-
PDF
- Split View
-
Views
-
Cite
Cite
Wafi Siala, Françoise Van Bambeke, Vincenzo Taresco, Antonella Piozzi, Iolanda Francolini, Synergistic activity between an antimicrobial polyacrylamide and daptomycin versus Staphylococcus aureus biofilm, Pathogens and Disease, Volume 74, Issue 5, July 2016, ftw042, https://doi.org/10.1093/femspd/ftw042
- Share Icon Share
Antibiotic resistance of bacteria growing in biofilms compared to their planktonic counterparts enhances the difficulty to eradicate biofilm-associated infections. In the last decade, combination antibiotic therapy has emerged as an attractive strategy for treating biofilm infections, even if in most of tolerant biofilms the optimal combinations are still unknown. In this study, an antimicrobial cationic polyacrylamide was used in combination with daptomycin or moxifloxacin against mature biofilms of Staphylococcus aureus clinical isolates to examine a possible improvement of the antibiofilm activity of the two antibiotics. The polymer did not have an effect on moxifloxacin but significantly increased the antibiofilm efficacy of daptomycin. These findings are presumably related to the different mechanism of action of the two drugs. In summary, our data highlighted the ability of polycations to increase daptomycin antibiofilm activity providing a potential strategy to eradicate biofilms in industrial or medical settings.
INTRODUCTION
In the last decade, polymeric materials with antimicrobial properties have emerged as promising tools to prevent microbial contamination and biofilm formation in different areas including food industry and medical settings (Munoz-Bonilla and Fernandez-Garcia 2012).
Polymers can act as carriers for antimicrobial agents that once released from the polymeric matrix kills surrounding microorganisms. In this case, polymer properties such as hydrophilicity, crystallinity and molecular weight greatly influence the performance of the system. Alternatively, polymers can be inherently biocidal and exert their killing action when interacting with microorganisms (Francolini etÃÂ al.2015).
In this regard, polymers bearing positive charges (cationic polymers) are extensively investigated for various therapeutic applications including gene delivery, tissue engineering and infection treatment (Samal etÃÂ al.2012). Similarly to antimicrobial peptides, cationic polymers are membrane active antimicrobial compounds and their mechanism of action is based on two elements: (i) electrostatic interactions of cationic groups with the polyanionic bacterial cell surface and (ii) insertion of the stiff hydrophobic polymer backbone into the bacterial membrane. This polymer/membrane interaction is devastating for the membrane and can cause leakage of cytoplasmic material up to cell lysis (Friedrich etÃÂ al.2000; Kenawy, Worley and Broughton 2007; Timofeeva and Kleshcheva 2011). Additionally, it has been demonstrated that balancing positive charge/hydrophobicity ratio, thus polymer amphiphilicity structure, it is possible to both improve bactericidal effect and reduce polymer cytotoxicity (Palermo and Kuroda 2009; Taresco etÃÂ al.2015a,b).
Different kinds of cationic synthetic polymers have been lately developed, among which polyacrylamides and polyacrylates bearing tertiary or quaternary ammine groups are the most investigated due to their wide versatility and ease of synthesis (Butun, Armes and Billingham 2001a,b; Palermo and Kuroda 2009, 2010; Kuroda and Caputo 2013). Indeed, the antimicrobial activity and physicochemical features of these polymers can be properly modulated by varying the type of monomers, polymer amphiphilicity, type of counterion of charged groups and the alkyl chain length attached to the cationic groups (Kenawy, Worley and Broughton 2007; Palermo and Kuroda 2010). In addition, they have been shown to possess a broad spectrum of activity against both Gram-negative and Gram-positive bacteria (Shai 1999).
Our group has recently developed a water-soluble polyacrylamide (poly-N-[2-N,N-diethylamino)ethyl] acrylamide, pAcDED) bearing a tertiary amine showing an interesting antimicrobial activity versus Staphylococcus epidermidis (Francolini etÃÂ al.2013). From in vitro assays, this polymer resulted to be neither cytotoxic nor hemolytic (Taresco etÃÂ al.2015a). So far, this polymer was successfully employed as an active nanocarrier for usnic acid (Francolini etÃÂ al.2013).
In this work, we investigated the possibility to employ this antimicrobial polyacrylamide to potentiate the activity of antibiotics against microbial biofilms. We expect that the polyacrylamide binding, insertion and destabilization to the phospholipid bilayer bacterial membrane could enhance antibiotic uptake and promote microbial killing.
