-
PDF
- Split View
-
Views
-
Cite
Cite
Antje Munder, Florian Wölbeling, Jens Klockgether, Lutz Wiehlmann, Burkhard Tümmler, In vivo imaging of bioluminescent Pseudomonas aeruginosa in an acute murine airway infection model, Pathogens and Disease, Volume 72, Issue 1, October 2014, Pages 74–77, https://doi.org/10.1111/2049-632X.12184
- Share Icon Share
Abstract
Non invasive bioluminescence imaging allows the analysis of infectious diseases in small animal models. In this study, an acute airway infection of C3H/HeN mice with luxCDABE transformed Pseudomonas aeruginosa TBCF10839 and an isogenic transposon mutant was followed by optical imaging in vivo. Using the disease-causing dose of 2.0 × 106 CFU of the cystic fibrosis airway isolate TBCF10839, subtle luminescence of the lungs was inconsistently visible for the first hour after infection. Conversely, using a 100 fold higher dose of the strongly virulence attenuated transposon mutant, the robust signal of bioluminescent bacteria increased over 24 h. To monitor murine airway infections with P. aeruginosa in vivo by bioluminescence, one should select an attenuated mutant of a virulent strain or a wild type strain that naturally lacks virulence determinants and/or that has acquired a low virulence persister phenotype by patho-adaptive mutations.
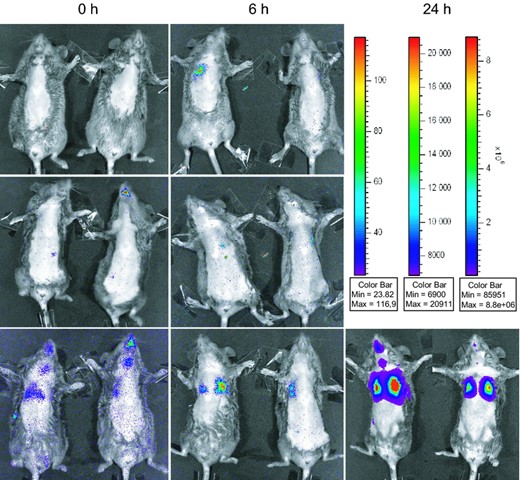
Monitoring of acute airway infection in a mouse model using in vivo imaging with bioluminescent Pseudomonads faces some difficulties in getting robust signals with sublethal infection doses of virulent bacteria.
Bioluminescence imaging allows the analysis of bacterial infectious diseases in animal models (Chang et al., 2011; Kong et al., 2011). During the last 20 years, non invasive imaging studies have been performed with numerous micro organisms including Pseudomonas aeruginosa (reviewed by Andreu et al., 2011). Here, we tested the suitability of the in vivo monitoring of acute lung infections with bioluminescent P. aeruginosa in a standardized murine airway infection model (Munder et al., 2011). The cystic fibrosis (CF) airway isolate TBCF10839 (Klockgether et al., 2013) whose virulence in murine airways had been established in previous studies (Munder et al., 2011; Wölbeling et al., 2011), and its isogenic virulence attenuated transposon mutant D8A6 that displays an inactivated ORF 5PG21 in genomic island PAGI 5 (Wiehlmann et al., 2007; Klockgether et al., 2013) were used in this study. Luminescent derivatives of the two strains were generated by integrating a luxCDABE luciferase reporter gene operon into the bacterial chromosome. Therefore, a miniCTX lac lux plasmid was isolated from recombinant Escherichia coli Topo one shot CTX lac lux cells (DiGiandomenico et al., 2007) and integrated site specifically to the attB site of the P. aeruginosa chromosome via electroporation (Choi et al., 2006). The functional efficiency of the insertion was controlled by measuring luminescent signals with the IVIS system. To excise the miniCTX backbone, we performed a Flp FRT recombination (Hoang et al., 1998; Hoang et al. 2000).
The kinetics of the infection was followed by the sensitive IVIS® Imaging System 200 Series (Perkin Elmer, Waltham, MA; formerly manufactured by Xenogen, Alameda, CA). To monitor infections with P. aeruginosa in the mouse, the IVIS system has so far primarily applied to systemic (BitMansour et al., 2002), burn (Hamblin et al., 2003; Burkatovskaya et al., 2006; Barman et al., 2011) and biofilm (Kadurugamuwa et al., 2003), but less to airway infection models (DiGiandomenico et al., 2007; Riedel et al., 2007; Ramphal et al., 2008).
