-
PDF
- Split View
-
Views
-
Cite
Cite
Xinyang Li, Yingying Xing, Le Guo, Xiaobo Lv, Hui Song, Tao Xi, Oral immunization with recombinant Lactococcus lactis delivering a multi-epitope antigen CTB-UE attenuates Helicobacter pylori infection in mice, Pathogens and Disease, Volume 72, Issue 1, October 2014, Pages 78–86, https://doi.org/10.1111/2049-632X.12173
- Share Icon Share
Abstract
Urease is an essential virulence factor and colonization factor for Helicobacter pylori (H. pylori) and is considered as an excellent vaccine candidate antigen. However, conventional technologies for preparing an injectable vaccine require purification of the antigenic protein and preparation of an adjuvant. Lactococcus lactis NZ9000 (L. lactis) could serve as an antigen-delivering vehicle for the development of edible vaccine. In previous study, we constructed a multi-epitope vaccine, designated CTB-UE, which is composed of the mucosal adjuvant cholera toxin B subunit (CTB), three Th cell epitopes and two B-cell epitopes from urease subunits. To develop a novel type of oral vaccine against H. pylori, genetically modified L. lactis strains were established to secrete this epitope vaccine extracellularly in this study. Oral prophylactic immunization with recombinant L. lactis significantly elicited humoral anti-urease antibody responses (P < 0.001) and reduced the gastric colonization of H. pylori from 7.14 ± 0.95 to 4.68 ± 0.98 log10 CFU g−1 stomach. This L. lactis oral vaccine offers a promising vaccine candidate for the control of H. pylori infection.

This article reports that Lactococcus lactis secretory expression systems were established to deliver a novel type of multi epitope vaccine CTB UE which could attenuate Helicobacter pylori infection significantly.
Introduction
Helicobacter pylori (H. pylori) is a spiral shaped, microaerophilic, Gram negative bacterium that colonizes the human gastric and duodenal mucosa, where it induces chronic gastritis and peptic ulcer (Blaser, 1990). More than 50% of the population worldwide is infected with this pathogenic bacterium, with a higher prevalence in the developing countries (Dunn et al., 1997; Brown, 2000). Unfortunately, current treatments that are based on a combination of at least two antibiotics and a proton pump inhibitor are not only costly but are also associated with a number of problems such as poor patient compliance, increased antibiotic resistance, and reinfection (Cameron & Bell, 2005). Consequently, it is feasible to develop an effective prophylactic vaccine which will be of enormous scientific value and economic benefits.
Epitope vaccine based on H. pylori urease is a new strategy for prophylactic and therapeutic vaccination against H. pylori infection, avoiding some crucial drawbacks to the current procedures for vaccine preparation, such as the difficulty of the in vitro culturing of H. pylori, the loss of efficacy due to the genetic variation in the pathogen, as well as the side effects of other unfavorable epitopes in the complete antigen (Sette & Fikes, 2003; Zhou et al., 2009). Therefore, epitope vaccine may be effective to control H. pylori infection. In our previous study, we designed a multi epitope vaccine CTB UE (CUE, Guo et al., 2014) based on cholera toxin B subunit (CTB) and the identified and characterized five cell epitopes from UreA (UreA74–79 and UreA183–203) and UreB (UreB229–244, UreB237–251 and UreB327–334). It has been reported that these epitopes can activate specific CD4+ T cell responses and induce high levels of serum IgG and mucosal sIgA against H. pylori urease in BALB/c mice (Zhou et al., 2009; Guo et al., 2012a, b, 2014).
The commonly used antigen delivery vehicle is the attenuated Salmonella typhimurium (Corthesy Theulaz et al., 1998). Live attenuated S. typhimurium is still able to bring about a limited infection in people such as the old, the infant, and people with immunologic deficiencies (Gomez Duarte et al., 1998). Lactococcus lactis NZ9000 (L. lactis) is a nonpathogenic, noncolonizing Gram positive bacterium and has a long history of widespread use in the dairy industry to make cheese and other fermented foods (Adams & Marteau, 1995). Because L. lactis does not naturally colonize the intestines of humans or animals, it is perhaps more analogous to inert microparticle vaccine delivery systems (Wells et al., 1996). Recently, a number of research groups have reported that L. lactis can be genetically engineered to express bacterial or viral antigens, including staphylococcal enterotoxin B (Asensi et al., 2013), hepatitis A virus antigen (Berlec et al., 2013) and so on.
Previous studies revealed that oral administration with recombinant L. lactis expressing the whole antigen such as the outer membrane protein Cag12 of H. pylori (Kim et al., 2006) and the antigen subunit such as H. pylori UreB (Gu et al., 2009) generated the antigen specific antibodies in the serum of mice, demonstrating that the systemic humoral immune response was successfully induced against the target proteins delivered by L. lactis via the mucosal route. To our knowledge, there were few studies about using L. lactis as delivering vehicle of the multi epitope antigen against H. pylori infection. In the present study, this multi epitope vaccine antigen CUE was expressed in L. lactis by both the constitutive and nisin controlled expression (NICE) systems.