Besides, the use of antimicrobial polymer/antibiotic combinations could represent a strategy to counteract drug resistance. Indeed biofilm growing bacteria display increased antimicrobial resistance and tolerance compared to planktonic bacteria (Fux etÃÂ al.2005; Lewis 2007). The minimal biofilm-eradication concentrations (MBECs) of antibiotics commonly used for treatment of staphylococcal infections, such as vancomycin (VAN), daptomycin (DAP), teicoplanin, linezolid and ciprofloxacin, are up to 4000-fold higher than the MBCs (LaPlante and Mermel 2009; Mataraci and Dosler 2012). Mechanisms of antimicrobial resistance and tolerance in biofilm are limited drug diffusion through the biofilm matrix, drug deactivation by binding to matrix components or enzymatic degradation and nutrient limitation in the inner biofilm layers inducing cell starvation (Lewis 2008; Hoiby etÃÂ al.2010). The poor drug penetration through the biofilm is actually controversial. Indeed, Jefferson, Goldmann and Pier (2005) demonstrated that, under static conditions, VAN permeated only partially a S. aureus biofilm during 1 h of exposure to the drug. On the contrary, Stewart, Davison and Steenbergen (2009) showed that DAP rapidly penetrated into staphylococcal biofilms. Similarly, ciprofloxacin has been shown to have a good penetration into a staphylococcal biofilm (Singh etÃÂ al.2010).
To find out a combination therapy to eradicate microbial biofilms, in this work, two antibiotics, the fluoroquinolone moxifloxacin and the lipopetide DAP, were tested in combination with the polyacrylamide pAcDED versus staphylococcal biofilms. Different S. aureus strains were employed: a standard methicillin-sensible S. aureus (MSSA) and six clinical isolates of methicillin-resistant S. aureus (MRSA). The two antibiotics were chosen because of their antimicrobial activity versus Gram positives.
Moxifloxacin, a fourth-generation oral fluoroquinolone, exerts its antimicrobial effect by preventing bacterial DNA duplication. In previous studies, the ability of moxifloxacin to reduce biofilms has been demonstrated against slimes synthesized by different Gram-negative and Gram-positive microorganisms. The drug has decreased the density of biofilms formed in vitro by clinical isolates of Stenotrophomonas maltophilia, S. aureus, coagulase-negative staphylococci and viridans streptococci (Di Bonaventura etÃÂ al.2004; Perez-Giraldo etÃÂ al.2004). Moxifloxacin was the most effective antibiotic even when tested against biofilms produced by periodontopathic bacteria such as Actinobacillus actinomycetemcomitans, Porphyromonas gingivalis and Streptococcus constellatus (Eick and Pfister 2004). However, moxifloxacin showed moderate activity when tested against S. aureus biofilms as recently showed by Bauer etÃÂ al. (2013), who developed an in vitro pharmacodynamic model allowing for comparison of antibiotic relative potencies and maximal efficacies against biofilms.
DAP produces membrane depolarization which leads to disrupt bacterial cell membrane barrier functions. Roveta, Marchese and Schito (2008) showed that DAP at concentrations achievable during therapy prevent biofilm building and induced disaggregation of its structure in young and mature biofilms on a plastic support in S. aureus and S. epidermidis. When tested in the in vitro pharmacodynamic biofilm model cited previously, DAP was highly effective, being able to reduce metabolic and respiratory activities of 85%âÂÂ90% of bacteria within the biofilm of lab strains (Bauer etàal.2013). However, when using clinical isolates collected from patients suffering from persistent infections, a marked decrease in DAP potency was observed (Siala etàal.2014).
Therefore, in this study we examined if pAcDED could improve in vitro activities of moxifloxacin and DAP against mature biofilms of S. aureus clinical isolates.
MATERIALS AND METHODS
Materials
Sodium metabisulphite (Na2S2O5) and potassium monobasic phosphate (K2HPO4) were purchased from Carlo Erba. Acryloyl chloride (Ac) 96% and N, N-diethylethylendiamine (DED) were supplied from FLUKA. Potassium persulfate (K2S2O8) and ferrous sulfate (FeSO4) were purchased from Sigma-Aldrich, while DAP (potency: 100%, molecular weight = 1617 gàmLâÂÂ1) from Novartis Pharma AG (Basel, Switzerland) and moxifloxacin (molecular weight = 401 gàmolâÂÂ1) from Bayer HealthCare (Leverkusen, Germany). Media for bacterial culture were from Becton Dickinson Company (Franklin Lakes, NJ). The redox indicator resazurin for biofilm quantification was purchased from Sigma-Aldrich. All of chemicals were of analytical grade and used as received.
