-
PDF
- Split View
-
Views
-
Cite
Cite
Oren Kashi, Hadassa Roness, Itai Spector, Sanaz Derech-Haim, Dror Meirow, Dual suppression of follicle activation pathways completely prevents the cyclophosphamide-induced loss of ovarian reserve, Human Reproduction, Volume 38, Issue 6, June 2023, Pages 1086–1098, https://doi.org/10.1093/humrep/dead064
- Share Icon Share
Abstract
To what extent and how does combined administration of the follicle activation pathway suppressive agents temsirolimus (Tem) and c-terminus recombinant anti-Müllerian hormone (rAMH) protect against chemotherapy-induced ovarian reserve loss?
Combined administration of Tem and rAMH completely prevents cyclophosphamide (Cy)-induced follicle depletion and protects the ovarian reserve in mice, primarily via primordial follicle (PMF) suppression of activation and to a lesser degree by reducing apoptosis.
There is conflicting evidence regarding the contributory roles of apoptosis and follicle activation in chemotherapy-induced PMF loss. Tem, a mammalian target of rapamycin (mTOR) inhibitor, reduces activity of the phosphoinositide 3-kinases–phosphatase and tensin homolog (PI3K-PTEN) pathway which provides intrinsic regulation of PMF activation. Anti-Müllerian hormone (AMH), secreted by early growing follicles, is an extrinsic regulator of PMF activation.
Whole ovaries of 12-day-old mice were cultured ex vivo for 7 days in the presence of Cy ± rAMH or Tem. Eight-week-old mice were randomized into eight treatment groups: vehicle control/rAMH/Tem/Cy/Tem + rAMH/Cy + Tem/Cy + rAMH/Cy + Tem + rAMH. Twelve hours after treatment, ovaries were removed for DNA damage analysis, and 24 h after treatment either for analysis of PI3K pathway proteins or to be fixed and immunostained for analyses of proliferation and apoptosis. Three or 21 days following treatment, ovaries were fixed and sectioned for follicle counting.
Hematoxylin and eosin staining was used for differential follicle counts of primordial, primary, and secondary follicles in ex vivo (n = 16–18 ovaries per group) and in vivo ovaries (n = 8 mice per group). Histological analyses were carried out to measure proliferation by quantifying Ki-67-positive granulosa cells in primary follicles (n = 4 mice per group). DNA damage and apoptosis were measured by quantification of phosphorylated form of histone 2AX (γH2AX) and cleaved poly (ADP-ribose) polymerase (cPARP)-positive PMF oocytes, respectively (n = 8 mice per group). Protein extracts from whole ovaries were analyzed by western blotting.
In vivo experiments show that treatment with Cy alone caused significant loss of PMF reserve (32 ± 2.12 versus 144 ± 2.8 in control, P < 0.001), and this was significantly attenuated by treatment with either Tem (P < 0.001) or rAMH (P < 0.001). Combined cotreatment with Cy + Tem + rAMH provided complete protection of the PMF reserve, with no significant difference in numbers of PMF versus untreated animals. Similar results were demonstrated in the ex vivo experiments. Proliferation marker Ki-67 staining was significantly reduced in granulosa cells of primary follicles in the Cy + Tem + rAMH group compared with Cy alone group (after 24 h in vivo administration of Cy, 16% versus 65%, respectively; P < 0.001). Protein analysis demonstrated not significant increased phosphorylation of follicle activation proteins rpS6 and mTOR with in vivo administration of Cy alone (1.9 and 1.4 times the control ovaries, respectively), and this was reduced to below control levels in the Cy + Tem + rAMH group (P < 0.01). The Cy + Tem + rAMH combined cotreatment protected the follicle reservoir via inhibition of Cy-induced upregulation of the PI3K signaling pathway, together with replacement of AMH suppression of PMF activation with rAMH, implying a complementary effect of the two inhibitors. The DNA damage marker γH2AX was highly positive in PMF oocytes from Cy-treated ovaries 12 h after treatment, compared with controls (94% versus 59%, respectively, P < 0.001) and was significantly reduced to (69%) in Cy + Tem + rAMH cotreated ovaries (P < 0.001). However, only 22% of PMF oocytes of the Cy group showed apoptosis at 24 h, and this was significantly reduced (12%) in ovaries after treatment with Cy + Tem + rAMH (P < 0.01). This suggests that it is not possible to equate DNA damage with oocyte death, and also indicates that less than one-third of the total PMF loss can be attributed to apoptosis, implying that most of the PMF depletion results from PMF activation but that both mechanisms play a significant role.
N/A.
The experimental design was limited by the selection of one time point for analysis of PMF activation and apoptosis (i.e. 24 h after Cy administration), although DNA damage was measured at 12 h after Cy administration and any impact on short-term follicle dynamics at 3 days after treatment. Protein analysis was conducted on whole ovary lysates therefore the protein changes identified cannot be localized to specific cells within the ovary. However, this complementary assay showed that there was activation in the ovary through massive reduction in the phosphorylation of key proteins in the PI3K cascade (rpS6 and mTOR), which is consistent with the sequence of events after Cy administration.
Understanding the complementary nature of different follicle activation pathways and the impact of their suppression in prevention of chemotherapy-induced ovotoxic damage, as well as their involvement in DNA damage inhibition, provides an interesting direction for future research, and the potential for noninvasive pharmacological fertility preservation.
This work was supported by a grant from the Morris Kahn Foundation. The authors declare no conflicts of interest.
Introduction
Premature ovarian insufficiency (POI) is a major side effect of several types of gonadotoxic chemotherapies and is of significant concern to young cancer survivors. POI is caused by chemotherapy-induced depletion of the primordial follicles (PMFs), which constitute the ovarian reserve (Meirow et al., 1999; Jayasinghe et al., 2018). It has been suggested that this loss results either from direct induction of DNA damage and subsequent apoptosis (Soleimani et al., 2011; Winship et al., 2018; Titus et al., 2021), or indirectly via over-recruitment of the dormant PMFs into the pool of growing follicles, leading to diminished follicular reserve (Kalich-Philosoph et al., 2013; Chang et al., 2015; Jang et al., 2016; Spears et al., 2019).