For in vivo imaging, C3H/HeN mice were infected by view controlled intratracheal instillation (i.t.) (Munder et al., 2002) with doses of 2.0 × 106 and 2.0 × 108 CFU of P. aeruginosa strains TBCF10839lux or D8A6lux. For survival experiments, mice were infected with 7.5 × 106 CFU, a dose which resulted in 50% lethality of the animals within the first 120 h of infection for strain TBCF10839. In contrast, the same number of D8A6 bacteria was not able to induce any mortality (Fig. 1a). A similar picture emerged when looking on the loss of body weight, which was dramatically for TB10839 infected mice, whereas D8A6 infected animals showed milder weight loss and were able to compensate this within the observation period of 192 h (Fig. 1b). The multiparametric disease score of infected mice (Munder et al., 2005) displayed a strongly affected body condition in TB10839 infected mice, but D8A6 infected animals showed only slight impairment (Fig. 1c). Lung slices were examined pathohistologically for inflammatory changes. Whereas TBCF10839 had caused an acute catarrhalic suppurating alveolar pneumonia in the murine lungs (Fig. 1d), only very slight signs of inflammation, such as a minor migration of PMNs and alveolar macrophages into the alveoli, could be observed in the lungs of D8A6 infected mice (Fig. 1e) compared with mock infected mice instilled with PBS (Fig. 1f).
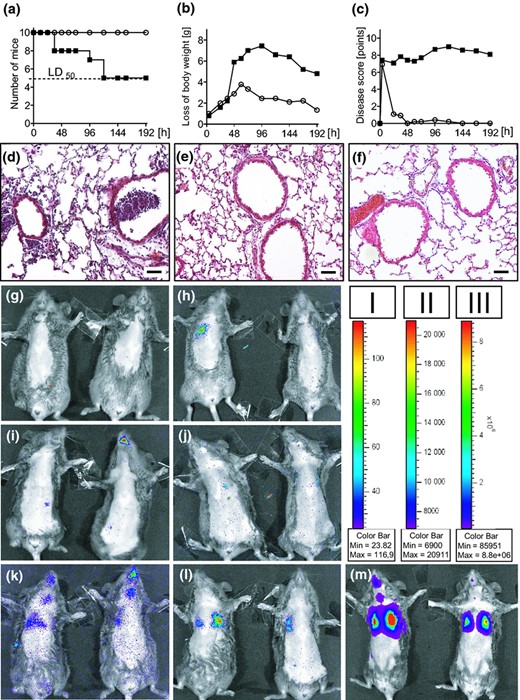
(a–c) Physiological parameters of C3H/HeN mice after i.t. infection with 7.5 × 106 CFU of Pseudomonas aeruginosa TBCF10839 (closed squares; n = 12) and the isogenic mutant D8A6 (open circles; n = 12). (a) Survival. The applied dose of 7.5 × 106 CFU was equivalent to a LD50 for strain TBCF10839, but did not cause any mortality in D8A6 infected mice. (b) Body weight. TB10839 infected mice displayed a dramatic loss of body weight under infection, D8A6 infected animals showed milder weight loss, started regaining of weight earlier (72 h p.i.) and were almost able to return to their initial weight within the observation period of 192 h. (c) Disease score. The body condition of mice was strongly impaired under TBCF10839 infection, in contrast, all D8A6 infected animals recovered within 48 h p.i. to normal body condition. (d) TBCF10839 shows a strong purulent inflammation in murine lungs with intra and peribronchiolar infiltrates of leucocytes and perivascular oedema 48 h after i.t. infection with 7.5 × 106 CFU. (e) Only single leucocytes are seen 48 h after i.t. infection around the bronchi with the same dose of the isogenic mutant D8A6 (f) Vehicle control, 48 h post instillation of 30 μL PBS, original magnification × 200, bar 50 μm, H&E. (g–m) In vivo monitoring of P. aeruginosa in acute murine airway infection with the IVIS® imaging system (binning 4, exposure time 2 m, f/stop 1, FOV 12,8 cm). Bacterial distribution was monitored 0 h (Fig. 1g, i and k), 6 h (Fig. 1h, j and l) and 24 h (Fig. 1m) after i.t. instillation with TBCF10839.lux and D8A6.lux in infected mice. Infection doses were 2.0 × 106 CFU (TBCF10839.lux: Fig. 1g/h; D8A6: Fig. 1i/j) and 2.0 × 108 CFU (for D8A6.lux exclusively; Fig. 1k–m). Animals were depiliated using hair removal cream, imaged ventrally and although under anaesthesia additionally fixed with adhesive tape to reduce movement artefacts during imaging. Luminescence is observed emanating from the nasopharynx, tracheas and lungs. The colour bars indicate the intensity of the luminescent signal, with red and blue serving as the high and low signals, respectively (Fig 1, bar I: g–k; bar II: l; bar III: m).