Synthesis of the tertiary amine-bearing polyacrylamide
The tertiary amine-bearing polyacrylamide was obtained by classic-radical polymerization of a cationic acrylic monomer (AcDED) obtained by reaction of Ac and DED, as described elsewhere (Francolini etÃÂ al.2013). Briefly, AcDED was synthesized by adding DED (0.029 mol) into a solution of Ac (0.038 mol) in dimethylcarbonate (75 mL) containing K2HPO4 (0.08 moles) (Zhang etÃÂ al.2009). Following 4 h at room temperature, the solution was filtered and the monomer was recovered by solvent evaporation.
For polymer synthesis, 5 mL of monomer aqueous solution (1.0 M) was mixed with the radical initiators (K2S2O8, 2.8 à10âÂÂ4 mmoles and FeSO4 2.4 à10âÂÂ4 mmoles) and let polymerize for 24 h at 25ðC. The resulting polyacrylamide (pKb = 8.61) was called pAcDED.
Bacterial strains and biofilm culture conditions
ATCC25923 (MSSA) was used as a reference strain. Six clinical strains isolated from various sites (infections on medical devices or chronic tissue infections) were selected from the collection of the Belgian reference center for S. aureus (HôpitalErasme, Universitélibre de Bruxelles, Brussels) or from microbiology Laboratory (Cliniques universitaires Saint Luc, Université catholique de Louvain, Brussels) (see Tableà1). They were characterized as previously described with respect to toxin expression and molecular typing (Denis etàal.2004).
Strainsa . | Origin . | pAcDedb . | pAcDEDb . | Daptomycin . | Daptomycin . | Moxifloxacin . | Moxifloxacin . |
---|---|---|---|---|---|---|---|
. | . | MIC (mgàLâÂÂ1) . | MBEC (mgàLâÂÂ1) . | MIC (mgàLâÂÂ1) . | MBEC (mgàLâÂÂ1) . | MIC (mgàLâÂÂ1) . | MBEC (mgàLâÂÂ1) . |
ATCC25923 (MSSA) | Reference strain | 100 | ndc | 0.5 | 128 | 0.032 | 64 |
Surv375 (MSSA) | Chirurgical wound | 50 | nd | 1 | 1024 | 0.25 | 256 |
Surv651 (MRSA) | Respiratory infection | 100 | nd | 0.5 | 1024 | 2 | 1024 |
Surv456 (MRSA) | Skin | 50 | nd | 1 | 1024 | 0.5 | 256 |
Surv999 (MRSA) | KneePeriprosthetic joint | 50 | nd | 0.5 | 512 | 0.125 | 512 |
infection(PJI) | |||||||
SurvS027 (MSSA) | Cellulitis and bacteremia | 50 | nd | 0.5 | 128 | 0.125 | 64 |
Surv179 (MRSA) | Skin | 100 | nd | 0.5 | 1024 | 2 | 256 |
Strainsa . | Origin . | pAcDedb . | pAcDEDb . | Daptomycin . | Daptomycin . | Moxifloxacin . | Moxifloxacin . |
---|---|---|---|---|---|---|---|
. | . | MIC (mgàLâÂÂ1) . | MBEC (mgàLâÂÂ1) . | MIC (mgàLâÂÂ1) . | MBEC (mgàLâÂÂ1) . | MIC (mgàLâÂÂ1) . | MBEC (mgàLâÂÂ1) . |
ATCC25923 (MSSA) | Reference strain | 100 | ndc | 0.5 | 128 | 0.032 | 64 |
Surv375 (MSSA) | Chirurgical wound | 50 | nd | 1 | 1024 | 0.25 | 256 |
Surv651 (MRSA) | Respiratory infection | 100 | nd | 0.5 | 1024 | 2 | 1024 |
Surv456 (MRSA) | Skin | 50 | nd | 1 | 1024 | 0.5 | 256 |
Surv999 (MRSA) | KneePeriprosthetic joint | 50 | nd | 0.5 | 512 | 0.125 | 512 |
infection(PJI) | |||||||
SurvS027 (MSSA) | Cellulitis and bacteremia | 50 | nd | 0.5 | 128 | 0.125 | 64 |
Surv179 (MRSA) | Skin | 100 | nd | 0.5 | 1024 | 2 | 256 |
All clinical isolates belong to the epidemic CC5 or CC8 clonal complexes.
MICs were determined by microdilution according to CLSI recommendations (Clinical and Laboratory Standards Institute 2012).
nd: not determined at tested concentrations, higher than 2048 mgàLâÂÂ1.