While chemotherapy-induced apoptosis in granulosa cells of growing follicles has been unequivocally demonstrated (Gonfloni et al., 2009; Kalich-Philosoph et al., 2013; Kim et al., 2013), there is a debate about the role of apoptosis in PMFs. While some in vivo mouse model studies have shown evidence of apoptosis in the PMF population following cyclophosphamide (Cy) (Bellusci et al., 2019; Luan et al., 2019; Nguyen et al., 2019; Titus et al., 2021), other studies failed to show evidence of apoptosis (Kalich-Philosoph et al., 2013; Goldman et al., 2017; Zhou et al., 2017; Pascuali et al., 2018).
Aside from inducing apoptosis, it has also been proposed that chemotherapy dysregulates the factors which act to maintain dormancy of the PMFs, triggering over activation and growth, followed by death of the PMF population. PMF activation is regulated by growth factors, cytokines, and transcription factors and is controlled by interactions between several signaling pathways (Adhikari and Liu, 2009; McLaughlin and McIver, 2009). A major pathway controlling dormancy, which is intrinsic to the oocyte and granulosa cells of the PMF, is the phosphatidylinositol 3-kinase (PI3K) pathway, in which key upstream molecules PI3K and protein kinase B (AKT) control the phosphorylation of principal modulators Forehead Box O3 (FOXO3a) and Mammalian target of rapamycin (mTOR, Castrillon et al., 2003; John et al., 2008; Adhikari and Liu, 2009; Reddy et al., 2009). Follicle activation is also regulated by factors extrinsic to the PMF, including paracrine factors, such as anti-Müllerian hormone (AMH, Durlinger et al., 1999; Adhikari and Liu, 2009; Kawamura et al., 2013), as well as physical properties of the microenvironment such as increased pressure (Nagamatsu et al., 2019) and PMF clustering (Hovatta et al., 1999).
Studies have shown that gonadotoxic chemotherapy impacts both the intrinsic and extrinsic pathways of PMF activation. In mice, the ovotoxic agents Cy and cisplatin increased phosphorylation of Akt, mTOR, and FOXO3a (Kalich-Philosoph et al., 2013; Chang et al., 2015; Jang et al., 2016) triggering excessive follicle activation and loss of PMFs, resulting in decreased fertility (Kalich-Philosoph et al., 2013; Goldman et al., 2017). Administration of mTOR inhibitors (rapamycin or everolimus) has prevented Cy-induced upregulation of the PI3K pathway, partially attenuated PMF loss, and improved fertility outcomes (Goldman et al., 2017; Tanaka et al., 2018; Sato and Kawamura, 2020). In a similar manner, administration of the suppressive agent AMH reduced follicle activation and ovarian reserve depletion and improved fertility in mouse models with Cy (Kano et al., 2017; Roness et al., 2019; Sonigo et al., 2019).
It is not clear whether these pathways interact and impact on one another, or whether they are independent of each other. It is further unclear whether they are involved in PMF apoptotic pathways. The aims of this study are to investigate the effects in mice of a combination of both intrinsic and extrinsic activation pathway inhibitors [rapamycin derivative, temsirolimus (Tem), and recombinant human AMH (rAMH)] to explore whether this protection is achieved through inhibition of over activation, attenuation of apoptosis, or a combination of both mechanisms.
Materials and methods
In vivo experiments
All mice (Envigo, Israel) were housed under special pathogen free conditions, with a 12-h light/dark cycle and free access to an autoclaved pelleted diet and water.
Eight-week-old BALB/c female mice were used for the in vivo experiments (Fig. 1).
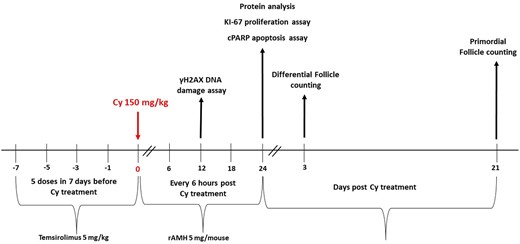
In vivo experimental outline. Female 8-week-old BALB/c mice were randomly allocated to one of four treatment groups (n = 8 mice per treatment group): mice were given a single intraperitoneal injection of 200 µl of PBS; mice received Temsirolimus (Tem) alone (5 mg/kg, 5 doses in 7 days before Cyclophosphamide (Cy) treatment); mice received a single intraperitoneal injection of Cy (150 mg/kg); mice received Tem (5 mg/kg, 5 doses in 7 days before Cy treatment) followed by a single intraperitoneal injection of Cy (150 mg/kg). In the second unit of in vivo experiments (n = 8 mice per treatment group), mice received recombinant anti-Müllerian hormone (rAMH) as well (5 µg/mouse, 5 doses in 24 h—every 6 h following the administration of Cy), in addition to Tem, Cy, both Tem and Cy, or alone. Four of the mice were killed 24 h after Cy treatment and their ovaries removed and either snap frozen in liquid nitrogen for protein analysis or immersed in 4% paraformaldehyde (PFA) for cytohistological analysis. The remaining mice were killed 3 or 21 days after Cy treatment, and their ovaries removed, immersed in 4% PFA and processed for follicle counting hematoxylin and eosin staining.
In the first line of in vivo experiments, we performed follicle counting 3 and 21 days following Cy (Sigma-Aldrich, Israel) administration, when Tem (Wyeth-Ayerst, Philadelphia, PA, USA) was the only inhibitor used. Mice were randomly allocated to one of four treatment groups (n = 8 mice per treatment group, 32 mice in total): Control—a single i.p injection of 200 µl PBS; Tem alone—5 mg/kg, 5 doses of Tem in 7 days before Cy treatment; Cy—a single i.p injection of Cy (150 mg/kg); and Tem + Cy—5 doses of Tem in 7 days before Cy treatment followed by a single i.p injection of Cy (150 mg/kg). Three of the mice were killed by cervical dislocation 3 days after Cy treatment and five 21 days after Cy treatment, and their ovaries removed, immersed in 4% paraformaldehyde (PFA) and processed for follicle counting by hematoxylin and eosin (H&E) staining.