To express CUE extracellularly, the signal peptide (SP) of the L. lactis major secreted protein USP45 (GenBank Accession No. AAA25230) was fused to the N terminal of the antigen CUE, that is, SP CTB UE (SCE). Cloning the double digested SCE gene into three Escherichia coli (E. coli)–L. lactis shuttle vectors, we acquired one inducible expressing vector pCYT SCE and two constitutive expressing vectors pHJ SCE and pMG36e SCE (pMG SCE). Oral immunization with live L. lactis delivering vaccine could avoid complex purification and expensive production costs. Additional two advantages of oral live vaccines are convenient administration and good compliance. The aim of the present study was to express multi epitope antigen CUE in L. lactis and evaluate its immunogenicity and preventive effect after immunization with live delivery vehicle expressing CUE.
Materials and methods
Strains, plasmids, and growth conditions
The bacterial strain L. lactis was grown in M17 medium (Hopebiol, Qingdao, China) supplemented with 0.5% glucose (GM17) at 30 °C without shaking. Escherichia coli DH5α (ATCC 53868) and E. coli BL21 (DE3) were grown in aerated Luria–Bertani medium at 37 °C with shaking. Lactococcus lactis, pCYT NUC (pCYT, Cortes Perez et al., 2009), and pHJ NUC (pHJ, Hou et al., 2011) plasmids were kindly provided by Assoc Prof. Jie Wu (School of Life Science and Technology, China Pharmaceutical University). The pMG36e plasmid (Van De Guchte et al., 1989) was kindly provided by Assoc Prof. Xuegang Luo (College of Bioengineering, Tianjin University of Science & Technology, China). The pET 28a CTB UE (pET CUE) plasmid was constructed before in our laboratory. When required, Kanamycin resistance (Kanr) was used at a concentration of 50 μg mL−1 for E. coli and chloramphenicol resistance (Cmr) was used at 10 μg mL−1 for E. coli and 7.5 μg mL−1 for L. lactis. Also, 10 μg mL−1 erythromycin resistance (Emr) for E. coli and 5 μg mL−1 for L. lactis was added for culture fermentation and transformant selection. The bacteria strain of L. lactis was centrifuged, washed, and resuspended in phosphate buffer solution (PBS) at an appropriate concentration (2.0 × 1011 CFU mL−1 PBS buffer) for oral immunization. The mouse adapted H. pylori strain SS1, first developed by Lee et al. (1997), was preserved in our laboratory. Helicobacter pylori SS1 were cultured on brain–heart infusion (BHI) plates containing 7% calf blood, trimethoprim (5 μg mL−1), polymyxin B (5 μg mL−1), and vancomycin (10 μg mL−1) under microaerophilic conditions at 37 °C for 3 days. The bacteria were harvested as above.
Acquirement and cloning of SCE gene in pEASY T1 vector
The primers used in this study are shown in Table 1. Heterologous proteins can be fused to lactococcal SPs to direct their secretion in the medium (Le Loir et al., 2001). The SP from L. lactis major secretion protein USP45 was adopted to guide the multi epitope antigen CUE releasing in the culture supernatant. Schematic representation of the antigen SCE is shown in Fig. 1a. CTB was selected as the intra mucosal adjuvant to increase the immunogenic activity of the multiple epitope peptide UE. The B epitopes from urease were aimed to generate the epitope specific antibodies against urease. Besides, the Th epitopes from urease were joined for cell mediated immune responses to H. pylori. To retain the adjuvanticity of CTB and the immunogenicity of UE, the linker (DPRVPSS) was selected. In addition, the linkers (KK and GS) were designed to avoid the generation of new epitopes. To obtain the fused SCE gene, we preceded three sequential polymerase chain reaction (PCR) procedures to fuse the 87 bp SP gene to the 5′ end of CUE, lengthening about 29 bp once (shown in Fig. 1b). Firstly, the 843 bp CUE gene was amplified from pET CUE using a sense primer P1 and an antisense primer P4, obtaining an 868 bp gene product. Secondly, the forward PCR products used as template, we could acquire a 900 bp gene product with the primers P2 and P4. Finally, using the primers P3 and P4, we could acquire the 930 bp PCR product of the whole SCE gene by lengthening the second product. Besides, an NsiI site (P3) at the 5′ end of SCE, 6×His tag, and an XhoI site (P4) at the 3′ end of SCE was added (Fig. 2). The subclone pEASY SCE was constructed using TA cloning vector pEASY T1 (shown in Fig. 1c). The whole process of construction could refer to the manufacturer's instructions (TransGen Biotech, Beijing, China).