Strainsa . | Origin . | pAcDedb . | pAcDEDb . | Daptomycin . | Daptomycin . | Moxifloxacin . | Moxifloxacin . |
---|---|---|---|---|---|---|---|
. | . | MIC (mgàLâÂÂ1) . | MBEC (mgàLâÂÂ1) . | MIC (mgàLâÂÂ1) . | MBEC (mgàLâÂÂ1) . | MIC (mgàLâÂÂ1) . | MBEC (mgàLâÂÂ1) . |
ATCC25923 (MSSA) | Reference strain | 100 | ndc | 0.5 | 128 | 0.032 | 64 |
Surv375 (MSSA) | Chirurgical wound | 50 | nd | 1 | 1024 | 0.25 | 256 |
Surv651 (MRSA) | Respiratory infection | 100 | nd | 0.5 | 1024 | 2 | 1024 |
Surv456 (MRSA) | Skin | 50 | nd | 1 | 1024 | 0.5 | 256 |
Surv999 (MRSA) | KneePeriprosthetic joint | 50 | nd | 0.5 | 512 | 0.125 | 512 |
infection(PJI) | |||||||
SurvS027 (MSSA) | Cellulitis and bacteremia | 50 | nd | 0.5 | 128 | 0.125 | 64 |
Surv179 (MRSA) | Skin | 100 | nd | 0.5 | 1024 | 2 | 256 |
Strainsa . | Origin . | pAcDedb . | pAcDEDb . | Daptomycin . | Daptomycin . | Moxifloxacin . | Moxifloxacin . |
---|---|---|---|---|---|---|---|
. | . | MIC (mgàLâÂÂ1) . | MBEC (mgàLâÂÂ1) . | MIC (mgàLâÂÂ1) . | MBEC (mgàLâÂÂ1) . | MIC (mgàLâÂÂ1) . | MBEC (mgàLâÂÂ1) . |
ATCC25923 (MSSA) | Reference strain | 100 | ndc | 0.5 | 128 | 0.032 | 64 |
Surv375 (MSSA) | Chirurgical wound | 50 | nd | 1 | 1024 | 0.25 | 256 |
Surv651 (MRSA) | Respiratory infection | 100 | nd | 0.5 | 1024 | 2 | 1024 |
Surv456 (MRSA) | Skin | 50 | nd | 1 | 1024 | 0.5 | 256 |
Surv999 (MRSA) | KneePeriprosthetic joint | 50 | nd | 0.5 | 512 | 0.125 | 512 |
infection(PJI) | |||||||
SurvS027 (MSSA) | Cellulitis and bacteremia | 50 | nd | 0.5 | 128 | 0.125 | 64 |
Surv179 (MRSA) | Skin | 100 | nd | 0.5 | 1024 | 2 | 256 |
All clinical isolates belong to the epidemic CC5 or CC8 clonal complexes.
MICs were determined by microdilution according to CLSI recommendations (Clinical and Laboratory Standards Institute 2012).
nd: not determined at tested concentrations, higher than 2048 mgàLâÂÂ1.
The MICs of pAcDED, DAP and moxifloxacin against the selected strains were determined by microdilution according to CLSI recommendations (Clinical and Laboratory Standards Institute 2012).
Biofilms were obtained using as starting inoculum bacteria transferred from frozen stocks onto Trypticase Soy Agar plates and incubated overnight at 37ðC, after which 10 colonies were inoculated in Trypticase Soy Broth (TSB) supplemented with 2% NaCl and 1% glucose, and bacterial density was adjusted to an OD620 nm = 0.005. For quantitative analysis, 200 üL of bacterial suspension were cultivated in 96-well plates (VWR [Radnor, PA] tissues culture plates; European cat. number 734âÂÂ2327) for 24 h so as to generate a mature biofilm.
Biofilms were then exposed for 48 h to increasing concentrations of moxifloxacin or DAP (0.125âÂÂ32 mgàLâÂÂ1) alone or in combination with the polymer pAcDED (32 mgàLâÂÂ1). Antibiotics were prepared in TSB supplemented with 2% NaCl, 1% glucose and 50 mgàmLâÂÂ1 CaCl2 (CaTGN).