In the second line of in vivo experiments, we investigated PMF numbers 21 days following Cy administration when two inhibitors were examined—Tem and rAMH. Mice were randomly allocated to one of six treatment groups (n = 8 mice per treatment group, 48 mice in total): Control—Single i.p injection of 200 µl of PBS; Tem + rAMH: 5 doses of Tem in 7 days before Cy treatment and 5 µg/mouse rAMH (R&D Systems, USA), 5 doses in 24 h—every 6 h following the administration of Cy; Cy: single i.p injection of Cy (150 mg/kg); Tem + Cy: 5 doses of Tem in 7 days before Cy treatment followed by a single i.p injection of Cy; rAMH + Cy: 5 doses of rAMH in 24 h—every 6 h after a single i.p injection of Cy; Tem + rAMH + Cy: 5 doses of Tem in 7 days before Cy treatment followed by a single i.p injection of Cy, followed by 5 doses of rAMH in 24 h. All mice were killed 21 days after Cy treatment, and their ovaries removed, immersed in 4% PFA and processed for follicle counting (H&E staining).
In the third line of experiments, we carried out protein analysis and assessed cell proliferation (Ki-67 assay) 24 h after Cy treatment, when the two inhibitors were examined. Mice were randomly allocated to one of eight treatment groups (n = 4 mice per treatment group, 32 mice in total): Control—single i.p injection of 200 µl of PBS; Tem alone: 5 mg/kg, 5 doses of Tem in 7 days before Cy treatment; rAMH alone: 5 doses of rAMH in 24 h—every 6 h after a single i.p injection of Cy; Cy: single i.p injection of Cy (150 mg/kg); Tem + Cy; rAMH + Cy; Tem + rAMH; Tem + rAMH + Cy. Ovaries were collected 24 h after Cy treatment. All mice were sacrificed 24 h after Cy treatment, and their ovaries were removed. Four ovaries (one from each mouse) were snap-frozen in liquid nitrogen for protein analysis, while the other four ovaries were immersed in 4% PFA and processed for Ki-67 histochemical staining.
In the fourth line of experiments, DNA damage and cell death assays were performed 12 and 24 h after Cy treatment, when the two inhibitors were examined. Mice were randomly allocated to one of five treatment groups (n = 8 mice per treatment group, 40 mice in total): Control—single i.p injection of 200 µl of PBS; Cy: single i.p injection of Cy (150 mg/kg); Tem + Cy; rAMH + Cy; Tem + rAMH + Cy. Four mice were sacrificed 12 h after Cy treatment, and their ovaries were removed, immersed in 4% PFA and processed for phosphorylated form of histone 2AX (γH2AX) histochemical staining. The other four mice were sacrificed 24 h after Cy treatment, and their ovaries were removed, immersed in 4% PFA and processed for cleaved poly (ADP-ribose) polymerase (cPARP) histochemical staining.
All procedures were approved by the Institutional Animal Care and Use Institutional Ethics Committee.
Ex vivo experiments
Twenty-four 12-day-old BALB/c mice were sacrificed, and their ovaries were removed and transferred to Transwell culture dishes (6 well plates, 16–18 ovaries per well) with media alone [DMEM F12 media (Biological Industries) containing 1 mg/ml bovine serum albumin (Sigma-Aldrich, Israel), 1 mg/ml Albumax (Gibco), 50 μg/ml Ascorbic acid (Sigma-Aldrich, Israel), 10 ng/ml Insulin (Sigma-Aldrich, Israel), 0.1 mg/ml l-Glutamine (Biological Industries), 0.1 mg/ml pen-strep (Biological Industries), and 2.5 μg/ml transferrin (Sigma-Aldrich, Israel)] or media with 400 nM Tem or 100 ng/ml rAMH. Dishes containing ovaries were maintained at 37°C in humidified air with 5% CO2. After 2 h in culture, 12 ovaries were randomly allocated to one of four treatment groups: media alone; Cy metabolite, Phosphoramide Mustard CS (PM 69945, NCI Open Chemicals respiratory, NIH), 20 µM was added to ovaries in media for 4 h, then PM was removed, and ovaries were recultured in media alone; ovaries in media plus Tem or rAMH alone; PM was added to ovaries in media plus Tem or rAMH for 4 h, then PM was removed, and ovaries were recultured in media plus Tem or rAMH. Seven days after PM was added, ovaries from each group were fixed in PFA for follicle counting (H&E). The culture medium in all groups was changed daily.
Histology
Ovaries removed 12 h, 24 h, 3 days, or 21 days following in vivo Cy treatment, were fixed in PFA, paraffin-embedded and serially sectioned (5 µm).
Follicle counting
H&E staining was used for differential follicle counts in ovaries removed 3 and 21 days following Cy treatment, and carried out as previously described (Pedersen and Peters, 1968). Briefly, blind follicle counts were conducted on every tenth section of whole ovaries. Follicle stage was classified according to accepted definitions: a PMF was counted when the nucleus was identified surrounded by a single layer of flattened squamous pre-granulosa cells; a primary follicle was defined as an oocyte surrounded by a single layer of cuboidal granulosa cells; a secondary follicle had two or more layers of cuboidal granulosa cells but no antrum; and antral follicles had an antrum and were counted only if the oocyte was visible to avoid double counting. Follicles were classified as: primordial, primary, or secondary plus (i.e. secondary and antral) follicles, and these were also grouped into dormant (primordial) or growing (primary, secondary, and antral) follicles. Atretic follicles were not calculated. Only follicles with nonatretic and healthy granulosa cells were counted for total number.
Proliferation assay
Sample sections (5 µ) from ovaries removed 24 h after Cy treatment were stained to analyze proliferation using Ki-67 antibody (Cat No: 275R-14, Cell marque). At this time interval, the effect of treatment is mainly observed on primary follicles, which were counted and categorized as follows: no proliferation—no Ki-67 staining in granulosa cells. Mild proliferation: one to two granulosa cells per follicle stained for Ki-67. Extensive proliferation: three or more granulosa cells per follicle stained for Ki-67. In addition, the proportion of stained granulosa cells out of all granulosa cells in primary follicles was calculated. All PMF in two to three sections per ovary and per group were counted.
