-
PDF
- Split View
-
Views
-
Cite
Cite
Yiying Liu, Peter De Schryver, Bart Van Delsen, Loïs Maignien, Nico Boon, Patrick Sorgeloos, Willy Verstraete, Peter Bossier, Tom Defoirdt, PHB-degrading bacteria isolated from the gastrointestinal tract of aquatic animals as protective actors against luminescent vibriosis, FEMS Microbiology Ecology, Volume 74, Issue 1, October 2010, Pages 196–204, https://doi.org/10.1111/j.1574-6941.2010.00926.x
- Share Icon Share
The use of poly-β-hydroxybutyrate (PHB) was shown to be successful in increasing the resistance of brine shrimp against pathogenic infections. In this study, we isolated for the first time PHB-degrading bacteria from a gastrointestinal environment. Pure strains of PHB-degrading bacteria were isolated from Siberian sturgeon, European sea bass and giant river prawn. The capability of selected isolates to degrade PHB was confirmed in at least two of three setups: (1) growth in minimal medium containing PHB as the sole carbon (C) source, (2) production of clearing zones on minimal agar containing PHB as the sole C source and (3) degradation of PHB (as determined by HPLC analysis) in 10% Luria–Bertani medium containing PHB. Challenge tests showed that the PHB-degrading activity of the selected isolates increased the survival of brine shrimp larvae challenged to a pathogenic Vibrio campbellii strain by a factor 2–3. Finally, one of the PHB-degrading isolates from sturgeon showed a double biocontrol effect because it was also able to inactivate acylhomoserine lactones, a type of quorum-sensing molecule that regulates the virulence of different pathogenic bacteria. Thus, the combined supplementation of a PHB-degrading bacterium and PHB as a synbioticum provides perspectives for improving the gastrointestinal health of aquatic animals.
Introduction
The prophylactic use of antibiotics in aquaculture has resulted in multiple resistance in several pathogens, leading to ineffective treatment and an increased risk of resistance transfer to animal and human pathogens (Defoirdt et al., 2007a; Das et al., 2009). Also, trace amounts of antibiotics have been detected in aquatic products for human consumption and unwanted alterations of bacterial communities in sediment and water column have been observed (Cabello, 2006). Consequently, there is a growing awareness that antibiotics should be used more carefully in animal production and the prophylactic use should be stopped. However, a decreased use of antibiotics could result in a higher frequency of pathogenic bacteria, which in turn could lead to a higher incidence of infections.
Several research groups are currently investigating biological and environment-friendly alternative approaches to fight disease problems in aquaculture (Defoirdt et al., 2007a). In this respect, currently, there is considerable interest in short-chain fatty acids as biocontrol agents in animal production. However, there are some important limitations to the use of short-chain fatty acids (especially in aquaculture settings, where they can diffuse into the culture water). Therefore, we have recently started investigations on the use of polyhydroxyalkanoates, polymers of β-hydroxy short-chain fatty acids, as an alternative (for a review, see Defoirdt et al., 2009). The bacterial storage compound poly-β-hydroxybutyrate (PHB) is the most well-known polyhydroxyalkanoate. This compound is accumulated as a carbon (C) and energy storage reserve by a wide variety of bacteria residing in a broad range of habitats (Muller & Seebach, 1993). The use of PHB as an anti-infective strategy has been shown in studies using gnotobiotic Artemia franciscana (Defoirdt et al., 2007b; Halet et al., 2007). PHB is not water soluble, and consequently, it needs to be degraded into water-soluble products (i.e. β-hydroxybutyrate monomers and oligomers) in the gastrointestinal tract in order to have a beneficial effect on the host (Defoirdt et al., 2009). One strategy to improve the degradation of PHB into water-soluble products is to use PHB-degrading microorganisms, which have been shown before to increase the protective effect of PHB particles (Defoirdt et al., 2007b).