For in vivo monitoring with the IVIS system, imaging was started directly (0 h) after instillation of 2.0 × 106 CFU and 2.0 × 108 CFU of strains TBCF10839lux and D8A6lux and was repeated at 6, 12 and 24 h p.i. Prior to infection and to improve the luminescent signals, anaesthetized mice were depiliated ventrally and during imaging fixed with adhesive tape to minimize movement artefacts. Best results were achieved with a high resolution at binning 4 (that means four by four pixels had been grouped to form one larger pixel, thereby increasing the signal to noise ratio), a f/1 camera lens aperture and exposure times of 2–4 min. The Living Image Software® 2.50 (Perkin Elmer, Waltham, MA) was utilized to acquire, store and analyse the images with the connected computer.
Occasionally, luminescent signals were detected around the mouth and along the trachea of the mice at 0 h p.i. (Fig. 1i), indicating a contamination of the upper airways with bacteria during the instillation. These signals disappeared within the next few hours (Fig. 1h, j and l), but re emerged as time passed, presumably caused by multiplying of the bacteria in the lower airways (Fig. 1m). In the lungs, luminescence either emerged immediately after instillation or at the next time point of 6 h (Fig. 1h and k–m). Using the disease causing dose of 2.0 × 106 CFU of TBCF10839, subtle luminescence was observed over the left or right lung in several, but not all mice (Fig. 1h). The luminescent signal had disappeared by 24 h indicating that bacterial numbers had decreased below the levels of detection (data not shown). Conversely, using a 100 fold higher dose of the virulence attenuated D8A6 mutant, the luminescent bacteria showed signals with the IVIS that increased over time during the observation period of 24 h (Fig. 1k).
This study demonstrates that the monitoring of an acute airway infection of mice with bioluminescent P. aeruginosa is feasible. However, in case of virulent P. aeruginosa, the possible dose is too low to reliably follow the 5 day natural course of the acute infection up to recovery (Wölbeling et al., 2011) by in vivo imaging of the bacteria. A range of two orders of magnitude of the P. aeruginosa inoculum evokes the full spectrum of responses in the mouse from subtle signs of morbidity to full blown lethality. According to our experience, the LD50 of most P. aeruginosa strains from environment or disease habitats will be in the range of 106–107 CFU. A dose of about 10% of the LD50 results in a solid clinical manifestation of infection without mortality (Wölbeling et al., 2011). These doses result in a satisfactory imaging of bioluminescent bacteria in explanted lungs ex vivo (Henken et al., 2010), but are too low for an imaging of the lower airways in vivo. As the bioluminescence signal increases with the number of organ incorporated bacteria, P. aeruginosa strains should be selected that are attenuated in acute virulence but are still capable to persist in the murine lungs. Only then – as shown in this study for the D8A6 mutant – infection doses of more than 108 CFU become feasible. One may choose an attenuated mutant of a virulent strain – as shown in this study – or a wild type strain that naturally lacks virulence determinants such as the type III secretion system and/or that has acquired a low virulence persister phenotype by patho adaptive mutations.
Acknowledgements
The authors would like to thank Anna Silke Limpert for the support in the construction of the D8A6 mutant and Joanna Goldberg for kindly providing us with the strain E. coli Topo one shot CTX lac lux and the plasmid pFLP2. This study was supported by grants from the Deutsche Forschungsgemeinschaft (IRTG 653; SFB 587, A9) and the German Center for Lung Research.
References
This short communication describes in vivo imaging of bioluminescent bacteria as a tool to monitor murine airway infection caused by Pseudomonas aeruginosa. Using a virulent strain of the bacterial pathogen the inoculation of a sufficiently high dose to make the infection visible is followed by rapid death. Therefore, the authors apply a strain with reduced virulence to monitor airway infection over time. This may provide a useful approach for other pathogens with the same potential problem.
Editor: Nicholas Carbonetti