Primer | Sequence (5′ to 3′) | Underlined sequences |
P1 SCE F (P1) | AGCCCCGTTGTCAGGTGTTTACGCTATGGGCACACCTCAAAATATTACTG | |
P2 SCE F (P2) | ATTTTAATGTCTACAGTGATACTTTCTGCTGCAGCCCCGTTGTCAGGTGTTTACGCT | |
P3 SCE F (P3) | CCAATGCATATGAAAAAAAAGATTATCTCAGCTATTTTAATGTCTACAGTGATACTT | NsiI site |
P4 SCE R (P4) | CCGCTCGAGTCAGTGGTGGTGGTGGTGGTG | XhoI site |
P5 SCE F (P5) | ACGCGTCGACATGAAAAAAAAGATTATCTCAGCTA | SalI site |
P6 SCE R (P6) | CCCAAGCTTTCAGTGGTGGTGGTGGTGGTGCCCGAG | HindIII site |
Primer | Sequence (5′ to 3′) | Underlined sequences |
P1 SCE F (P1) | AGCCCCGTTGTCAGGTGTTTACGCTATGGGCACACCTCAAAATATTACTG | |
P2 SCE F (P2) | ATTTTAATGTCTACAGTGATACTTTCTGCTGCAGCCCCGTTGTCAGGTGTTTACGCT | |
P3 SCE F (P3) | CCAATGCATATGAAAAAAAAGATTATCTCAGCTATTTTAATGTCTACAGTGATACTT | NsiI site |
P4 SCE R (P4) | CCGCTCGAGTCAGTGGTGGTGGTGGTGGTG | XhoI site |
P5 SCE F (P5) | ACGCGTCGACATGAAAAAAAAGATTATCTCAGCTA | SalI site |
P6 SCE R (P6) | CCCAAGCTTTCAGTGGTGGTGGTGGTGGTGCCCGAG | HindIII site |
The introduced restriction sites are underlined in sequences. Italics (GTGGTGGTGGTGGTGGTG) represents 6×His tag sequence.
Primer | Sequence (5′ to 3′) | Underlined sequences |
P1 SCE F (P1) | AGCCCCGTTGTCAGGTGTTTACGCTATGGGCACACCTCAAAATATTACTG | |
P2 SCE F (P2) | ATTTTAATGTCTACAGTGATACTTTCTGCTGCAGCCCCGTTGTCAGGTGTTTACGCT | |
P3 SCE F (P3) | CCAATGCATATGAAAAAAAAGATTATCTCAGCTATTTTAATGTCTACAGTGATACTT | NsiI site |
P4 SCE R (P4) | CCGCTCGAGTCAGTGGTGGTGGTGGTGGTG | XhoI site |
P5 SCE F (P5) | ACGCGTCGACATGAAAAAAAAGATTATCTCAGCTA | SalI site |
P6 SCE R (P6) | CCCAAGCTTTCAGTGGTGGTGGTGGTGGTGCCCGAG | HindIII site |
Primer | Sequence (5′ to 3′) | Underlined sequences |
P1 SCE F (P1) | AGCCCCGTTGTCAGGTGTTTACGCTATGGGCACACCTCAAAATATTACTG | |
P2 SCE F (P2) | ATTTTAATGTCTACAGTGATACTTTCTGCTGCAGCCCCGTTGTCAGGTGTTTACGCT | |
P3 SCE F (P3) | CCAATGCATATGAAAAAAAAGATTATCTCAGCTATTTTAATGTCTACAGTGATACTT | NsiI site |
P4 SCE R (P4) | CCGCTCGAGTCAGTGGTGGTGGTGGTGGTG | XhoI site |
P5 SCE F (P5) | ACGCGTCGACATGAAAAAAAAGATTATCTCAGCTA | SalI site |
P6 SCE R (P6) | CCCAAGCTTTCAGTGGTGGTGGTGGTGGTGCCCGAG | HindIII site |
The introduced restriction sites are underlined in sequences. Italics (GTGGTGGTGGTGGTGGTG) represents 6×His tag sequence.
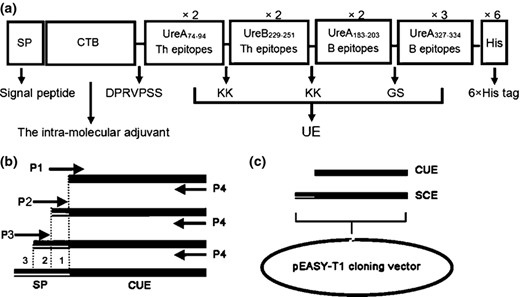
(a) Schematic representation of the designed multiple epitope vaccine SP CTB UE (SCE). SP, SPUSP45 secretion signal; CTB, cholera toxin B subunit, selected as the intramucosal adjuvant; DPRVPSS, the linker between CTB and UE; KK/GS, the linkers in epitopes. × 2/× 3 means two/three repeat times of the epitopes; 6×His tag, six histidine tag sequence. (b) Schematic representation of the process of acquiring SCE gene. The SP gene was fused into the 5′ end of CUE by means of three consecutive PCR (procedures 1, 2, and 3). P1 P4, primers used in lengthening CUE, as shown in Table 1. (c) The process of acquiring subclone pEASY SCE. pEASY T1, TA cloning vector.