Aerobic and anaerobic PHA-degrading microorganisms have been isolated from various environments (Jendrossek & Handrick, 2002; Calabia & Tokiwa, 2004). However, to the best of our knowledge, no such microorganisms have been isolated from gastrointestinal environments thus far. In this study, we report the isolation of PHB-degrading strains from the gastrointestinal environment of aquatic animals. Siberian sturgeon, European sea bass and giant river prawn were selected due to their importance for aquaculture and because they live in different environments (fresh water, seawater and brackish water, respectively) and have different feeding habits. The possibility of applying the isolates as protective agents was investigated in a model system with gnotobiotic brine shrimp (A. franciscana) challenged to a virulent Vibrio campbellii strain, in which the beneficial effects of PHB and PHB-degrading bacteria have been documented before (Defoirdt et al., 2007b).
Materials and methods
Origin of intestinal microbiota and enrichment of PHB degraders
Samples were taken from the posterior gut content of 5-month-old Siberian sturgeon (Acipenser baerii). The fish had been fed at 3% on wet body weight day−1 for 45 days with an artificial diet (Joosen-Luyckx Aqua Bio, Turnhout, Belgium) containing 5% PHB (w/w). Secondly, fecal matter of 3-month-old European sea bass (Dicentrarchus labrax) juveniles was collected. These had been fed at 3% on wet body weight day−1 for 40 days with an artificial diet (Skretting, Boxmeer, the Netherlands) containing 10% PHB (w/w). Finally, intestinal microbiota were collected from larvae of the giant river prawn (Macrobrachium rosenbergii). These larvae had been fed ad libitum for 15 days with Artemia nauplii enriched for 24 h in a 5 g L−1 PHB solution. After sampling, all bacterial sources were stored in 20% glycerol at −80 °C.
Before use, the intestinal microbiota were washed three times in a salt solution [5.0 g L−1 synthetic sea salt (Instant Ocean®, Aquarium Systems Inc., Sarrebourg, France)]. Each washed sample was inoculated in 10 mL minimal medium containing PHB as the sole C source. The medium contained 5.0 g L−1 synthetic sea salt, 0.2 g L−1 NH4Cl and 1.0 g L−1 PHB particles (average diameter 25 μm; Goodfellow Cambridge Ltd, Huntingdon, UK). The cultures were incubated at 28 °C on an orbital shaker at 130 r.p.m. After 3 days, each of the cultures was transferred at a concentration of c. 105 CFU mL−1 into 100 mL of a fresh medium. This procedure was repeated to enrich PHB-degrading bacteria.
Isolation of PHB-degrading strains
The PHB-degrading mixed enrichment cultures were plated on Luria–Bertani (LB) agar and the morphologically most abundant colonies were picked using a sterile inoculation loop. Each colony type was incubated in minimal medium containing PHB for 72 h and subsequently purified by streak plating on LB agar. In this way, 38 pure cultures were obtained. Based on preliminary tests (growth in minimal medium containing PHB and effect toward Artemia), the six most promising strains were retained for further experimenting: S4 and S7 from sturgeon, B7 and B12 from sea bass and M13 and M15 from giant river prawn.
Identification of the isolates by 16S rRNA gene sequencing
PCR targeting a 1500-bp fragment of the 16S rRNA gene of the isolates was performed according to Boon et al. (2002) using the primer pair GM3f and GM4r (Biolegio, Nijmegen, the Netherlands) (Muyzer et al., 1995). PCR was performed using a GeneAmps PCR system 2700 thermal cycler (PE Applied Biosystems, Nieuwerkerken a/d Ijssel, the Netherlands) using the following program: 95 °C for 5 min, 32 cycles of 94 °C for 1 min, 42 °C for 1 min, 72 °C for 3 min and finally an extension period of 72 °C for 10 min.
DNA sequencing of the PCR products obtained was carried out at IIT Biotech (Bielefield, Germany). The forward and reverse sequences obtained with the sequencing primers GM3f and GM4r were processed using ebiox software, and homology searches were completed with the blast server of the National Centre for Biotechnology Information using the blast algorithm (Altschul et al., 1997) for the comparison of the nucleotide query sequence against a nucleotide sequence database (blastn). The nucleotide sequences of the isolates were deposited in the GenBank database (http://www.ncbi.nlm.nih.gov/Genbank) with the accession numbers GU372408, GU372409, GU3 72410, GU372411, GU372412 and GU372413 for the strains S4, S7, B7, B12, M13 and M15, respectively.
