcium release, which seems to be related to the cytoplasmic rather than meiotic maturation. The imbalance in oestrogen/androgen production might interfere with the calcium release (Tesarik and Mendoza, 1997), as observed also in our study, taking into account the long term testosterone treatment of the patients studied. Follicles express androgen receptors in the theca cells already from the secondary stage. Testosterone has high affinity for the androgen receptor present in the follicle (Chimote and Chimote, 2018), which could possibly explain the compromised calcium profile observed. Nevertheless, taking into account that the OTO-IVM oocytes originate from small immature antral follicles, we cannot exclude that the inferior calcium release is attributed to a general low oocyte quality. To confirm this, OTO-IVM oocytes from women or from transgender men with no previous treatment should have been included, which was not possible for this study.
Even though our Control oocytes consisted mostly of IVM oocytes from the clinic, these oocytes originate from ovarian stimulated women, in contrast with their OTO-IVM counterparts. It is possible that cytoplasmic maturation is not synchronized to nuclear maturation in the OTO-IVM oocytes (Sánchez et al., 2015). Since no statistically significant difference was observed between OTO-Cold and OTO-Warm oocytes, we concluded that the cytoplasmic quality in both OTO-IVM groups was similar.
In parallel to calcium imaging, we performed ICSI to evaluate the fertilization rate and embryo developmental potential of OTO-IVM oocytes collected in both collection conditions. The fertilization rate was inferior to that observed in the Control oocytes, but still similar between OTO-Cold and OTO-Warm oocytes. These results are in line with the poor Ca+2 release observed, as a lower fertilization rate is expected when Ca+2 oscillations are not sufficient to support oocyte activation (Yeste et al., 2017). From the 47 OTO-Cold injected oocytes, no blastocysts were generated on Day 5, but one delayed blastocyst was formed on Day 6. In the OTO-Warm group, only one Day 5 and one Day 6 blastocysts were generated after the injection of 48 oocytes. These results demonstrate that embryo development is similar when ovaries are collected in warm or cold collection medium, suggesting that temperature collection of ovarian tissue has potentially no effect on embryo development. The number of observed blastocysts was significantly lower compared to Control oocytes, indicating, again, the poor quality of OTO-IVM counterparts.
Noteworthy, while ICSI was performed in fresh OTO-Cold and OTO-Warm oocytes in our study, our results are similar to the study of Lierman et al. (2021), where ICSI was performed in vitrified-thawed OTO-IVM oocytes. We cannot rule out the possibility that the poor cytoplasmic quality observed in the OTO-IVM transgender derived oocytes could be attributed to prolonged testosterone treatment. Nevertheless, the literature remains inconclusive on this regard. The so far existing studies on transgender men have not identified a correlation between the length of testosterone treatment and maturation or embryo developmental potential rate of derived OTO-IVM oocytes (De Roo et al., 2017; Lierman et al., 2017, 2021). The growing follicles, enclosing the COCs, possess androgen receptors as mentioned above (Walters and Handelsman, 2018), and it cannot be excluded that testosterone could have an effect on follicle development, similar to that noticed in patients with polycystic ovarian syndrome (Pache et al., 1991; Franks et al., 2008). This androgenic environment in the transgender ovaries could potentially affect oocyte quality, as there is a higher number of anovulatory follicles, which are released only during ovarian tissue manipulation (De Roo et al., 2017).
In our study, we did not observe a correlation between testosterone treatment length and the number of oocytes retrieved during tissue manipulation, although only a few subjects were included. A correlation between testosterone length with embryo development was not evaluated due to the two different oocyte subpopulations in OTO-Cold and OTO-Warm groups.
Results from IVF cycles of transgender patients have demonstrated that oocyte quality and embryo development in these patients are similar to that observed in for women of the same age (Leung et al., 2019; Amir et al., 2020). However, the transgender patients undergoing ovarian stimulation have previously ceased testosterone treatment for several months beforehand (De Roo et al., 2016).