DNA damage assay
Sample sections (5 µ) from ovaries removed 12 h after Cy treatment were stained to assess DNA damage using an γH2AX antibody (Cat# 80312s, Cell Signaling), and all the PMF oocytes were classified as positive/negative for DNA damage. Oocytes were counted from six to eight ovaries and the ratio of stained oocytes to total oocytes was calculated.
Apoptosis assay
Sample sections (5 µ) from ovaries removed 24 h after Cy treatment were stained using an apoptotic marker cPARP antibody (Cat# ab32064, Abcam), and all the PMF oocytes were classified as positive/negative for apoptosis. Oocytes were counted from six to eight ovaries and the ratio of stained oocytes to total oocytes was calculated.
Western blotting
For protein analysis, ovaries were snap frozen in liquid nitrogen and stored at −80°. Ovaries were homogenized with 60 µl RIPA buffer (Sigma-Aldrich, Israel) with protease/phosphatase inhibitors (Roche, Switzerland) and centrifuged at 20 000 g for 20 min. Protein concentration was determined using a bicinchoninic acid assay, and 40 µg of protein were loaded onto 10% sodium dodecyl sulphate–polyacrylamide gels. After transferring proteins to nitrocellulose membranes, blots were probed with appropriate antibodies—Akt (C67E7) [rabbit monoclonal antibody (mAb) Cat# 4691], phospho-Akt (Ser473) (D9E) (XP rabbit mAb Cat# 4060), rpS6 (5G10) (rabbit mAb 2217), phospho-rpS6 (Ser235/236) (antibody 2211), mTOR (7C10) (rabbit mAb 2983), phospho-mTOR (Ser2448) (antibody 2971), phospho-FOXO3a (Thr32) (antibody 9464) (all from Cell Signaling), and vinculin or anti-mouse β-Actin (Sigma-Aldrich). Integrated light intensity of each band was quantified with Image Lab 6.0.1 software (Bio-Rad) and used to compare treatment-induced changes in the concentration of phosphoproteins and to calculate the ratio of phosphorylated proteins to their nonphosphorylated forms and housekeeping proteins. Vinculin or β-actin was used to normalize the intensity values of the phosphoproteins.
Statistical analysis
A mixed model for repeated measurements (Verbeke and Molenberghs, 2000) was used to compare the different treatment groups. Comparisons were calculated using the mixed procedure in SAS (version 9.1.3; SAS Institute, Inc., Cary, NC, USA) and were adjusted for multiple comparisons using the Tukey–Kramer test, Kruskal–Wallis test, and Dunns multiple comparison. Data were expressed as the mean ± SEM. Significance levels were: *P < 0.05; **P < 0.01; and ***P < 0.001.
Results
Tem or rAMH alone attenuate follicle loss in ex vivo culture and in vivo
For initial testing of the functional ability of Tem or rAMH to prevent Cy-induced follicle loss, we used an ex vivo model of chemotherapy-induced follicle activation, culturing neonatal mouse ovaries in the presence of PM, with or without the addition of Tem or rAMH. Ovaries cultured with PM alone had significantly fewer PMFs on Day 7 of culture compared to those in control media; 75 ± 5.1 compared to 244 ± 17.8, respectively (Fig. 2A, P < 0.001). This follicle loss was attenuated in ovaries cocultured with Tem or with rAMH. Ovaries cocultured with Tem had significantly more PMFs on Day 7 of culture compared to PM alone; 124 ± 5.9 versus 75 ± 5.1, respectively (Fig. 2A, P < 0.05), as did those cocultured with rAMH (113 ± 4.6 compared to 86 ± 8.4, respectively (Fig. 2B, P < 0.01)).
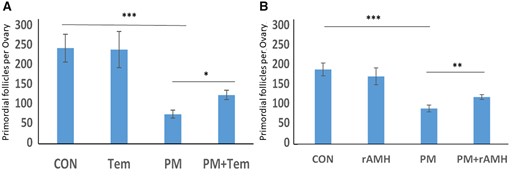
Temsirolimus and recombinant AMH ex vivo primordial follicle counting. Ovaries from 12-day-old mice were placed at an ex vivo system for 7 days after treatment with phosphoramide mustard (PM, 20 ng/ml) or the equivalent volume of media with or without cotreatment with (A) Temsirolimus (Tem) or (B) recombinant anti-Müllerian hormone (rAMH), and then were stained with hematoxylin and eosin for primordial follicle count. *P < 0.05 **P < 0.01, ***P < 0.001. Data were expressed as the mean ± SEM. Statistical analysis was performed by the mixed procedure followed by Tukey’s multiple comparison test. CON: control.
We then examined the effect of cotreatment with Tem on PMF depletion induced by Cy in an in vivo mouse model. Ovaries were removed 3 days after treatment with Cy, with or without cotreatment with Tem, to examine short-term changes in follicle dynamics, and at 21 days following exposure to examine long-term impact on the follicle reserve. Total follicle counts at 21 days showed that 150 mg/kg Cy caused a significant reduction in PMF reserve (67.8 ± 2.5 compared to 183 ± 3.32 in untreated ovaries (Fig. 3A, P < 0.001)). Ovaries from mice which received cotreatment with Cy and Tem retained a significantly higher number of PMFs compared to those treated with Cy alone, 122.5 ± 1.7 (Fig. 3A, P < 0.001).