Bacterial viability in the biofilm was quantified using the redox indicator resazurin which is reduced by viable bacteria to the pink fluorescent compound resorufin. In brief, at the end of the incubation period, the medium was removed and wells were washed twice with 250 üL of phosphate-buffered saline (PBS). Biofilms were incubated with 200 üL of 10 ügàmLâÂÂ1 resazurin during 30 min at room temperature in the dark. Resorufin fluorescence was measured at a wavelength of 590 nm using an excitation wavelength of 560 nm (SPECTRAmax Spectrofluorometer, MolecularDevices).
Determination of MBEC
The 24 h biofilms in a 96-well plates were washed twice with 250 üL PBS solutions. Serial dilutions ranging from 2048 to 0.03 mgàLâÂÂ1 for DAP and moxifloxacin were prepared in CATGN. 200 üL of each concentration was added to each corresponding well and plates were incubated 24 h at 37 C. The antibiotics were removed, the plates were washed twice with PBS and the wells were thoroughly scraped with particular attention to the edges of the wells. The contents of each well were removed, placed in 1 mL PBS, incubated in a sonicating water bath (Branson ultrasonic 5510) for 5 min to disrupt the biofilms, and 100 üL samples were plated on TSA. The colonies were counted after 24 h of incubation at 37ðC. MBEC was defined as the lowest concentration of antibiotics which bacteria fail to regrow after exposure to the antimicrobial agents. The same tested was performed by using the drugs in combination with pAcDED (32 mgàLâÂÂ1).
RESULTS AND DISCUSSION
The biofilm mode of growth is now recognized as the predominant form in which bacteria are present in many different environments (Costerton etÃÂ al.1995). Particularly, bacteria in biofilms display a different phenotype than their planktonic counterparts and are able to cooperate against external stresses (environmental changes, antibiotic treatments, etc.) thanks to an internal communication mechanism called quorum sensing (Stoodley etÃÂ al.2002; Sifri 2008). Thanks to this cooperation, bacteria growing as biofilms exhibit high antimicrobial resistance compared to planktonic cells. Combination therapy can be required for the treatment of biofilm-based infections. Several studies have investigated the efficacy in vitro and in vivo of combination therapy against biofilms by Gram positives. Specifically, Olson etÃÂ al. (2010) showed that rifampin (RIF) was able to enhance the in vitro activity of DAP or VAN against S. epidermidis biofilms. This finding was later confirmed by the Rybak's research group by using a novel in vitro pharmacokinetic/pharmacodynamic (PK/PD) model of bacterial biofilm (Hall Snyder etÃÂ al.2014). Particularly, the authors tested DAP or VAN alone and in combination with RIF or clarithromycin (CLA) against strains of S. aureus and S. epidermidis grown in biofilm on three prosthetic device materials (titanium, Teflon and steel). While CLA did not enhance DAP or VAN killing activities, RIF increased activity of DAP or VAN against embedded biofilm cells in all tested materials compared to DAP or VAN alone.
Combination therapy of RIF with linezolid, VAN or tigecycline was shown to have an enhanced efficacy compared to RIF monotherapy in a rat model of MRSA chronic foreign body osteomyelitis (Vergidis etÃÂ al.2011, 2015).
However, the combination of RIF and VAN has shown conflicting results, since some studies indicate that, although this combination might be effective against MRSA, it may not hold promise for use in treating MRSA biofilm infections (Salem, Elkhatib and Noreddin 2011; Zimmerli 2014).
More recently, ò-lactams in combination with DAP were shown to provide better killing and prevent resistance in both VAN-resistant enterecocci (Smith etàal.2015) and MRSA (Barber etàal.2015). ò-Lactams seem to be also able to potentiate the activity of the new lipoglycopeptide antibiotic oritavancin against multidrug-resistant S. aureus (Smith etàal.2016).
On the contrary, linezolid was demonstrated to antagonize VAN or DAP activity against MRSA biofilm in an in vitro pharmacodynamic model (Luther and LaPlante 2015).
In this framework, the aim of this study was to test whether an antimicrobial acrylic polycation was able to improve/amplify the effectiveness of two antibiotics, DAP and moxifloxacin (Fig.ÃÂ 1), against bacterial biofilms.
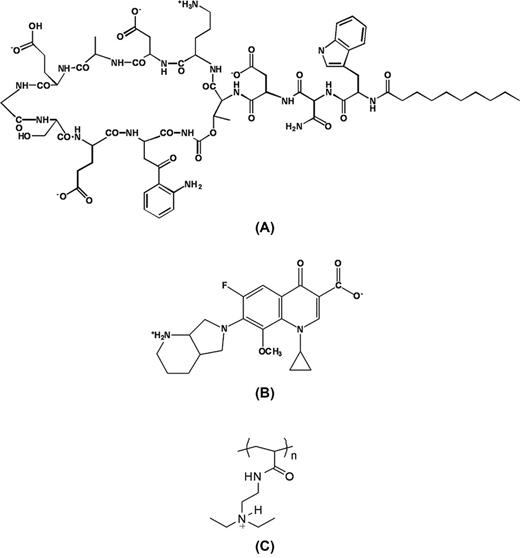
Chemical structure of daptomycin (A), moxifloxacin (B) and pAcDED (C).