Following genetic analysis for CNVs in the derived embryos from OTO-Cold, OTO-Warm, and ICSI-Control oocytes, we observed that in all three groups, almost the same percentage of euploid embryos was present (64.7–72.7%). Even though the number of analysed embryos was limited, it is evident that the CNVs appear within the expected rate in the OTO-IVM embryos. These results, together with poor embryonic development and calcium releasing ability, are suggesting cytoplasmic factors as the reason for the limited blastocyst development (Mao et al., 2014; Reader et al., 2017) in the OTO-IVM oocytes.
Nevertheless, the mitochondrial membrane potential, reflecting the quality of one of the most important organelles in the oocytes, did not seem to be compromised in the OTO-IVM oocytes. Of course, other important parameters, such as mitochondria numbers and cytoplasmic proteins in the oocytes, as well as coenzymes NAD(P)H and FAD in the derived embryos, were not investigated in this study.
To confirm the cytoplasmic inferiority of the OTO-IVM oocytes, we performed ST. NT has been considered as means to overcome poor cytoplasmic quality of oocytes causing failed fertilization (Tang et al., 2022) or embryo developmental arrest (Tang et al., 2020). The safety of the technique (including ST) has been confirmed in several animal (Tachibana et al., 2009; Neupane et al., 2014; Wang et al., 2014) and human preclinical studies (Tachibana et al., 2013; Hyslop et al., 2016; Kang et al., 2016; Ma et al., 2017). However, certain considerations, involving mainly mitochondrial carry-over and mitonuclear mismatch, still remain a matter of debate (Ma et al., 2016; Yamada et al., 2016; Greenfield et al., 2017). The application of NT was beneficial for a case study of embryo developmental arrest, with the first reported pregnancy albeit without live birth (Zhang et al., 2016), and for another case of failed fertilization following ICSI (Tang et al., 2022) in a preclinical study. Currently, the only publication on the application of ST in humans leading to a healthy offspring in order to overcome mitochondrial diseases is from Zhang et al. (2017). Apparently, clinical trials of this technique for certain types of infertility are ongoing in two centres in Greece and Ukraine, but no peer reviewed papers have been published yet (Cohen et al., 2020).
The use of Control oocytes as cytoplasmic donors for the spindles of OTO-Cold oocytes in our study, significantly improved the rate of blastocyst development. A similar blastocyst development rate was observed in ST-Control reconstructed oocytes, showing that the improved embryo development is attributed to the cytoplasm of Control oocytes. The similar blastocyst rate observed in ICSI-Control oocytes further showed that ST does not have a negative effect on the rate of embryo development. However, a higher abnormal fertilization rate was observed in the ST-Control group when compared to ICSI-Control oocytes. It is important to note that ST remains an invasive technique and the source of oocytes used in this study as Controls were not the optimal oocytes for cytoplasmic recipients, as in vitro matured oocytes originating from infertile patients, were mostly used; this is reflected by the poor quality of the generated blastocysts.
Genetic analysis of ST-Control and ST-OTO embryos demonstrated that the technique does not result in a higher rate of genetic abnormalities when compared to ICSI-Control embryos. Even though the number of embryos analysed in our study was limited, normal ploidy rates after NT technology in human embryos have been reported previously also by other studies (Hyslop et al., 2016; Zhang et al., 2016, 2017; Tang et al., 2019). We did not study the mitochondrial carry-over following ST, since our interest was on infertility. In previous studies, the average mtDNA carry-over after ST is quite minimal, reaching maximum percentages of 0.5% (Tachibana et al., 2013), 0.31% (Paull et al., 2013), to 5.7% (Zhang et al., 2017). This was also previously confirmed by our group with a maximum of 2.9% carry over (Tang et al., 2022). Nevertheless, mitochondrial DNA carry over and its possible reversion is still an important enigma in NT and should be further investigated, especially when the application focuses on overcoming mitochondrial diseases.