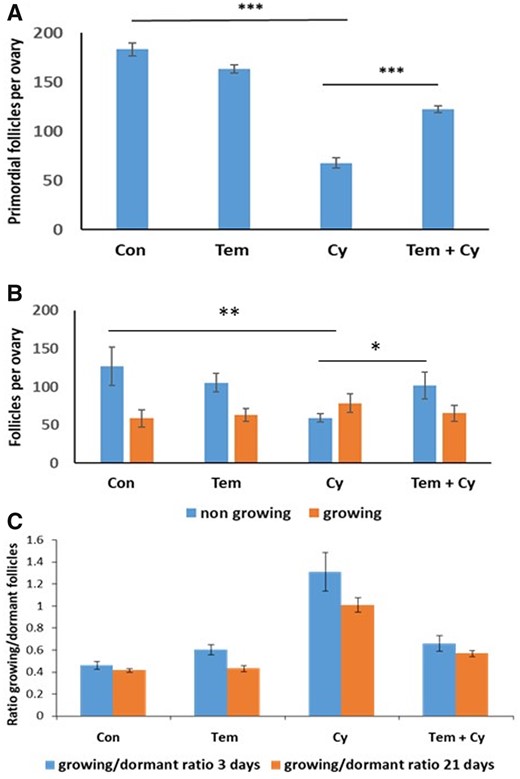
Temsirolimus in vivo counting. Ovaries from 8-week-old mice that were removed 3 or 21 days after a single dose of Cyclophosphamide (Cy, 150 mg/kg) or the equivalent volume of PBS with or without cotreatment with Temsirolimus (Tem) and were stained with hematoxylin and eosin for follicle count. (A) Primordial follicle counting 21 days after Cy treatment: 5–10 ovaries were counted for follicles per group. Statistical analysis was performed by the mixed procedure followed by Tukey’s multiple comparison test. (B) Growing (primary + secondary) and dormant (primordial) follicles counting 3 days after Cy treatment: 5–7 ovaries were counted for follicles per group. Statistical analysis was performed by the mixed procedure followed by Tukey’s multiple comparison test. (C) Comparison of the growing/dormant follicle ratio between ovaries removed at 3 and 21 days following Cy exposure. *P < 0.05, **P < 0.01, and ***P < 0.001. Data were expressed as the mean ± SEM. Statistical analysis was performed by Brown Forsythe ANOVA followed by Dunnett’s multiple comparison test. Con: control.
Follicle counts in ovaries removed 3 days after Cy treatment also showed a decrease in PMFs in Cy group compared to control and Tem cotreated ovaries, although to a lesser degree: 59.5 ± 2.7 versus 127.2 ± 12.5 and 101.6 ± 8.9, respectively (Fig. 3B, P < 0.01 and P < 0.05, respectively). This was reflected in the ratio between growing and dormant follicles, which was significantly higher in Cy-treated ovaries (1.31, Fig. 3C), compared to ovaries cotreated with Cy + Tem (0.66, P < 0.01, Fig. 3C) and untreated ovaries (0.46, P < 0.001, Fig. 3C).
Combined cotreatment with Tem and rAMH provides 100% protection against Cy-induced ovarian reserve depletion in vivo
Using the same in vivo mouse model of chemotherapy-induced ovotoxicity, we then examined whether a combination of Tem and rAMH would increase protection of the ovarian reserve from Cy-induced follicle depletion.
Three weeks after treatment, mice that received the combined treatment of Tem and rAMH with Cy had complete retention of the PMF reserve, to the extent that the number of PMFs in this group was almost identical to untreated animals (144 ± 2.8 in control versus 150.5 ± 4.9 in ovaries treated with Cy + Tem + rAMH, P < 0.001, Fig. 4). Cy cotreatment with Tem (68.5 ± 2.69 PMF, P < 0.001) or with AMH (67 ± 2.43 PMF, P < 0.001) partially decreased the extent of follicle loss to a similar degree: PMF loss of 52%, and, compared to a PMF loss of 77% in Cy-treated ovaries (32 ± 2.12, P < 0.001, Fig. 4).
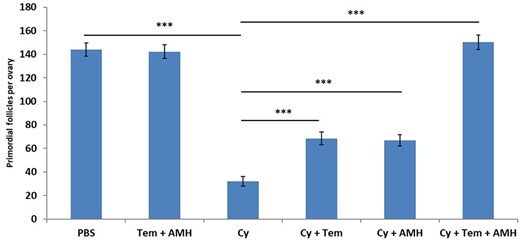
Temsirolimus and recombinant AMH in vivo primordial follicle counting. Ovaries from 8-week-old mice that were removed 21 days after a single dose of Cyclophosphamide (Cy, 150 mg/kg) or the equivalent volume of PBS with or without cotreatment with Temsirolimus (Tem) and/or recombinant anti-Müllerian hormone (AMH) and were stained with hematoxylin and eosin for follicle count: primordial follicles were counted 21 days after Cy treatment. Fourteen to 16 ovaries were counted for follicles per group, ***P < 0.001. Data were expressed as the mean ± SEM. Statistical analyses were performed by the mixed procedure followed by Tukey’s multiple comparison test.
Tem inhibits primary follicle proliferation
Assessment of primary follicle proliferation using Ki-67 staining in ovaries removed 24 h after in vivo treatment with Cy showed that 17.7% of all granulosa cells in Cy-treated ovaries were proliferating, as opposed to only 4.5% in ovaries cotreated with Tem and Cy (P < 0.0001) and 10.2% in control (P < 0.001, Fig 5B). Primary follicle average size (number of granulosa cells) was similar in all eight groups (between an average of 12.7 granulosa cells per primary follicle in Tem + AMH + Cy group and 14.1 in Cy group, P > 0.05).
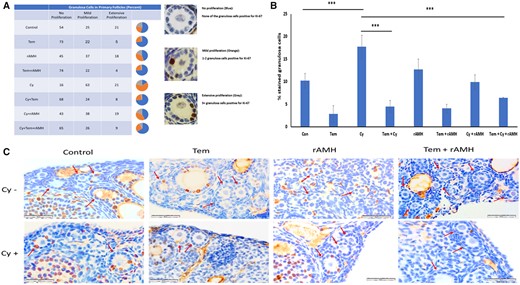
Inhibition of primary follicle proliferation by Temsirolimus and recombinant AMH. Sample mouse ovary sections were used for immunohistochemistry and stained for proliferation marker Ki-67. An analysis of ovaries from 8-week-old mice that were removed 24 h after a single dose of Cyclophosphamide (Cy, 150 mg/kg) or the equivalent volume of PBS with or without cotreatment with Temsirolimus (Tem) and/or recombinant anti-Müllerian hormone (rAMH). (A) Categorization of primary follicles according to number of stained granulosa cells (as percentage of total primary follicles per group). In the pie charts: blue—no staining in any granulosa cell; orange—1–2 granulosa cells stained; and grey—3+ granulosa cell stained. Representative images of primary follicles group types are demonstrated on the right. (B) Quantification of stained granulosa cells out of all granulosa cells in total primary follicles per group (percentage of total granulosa cells): the number of granulosa cells per follicle was similar in all groups (12.7–14.1). ***P < 0.001. Data were expressed as the mean ± SEM. Statistical analyses were performed by one-way ANOVA followed by Kruskal–Wallis test and Dunns multiple comparison. (C) Ovary sections were used for immunohistochemistry and stained for proliferation marker Ki-67. Red arrows indicate primary follicles. Sections from a minimum of three animals in each group were stained. Con: control.
