-
PDF
- Split View
-
Views
-
Cite
Cite
Girish Ramachandran, Komi Aheto, Mark E. Shirtliff , Sharon M. Tennant, Poor biofilm-forming ability and long-term survival of invasive Salmonella Typhimurium ST313, Pathogens and Disease, Volume 74, Issue 5, July 2016, ftw049, https://doi.org/10.1093/femspd/ftw049
- Share Icon Share
Salmonella enterica serovar Typhimurium, an enteric pathogen that causes a self-limiting gastroenteritis, forms biofilms on different surfaces. In sub-Saharan Africa, Salmonella Typhimurium of a novel sequence type (ST) 313 was identified and produces septicemia in the absence of gastroenteritis. No animal reservoir has been identified, and it is hypothesized that transmission occurs via human to human. In this study, we show that invasive Salmonella Typhimurium ST313 strains from Mali are poor biofilm producers compared to Salmonella Typhimurium ST19 strains, which are found worldwide and are known to be associated with gastroenteritis. We evaluated biofilms using crystal violet staining, examination of the red, dry and rough morphotype, pellicle formation and a continuous flow system. One month-old Salmonella Typhimurium ST19 colonies survived in the absence of exogenous nutrients and were highly resistant to sodium hypochlorite treatment compared to Salmonella Typhimurium ST313. This study for the first time demonstrates the comparative biofilm-forming ability and long-term survival of clinical Salmonella Typhimurium ST19 and ST313 isolates. Salmonella Typhimurium ST19 strains are strong biofilm producers and can survive desiccation compared to Salmonella Typhimurium ST313 that form weak biofilms and survive poorly following desiccation. Our data suggest that like Salmonella Typhi, Salmonella Typhimurium ST313 lack mechanisms that allow it to persist in the environment.
INTRODUCTION
Salmonella enterica subspecies enterica serovar Typhimurium is generally acquired by ingesting contaminated food or water and produces a self-limited gastroenteritis in healthy hosts. However, in immunocompromised hosts, Salmonella Typhimurium can become invasive and is associated with high fatality rates (Gordon 2008). In particular, Salmonella Typhimurium is commonly isolated from blood and other typically sterile sites of infants and HIV-infected adults in sub-Saharan Africa (Feasey et al.2012).
Multilocus sequence typing analysis (Achtman et al.2012) has shown that almost all the invasive Salmonella Typhimurium strains from Malawi and both eastern and western Kenya fall into a rare sequence type (ST), designated 313 (Kingsley et al.2009). In comparison, the sequenced Salmonella Typhimurium strains LT2, SL1344 and NCTC13348 are ST19 and are associated with classic gastroenteritis. Elucidation of the complete genomic sequence of a prototype multiple antibiotic-resistant ST313 Salmonella Typhimurium isolate from Malawi (D23580) revealed this to be an unusual Salmonella Typhimurium variant that had undergone genomic degradation, manifested by deletions of portions of the genome and acquisition of multiple pseudogenes. Some of the pseudogenes are homologous to those that exist in Salmonella Typhi or Salmonella Paratyphi A (Parkhill et al.2001; Deng et al.2003; McClelland et al.2004). These findings suggest that Salmonella Typhimurium strains circulating in sub-Saharan Africa have evolved to become genetically more similar to Salmonella Typhi and Salmonella Paratyphi A (Kingsley et al.2009). We have recently shown that Salmonella Typhimurium ST313 strains are also more similar to Salmonella Typhi and Salmonella Paratyphi A phenotypically (Ramachandran et al.2015). We determined that invasive Salmonella Typhimurium ST313 exhibit enhanced survival and replication within primary human and mouse macrophage and macrophage cell lines compared to ST19 strains. Moreover, it has also been shown that Salmonella Typhimurium ST313 strains induce less inflammation resulting in reduced apoptosis of immune cells all of which are characteristics similar to what is observed for Salmonella Typhi rather than gastroenteritis-causing Salmonella Typhimurium (Carden et al. 2015; Ramachandran et al. 2015).
Non-typhoidal Salmonella (NTS) such as Salmonella Typhimurium can often be traced back to contaminated food sources in outbreak situations (Jackson et al.2013). Invasive non-typhoidal Salmonella (iNTS) from sub-Saharan Africa on the other hand are thought to be acquired by human-to-human transmission. Kariuki et al. (2006) followed iNTS cases in Nairobi, Kenya, to their homes and obtained samples from parents and siblings and environmental sources in an effort to identify the reservoir of iNTS. Interestingly, only 3 out of 180 samples from environmental sources had NTS that matched the index case, whereas 21 out of 32 NTS (65.6%) obtained from household contacts had pulsed-field gel electrophoresis profiles indistinguishable from the index case. This suggests that iNTS strains circulating in sub-Saharan Africa are transmitted human to human and may lack mechanisms that allow them to persist in the environment, similarly to Salmonella Typhi.
Salmonella Typhi is difficult to isolate from water and the environment (Sears et al.1984; Lewis et al.2005) and only survives for a limited amount of time outside the host (Cho and Kim 1999). Human carriers are believed to be the main reservoir of Salmonella Typhi and indeed, whole genome sequencing has produced evidence to support this hypothesis. Salmonella Typhi has acquired genetic deletions and loss of gene function which is consistent with a small population size (Holt et al.2008, 2009).
One of the mechanisms that Salmonella Typhimurium uses to survive in the environment is via biofilm formation. A biofilm is a collection of microorganisms that coaggregate and are often embedded within an extracellular matrix composed of DNA, proteins and/or polysaccharides (Peters et al.2012). Cells growing within a biofilm are physiologically distinct from planktonic cells. Salmonella biofilms manifest as red, dry and rough (rdar) colonies on Congo red agar (Romling 2005). The extracellular matrix of these rdar colonies is composed of curli fimbriae (Zogaj et al.2001) and cellulose (Solano et al.2002) and likely contains several other macromolecular complexes. It is hypothesized that the rdar phenotype provides resistance to desiccation and enables bacteria to survive for up to several years (Scher, Romling and Yaron 2005; Apel et al.2009). There is also a correlation between Salmonella biofilm formation and persistence in feed factories (Vestby et al.2009). The large cell surface protein BapA has also been shown to be required for Salmonella Typhimurium biofilm formation (Latasa et al.2005). Other biofilm determinants include the O antigen capsule (Gibson et al.2006), colanic acid (Ledeboer and Jones 2005) and Lpf and Pef (Ledeboer et al.2006).