Extracellular PHB depolymerase assay
Extracellular PHB depolymerase production was assessed qualitatively by spotting strains on solid medium containing PHB particles as the sole C source as described previously (Defoirdt et al., 2007b). The medium contained 500 mg L−1 PHB particles, 1 g L−1 NH4Cl, 5 g L−1 artificial sea salt and 15 g L−1 agar. The plates were incubated at 28 °C and examined daily for the presence of a clearing zone around the colonies. Comamonas testosteroni LMG 19554 was used as a positive control (Defoirdt et al., 2007b).
Degradation of PHB in a nutrient-rich background
In order to study whether the PHB-degrading bacteria could degrade PHB in a nutrient-rich background, the bacteria were inoculated in 10% LB medium containing 1.25 g L−1 PHB at an initial density of 105 CFU mL−1. After 3 days of incubation on a shaker at 28 °C, residual PHB concentrations were determined by HPLC as described by De Schryver & Verstraete (2009). Briefly, samples were thawed in warm water and centrifuged for 10 min at 7000 g. The pellets were resuspended in 1 mL of distilled water and transferred to Eppendorf tubes. Samples were centrifuged for 5 min at 13 000 g and dried overnight at 100 °C. Dried pellets were digested with 1 mL of 96% H2SO4 at 100 °C for 1 h to form crotonic acid. The reaction mixture was then cooled to room temperature on ice, diluted 50-fold with 0.014 M H2SO4 and crotonic acid concentrations were determined by HPLC using a Dionex ASI-100 autosampler injector (Dionex Corporation, Sunnyvale, CA) equipped with an Aminex HPX-87 H ion-exchange organic acids column (300 × 7.8 mm) (Bio-Rad Laboratories, Richmond, CA). The solvent used was 0.014 M H2SO4 at a flow rate of 0.7 mL min−1. The elution peaks were monitored at 210 nm using a Dionex UV detector. The PHB content was calculated from a calibration curve for standards of commercial PHB (Goodfellow Cambridge Ltd) treated in the same manner as the samples.
N-hexanoyl-l-homoserine lactone (HHL) inactivation assay
Autoclaved LB broth was supplemented with a 0.2-μm filter-sterilized HHL (Sigma-Aldrich, Germany) solution to a final concentration of 10 mg HHL L−1 [buffered at pH 6.5 by the addition of 3-(N-morpholino)-propanesulfonic acid]. Each strain was inoculated at 106 CFU mL−1 and incubated on an orbital shaker (130 r.p.m.) at 28 °C. After 48 h, the culture was 0.2-μm filter-sterilized and the remaining HHL was measured by an agar diffusion assay using Chromobacterium violaceum CV026 as the reporter strain as described before (Baruah et al., 2009). Pseudomonas spp. P3/pME6000 and Pseudomonas spp. P3/pME6863 (=pME6000+the Bacillus AHL lactonase gene aiiA), grown under the same culture conditions, were used as the negative and positive control, respectively (Dang et al., 2009).
Culture preparation for Artemia in vivo challenge tests
Isolates B7, B12, M13 and M15 were inoculated in marine broth (Difco Laboratories, Detroit), and S4 and S7 in LB broth. After incubation for 24 h at 28 °C on an orbital shaker at 130 r.p.m., the cultures were washed with autoclaved synthetic seawater and added to the Artemia culture at c. 107 CFU mL−1.
Artemia in vivo challenge tests
Artemia franciscana (EG® Type, INVE Aquaculture, Belgium) nauplii were hatched axenically as described by Defoirdt et al. (2005). After hatching, groups of 20 nauplii were transferred into sterile 50-mL cylindrical tubes containing 20 mL filtered and autoclaved artificial seawater. The animals were challenged with 105 CFU mL−1V. campbellii LMG 21363, added to the Artemia culture water. At the start of the challenge test, a suspension of autoclaved LVS3 bacteria (Verschuere et al., 1999) was added as feed at a concentration of 107 CFU mL−1 to the culture water. In a first group of challenge tests, challenged nauplii were either untreated or treated with the isolates (107 CFU mL−1), with and without PHB (100 mg L−1). In a second challenge test, challenged nauplii were treated with PHB (100 mg L−1) in combination with either live or dead isolates. After addition of feed, PHB (100 mg L−1), isolates and pathogen, tubes were placed on a rotor at 4 r.p.m. under continuous light at 28 °C. After 48 h, the survival was determined. All treatments were performed in triplicate.