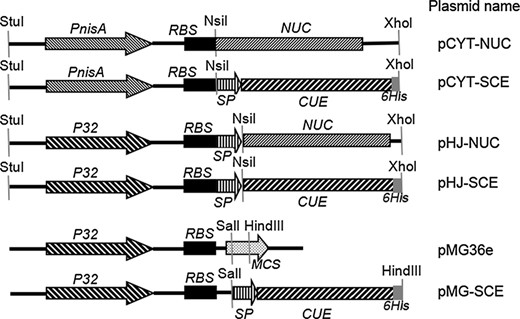
Schematic representation of Lactococcus lactis genetic constructs used in the study. PnisA, nisin inducible promoter; P32, constitutive promoter; RBS, ribosome binding site; NUC, staphylococcal nuclease gene; SP, SPUSP45 secretion signal; CUE, multi epitope antigen CTB UE gene; MCS, multiple cloning sites; 6 His, six histidine tag sequence. StuI, NsiI, XhoI, SalI, and HindIII represent the restriction sites.
Construction of the recombinant L. lactis strains producing the multi epitope antigen CTB UE
The NsiI and XhoI enzymes digested SCE and CUE genes were inserted into NsiI/XhoI site of the expression vectors pCYT and pHJ, resulting in pCYT SCE and pHJ SCE. Similarly, a SalI site at the 5′ end and a HindIII site at the 3′ end were added to the SCE gene using the primers P5 and P6; then, the recombinant vector pMG SCE was constructed by inserting the SalI/HindIII enzymes digested SCE gene into the corresponding sites of the vector pMG36e. All the three plasmid constructions were first established in E. coli DH5α and then introduced into L. lactis via electroporation as previously described (McIntyre & Harlander, 1989). So, three vectors were constructed for the expression of the multi epitope antigen CUE in L. lactis: the nisin inducible pCYT SCE vector, the constitutive pHJ SCE vector, and the constitutive pMG SCE vector. For the inducible antigen expression, the nisin inducible promoter PnisA was used in pCYT SCE while for constitutive P32 promoter (Hou et al., 2011) was used in pHJ SCE and pMG SCE. They were designed to secrete CUE using the powerful N terminal secretion signal of the USP45 protein (van Asseldonk et al., 1990). Expression cassettes of the three plasmid encoding CUE gene and their corresponding empty plasmids are described in Fig. 2.
Expression of the multiple epitope antigen CTB UE and Western blot analysis
For the strains containing inducible vectors (pCYT and pCYT SCE), the culture was induced by 5 ng mL−1 nisin (Silver Elephant Bio engineering Co., Ltd, Zhejiang, China) at an optical density at 600 nm (OD600 nm) of 0.5. These strains were allowed to grow for a further 6 h (OD600 nm = 2.0), and then, cells were harvested by centrifugation. For the constitutive L. lactis (pHJ SCE and pMG SCE) strains, overnight cultures were grown to an OD600 nm of 2.0 at which point cells were similarly harvested. Culture supernatant was collected using cold trichloroacetic acid precipitation method (15%, mass to volume ratio), and the protein precipitate was mixed with SDS PAGE 1× sample buffer after washing three times with cold acetone. Cells were resuspended in 10 mM Tris HCl buffer (pH 8.0), containing 20 mg mL−1 of lysozyme (Sigma) for 1 h at 37 °C and then were mixed with SDS PAGE 1× sample buffer. This was followed by boiling for 10 min and centrifugation at 4800 g for 10 min at 4 °C.
To determine whether CUE was produced by recombinant L. lactis strains, Western blot analysis was performed. Six groups of samples were applied to 12% SDS PAGE and electrotransferred on to a polyvinylidene difluoride membrane (Millipore) by electroblotting membrane at 200 mA for 1 h. Nonspecific binding sites were blocked overnight at 4 °C in blocking buffer [8% dry milk in TBST (20 mM Tris Cl, pH 7.4, with 0.05% Tween 20 and 500 mM NaCl)]. Subsequently, the membrane was incubated for 1 h at 37 °C with gentle agitation in a 1 : 2000 dilution of mouse anti CUE polyclonal serum (previously prepared in our laboratory). After being washed four times with TBST, the membrane was incubated in horseradish peroxidase (HRP) conjugated goat anti mouse IgG (Jackson ImmunoResearch; 1 : 10 000) at room temperature for 1.5 h. Detection of each protein was performed using the electrochemical luminescence Western blot detection system (Biouniquer, Nanjing, China), according to the manufacturer's instructions.
Immunization, sample collection, and challenge with H. pylori
Five to six week old specific pathogen free (SPF) male BALB/c mice were purchased from Comparative Medicine Center of Yangzhou University. All animal experiments were approved by the Animal Ethical and Experimental Committee of China Pharmaceutical University. SPF BALB/c mice were randomized into six groups (six mice in each group) and were inoculated orally with PBS, L. lactis (pCYT), L. lactis (pMG36e), L. lactis (pCYT SCE), L. lactis (pHJ SCE), and L. lactis (pMG SCE), respectively. The L. lactis (pCYT) and L. lactis (pMG36e) groups were the control for subtracting background strains and expression vectors. A total of 2.0 × 1011 CFUs (prepared as described above) of each recombinant L. lactis strain was resuspended in 200 μL of PBS, and 200 μL of this suspension was administered with an intragastric catheter. The L. lactis strains bearing pCYT vectors were induced by nisin for 6 h before collection. For oral administration (shown in Fig. 3), each group mice received six treatments in total, three in the first week and the other three once a week. Antiserum and fecal pellets were separated on the fifteenth day after the last booster. Helicobacter pylori infection models of mice were established at the 42th day, and all mice were inoculated with 300 μL H. pylori SS1 dilution (2 ×109 CFUs) by oral gavage five times every 2 days. Then, 4 weeks after the last H. pylori infection, the immunized and control mice were sacrificed for evaluation of H. pylori infection.