When we categorized primary follicles according to their progress of proliferation, we found that in ovaries treated with Cy alone 84% of the primary follicles were classified as proliferating (either mild or extensive proliferation) (P < 0.001, Fig. 5A). Tem reduced the level of proliferation seen in the primary follicles, such that only 32% showed proliferation in ovaries cotreated with Tem and Cy (P < 0.001). In particular, treatment with Tem reduced the number of primary follicles with extensive proliferation compared to Cy-treated ovaries (8% versus 21%, respectively Fig. 5A). rAMH, however, did not reduce primary follicle proliferation. In ovaries cotreated with rAMH and Cy 9.9% of granulosa cells were proliferating, and 57% of primary follicles were classified as showing mild or extensive proliferation (Fig. 5A and B, no significant difference compared to control or Cy). Proliferation rate of primary follicles in ovaries treated with Cy + Tem + rAMH was similar to that of Cy + Tem (Fig. 5A and B). Representative images demonstrate the increase in Ki-67 staining in Cy-treated ovaries compared to Cy + Tem and Cy + Tem + rAMH groups (Fig. 5C).
Combined cotreatment of Tem and rAMH reduced Cy-induced PI3K pathway activation
Protein analysis of whole ovaries removed 24 h after in vivo treatment with Cy showed decreased phosphorylation of the key PI3K pathway activation proteins mTOR and rpS6 when the ovaries were cotreated with Cy + Tem + rAMH: a decrease in phosphorylated form of rpS6 and mTOR was seen in ovaries exposed to Cy + Tem alone (relative band intensity of 0.3 versus 1.88, P < 0.05, and 0.57 versus 1.44, P < 0.01, respectively, Fig. 6), and an even greater reduction in the ovaries cotreated with Cy + Tem + rAMH (relative band intensity of 0.21 versus 1.88, P < 0.01, and 0.38 versus 1.44, P < 0.01, respectively, Fig. 6).
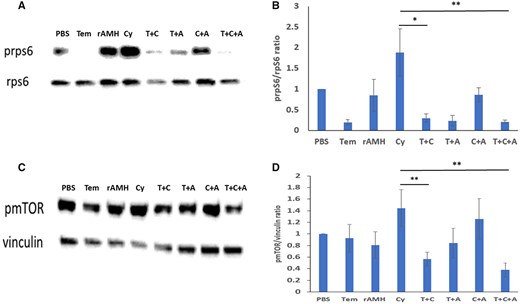
Combined treatment of Temsirolimus and recombinant AMH reduced cyclophosphamide-induced PI3K pathway activation. Protein analysis of ovaries from 8-week-old mice that were removed 24 h after a single dose of cyclophosphamide (Cy and C, 150 mg/kg) or the equivalent volume of PBS with or without cotreatment with Temsirolimus (Tem and T) and/or recombinant anti-Müllerian hormone (rAMH and A). (A) Representative western blot of A: prpS6 and rpS6, (C) pmTOR, and vinculin. (B) Intensity of the prpS6/rpsS6 ratio from five ovaries. (D) pmTOR/mTOR ratio from three ovaries. *P < 0.05 and **P < 0.01. Data were expressed as the mean ± SEM. Statistical analyses were performed by the mixed procedure followed by Tukey’s multiple comparison test. mTOR: mammalian target of rapamycin.
Tem and rAMH decreased Cy-induced DNA damage and apoptotic oocyte death
In addition to examining the ovaries for evidence of follicle activation, we also wanted to see if there was evidence of increased apoptosis. We conducted staining of the ovaries for the DNA damage marker γH2AX and for the apoptosis marker cPARP.
We found high levels of basal DNA damage in untreated ovaries: 59% of PMF oocytes were stained positive for γH2AX. However, following Cy treatment, a significant increase in DNA damage was demonstrated, when more than 90% of the PMF oocytes were stained for γH2AX (P < 0.001, Fig. 7A and B). This increase in DNA damage in PMF oocytes was significantly attenuated in ovaries which received the combined cotreatment Cy + Tem + rAMH. In this treatment group, only 69% of the PMF oocytes were positive for DNA damage (compared to 94% in Cy group, P < 0.001, Fig. 7A and B). This was not significantly higher than in untreated ovaries.
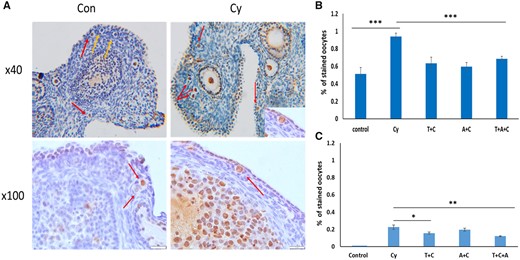
Temsirolimus and recombinant AMH decreased cyclophosphamide-induced DNA damage and apoptotic oocyte death. Ovary sections from (A) representative hematoxylin and eosin images of ovaries from control (Con, left) and Cyclophosphamide (Cy, right) treatment groups demonstrating positive (red arrows) and negative (yellow arrows) phosphorylated form of histone 2AX (γH2AX) staining. (B) Ovaries removed 12h, or (C) 24 h after Cy (also C) treatment, were stained for DNA damage using γH2AX antibody, and the apoptotic marker cleaved poly (ADP-ribose) polymerase (cPARP) antibody. Primordial follicle oocytes from one or two sections from six to eight ovaries per group were evaluated (at least 70 follicles from each group), follicles were classified as positive/negative. *P < 0.05, **P < 0.01, and ***P < 0.001. Data were expressed as the mean ± SEM. Statistical analyses were performed by the mixed procedure followed by Tukey’s multiple comparison test. Tem and T: Temsirolimus; rAMH and A: recombinant anti-Müllerian hormone.