Statistics
Statistical analyses were carried out using the spss statistical software (version 15). Survival data of Artemia were compared using one-way anova, followed by Duncan's post hoc test. For all statistical analyses, a 5% significance level was used.
Results
Enrichment of PHB-degrading bacteria from intestinal microbiota
Intestinal microbiota were collected from sturgeon, sea bass and giant river prawn that had received PHB in their diets. The microbiota were enriched for PHB degraders in a medium containing PHB as the sole C source. In this medium, cell concentrations rapidly increased with 2–3 log units within 24 h after inoculation (Fig. 1a). This was similar to the growth of C. testosteroni LMG 19554, which served as a positive control (Defoirdt et al., 2007b). This indicated that the enrichment cultures were able to (partially) degrade the PHB particles into water-soluble products.
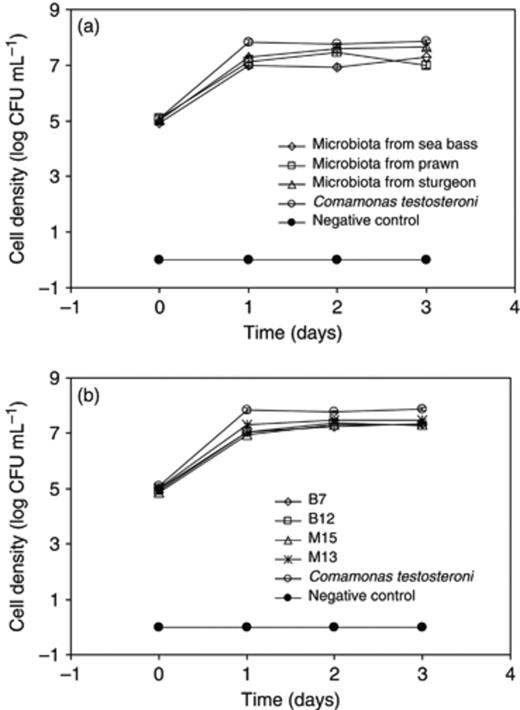
Growth of intestinal microbiota (a) and selected isolates (b) in minimal medium containing PHB as the sole C source. Noninoculated medium was used as a control. The data points represent the mean values of three replicates. Error bars are too small to be visible.
In order to confirm PHB-degrading activity, the enrichment cultures were spotted on minimal agar containing PHB particles as the sole C source. After incubation at 28 °C, clearing zones were observed for the sturgeon and sea bass microbiota, but not for the prawn microbiota (Fig. 2a–c). The production of clearing zones requires complete degradation of the PHB particles, and the absence of clearing zones for the prawn microbiota suggests weaker PHB-degrading activity under these conditions. In a further experiment, we studied the degradation of PHB in a nutrient-rich background (10% LB medium). The addition of 10% LB medium simulated the presence of other easily degradable C sources in addition to PHB, as will be the case in the intestinal environment of aquaculture animals that contains feed. After 3 days of incubation at 28 °C on an orbital shaker, a very low residual PHB concentration was measured in the cultures of the sturgeon microbiota (Table 1). Intermediate concentrations were measured in cultures of the sea bass microbiota and the highest ones in those of the prawn microbiota, which is consistent with the weaker PHB-degrading activity of these microbiota as observed on the plates with PHB as the sole C source.