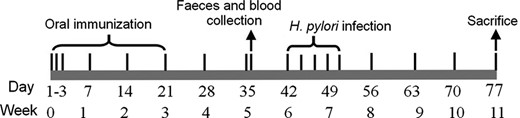
Scheme of immunization protocol. Each group mice received three treatments once in the first 3 days of the week 0, then once every week in the first day of the other 3 weeks. Oral immunization refers to groups of mice with orally administered PBS (normal control group) and bacteria containing pCYT, pMG36e, pCYT SCE, pHJ SCE, and pMG SCE. Days of intervention are specified. Feces and blood samples were collected at the thirty sixth day. Helicobacter pylori infection models of mice were established at the sixth week. The mice were bred for 4 weeks before being used to evaluate H. pylori infection.
Assessment of antigen specific antibody responses
Antigen specific antibodies were measured by enzyme linked immunosorbent assay (ELISA). Briefly, ELISA plates (Corning Costar Corp., MA) were coated with 0.5 μg per well of natural H. pylori urease (Linc Bio, Shanghai, China), at 4 °C overnight. The plates were washed with TBST and blocked with 3% (mass to volume ratio) BSA in PBS. The plates were then washed with TBST, incubated with 100 μL of mouse serum and serially diluted in PBS at 37 °C for 1 h. After washing, HRP conjugated goat anti mouse IgG, IgG1, and IgG2a (Santa Cruz Biotech) was added, and the plates were incubated again for 1 h. The color reaction based on tetramethylbenzidine was terminated after incubation for 10 min at room temperature by the addition of 50 μL of H2SO4 (1 M), and the absorbance at 450 nm was measured by a microplate reader (Bio Rad). Serum samples from mice were assayed in triplicate.
To assess specific mucosal secretory IgA (sIgA) production, c. 150 mg of fecal pellets was suspended in PBS with 0.2% BSA and phenylmethanesulfonyl fluoride (PMSF, Bio Basic Inc., Markham, Canada) and incubated overnight at 4 °C. Suspension was centrifuged at 4800 g for 20 min at 4 °C and supernatant stored at −80 °C (Berlec et al., 2013). ELISA was performed essentially as described for serum samples with minor modifications as follows. Fecal suspensions were applied in 1 : 3 and 1 : 6 dilutions in three parallels. HRP conjugated anti mouse IgA antibodies (Santa Cruz Biotech) at 1 : 2000 dilution were used instead of anti mouse IgG antibodies.
Assessment of prophylactic immunization effect
To evaluate the prophylactic effect of the live vaccine of recombinant L. lactis delivering CUE, urease activity test and quantitative culture of H. pylori from the stomach of mice were designed. The immunized and control mice were sacrificed, and the stomach tissues were separated into two equiponderant portions. Urease activity determination was performed as previously described, with some modifications (Fujii et al., 2004). Briefly, one half of stomach tissues was immediately placed inside an Eppendorf tube containing 500 μL of the urease substrate containing 500 mM urea, 0.02% phenol red, and 0.1 mM dithiothreitol (DTT). The stomach samples were incubated for 4 h at room temperature and the absorbance measured at 550 nm (OD550 nm) by a microplate reader (Bio Rad). Quantitative culture of H. pylori was performed as previously described, with some modifications (Zhao et al., 2007; Zhou et al., 2009). Another half of the stomach was cut into pieces and was homogenized in 500 μL of PBS with a tissue homogenizer. Serial dilutions of the homogenates were plated on BHI plates containing calf blood and antibiotics as described above. After 3 days of culture, the colonies were counted and the number of CFUs per stomach calculated. The bacteria were identified further by microscopy, catalase test, oxidase test, and urease test, according to the methods reported by Ferrero et al. (1998).
Statistical analyses
All the statistical analyses were performed with the GraphPad prism 5 software. Data were expressed as mean ± SD. Statistical significance was tested using Student's paired t test. P < 0.05 was considered as statistically significant (*P < 0.05, **P < 0.01, ***P < 0.001; ns: not significant).
Results
Acquirement of the SCE gene and construction of the recombinant L. lactis strains
Three sequential PCR products of SCE gene (930 bp) were identified by 2.0% agarose gel electrophoresis (Fig. 4). Then, SCE gene was subcloned into pEASY T1 simple vector (3928 bp, TransGen Biotech, Beijing, China), yielding pEASY SCE (Fig. 1c). The construction of the subclone pEASY SCE (4858 bp) was confirmed by sequencing. The DNA fragment encoding the multi epitope antigen CUE was cloned and expressed in L. lactis using three different expression vectors pCYT, pHJ, and pMG36e (Fig. 2). The constructions of recombinant plasmids were confirmed by sequencing. The plasmids were introduced in L. lactis strains by electroporation using a Gene Pulser Xcell electroporation system (Bio Rad) following the manufacturer's instructions.