The goal of this study was to determine whether invasive Salmonella Typhimurium ST313 strains are able to produce a biofilm. We hypothesized that Salmonella Typhimurium ST313 strains which display genomic degradation are, like Salmonella Typhi, unable to form biofilms and this may help to explain why they do not persist in the environment.
METHODS
Bacterial strains
The bacterial strains used in this study are shown in Table 1. Salmonella strains were grown in either animal product-free HY-Soy (HS) media (0.5% Hy-yeast [Sigma-Aldrich, St. Louis, MO], 1% Soytone [TEKNova, Hollister, CA]) or LB media (1% Tryptone [Sigma-Aldrich, St. Louis, MO], 0.5% yeast extract [Sigma-Aldrich, St. Louis, MO], 1% NaCl [Sigma-Aldrich, St. Louis, MO]) at 28°C, 30°C or 37°C as specified in the assay.
Serovar/ST . | Strain . | Source/characteristics . | Reference . |
---|---|---|---|
Typhimurium ST19 | I77 | Blood, Mali | Levy et al.2008; Tennant et al.2010 |
I41 | Blood, Mali | Levy et al.2008; Tennant et al.2010 | |
I89 | Blood, Mali | Levy et al.2008; Tennant et al.2010 | |
S52 | Blood, Mali | Levy et al.2008; Tennant et al.2010 | |
SL1344 | Sequenced reference strain | Hoiseth and Stocker 1981 | |
Typhimurium ST313 | D65 | Blood, Mali | Levy et al.2008; Tennant et al.2010 |
Q55 | Blood, Mali | Levy et al.2008; Tennant et al.2010 | |
S11 | Blood, Mali | Levy et al.2008; Tennant et al.2010 | |
S12 | Blood, Mali | Levy et al.2008; Tennant et al.2010 | |
A13 | Blood, Mali | Levy et al.2008; Tennant et al.2010 | |
D70 | Blood, Mali | Levy et al.2008; Tennant et al.2010 | |
S42 | Blood, Mali | Levy et al.2008; Tennant et al.2010 | |
P142 | Blood, Mali | Levy et al.2008; Tennant et al.2010 | |
P104 | Blood, Mali | Levy et al.2008; Tennant et al.2010 | |
Q65 | Blood, Mali | Levy et al.2008; Tennant et al.2010 | |
S72 | Blood, Mali | Levy et al.2008; Tennant et al.2010 | |
D23580 | Blood, Malawi; sequenced reference strain | Kingsley et al.2009 | |
Typhi | W10 | Blood, Mali | Levy et al.2008; Tennant et al.2010 |
W16 | Blood, Mali | Levy et al.2008; Tennant et al.2010 | |
W35 | Blood, Mali | Levy et al.2008; Tennant et al.2010 | |
W41 | Blood, Mali | Levy et al.2008; Tennant et al.2010 | |
A19 | Blood, Mali | Levy et al.2008; Tennant et al.2010 | |
Ty2 | Wild-type reference strain | Felix and Pitt 1951 | |
Paratyphi A | S39 | Blood, Mali | Levy et al.2008; Tennant et al.2010 |
W30 | Blood, Mali | Levy et al.2008; Tennant et al.2010 | |
ATCC9150 | Wild-type reference strain | American Type Culture Collection, Manassas VA |
Serovar/ST . | Strain . | Source/characteristics . | Reference . |
---|---|---|---|
Typhimurium ST19 | I77 | Blood, Mali | Levy et al.2008; Tennant et al.2010 |
I41 | Blood, Mali | Levy et al.2008; Tennant et al.2010 | |
I89 | Blood, Mali | Levy et al.2008; Tennant et al.2010 | |
S52 | Blood, Mali | Levy et al.2008; Tennant et al.2010 | |
SL1344 | Sequenced reference strain | Hoiseth and Stocker 1981 | |
Typhimurium ST313 | D65 | Blood, Mali | Levy et al.2008; Tennant et al.2010 |
Q55 | Blood, Mali | Levy et al.2008; Tennant et al.2010 | |
S11 | Blood, Mali | Levy et al.2008; Tennant et al.2010 | |
S12 | Blood, Mali | Levy et al.2008; Tennant et al.2010 | |
A13 | Blood, Mali | Levy et al.2008; Tennant et al.2010 | |
D70 | Blood, Mali | Levy et al.2008; Tennant et al.2010 | |
S42 | Blood, Mali | Levy et al.2008; Tennant et al.2010 | |
P142 | Blood, Mali | Levy et al.2008; Tennant et al.2010 | |
P104 | Blood, Mali | Levy et al.2008; Tennant et al.2010 | |
Q65 | Blood, Mali | Levy et al.2008; Tennant et al.2010 | |
S72 | Blood, Mali | Levy et al.2008; Tennant et al.2010 | |
D23580 | Blood, Malawi; sequenced reference strain | Kingsley et al.2009 | |
Typhi | W10 | Blood, Mali | Levy et al.2008; Tennant et al.2010 |
W16 | Blood, Mali | Levy et al.2008; Tennant et al.2010 | |
W35 | Blood, Mali | Levy et al.2008; Tennant et al.2010 | |
W41 | Blood, Mali | Levy et al.2008; Tennant et al.2010 | |
A19 | Blood, Mali | Levy et al.2008; Tennant et al.2010 | |
Ty2 | Wild-type reference strain | Felix and Pitt 1951 | |
Paratyphi A | S39 | Blood, Mali | Levy et al.2008; Tennant et al.2010 |
W30 | Blood, Mali | Levy et al.2008; Tennant et al.2010 | |
ATCC9150 | Wild-type reference strain | American Type Culture Collection, Manassas VA |
Serovar/ST . | Strain . | Source/characteristics . | Reference . |