Particularly, a cationic polyacrylamide pAcDED (Fig.à1), recently synthesized by Francolini etàal. (2013), has been tested alone or in combination with DAP or moxifloxacin against staphylococcal biofilms by different strains. pAcDED (Mw = 70000 gàmolâÂÂ1) is a hydrophilic polymer forming in water stable 500 nm in size nanoparticles. In vitro cytotoxicity tests showed that at the pAcDED MIC (100 mgàLâÂÂ1) cell viability was ca. 80% at 30 min incubation in the presence of pAcDED. The hemolytic activity was poor both at the MIC and at concentrations 10 times higher than that of MIC (Taresco etàal.2015a).
DAP and moxifloxacin were chosen not only because of their proved activity against Gram-positive bacteria but also because possessing anionic carboxylic groups (four groups displayed by DAP and one group displayed by moxifloxacin, Fig.ÃÂ 1) enabling their interaction with the cationic pAcDED. In addition, the mechanism of action of the two drugs is different, moxifloxacin activity resulting from inhibition of the enzymes topoisomerase II and IV while DAP acting at the level of the cell membrane causing disruption of membrane function with K+ efflux and membrane depolarization.
In TableÃÂ 1, the MIC and MBEC of DAP, moxifloxacin and pAcDED are reported for the seven tested strains. pAcDED exhibited activity towards all the tested staphylococcal strains with MIC values comparable to those reported in the literature for cationic polymers based on ammonium salts (Palermo and Kuroda 2009).
In Tableà2, the MBEC values for DAP and moxifloxacin in combination with pAcDED are reported. DAP MBECs ranged between 128 and 1024 mgàLâÂÂ1 depending on the strain. However, when combined with pAcDED, biofilm eradication was observed at 32 mgàLâÂÂ1 for all strains (Tableà2). These findings show a synergistic activity in biofilm eradication between DAP and pAcDED. Differently, pAcDED/moxifloxacin combination had only a slight positive effect on drug MBEC in two strains (Surv375 and Surv179), no effect in three strains (ATCC25923, Surv651 and SurvS027) and a negative effect in two strains (Surv456 and Surv999).
Strainsa . | Daptomycin . | MBEC (mgàLâÂÂ1) . | Moxifloxacin . | MBEC (mgàLâÂÂ1) . |
---|---|---|---|---|
. | MBEC (mgàLâÂÂ1) . | daptomycin + pAcDEDb . | MBEC (mgàLâÂÂ1) . | moxifloxacin + pAcDEDb . |
ATCC25923 (MSSA) | 128 | 32 | 64 | 64 |
Surv375 (MSSA) | 1024 | 32 | 256 | 128 |
Surv651 (MRSA) | 1024 | 32 | 1024 | 1024 |
Surv456 (MRSA) | 1024 | 32 | 256 | 512 |
Surv999 (MRSA) | 512 | 32 | 512 | 1024 |
SurvS027 (MSSA) | 128 | 32 | 64 | 64 |
Surv179 (MRSA) | 1024 | 32 | 256 | 128 |
Strainsa . | Daptomycin . | MBEC (mgàLâÂÂ1) . | Moxifloxacin . | MBEC (mgàLâÂÂ1) . |
---|---|---|---|---|
. | MBEC (mgàLâÂÂ1) . | daptomycin + pAcDEDb . | MBEC (mgàLâÂÂ1) . | moxifloxacin + pAcDEDb . |
ATCC25923 (MSSA) | 128 | 32 | 64 | 64 |
Surv375 (MSSA) | 1024 | 32 | 256 | 128 |
Surv651 (MRSA) | 1024 | 32 | 1024 | 1024 |
Surv456 (MRSA) | 1024 | 32 | 256 | 512 |
Surv999 (MRSA) | 512 | 32 | 512 | 1024 |
SurvS027 (MSSA) | 128 | 32 | 64 | 64 |
Surv179 (MRSA) | 1024 | 32 | 256 | 128 |
All clinical isolates belong to the epidemic CC5 or CC8 clonal complexes.