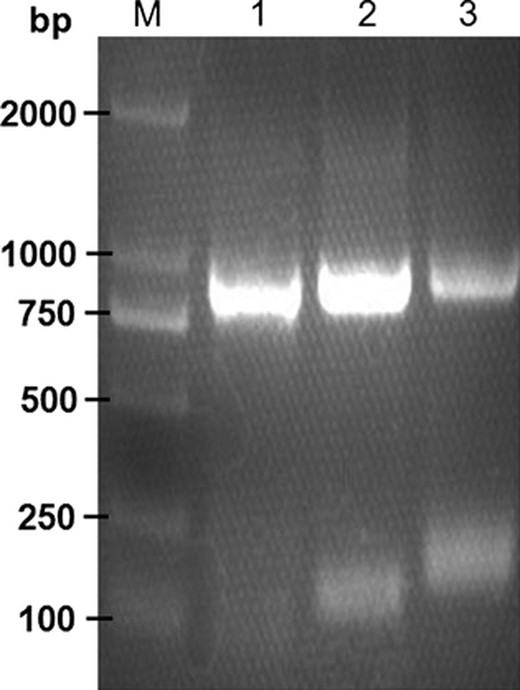
Identification of three sequential PCR products of SCE gene by 2.0% agarose gel electrophoresis. M: DNA marker DL2000; 1–3, three PCR products of SCE gene (868, 900, and 930 bp).
Expression and identification immunoreactivity of the CUE by L. lactis expression system
To examine the expression of the recombinant proteins in L. lactis, we next analyzed protein samples from the transfected L. lactis strains. All the samples were separated in cellular (C) and supernatant (S) fractions respectively and separated by SDS PAGE following nisin mediated induction and visualized with Coomassie brilliant blue (CBB). The bands of CUE from all the cellular fractions were detected in CBB stained gels. Unfortunately, the recombinant protein CUE from all the supernatant fractions could not be detected because of weak signals. With the aim of further confirming whether L. lactis possessing recombinant vectors could produce the CUE protein extracellularly, Western blotting analysis of the proteins from isopycnic cell lysates and culture supernatant was performed after adjusting all the L. lactis to 2.0 × 1011 CFUs, and the anti CUE mouse polyclonal antibody (prepared in our laboratory) was used as the primary antibody. In the L. lactis group (lane 1–2, Fig. 5b) and two empty vector control groups (lane 3–6, Fig. 5b), recombinant proteins could not be detected. One band (c. 35 kDa) corresponding to the precursor SCE was detected in all the cell fractions from three recombinant L. lactis groups (lanes: 9, 11, and 13). However, there were weak bands (c. 33.6 kDa) of mature CUE in the lanes (lanes: 10, 12, and 14) of all the culture supernatant. The results suggested that three recombinant L. lactis strains could successfully produce CUE intracellularly and extracellularly, and about 20% protein quantified by the software gelpro32 was expressed in secretory form.
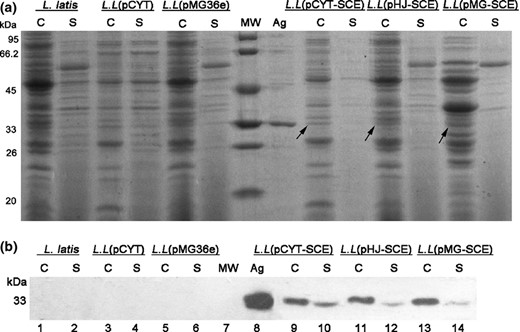
SDS PAGE and Western blotting of recombinant Lactococcus lactis strains expressing multi epitope vaccine CUE (lanes: 9–14) and the control groups (lanes: 1–6). C, protein extracts were prepared from cell pellets; S, protein extracts were prepared from culture supernatant. MW, protein molecular weight marker; Ag, antigen CUE purified from Escherichia coli BL21 (DE3), served as positive control. L.L represents the strains L. latis. (a) The total proteins extracted from cell pellets and culture supernatant of six L. lactis groups were separated by 12% SDS PAGE gel. (b) Western blotting analysis with the anti CUE mouse polyclonal antibody as primary antibody.
Assessment of recombinant L. lactis immunized mouse sera and feces by ELISA
Groups of mice were immunized orally with L. lactis expressing the CUE protein. Control mice were vaccinated in the same way with L. lactis (pCYT), L. lactis (pMG36e) strains, and PBS. Two weeks after the final vaccination, the serum from each group was then diluted 400, 800, and 1600 times; then, antibodies of humoral immune responses including IgG, IgG1, and IgG2a (Fig. 6) were detected by a microplate reader (Bio Rad) at 450 nm. A significant increase (***P < 0.001) in the immune responses was observed in all tested groups compared with the PBS group (Fig. 6). Also, the IgG responses of animals that received L. lactis (pHJ SCE) strains orally were elicited significantly in all three recombinant L. lactis immunized groups. Animals that were orally immunized with bacteria bearing L. lactis (pCYT SCE) and L. lactis (pMG SCE) strains showed no significant difference in the IgG antibody titer. Mucosal IgA responses observed with the control groups (PBS and the empty vector pCYT) were low and differed significantly (**P < 0.01) from those of the L. lactis (pHJ SCE) strains group (Fig. 6d) when the samples were diluted 1 : 3 and 1 : 6.