However, despite the high levels of DNA damage evident in many of the PMF oocytes, including in untreated ovaries (59%), we found that the actual levels of apoptosis in the PMF oocytes, as indicated by positive staining for cPARP, were far lower. In untreated ovaries, none of the PMF oocytes stained positive for cPARP (Fig. 7C). In ovaries treated with Cy, 22% of the PMF oocytes stained positive for cPARP and this was significantly reduced in ovaries which were cotreated with Cy and Tem, or with the combined cotreatment of Cy + Tem + rAMH (14% and 12% cPARP-stained PMF oocytes, P < 0.05, P < 0.01, respectively, Fig. 7C).
The high rates of DNA damage seen in the PMF oocytes in all treatment groups raised a concern, since the vast majority of these PMFs survived and were present 21 days after treatment. In order to assess if there was sustained DNA damage in the surviving PMF oocytes, we conducted three cycles of mating with proven fertile males, litter sizes were evaluated, and pups were examined for physical abnormalities or malformations. The mean litter size of the mice in the Cy + Tem + rAMH group was similar to both the untreated mice and the Cy-treated mice (5.71 (n = 14) versus 4.56 (n = 21) and 4.62 (n = 19), respectively, not significant). Pups from all the treatment groups were assessed as healthy with no gross malformations or abnormalities.
Discussion
The results of this study demonstrate that combined administration of two PMF activation pathway regulators, namely Tem which is an intrafollicular PI3K pathway inhibitor, and rAMH, which is an extrafollicular suppressive agent, completely prevents Cy-induced follicle depletion and protects ovarian reserve in mice.
Various factors cause ovarian reserve depletion, including aging, pathological conditions, drug treatments, radiation, and chemotherapy. Drugs that are commonly used to induce ovulation in humans generate reactive oxygen species (ROS) and consequential apoptotic cell death in rat oocytes (Chaube, 2005), and sometimes nonapoptotic-mediated pathways of programmed cell death, such as stress-mediated necroptosis (Chaudhary, 2019). In addition, hypoxia generates excess ROS in the follicular microenvironment and induces autophagic cell death in mammalian ovaries (Yadav, 2019).
Cy has both short-term temporary and long-term persistent effects on the ovary. Direct short-term Cy effects cause apoptosis in proliferating granulosa cells of growing follicles, resulting in loss of growing follicles at all stages, presented as temporary amenorrhea (Berliere et al., 2008; Anderson and Spears, 2015), and acute disruption of AMH secretion (Kano et al., 2017; Roness et al., 2019; Sonigo et al., 2019). Long-term Cy effects are secondary to loss of the dormant PMF reservoir. Significant PMF depletion after chemotherapy has been demonstrated in human ovaries (Familiari et al., 1993; Bath et al., 2003; Shai et al., 2021), mice (Meirow et al., 1999; Pascuali et al., 2018) and rhesus monkeys ovaries (Ataya et al., 1995). While complete loss of the PMF population results in immediate amenorrhea and permanent sterility, partial loss will lead to gradual POI when the reduced follicle reserve is exhausted (Donnez et al., 2006; Petrek et al., 2006; Sklar et al., 2006; Berliere et al., 2008; Partridge et al., 2010; Gracia et al., 2012). It is therefore crucial to understand the mechanisms of chemotherapy-induced loss of the PMF population.
Tem, a rapamycin macrocyclic antibiotic analog, decreases mTOR activity by inhibition of mTOR-responsive gene translation, resulting in reduced proliferation (Yu et al., 2012; Mita et al., 2016). Studies have demonstrated that coadministration of rapamycin or its derivatives during chemotherapy partially protects ovarian reserve from Cy- or cisplatin-induced PMF loss (Goldman et al., 2017; Tanaka et al., 2018; Sato and Kawamura, 2020). However, an important limitation of PI3K pathway inhibition compounds (through mTOR inhibition) is their broad systemic regulatory activity in all cells (Manning and Cantley 2007). On the other hand, AMH activity in the adult female is almost entirely targeted to the ovary (Bedenk et al., 2020). Produced by granulosa cells of early growing follicles, AMH inhibits initiation of PMF growth in rodents (Durlinger et al., 2002; Gigli et al., 2005; Yang et al., 2017) and in human ovaries, as was shown in xenotransplantation and in most in vitro studies (Carlsson et al., 2006; Man et al., 2017). A single study on human ovarian sections in vitro did show activation rather than suppression following exposure to AMH (Schmidt et al., 2005). However, there has been no other study to support this result. In vivo mouse studies have demonstrated that coadministration of AMH during chemotherapy attenuates ovarian reserve depletion and improves fertility in mouse models (Kano et al., 2017; Roness et al., 2019; Sonigo et al., 2019). Little is known about the downstream pathways through which AMH acts on the PMFs, and it is possible that AMH suppression of follicle activation is also mediated by downregulation of the PI3K pathway and inhibition of FOXO3a phosphorylation (Sonigo et al., 2019), thereby maintaining the PMFs in a quiescent state.
This study in mice suggests the ability of follicle activation inhibitors Tem or rAMH alone to partially attenuate chemotherapy-induced PMF activation in both ex vivo and in vivo models. Each inhibitor alone protects against Cy-induced PMF stockpile depletion by 40–50%. But combined suppression of follicle activation via inhibition of both intrinsic and extrinsic pathways provided complete protection of the PMF population from Cy-induced follicle loss, resulting in ovarian morphology similar to control ovaries (Fig. 5C). The suppression of pathological follicle activation was confirmed by the changes in molecular regulators of follicle activation (decreased phosphorylation of rpS6 and mTOR) as well as reduction in Ki-67 immunostaining in early growing follicles in the short term following treatment. A possible connection between the two pathways might be through the PI3K pathway regulator, FOXO3a. The PI3K signaling network promotes cell survival by phosphorylating FOXO3a, leading to its export from the nucleus into the cytoplasm, thus inhibiting FOXO pro-apoptotic transcription factors and promoting cellular proliferation (Liu et al., 2007; Jang et al., 2016). Phosphorylation of FOXO3A has also been shown to be disrupted by AMH treatment (Reddy et al., 2010).