---|---|---|---|
Typhimurium ST19 | I77 | Blood, Mali | Levy et al.2008; Tennant et al.2010 |
I41 | Blood, Mali | Levy et al.2008; Tennant et al.2010 | |
I89 | Blood, Mali | Levy et al.2008; Tennant et al.2010 | |
S52 | Blood, Mali | Levy et al.2008; Tennant et al.2010 | |
SL1344 | Sequenced reference strain | Hoiseth and Stocker 1981 | |
Typhimurium ST313 | D65 | Blood, Mali | Levy et al.2008; Tennant et al.2010 |
Q55 | Blood, Mali | Levy et al.2008; Tennant et al.2010 | |
S11 | Blood, Mali | Levy et al.2008; Tennant et al.2010 | |
S12 | Blood, Mali | Levy et al.2008; Tennant et al.2010 | |
A13 | Blood, Mali | Levy et al.2008; Tennant et al.2010 | |
D70 | Blood, Mali | Levy et al.2008; Tennant et al.2010 | |
S42 | Blood, Mali | Levy et al.2008; Tennant et al.2010 | |
P142 | Blood, Mali | Levy et al.2008; Tennant et al.2010 | |
P104 | Blood, Mali | Levy et al.2008; Tennant et al.2010 | |
Q65 | Blood, Mali | Levy et al.2008; Tennant et al.2010 | |
S72 | Blood, Mali | Levy et al.2008; Tennant et al.2010 | |
D23580 | Blood, Malawi; sequenced reference strain | Kingsley et al.2009 | |
Typhi | W10 | Blood, Mali | Levy et al.2008; Tennant et al.2010 |
W16 | Blood, Mali | Levy et al.2008; Tennant et al.2010 | |
W35 | Blood, Mali | Levy et al.2008; Tennant et al.2010 | |
W41 | Blood, Mali | Levy et al.2008; Tennant et al.2010 | |
A19 | Blood, Mali | Levy et al.2008; Tennant et al.2010 | |
Ty2 | Wild-type reference strain | Felix and Pitt 1951 | |
Paratyphi A | S39 | Blood, Mali | Levy et al.2008; Tennant et al.2010 |
W30 | Blood, Mali | Levy et al.2008; Tennant et al.2010 | |
ATCC9150 | Wild-type reference strain | American Type Culture Collection, Manassas VA |
Serovar/ST . | Strain . | Source/characteristics . | Reference . |
---|---|---|---|
Typhimurium ST19 | I77 | Blood, Mali | Levy et al.2008; Tennant et al.2010 |
I41 | Blood, Mali | Levy et al.2008; Tennant et al.2010 | |
I89 | Blood, Mali | Levy et al.2008; Tennant et al.2010 | |
S52 | Blood, Mali | Levy et al.2008; Tennant et al.2010 | |
SL1344 | Sequenced reference strain | Hoiseth and Stocker 1981 | |
Typhimurium ST313 | D65 | Blood, Mali | Levy et al.2008; Tennant et al.2010 |
Q55 | Blood, Mali | Levy et al.2008; Tennant et al.2010 | |
S11 | Blood, Mali | Levy et al.2008; Tennant et al.2010 | |
S12 | Blood, Mali | Levy et al.2008; Tennant et al.2010 | |
A13 | Blood, Mali | Levy et al.2008; Tennant et al.2010 | |
D70 | Blood, Mali | Levy et al.2008; Tennant et al.2010 | |
S42 | Blood, Mali | Levy et al.2008; Tennant et al.2010 | |
P142 | Blood, Mali | Levy et al.2008; Tennant et al.2010 | |
P104 | Blood, Mali | Levy et al.2008; Tennant et al.2010 | |
Q65 | Blood, Mali | Levy et al.2008; Tennant et al.2010 | |
S72 | Blood, Mali | Levy et al.2008; Tennant et al.2010 | |
D23580 | Blood, Malawi; sequenced reference strain | Kingsley et al.2009 | |
Typhi | W10 | Blood, Mali | Levy et al.2008; Tennant et al.2010 |
W16 | Blood, Mali | Levy et al.2008; Tennant et al.2010 | |
W35 | Blood, Mali | Levy et al.2008; Tennant et al.2010 | |
W41 | Blood, Mali | Levy et al.2008; Tennant et al.2010 | |
A19 | Blood, Mali | Levy et al.2008; Tennant et al.2010 | |
Ty2 | Wild-type reference strain | Felix and Pitt 1951 | |
Paratyphi A | S39 | Blood, Mali | Levy et al.2008; Tennant et al.2010 |
W30 | Blood, Mali | Levy et al.2008; Tennant et al.2010 | |
ATCC9150 | Wild-type reference strain | American Type Culture Collection, Manassas VA |
Salmonella morphotype analysis
This assay determines the multicellular status of bacterial cells expressing the extracellular matrix components curli and cellulose. Salmonella strains were adjusted to an OD600 of 3.0, and 5 μl of bacterial suspension was dropped onto LB agar lacking NaCl and supplemented with 40 μg/mL Congo red [Fishers Scientific, Pittsburgh, PA] and 20 μg/mL Coomassie Brilliant Blue G-250 [Bio-Rad, Hercules, CA]. Plates were incubated at 28°C for 24–48 h or up to 8 days.
Pellicle formation
The mat model measures biofilm production on the air–liquid interface. Salmonella strains were grown in 3 mL of LB medium without NaCl in glass test tubes at 28°C for 5 days without shaking. To more easily visualize the biofilm that forms at the liquid–air interface (Latasa et al.2012), the liquid was carefully poured off and stained with 1% crystal violet [Fluka BioChemika, Buchs, Switzerland].