The most prominent outcome of this study is certainly that cytoplasmic factors are the possible reason for the poor embryo development observed in OTO-IVM oocytes from transgender men. Cytoplasmic and nuclear maturation are not always synchronized. Cytoplasmic factors, including mitochondria numbers, mitochondrial membrane potential, maternal proteins, and mRNAs, are important for embryo development (Mao et al., 2014; Coticchio et al., 2015; Reader et al., 2017). Recent studies that make use of a prematuration medium for immature oocytes originating from small follicles of patients with polycystic ovarian syndrome and for OTO-IVM in cancer patients, demonstrate an impressive increase in oocyte maturation and embryo developmental potential (Sánchez et al., 2019; Kirillova et al., 2021). This prematuration step, using physiological inhibitors of oocyte nuclear maturation, slows down nuclear maturation, in order to synchronize it with cytoplasmic maturity (Sánchez et al., 2017).
For more accurate conclusions on the effect of testosterone treatment in the quality of OTO-IVM oocytes, the transcriptome or proteome should be further studied to investigate the underlying cytoplasmic factors causing the poor blastocyst development. Nevertheless, a more suitable Control is lacking. It should be stressed that we used as Controls in vitro matured and SERa oocytes from ovarian stimulated infertility patients. In order to make more accurate comparisons, oocytes from women undergoing oophorectomy or from transgender men with no previous exposure to testosterone treatment should be used.
In the current study, we demonstrate the low quality of OTO-IVM oocytes originating from transgender men. The limited calcium releasing ability and poor embryo development point towards a cytoplasmic factor responsible for the inferior oocyte quality, for which the collection temperature of ovarian tissue did not seem to play an important role. ST was able to rescue the poor embryo development rate of OTO-IVM oocytes, further supporting the existence of a cytoplasmic inferiority but also suggesting the potential of this technology to overcome embryo developmental arrest. However, the quality of the generated blastocysts was not ideal. We believe that these results would have been more impactful if oocytes from non-infertile donors could be used as cytoplasmic recipients, to further improve the embryo development. Although technically promising, several ethical concerns surround NT, including its safety, the alternative of non-genetically related offspring, and philosophical questions regarding an individual’s identity, still demand caution in the use of the technology. Additional research on the effect of testosterone treatment duration and on the oocyte maturation protocols would be also beneficial to create valuable guidelines for the fertility preservation of transgender men.
Data availability
The data underlying this article are available in the article and in its online Supplementary Material.
Acknowledgements
We would like to thank the patients who were willing to participate in our study and also the surgical team of Ghent University Hospital for the collection of the ovarian tissue. Special thanks go to the study coordinator team for the recruitment of the patients and to the fertility clinic for the daily collection of immature and SERa oocytes. The figures were created with Biorender.com.
Authors’ roles
A.C. performed and designed the experiments, collected samples, analysed data, and wrote the manuscript. H.H., M.Z., and A.C.B. contributed to experiments. B.H., A.B., S.W., S.M.C.D.S.L., D.S., P.D.S., A.V.S., and C.D.R. designed and supervised the experiments, reviewed and edited the manuscript. B.M. and M.B. contributed to the genetic analysis and interpretation of data. All authors contributed to the analysis of data and revision of the manuscript.
Funding
A.C. is a holder of 1S80220N and 1S80222N FWO-Vlaanderen (Flemish Fund for Scientific Research) funding. A.B. is a holder of an FWO grant (1298722N). B.H. and A.V.S. have been awarded BOF (Bijzonder Onderzoeksfonds) GOA (Geconcerteerde onderzoeksacties) and 2018000504 (GOA030-18 BOF) funding. B.H. has additional grants from FWO-Vlaanderen (Flemish Fund for Scientific Research, G051516N and G1507816N) and Ghent University Special Research Fund (Bijzonder Onderzoeksfonds, BOF funding (BOF/STA/202109/005)), and has been receiving unrestricted educational funding from Ferring Pharmaceuticals (Aalst, Belgium).
Conflict of interest
The authors declare that they have no conflict of interest.