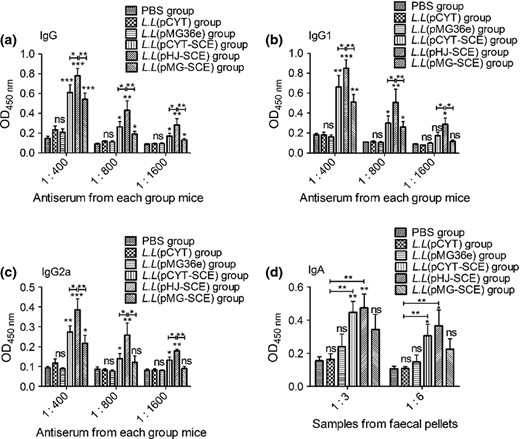
Detection of specific antibodies in recombinant Lactococcus lactis immunized mice by ELISA. Data are expressed as mean ± SD. L.L represents the strains L. latis. P < 0.05 was considered as statistically significant. *P < 0.05, **P < 0.01, ***P < 0.001, ns: not significant. The mice were orally immunized with PBS, L.L (pCYT), L.L (pMG36e), L.L (pCYT SCE), L.L (pHJ SCE), and L.L (pMG SCE), respectively. Antiserum and feces samples were collected on the fifteenth day after the last booster. The serum was diluted 1 : 400, 1 : 800, and 1 : 1600, and six groups of fecal suspensions were prepared and applied in 1 : 3 and 1 : 6 dilutions in three parallels. Serum total IgG (a), IgG1 (b), IgG2a (c), and feces mucosal IgA (d) antibodies against Helicobacter pylori urease after oral prophylactic immunization were measured by ELISA.
Urease activity determination and quantitative culture of H. pylori from the stomach of mice
The prophylactic effect of the L. lactis producing multi epitope antigen CUE was assessed by urease tests and quantitation of viable bacteria colonies from mice stomachs. In the prophylactic experiment, the results of the urease test (Fig. 7a) and quantitation of culturable H. pylori (Fig. 7b) showed significant differences between the groups vaccinated with the L. lactis delivering vaccine antigen CUE and PBS, but no differences between the empty vectors groups and the PBS control. Consistent with antibodies level, H. pylori urease activity and the number of H. pylori colonization from the L. lactis (pHJ SCE) group were lowest compared with that of the other two recombinant L. lactis immunized groups.
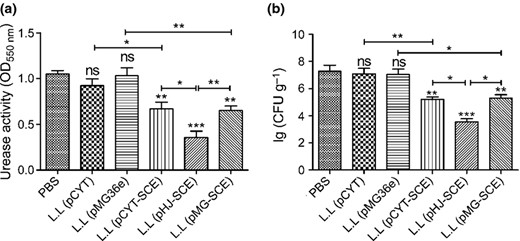
Evaluation of Helicobacter pylori infection after prophylactic vaccination. Data are expressed as mean ± SD. L.L represents the strains L. latis. A value of P < 0.05 was considered statistically significant: *P < 0.05, **P < 0.01, ***P < 0.001, ns: not significant. The mice were orally immunized with PBS, L.L (pCYT), L.L (pMG36e), L.L (pCYT SCE), L.L (pHJ SCE), and L.L (pMG SCE), respectively, and infected with H. pylori. (a) Urease activity in the stomach after oral prophylactic immunization. Data are the mean ± SD. (b) Colonization of H. pylori in the stomach after oral prophylactic immunization. The number of bacteria (CFU) per stomach was determined for individual mice in each group by quantitative culture. Data are the mean ± SD.
Discussion
Infection with H. pylori is the most frequent gastroduodenal human disease in the world caused by bacteria. In recently years, using the subunit vaccines and epitope vaccines to prevent or fight H. pylori infection has been attracting more and more attention (Zhou et al., 2009; Guo et al., 2012a, b; Huang et al., 2013). Multi epitope vaccine contains more effective epitopes carefully selected by bioinformatics software than signal epitope vaccines and it is speculated that multi epitope vaccines should show stronger immunogenicity and immunoreactivity comparing with signal epitope ones. In our previous study, we had designed signal epitope vaccine CTB UA (Guo et al., 2012a, b). Based on it, to further increase the immunogenicity and immunoreactivity, the multi epitope vaccine CUE was designed and constructed and it showed better characteristics than CTB UA (Guo et al., 2014).
Lactococcus lactis has been given generally recognized as safe (GRAS) status by the American Food and Drug Administration. Oral immunization with live L. lactis could avoid complex purification procedures and protein degradation because of the protection of cell wall. In the present study, we constructed three expression vectors including one inducible plasmid (pCYT SCE) and two constitutive ones (pHJ SCE and pMG SCE); then, L. lactis was used as a live delivery vector system of multi epitope vaccine antigen CUE for oral vaccination.