Crystal violet assay
This is a static biofilm model which measures biofilm formation on hydrophobic abiotic surfaces. Crystal violet biofilm assays were performed essentially as described by Baugh et al. (2012). Briefly, 200 μl bacterial suspension (in LB or HS lacking NaCl) was grown in 96-well polystyrene trays for 48 h at 30°C with gentle agitation. Wells were washed with water and the biofilm was stained using 1% crystal violet. Bound crystal violet was solubilized in 70% ethanol and the OD600 was measured.
Continuous flow system
This model measures biofilms that are produced in continuously flowing liquid. The reactor system was constructed within a 37°C incubator and consisted of silicon tubing through which 0.1× LB media (pH 5.0 to 6.5) flowed under the control of a peristaltic pump into a waste container as previously described (Brady et al.2006; O'May et al.2009). This system incorporated square cross-section glass flow cells for use in confocal microscopy studies. Prior to inoculation, 0.1× LB was pumped through the system and allowed to equilibrate to temperature for 24 h. An overnight culture of Salmonella Typhimurium grown in LB was diluted 1:100 into fresh LB and allowed to grow at 37°C with shaking for 2 h. The tubing was clamped upstream of the injection ports and 1.0 mL of exponential phase bacterial culture injected. The system was incubated without flow for 20 min at either 28°C or 37°C so that bacteria could adhere to the luminal surface. The media flow was restored to a flow rate of 0.5 mL/min at 28°C or 37°C for 10 days. Biofilm was harvested by removal of the square glass flow cells for confocal microscopy.
Long-term survival and sodium hypochlorite treatment
Long-term survival and sodium hypochlorite treatment were performed as previously described (White et al.2006). Bacterial cells from 1 μl of overnight cultures were inoculated onto T agar [1% Tryptone, 1.5% agar] plates and grown at room temperature (25°C) or 28°C for 6 days. Colonies were removed from the surface of the plates and tested to determine the number of colony-forming units (CFU) within each colony. Another set of colonies were removed from the surface of the T agar and stored in 24-well plates (one colony/well) for 1 month at room temperature (25°C) or 28°C. The colonies were then rehydrated in 500 μl of PBS. 100 μl of 60 parts per million (ppm) sodium hypochlorite or PBS was added and the cell suspension mixed gently on a microcentrifuge rotating platform for 20 min at room temperature. Once the supernatant was removed, 500 μl of fresh PBS was added and the colonies were disrupted using a tissue homogenizer [Omni International, NW Kennesaw, GA] until the colonies were broken down evenly. For planktonic cells, overnight cultures in 1% Tryptone [Sigma-Aldrich, St. Louis, MO] were normalized to an OD600 of 1.0 and treated exactly as per the colonies grown on T agar but without the homogenization. Bacterial suspensions were serially diluted and plated on HS agar plates to determine CFU.
Alternatively, overnight planktonic cultures of Salmonella Typhimurium were diluted to an optical density (600 nm, 1-cm path length) of 0.200 in PBS. Three 5 μl drops (for long-term survival or sodium hypochlorite susceptibility) of diluted planktonic culture were used to inoculate individual sterile, black, polycarbonate membrane filters (diameter, 25 mm; pore size, 0.2 μm; Poretics Corp., Livermore, CA) resting on HS agar plates. The membranes were sterilized by UV exposure (15 min per side) prior to inoculation. The plates were inverted and incubated at 28°C for 6 days, to form membrane-supported biofilms on the surface of the membranes. Membranes were removed from the surface of the plates and tested to determine the number of CFUs within each colony by disrupting the membrane using a tissue homogenizer. Another set of membranes were removed from the surface of the HS agar and stored in 12-well plates (one membrane/well) for 1 month at 28°C. The membranes were then rehydrated in 500 μl of PBS. 100 μl of 60 parts per million (ppm) sodium hypochlorite or PBS was added and the cell suspension mixed gently on a microcentrifuge rotating platform for 20 min at room temperature. Once the supernatant was removed, 500 μl of fresh PBS was added and the colonies were disrupted using a tissue homogenizer until the membranes were broken down evenly. For testing planktonic cells, the procedure was the same as described above. Bacterial suspensions were serially diluted and plated on HS agar plates to determine CFU.
Statistical analysis
Student's t-test or Mann–Whitney test was performed using Prism software [GraphPad Software]. A two-tailed P-value of <0.05 was taken to indicate statistical significance.
RESULTS
Salmonella morphotype
We tested the ability of Salmonella Typhimurium ST19 and ST313 strains isolated from the blood of children in Mali to produce the rdar phenotype on media containing Congo red at room temperature for 8 days. As a positive control, we used Salmonella Typhimurium SL1344 (ST19) and as negative controls, we used Salmonella Typhi Ty2 and Salmonella Paratyphi A ATCC9150. We also tested several clinical Salmonella Typhi and Salmonella Paratyphi A strains from Mali. As shown in Fig. 1 and Table 2, only the Salmonella Typhimurium ST19 strains produced a strong rdar morphotype. Interestingly, we did not observe the rdar morphotype for any of the Salmonella Typhimurium ST313 strains tested. The Salmonella Typhimurium ST313 strains appeared to be an intermediate between the rdar and the smooth and pale brown morphotype. The morphotype of Salmonella Typhimurium ST313 strains appeared to be more brown and smooth (bas).

rdar phenotype of Salmonella. (A) Clinical Salmonella Typhimurium ST19 and ST313, Salmonella Typhi and Salmonella Paratyphi A isolated from the blood of children in Bamako, Mali, West Africa. Reference strains Salmonella Typhimurium SL1344, Salmonella Typhi Ty2 and Salmonella Paratyphi A ATCC 9150 were included for comparison. (B) Close up images of Salmonella Typhimurium I41 (ST19) and Salmonella Typhimurium S11 (ST313).
Collated biofilm assay results of invasive Salmonella tested in this study.