However, our preliminary experiments indicated that Tem inhibited rpS6 phosphorylation in mouse ovary at a Tem concentration of 0.1, and at 1 mg/kg, reaching a maximal effect at 10 mg/kg Tem (apparently Tem capability to inhibit rpS6 phosphorylation reached a plateau in this range, and we used 5 mg/kg). Moreover, in our activation (protein analysis) and proliferation assays, there was no significant difference between Tem + Cy group and Tem + rAMH + Cy group. This indicates that Tem had the maximal effect on the PI3K pathway, and the increased protection of the two inhibitors on PMF depletion was probably obtained by two different cascades.
Indeed, the fact that both inhibitors had similar degrees of protection on PMF numbers, and full protection when combined, suggests that rAMH may act primarily via an alternative PMF suppression pathway to the PI3K pathway, and implies a complementary effect between the two inhibitors.
According to the studies which propose that PMF loss is primarily attributable to direct PMF oocyte apoptotic death (Gonfloni et al., 2009; Kerr et al., 2012; Klinger et al., 2015; Bellusci et al., 2019; Luan et al., 2019; Nguyen et al., 2019; Titus et al., 2021), chemotherapy induces double-stranded DNA breaks in the PMF oocyte and, if not repaired, the oocyte undergoes apoptosis and follicular atresia within 24 h of chemotherapy. Although other studies did not find evidence of apoptosis within this timeframe (Kalich-Philosoph et al., 2013; Chang et al., 2015; Jang et al., 2016; Kano et al., 2017; Zhou et al., 2017; Pascuali et al., 2018; Shai et al., 2021) our results do show evidence of significant genetic damage in almost all PMF oocytes 12 h following exposure to Cy (94% as shown by DNA damage marker γH2AX). Even in untreated ovaries, 51% of control oocytes were positively stained for γH2AX. However, only 22% of these subsequently underwent apoptosis (as indicated by apoptotic marker cPARP) 24 h following 150 mg/kg Cy exposure, and none of the oocytes in untreated ovaries These results support a lesser role for direct apoptosis in PMF loss, but also indicate that it is not possible to equate DNA damage with oocyte death. Although most often researched separately, involvement of both follicle activation and apoptosis in PMF depletion caused by chemotherapy is plausible and was previously proposed (Bellusci et al., 2019). The data presented here indicate that less than one-third of the total PMF loss can be attributed to apoptosis, implying that most of the PMF depletion is linked to PMF activation but that both mechanisms play a significant role. The combined treatment of Cy + Tem + rAMH acted not only on the follicle activation pathways but also significantly decreased DNA damage and oocyte death, which may explain the maximal protective effect of the combined treatment. Links between activation of the PI3K pathway and DNA damage/apoptosis pathways have previously been shown; γH2AX was elevated when the AKT-FOXO3 pathway was activated, and high intracellular levels of Akt have been reported to increase DNA damage, repress nuclear translocation of BRCA1 and compromise homologous recombination in breast cancer cells (Bellusci et al., 2019; Maidarti et al., 2020). Although most follicles that demonstrated DNA damage following Cy survived, there was no persistent major damage to DNA integrity or reproductive outcome, as we observed no increase in fetal resorptions or malformation rates in pregnancies following treatment with Cy or any combination of the protective agents in three consecutive mating rounds.
A limitation of the study is that protein analysis of activation proteins was conducted on lysates, such that the protein changes identified cannot be localized to specific cells within the ovary. However, protein analysis as well as primary follicle proliferation and Day 3 counting were complementary assays, and the protein analysis results indicated massive reduction in the phosphorylation of key proteins in the PI3K cascade—the pathway one of the inhibitors inhibited. These complementary assays were consistent with the sequence of events after Cy administration, i.e. reduced activation of primordial oocytes through PI3K pathway inhibition. This sequence of events occurs separately and needs to be evaluated at different time points. Western blot analysis of the complete lysate shows the protein changes that occurred with Cy. This is a valid and well-accepted method for protein analysis and serves as the common technique for this purpose.
Another limitation, as well as a source of conflicting evidence, in studies which investigate the mechanisms of follicle loss post-chemotherapy is the varying time frames which are chosen for analysis. Studies which did not show evidence of over activation (Luan et al., 2019; Eldani et al., 2020; Titus et al., 2021) may not have noticed it owing to shorter time intervals between Cy administration and evaluation (12 h instead of 24 h or a few days in Titus et al. (2021)) or because they used nonspecific proteins, such as pAkt, as the marker of activation instead of more specific activation markers such as pFOXO3A, pMTOR, and prpS6 (Luan et al., 2019; Eldani et al., 2020). In this study, we chose 24 h following Cy administrations as the time point for exploring activation parameters and apoptosis, based on other studies (Goldman et al., 2017; Zhou et al., 2017; Nguyen et al., 2019; Sonigo et al., 2019). It is possible that the peak effect of rAMH on proliferation occurs at a later time point. This should be investigated in the future.
One more limitation is the Ki-67 assay quantification. This marker is usually used qualitatively. However, we used it for the first time to assess proliferation status of individual primary follicles, and as a comparison tool between the treatment groups. As there is no reference for this in the literature, we counted at least 70 follicles per group and also normalized the number of positive granulosa cells to the total number of primary follicles granulosa cells, in order to increase the reliability of our method.
In conclusion, the combination of intrinsic–extrinsic inhibitors of follicle activation completely eliminated Cy-induced follicle depletion, shedding light on the complementary suppression of upregulated follicle activation, and to a lesser degree their role in decreasing DNA damage and PMF oocyte death. These results provide an interesting basis for future research, and a potential direction for noninvasive pharmacological fertility preservation.
Data availability
The data underlying this article are available in the article, and when necessary, via [email protected].
Authors’ roles
O.K. and H.R. designed the study, performed the experiments, collected the data, and analyzed it. O.K. wrote the article. I.S. assisted in performing the histological immunostainings, S.D.-H. assisted in western blot assays and in discussing the results, and D.M. initiated and supervised the study. D.M. and H.R. edited the article.
Funding
This work was financially supported by grant from the Morris Kahn Foundation.
Conflict of interest
The authors declare that they have no conflict of interest.