32 mgàLâÂÂ1 pAcDED was used for combinations.
Strainsa . | Daptomycin . | MBEC (mgàLâÂÂ1) . | Moxifloxacin . | MBEC (mgàLâÂÂ1) . |
---|---|---|---|---|
. | MBEC (mgàLâÂÂ1) . | daptomycin + pAcDEDb . | MBEC (mgàLâÂÂ1) . | moxifloxacin + pAcDEDb . |
ATCC25923 (MSSA) | 128 | 32 | 64 | 64 |
Surv375 (MSSA) | 1024 | 32 | 256 | 128 |
Surv651 (MRSA) | 1024 | 32 | 1024 | 1024 |
Surv456 (MRSA) | 1024 | 32 | 256 | 512 |
Surv999 (MRSA) | 512 | 32 | 512 | 1024 |
SurvS027 (MSSA) | 128 | 32 | 64 | 64 |
Surv179 (MRSA) | 1024 | 32 | 256 | 128 |
Strainsa . | Daptomycin . | MBEC (mgàLâÂÂ1) . | Moxifloxacin . | MBEC (mgàLâÂÂ1) . |
---|---|---|---|---|
. | MBEC (mgàLâÂÂ1) . | daptomycin + pAcDEDb . | MBEC (mgàLâÂÂ1) . | moxifloxacin + pAcDEDb . |
ATCC25923 (MSSA) | 128 | 32 | 64 | 64 |
Surv375 (MSSA) | 1024 | 32 | 256 | 128 |
Surv651 (MRSA) | 1024 | 32 | 1024 | 1024 |
Surv456 (MRSA) | 1024 | 32 | 256 | 512 |
Surv999 (MRSA) | 512 | 32 | 512 | 1024 |
SurvS027 (MSSA) | 128 | 32 | 64 | 64 |
Surv179 (MRSA) | 1024 | 32 | 256 | 128 |
All clinical isolates belong to the epidemic CC5 or CC8 clonal complexes.
32 mgàLâÂÂ1 pAcDED was used for combinations.
To confirm these data, bacterial viability in the biofilm was quantified using the redox indicator resazurin. Particularly, the reduction of resazurin in resorufin is proportional to the number of metabolically active cells present in the biofilm. FiguresÃÂ 2 andÃÂ 3 show the activity of DAP, moxifloxacin and combination against 24-h biofilms exposed for 48 h to drugs.
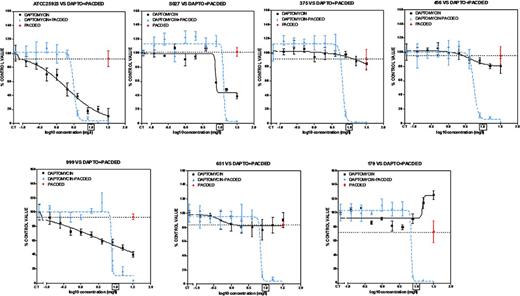
Concentration-response activity of increasing concentrations of daptomycin (0.125âÂÂ32 mgàLâÂÂ1) combined with pAcDED polymer (32 mgàLâÂÂ1) against S. aureus biofilms (Clinical and reference strains). 24-h biofilms were incubated with increasing concentrations of daptomycin alone or in combination with pAcDED polymer for 48 h. The ordinate shows the change in resorufin fluorescence (bacterial viability) in percentage of the control value (no treatment). Black lines, daptomycin alone; blue lines, combinations; red point on dotted line, effect of pAcDED alone (32 mgàlâÂÂ1). The squared concentration corresponds to daptomycin human Cmax reached in the serum of patient receiving conventional dosages. All values are means ñ standard deviations (SD) of four wells.
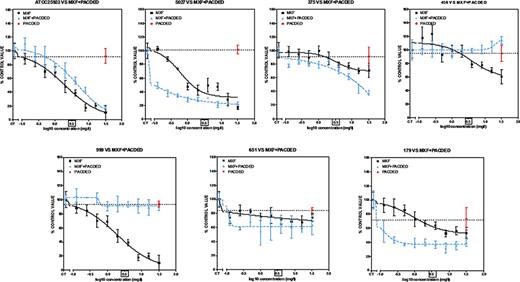
Concentration-response activity of increasing concentrations of moxifloxacin (0.125âÂÂ32 mgàLâÂÂ1) combined with pAcDED polymer (32 mgàLâÂÂ1) against S. aureus biofilms (Clinical and reference strains). 24-h biofilms were incubated with increasing concentrations of moxifloxacin alone or in combination with pAcDED polymer for 48 h. The ordinate shows the change in resorufin fluorescence (bacterial viability) in percentage of the control value (no treatment). Black line, moxifloxacin alone; blue line, combinations; red point on dotted line, effect of pAcDED alone (32 mgàLâÂÂ1).The squared concentration corresponds to moxifloxacin human Cmax reached in the serum of patient receiving conventional dosages. All values are means ñ standard deviations (SD) of four wells.