. | . | Crystal violet assay . | . | . | |
---|---|---|---|---|---|
. | . | (OD600) . | . | . | |
Serovar . | Strain . | Mean . | SD . | rdar Phenotypea . | Pellicle formationb . |
Typhimurium ST19 | I77 | 0.86 | 0.20 | + + + | + + + |
I89 | 1.30 | 0.65 | + + + | + + + | |
I41 | 0.71 | 0.22 | + + + | + + + | |
S52 | 1.56 | 0.28 | + + + | + + + | |
SL1344 | 0.58 | 0.21 | + + + | + + + | |
Typhimurium ST313 | Q55 | 0.35 | 0.18 | + | + |
D65 | 0.27 | 0.14 | + | + + | |
S11 | 0.31 | 0.10 | + | + | |
S12 | 0.33 | 0.21 | + | + | |
A13 | 0.30 | 0.14 | + | + | |
D70 | 0.31 | 0.14 | + | + | |
S42 | 0.22 | 0.14 | + | + + | |
P142 | 0.24 | 0.10 | + | + | |
P104 | 0.22 | 0.15 | + | + | |
Q65 | 0.16 | 0.13 | + | + | |
S72 | 0.21 | 0.20 | + | + + | |
Typhi | W10 | 0.96 | 0.67 | – | + |
W16 | 0.29 | 0.21 | – | + | |
W35 | 0.28 | 0.14 | – | + + | |
W41 | 0.32 | 0.11 | – | + + | |
A19 | 0.24 | 0.20 | – | + | |
Ty2 | 1.45 | 0.93 | – | + | |
Paratyphi A | S39 | 0.37 | 0.18 | – | + |
W30 | 0.27 | 0.15 | – | + | |
ATCC 9150 | 0.50 | 0.24 | – | + + |
. | . | Crystal violet assay . | . | . | |
---|---|---|---|---|---|
. | . | (OD600) . | . | . | |
Serovar . | Strain . | Mean . | SD . | rdar Phenotypea . | Pellicle formationb . |
Typhimurium ST19 | I77 | 0.86 | 0.20 | + + + | + + + |
I89 | 1.30 | 0.65 | + + + | + + + | |
I41 | 0.71 | 0.22 | + + + | + + + | |
S52 | 1.56 | 0.28 | + + + | + + + | |
SL1344 | 0.58 | 0.21 | + + + | + + + | |
Typhimurium ST313 | Q55 | 0.35 | 0.18 | + | + |
D65 | 0.27 | 0.14 | + | + + | |
S11 | 0.31 | 0.10 | + | + | |
S12 | 0.33 | 0.21 | + | + | |
A13 | 0.30 | 0.14 | + | + | |
D70 | 0.31 | 0.14 | + | + | |
S42 | 0.22 | 0.14 | + | + + | |
P142 | 0.24 | 0.10 | + | + | |
P104 | 0.22 | 0.15 | + | + | |
Q65 | 0.16 | 0.13 | + | + | |
S72 | 0.21 | 0.20 | + | + + | |
Typhi | W10 | 0.96 | 0.67 | – | + |
W16 | 0.29 | 0.21 | – | + | |
W35 | 0.28 | 0.14 | – | + + | |
W41 | 0.32 | 0.11 | – | + + | |
A19 | 0.24 | 0.20 | – | + | |
Ty2 | 1.45 | 0.93 | – | + | |
Paratyphi A | S39 | 0.37 | 0.18 | – | + |
W30 | 0.27 | 0.15 | – | + | |
ATCC 9150 | 0.50 | 0.24 | – | + + |
rdar phenotype; +++, rdar morphology; ++, orange-rdar morphology; +, bas morphology; −, smooth and pale brown morphology.
Pellicle formation; +++, strong pellicle; ++, moderate pellicle; + weak pellicle.
Collated biofilm assay results of invasive Salmonella tested in this study.
. | . | Crystal violet assay . | . | . | |
---|---|---|---|---|---|
. | . | (OD600) . | . | . | |
Serovar . | Strain . | Mean . | SD . | rdar Phenotypea . | Pellicle formationb . |
Typhimurium ST19 | I77 | 0.86 | 0.20 | + + + | + + + |
I89 | 1.30 | 0.65 | + + + | + + + | |
I41 | 0.71 | 0.22 | + + + | + + + | |
S52 | 1.56 | 0.28 | + + + | + + + | |
SL1344 | 0.58 | 0.21 | + + + | + + + | |
Typhimurium ST313 | Q55 | 0.35 | 0.18 | + | + |
D65 | 0.27 | 0.14 | + | + + | |
S11 | 0.31 | 0.10 | + | + | |
S12 | 0.33 | 0.21 | + | + | |
A13 | 0.30 | 0.14 | + | + | |
D70 | 0.31 | 0.14 | + | + | |
S42 | 0.22 | 0.14 | + | + + | |
P142 | 0.24 | 0.10 | + | + | |
P104 | 0.22 | 0.15 | + | + | |
Q65 | 0.16 | 0.13 | + | + | |
S72 | 0.21 | 0.20 | + | + + | |
Typhi | W10 | 0.96 | 0.67 | – | + |
W16 | 0.29 | 0.21 | – | + | |
W35 | 0.28 | 0.14 | – | + + | |
W41 | 0.32 | 0.11 | – | + + | |
A19 | 0.24 | 0.20 | – | + | |
Ty2 | 1.45 | 0.93 | – | + | |
Paratyphi A | S39 | 0.37 | 0.18 | – | + |
W30 | 0.27 | 0.15 | – | + | |
ATCC 9150 | 0.50 | 0.24 | – | + + |
. | . | Crystal violet assay . | . | . | |
---|---|---|---|---|---|
. | . | (OD600) . | . | . | |
Serovar . | Strain . | Mean . | SD . | rdar Phenotypea . | Pellicle formationb . |
Typhimurium ST19 | I77 | 0.86 | 0.20 | + + + | + + + |
I89 | 1.30 | 0.65 | + + + | + + + | |
I41 | 0.71 | 0.22 | + + + | + + + | |
S52 | 1.56 | 0.28 | + + + | + + + | |
SL1344 | 0.58 | 0.21 | + + + | + + + | |
Typhimurium ST313 | Q55 | 0.35 | 0.18 | + | + |
D65 | 0.27 | 0.14 | + | + + | |
S11 | 0.31 | 0.10 | + | + | |
S12 | 0.33 | 0.21 | + | + | |
A13 | 0.30 | 0.14 | + | + | |
D70 | 0.31 | 0.14 | + | + | |
S42 | 0.22 | 0.14 | + | + + | |
P142 | 0.24 | 0.10 | + | + | |
P104 | 0.22 | 0.15 | + | + | |
Q65 | 0.16 | 0.13 | + | + | |
S72 | 0.21 | 0.20 | + | + + | |
Typhi | W10 | 0.96 | 0.67 | – | + |
W16 | 0.29 | 0.21 | – | + | |
W35 | 0.28 | 0.14 | – | + + | |
W41 | 0.32 | 0.11 | – | + + | |
A19 | 0.24 | 0.20 | – | + | |
Ty2 | 1.45 | 0.93 | – | + | |
Paratyphi A | S39 | 0.37 | 0.18 | – | + |
W30 | 0.27 | 0.15 | – | + | |
ATCC 9150 | 0.50 | 0.24 | – | + + |
rdar phenotype; +++, rdar morphology; ++, orange-rdar morphology; +, bas morphology; −, smooth and pale brown morphology.