Using ternate continuous PCR, an 87 bp SP gene form L. lactis secretion protein USP45 was fused into the 5′ end of CUE, resulting in SCE (SP CTB UE). This method can avoid the costly gene synthesis and complex digestion and ligation. Besides, adding SP has two additional advantages. Firstly, SP could lead the multi epitope antigen CUE releasing in the culture medium without protein degradation because of few extracellular protease of L. lactis. Secondly, the CUE expressing intracellularly could also escape from protein degradation by protease because of the fusion with the host peptide SP (Le Loir et al., 2005). It can be noticed that Western blot analysis could detect the precursor SCE (c. 35 kDa) and mature CUE (c. 33.6 kDa) of all the recombinant constructs. Above evidence suggests that about 80% of the multi epitope antigen CUE existed in the form of precursor SCE intracellularly and a little mature CUE was released in the culture supernatant.
However, the secretion efficiency (SE) of mature CUE is a bit low (c. 20%). Many reports (Le Loir et al., 2005) showed that SE is correlated with protein conformation itself rather than protein molecular weight. On the base of this principle, low SE may be due to that CUE composed of two obvious separate parts (CTB and UE) is not very suitable for secretory expression because of its unique structure. Many studies have reported the survival and metabolic activity of lactococcal cells in digestive tracts (Klijn et al., 1995; Drouault et al., 1999; Kimoto et al., 2003). It was speculated that either intracellular or extracellular expression of protein would be targeted to gastric area or small intestine (Hou et al., 2011). The poor survival rate of lactococcal cells in gastrointestinal tract suggests that the CUE periplasmic and intracellular would gradually get access to the gastrointestinal mucosa with the collapse of the cells and then target to induce specific IgG antibody production on the one hand and the surviving L. lactis would continue to produce CUE on the other.
ELISA results indicated three groups of recombinant L. lactis expressing CUE could all induce the production of IgG antibodies. Peritoneal immunity with CUE antigen purified from E. coli could induce a high level of the specific IgG antibody, reaching 1 : 186 500 in mice (data not shown). Comparing with PBS or empty vector control groups, the expressing level of CUE of the constitutive expressing L. lactis (pHJ SCE) group was not the highest, but induced the highest level of antibody. It is worth noting that plasmids pHJ (Hou et al., 2011) and pMG36e (Van De Guchte et al., 1989) use the same promoter (P32), but differ in origin of replication and copy number. It may be a reason for the lower expressing level of CUE and the lower antibody level of L. lactis (pMG SCE) group compared with that of L. lactis (pHJ SCE) group. As to the inducible high expression of L. lactis (pCYT SCE) group, producing CUE may gradually stop without the inducer nisin after suspending the pellets in PBS for oral gavage, so the antibody level of the L. lactis (pCYT SCE) immunized group was lower. Helicobacter pylori urease activity assay showed that the antibodies induced by L. latis expressing CUE could react against H. pylori urease, and the inhibition of the L. lactis (pHJ SCE) immunized group was highest. That was consistent with the antibody level of this group.
Previous studies reported that oral immunization with recombinant L. lactis expressing UreB and UreB IL 2 (human interleukin 2, as the mucosal adjuvant) into mice could induce serum IgG titer reaching 104 and 105, respectively, and all decrease the colonization of H. pylori by about two orders of magnitude (Gu et al., 2009; Zhang et al., 2014). We have to point out that inducing IgG antibody titer (c. 2 × 103, data not shown) of this study was a bit low, probably resulting from low protein expression level. However, oral vaccine of this study could decrease the colonization of H. pylori by about two orders of magnitude (7.14 ± 0.95 vs. 4.68 ± 0.98 log10 CFU g−1 stomach, Fig. 7b) compared with the control groups. To improve it, our studies will optimize the CUE gene according to L. lactis codon preference, for example, modulating GC content to about 40%. Further more, we will continue to improve the protein expression conditions, for example: lowering temperature, prolonging induction time, and adding inducer into the solvent of gavage for inducing expression and so on.
In conclusion, we have constructed genetically modified lactic acid bacteria expressing multi epitope vaccine CUE with good immunogenicity and immunoreactivity, which could induce specific neutralizing antibodies against urease and attenuate H. pylori infection in mice in some degree. Ongoing studies will evaluate the therapeutic effects of the modified lactic acid bacteria against H. pylori infection in BALB/c mice. These data indicate that the recombinant lactic acid bacteria could be an effective tool against H. pylori, and this study will provide experimental evidences for the development of multi epitope vaccines carried by L. lactis for human use.
Acknowledgements
This work was supported by National Major Special Program of New Drug Research and Development (Grant No. 2012ZX09103301 008) and Natural Science Foundation of Jiangsu Province (Grant No. BK20130647). We thank especially Associate Prof. Jie Wu for her contributions in implementation of this experiment.
References
Helicobacter pylori is an important human pathogen. Current preventive strategies for this organism are ineffective. In this study, the authors utilize Lactobacillus to deliver a combinatorial vaccine construct harboring multiple epitopes linked to adjuvant. Combinatorial epitope-based vaccines are attractive as they can be designed to protect against multiple pathogens and against diverse strains of a given pathogen. This study is an important contribution to the growing field of multi-epitope-based vaccines.
Editor: Richard T. Marconi