Considering first the effect of pAcDED alone on viability, the polymer was poorly potent against biofilms of all strains (0%âÂÂ25% reduction vs control). At clinically achievable concentration (Cmax: 9.4 mgàLâÂÂ1) (Benvenuto etàal.2006), DAP alone showed low activity against bacteria within biofilms (no 90% reduction in viability of tested strains: 0/7). However, when DAP was combined with pAcDED, viability was reduced by more than 90% in all tested strains (7/7). Combination with 32 mgàLâÂÂ1 pAcDED improved highly antimicrobial activity of DAP against biofilms of all tested clinical isolates.
Moxifloxacin alone was poorly active on these biofilms, reducing less than 60% bacterial viability for three out of seven strains, at the Cmax (3.1 mgàLâÂÂ1). Generally, pAcDED had no effect on moxifloxacin activity and decreased drug activity in two strains (Fig.à3). These findings are in good agreement with the MBECs and are presumably related to the different mechanisms of action of the two tested drugs.
Although the mechanism behind the observed effects needs to be further investigated, we can hypothesize that the increased antibiofilm activity of pAcDED/DAP combination is related to the establishment of electrostatic interactions between the anionic drug and the cationic polymer. Indeed, DAP has four carboxilic groups per molecule that can interact with the pAcDED basic groups (one amino group per repeat unit, Fig.ÃÂ 1). At all the tested drug concentrations, pAcDED was in a molar excess with respect to the drug. Presumably, following pAcDED interaction with the drug acidic groups, DAP can gain a positive net charge facilitating its adsorption onto the cell membrane. Indeed, it is known that DAP requires complexation with Ca2+ to exert antimicrobial activity (Baltz 2009). Ca2+-bound DAP acts as a cationic peptide.
Also moxifloxacin can interact with pAcDED by its carboxylic group (Fig.ÃÂ 1). However, pAcDED had no effect on moxifloxacin activity. That could be explained by the different action mechanism of this drug compared to DAP. Indeed, while DAP acts on the bacterial membrane, moxifloxacin prevents bacterial DNA duplication. Presumably, the formation of a high molecular weight moxifloxacin/pAcDED complex hampered drug cellular uptake. This could explain the observed decreased drug activity in two of the seven tested strains.
In the literature, an enhanced antibiofilm activity was found by Chakraborty etÃÂ al. (2012) by using VAN in combination with carboxymethyl chitosan modified with folic acid. The VAN/modified chitosan conjugates decreased biofilm formation by different strains of S. aureus. The authors stated that chitosan likely caused membrane depolarization leading to cell membrane permeabilization. Zhang etÃÂ al. (2013) found an increased susceptibility of Listeria monocytogenes biofilms to chitosan/streptomycin conjugates. By using polyclonal antibody to streptomycin, an enhanced diffusion of the drug/polymer conjugate through the biofilm matrix compared to drug alone was demonstrated.
CONCLUSIONS
In this work, we report a strategy to eradicate staphylococcal biofilms in industrial or medical settings based on the combination of DAP and an antimicrobial polycation. The observed enhanced antibiofilm activity of DAP/pAcDED combination is likely due to the establishment of polymer/drug electrostatic interactions increasing drug accumulation to the bacterial cell membrane. The polymer pAcDED had no effect on moxifloxacin activity versus S. aureus biofilms. These findings are presumably related to the different mechanisms of action of the two tested drugs.
The interesting results obtained in this study prompt us to plan further experiments to provide mechanistic insights for the antibiofilm capacity of pAcDED/DAP combination.
The authors thank O. Denis (Belgian reference center for staphylococci, Hôpital Erasme, Université libre de Bruxelles, Brussels, Belgium) for the kind gift of the strains.
FUNDING
This work was financially supported by the Italian Ministry of Education, University and Research (MIUR), by the program Prospective Research for Brussels from Innoviris, Belgium, the Fonds de la Recherche Scientifique (grant T.0134.13), and the Interuniversity Attraction Poles Programme initiated by the Belgian Science Policy Office (program IAP P7/28).
Conflict of interest. None declared.
REFERENCES