Pellicle formation; +++, strong pellicle; ++, moderate pellicle; + weak pellicle.
Pellicle formation
We examined the pellicle that forms at the liquid–air interface of four Salmonella Typhimurium ST19 and four Salmonella Typhimurium ST313 strains grown in bacteriological medium. As shown in Fig. 2, a pellicle is clearly observed for the Salmonella Typhimurium ST19 strains but not for the ST313 isolates.
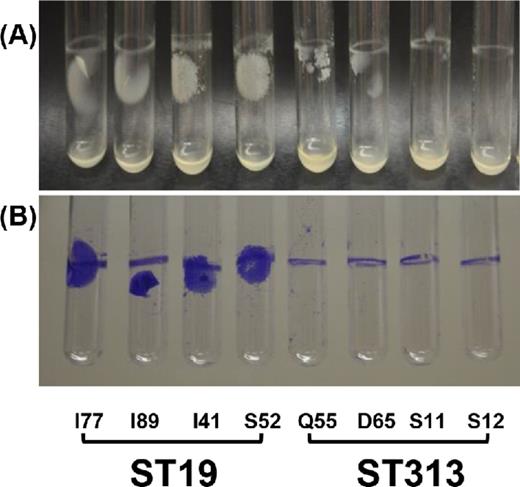
Pellicle formation at the liquid–air interface of invasive Salmonella Typhimurium ST19 and ST313 strains from Mali, West Africa.
Crystal violet assay
We tested 11 Salmonella Typhimurium ST313 and 5 Salmonella Typhimurium ST19 (including SL1344) in the crystal violet biofilm assay (at least three independent assays). As shown in Fig. 3, all Salmonella Typhimurium ST19 strains tested made significantly more biofilm than ST313 isolates (P < 0.001). Table 2 summarizes the biofilm-forming ability of each of the strains tested.
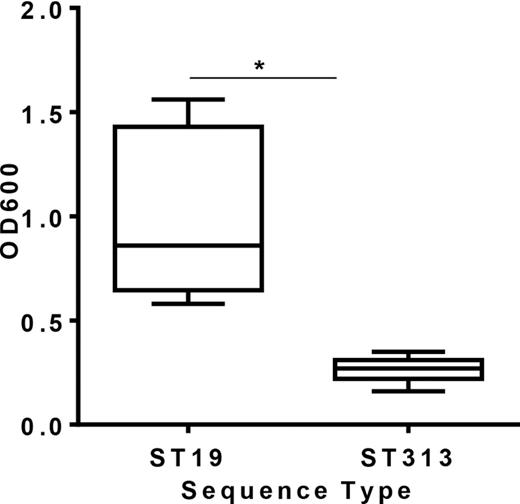
Biofilm production of 5 Salmonella Typhimurium ST19 and 11 ST313 strains as measured by crystal violet binding. Data are expressed as a box and whisker plot with whiskers representing the minimum and maximum value. The assay is representative of three independent experiments carried out in triplicates. Asterisk represents P < 0.05.
Continuous flow system
In order to test if the observed differences in biofilm formation between Salmonella Typhimurium ST19 and ST313 were consistent following a longer period of incubation, we carried out a continuous flow assay over a period of 10 days at 37°C (physiological temperature) and also at 28°C (the average environmental temperature in Mali). At both 37°C and 28°C, we confirmed that Salmonella Typhimurium I77 (ST19) was able to produce a thicker biofilm in a continuous flow cell (Fig. 4A, Supplementary Video 1A, Supporting Information). In contrast to strain I77, Salmonella Typhimurium D65 (ST313) showed only discrete clumps of cells when grown under continuous flow conditions (Fig. 4A, Supplementary Video 1B, Supporting Information). We found over 60% coverage of Salmonella Typhimurium I77 (ST19) biofilm on a linear segment of 240 μm of the glass slide when the bacteria were allowed to form a biofilm at 37°C. Less than 6% coverage was observed for Salmonella Typhimurium D65 (ST313) at the same temperature (Fig. 4B). We observed close to 80% coverage for Salmonella Typhimurium I77 (ST19) when grown at 28°C the average temperature in Mali, whereas less than 4% coverage was observed for Salmonella Typhimurium D65 (ST313) (Fig. 4B). The thickness of the Salmonella Typhimurium I77 (ST19) biofilm at 37°C was observed to be 18 μm, whereas no thickness was observed for the Salmonella Typhimurium D65 (ST313) (Fig. 4C). The thickness of Salmonella Typhimurium I77 (ST19) biofilm was significantly reduced to half (9 μm) when grown at 28°C (Fig. 4C). However, there was still a significantly increased thickness of Salmonella Typhimurium I77 (ST19) biofilm compared to Salmonella Typhimurium D65 (ST313) biofilm, where thickness was not measurable.
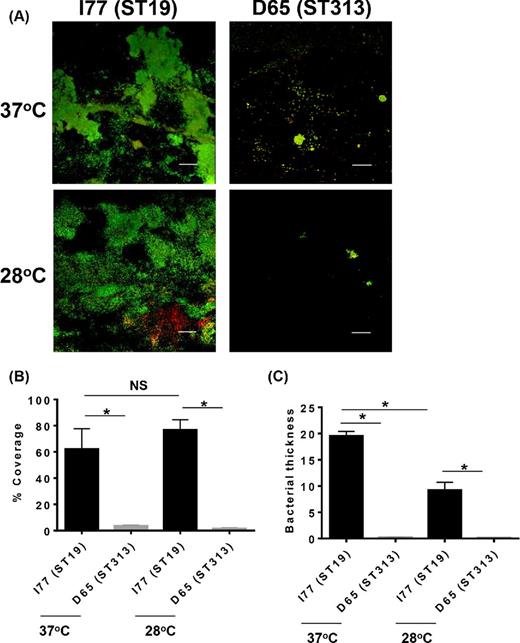
Biofilm formation of (A) Salmonella Typhimurium D65 (ST313) and Salmonella Typhimurium I77 (ST19) grown in continuous flow cells. The white bar on the figure represents 50 μm. (B) Percent coverage of the biofilms determined by viewing 10 different fields of view. (C) The average biofilm thickness on the surface of the glass in μm. Asterisk represents P < 0.05.
Resistance to desiccation and sodium hypochlorite treatment
To determine if the biofilm-producing Salmonella Typhimurium ST19 were more resistant to environmental conditions, we subjected these rdar and bas strains to desiccation at 25°C (room temperature) and 28°C (average environmental temperature in Mali) and sodium hypochlorite treatment. After 1 month of desiccation at 25°C without exogenous nutrients on 24-well plastic plates, the percent survival for the bas Salmonella Typhimurium (ST313) D65, Q55, S11 and S12 strains was found to be 13.1 ± 9.6% (mean ± SD) at 25°C. In comparison, at 25°C there was a significant increase in the percent survival for Salmonella Typhimurium ST19 strains I77, I41, S52 and I89 (59.7 ± 12.3%, P < 0.05) (Fig. 5A). We found a similar trend in the long-term survival for the Salmonella Typhimurium ST19 and ST313 strains at 28°C. We also examined the long-term survival of the rdar ST19 and the bas ST313 strains post desiccation at 25°C and 28°C when allowed to form a biofilm on a polycarbonate membrane filter. We observed similar results to storage on plastic with ST19 strains able to survive desiccation significantly more than ST313 strains at both temperatures (Fig. 5B).

Long-term survival of four Salmonella Typhimurium ST19 and four ST313 desiccated colonies. (A) Survival of cells from 1-month-old colonies on plastic rehydrated with PBS and counted for CFU. (B) Survival of 1-month-old biofilm on polycarbonate membrane filters rehydrated with PBS and counted for CFU. (C) Cells from 1-month-old colonies of Salmonella Typhimurium ST19 and ST313 on plastic were rehydrated with PBS and treated with 60 ppm sodium hypochlorite. (D) Survival of 1-month-old biofilm of Salmonella Typhimurium ST19 and ST313 on polycarbonate membrane filters rehydrated with PBS and treated with 60 ppm sodium hypochlorite. Bars represent average percent survival (CFU after treatment/CFU before treatment × 100) for four different strains for each ST. Asterisk represents P < 0.05.
We further examined the resistance of the rdar-forming Salmonella Typhimurium ST19 strains to sodium hypochlorite following desiccation compared to their planktonic state and the bas-forming Salmonella Typhimurium ST313 strains. We observed that the planktonic cultures of both Salmonella Typhimurium ST19 and ST313 were highly sensitive to sodium hypochlorite treatment. The rdar-forming desiccated Salmonella Typhimurium ST19 strains were found to be more resistant to 60 ppm sodium hypochlorite treatment compared to the bas Salmonella Typhimurium ST313 strains. The percent survival for Salmonella Typhimurium (ST313) D65, Q55, S11 and S12 was 23.5 ± 5.1% (mean ± SD), whereas the percent survival for Salmonella Typhimurium (ST19) I77, I41, S52 and I89 was 70.2 ± 7.7% following sodium hypochlorite treatment for 20 min (Fig. 5C). We also studied the resistance of Salmonella Typhimurium ST19 and ST313 biofilms on polycarbonate membrane filters to sodium hypochlorite treatment. Similar to what we observed above, 1-month old Salmonella Typhimurium ST19 biofilms were resistant to sodium hypochlorite treatment (Fig. 5D). The planktonic cultures for both Salmonella Typhimurium ST19 and ST313 were easily killed following exposure to sodium hypochlorite.
DISCUSSION
Multicellular behavior in Salmonella Typhimurium is characterized by the rdar morphotype. Salmonella Typhimurium morphotypes on Congo red plates can be distinguished by the formation of rdar (both curli fimbriae and cellulose expressed), bdar (curli fimbriae expressed but no cellulose), pdar (cellulose expressed but no curli fimbriae) and saw (no curli fimbriae or cellulose) (Romling et al.2003). Our rdar results support those of Romling et al. (2003), who found that greater than 90% of Salmonella Typhimurium and Salmonella Enteritidis strains isolated from humans, food and animals expressed the rdar phenotype, whereas invasive serovars such as Salmonella Typhi, Salmonella Choleraesuis and Salmonella Typhimurium var Copenhagen (causes septicemia in pigeons) do not produce rdar colonies (Romling et al.2003). The invasive Salmonella Typhimurium ST313 isolates from Mali were unable to form the rdar morphotype and appeared to be phenotypically more similar to Salmonella Typhi and Salmonella Paratyphi A. Our results corroborate those of a recently published study which showed that Salmonella Typhimurium D23580 (ST313) was unable to form the rdar morphotype (Singletary et al.2016). Salmonella Typhimurium D23580 has an amino acid change in CsgE, a single nucleotide mutation in the bcsC gene and a premature stop codon in bcsG (Singletary et al.2016). The bcsC and bcsG genes, part of the bcsABZC and bcsEFG operons, are required for the biosynthesis of cellulose and csgE is necessary for curli fimbriae production (Solano et al.2002).
We examined the biofilm-forming ability of Salmonella Typhimurium ST19 and ST313 clinical isolates in a crystal violet assay and a continuous flow system. We tested the ability of these strains to form biofilms at both room temperature (∼25°C) and 28°C which is the average environmental temperature in Mali. In both assays, we observed significantly enhanced biofilm formation by all the strains of Salmonella Typhimurium ST19 irrespective of the temperature. Interestingly, none of the Salmonella Typhimurium ST313 strains tested were able to produce any biofilm in the continuous flow system and they formed a very weak biofilm in the 96-well plate crystal violet assay. In both these assays, the initial attachment of the bacteria to the surface is a key step in its ability to form a biofilm. Flagella expression and motility has been shown to promote the early binding of various bacteria to surfaces during biofilm formation on different biotic and abiotic surfaces (O'Toole and Kolter 1998). Mutations in the sirA gene that regulates flagellar expression genes and motility lead to diminished biofilm formation in the crystal violet assay (Teplitski, Al-Agely and Ahmer 2006). We have previously shown that Salmonella Typhimurium ST313 clinical strains from Mali are severely attenuated in flagella expression and motility compared to the Salmonella Typhimurium ST19 strains (Ramachandran et al.2015). This may partly account for the reduced biofilm-forming ability of Salmonella Typhimurium ST313.
We speculated that since the rdar phenotype has been shown to allow Salmonella Typhimurium to persist on environmental surfaces outside the human host, then the absence of this characteristic implies that Salmonella Typhimurium ST313 strains do not persist in the environment for long periods of time. Instead, we hypothesized that Salmonella Typhimurium ST313 strains, like Salmonella Typhi, are transmitted between human hosts, making cellular functions related primarily to environmental persistence dispensable. Indeed, data from Kenya suggest human-to-human transmission to be the case (Kariuki et al.2006).
Long-term survival post desiccation in the absence of exogenous nutrients and resistance to sodium hypochlorite treatment were markedly reduced for Salmonella Typhimurium ST313 compared to ST19 at both 25°C and 28°C. The rdar morphotype has shown to be important for the long-term survival of Salmonella Typhimurium (White et al.2006). Production of thin aggregated fimbriae (Tafi) and cellulose are important characteristics of the rdar morphotype. Salmonella Typhimurium ΔagfA (deficient in Tafi production) and Salmonella Typhimurium ΔagfD (deficient in both Tafi and cellulose production) were highly sensitive to desiccation and sodium hypochlorite treatment compared to wild-type Salmonella Typhimurium (White et al. 2006). The presence of the rdar morphotype and the ability of Salmonella Typhimurium ST19 strains to survive over a long period in the absence of nutrients might provide an advantage to this ST to persist in harsh environmental conditions over an extended period of time compared to ST313 strains. Our observations were also consistent with other studies that show that strains that produce the rdar morphotype and a pellicle in the liquid–air interface in standing liquid culture were highly resistant to treatment by chlorination (Scher, Romling and Yaron 2005; White et al. 2006). Two recent studies showed that clinical strains of Salmonella Typhimurium ST313 (D23580 and A130) from Malawi are weak catalase producers when treated with hydrogen peroxide (Yang et al. 2015; Singletary et al. 2016). The reduced catalase expression was attributed to single amino acid change in the katE gene, a gene that is part of the RpoS regulon (Singletary et al. 2016). Salmonella Typhi Ty2 that is naturally defective for rpoS is deficient in its ability to produce catalase. RpoS is a master regulon that has shown to be important in positively regulating curli genes, csgA and csgAB, that are important in biofilm formation and resistance to oxidative stress in Salmonella Typhimurium (Romling et al. 1998). We know that all the clinical Salmonella Typhimurium ST19 and ST313 strains from Mali that we have tested harbor the rpos gene (data not shown). It would be interesting to investigate any possible differences in the regulation of rpoS between Salmonella Typhimurium ST19 and ST313 in future studies.
There are a variety of in vitro growth models that are used to study biofilms and each emphasizes different aspects of the biofilm mode of growth. For example, the crystal violet assay is a static model that identifies those factors responsible for biofilm initiation and extracellular polysaccharide but does not allow for the formation of mature biofilms (O'Toole 2011). In contrast, flow cell systems allow for a continuous nutrient feed, removal of waste and quorum-sensing products, and support mature biofilm formation (Brady et al.2006). Lastly, the membrane biofilm model allows for an air–liquid interface (Stewart and Franklin 2008). In order to test the veracity of our results, we used each of these models to test for biofilm-forming ability of Salmonella Typhimurium ST19 and ST313 strains. Indeed, we observed that the ST19 strains are consistently better biofilm producers than ST313 strains. Our study demonstrates the importance of the rdar morphotype and the biofilm-forming ability of Salmonella Typhimurium ST19 strains in their ability to survive for long periods under harsh conditions in absence of exogenous nutrients compared to Salmonella Typhimurium ST313 strains. Our data suggest that like Salmonella Typhi, Salmonella Typhimurium ST313 lack mechanisms that allow it to persist in the environment and instead may be transmitted human to human.
SUPPLEMENTARY DATA
The authors would like to thank Dr Robert Ernst for the gift of Salmonella Typhimurium SL1344 and Dr Robert Heyderman for the gift of Salmonella Typhimurium D23580.
AUTHOR CONTRIBUTIONS
Conceived and designed the experiments: SMT GR MES. Performed the experiments: GR KA MES. Analyzed the data: GR SMT MES. Wrote the manuscript: GR SMT.
FUNDING
This work was supported by a Career Development Award (Tennant) funded by the Mid-Atlantic Regional Center of Excellence, National Institute of Allergy and Infectious Diseases/National Institutes of Health 2 U54 AI057168 grant (MyronM. Levine, Principal Investigator).
Conflict of interest. None declared.
REFERENCES