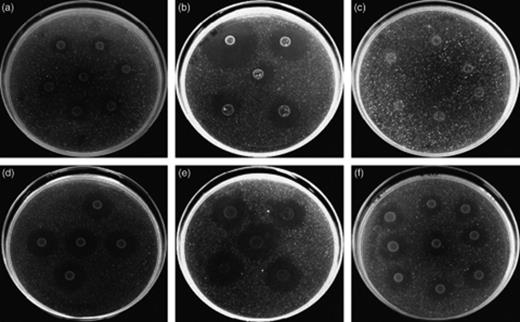
PHB degradation on minimal medium containing PHB particles as the sole C source. Complete degradation of the PHB particles results in clearing of the medium. (a) Intestinal microbiota from sturgeon, (b) intestinal microbiota from sea bass, (c) intestinal microbiota from prawn, (d) isolate S4, (e) isolate S7 and (f) the known PHB-depolymerizing bacterium Comamonas testosteroni LMG 19554.
Residual PHB concentration after culturing intestinal microbiota and selected isolates for 72 h in 10% LB medium supplemented with 1.25 g L−1 PHB
Inoculum | [PHB] after 72 h (g L−1) |
Negative control | 1.01 |
Intestinal microbiota from sturgeon | 0.01 |
Intestinal microbiota from sea bass | 0.63 |
Intestinal microbiota from giant river prawn | 0.81 |
Isolate S4 | 0.70 |
Isolate S7 | 0.68 |
Isolate B7 | 0.55 |
Isolate B12 | 0.82 |
Isolate M13 | 0.33 |
Isolate M15 | 0.82 |
Inoculum | [PHB] after 72 h (g L−1) |
Negative control | 1.01 |
Intestinal microbiota from sturgeon | 0.01 |
Intestinal microbiota from sea bass | 0.63 |
Intestinal microbiota from giant river prawn | 0.81 |
Isolate S4 | 0.70 |
Isolate S7 | 0.68 |
Isolate B7 | 0.55 |
Isolate B12 | 0.82 |
Isolate M13 | 0.33 |
Isolate M15 | 0.82 |
The initial microbial density was 105 CFU mL−1. Uninoculated medium was used as a negative control.
Residual PHB concentration after culturing intestinal microbiota and selected isolates for 72 h in 10% LB medium supplemented with 1.25 g L−1 PHB
Inoculum | [PHB] after 72 h (g L−1) |
Negative control | 1.01 |
Intestinal microbiota from sturgeon | 0.01 |
Intestinal microbiota from sea bass | 0.63 |
Intestinal microbiota from giant river prawn | 0.81 |
Isolate S4 | 0.70 |
Isolate S7 | 0.68 |
Isolate B7 | 0.55 |
Isolate B12 | 0.82 |
Isolate M13 | 0.33 |
Isolate M15 | 0.82 |
Inoculum | [PHB] after 72 h (g L−1) |
Negative control | 1.01 |
Intestinal microbiota from sturgeon | 0.01 |
Intestinal microbiota from sea bass | 0.63 |
Intestinal microbiota from giant river prawn | 0.81 |
Isolate S4 | 0.70 |
Isolate S7 | 0.68 |
Isolate B7 | 0.55 |
Isolate B12 | 0.82 |
Isolate M13 | 0.33 |
Isolate M15 | 0.82 |
The initial microbial density was 105 CFU mL−1. Uninoculated medium was used as a negative control.
Isolation of PHB-degrading strains from the enrichment cultures
The PHB-degrading enrichment cultures were spread-plated on LB agar; dominant colonies were picked and further purified. Based on preliminary tests, six isolates were retained for further experiments: S4 and S7 from sturgeon, B7 and B12 from sea bass and M13 and M15 from giant river prawn. The isolates B7, B12, M13 and M15 grew rapidly in minimal medium containing PHB as the sole C source, with a 2–3 log units increase in cell density after 24 h of incubation (Fig. 1b). It was not possible to accurately measure the cell density for isolates S4 and S7 because they were growing as flocs. However, these isolates showed clearing zone production on minimal agar containing PHB particles as the sole C source (Fig. 2d and e), indicating that they were able to degrade PHB.
The ability of the isolates to degrade PHB in a nutrient-rich background (10% LB medium) was investigated. After 3 days of incubation at 28 °C, the residual PHB concentrations were clearly lower than that in the negative (noninoculated) control for all isolates (Table 1).
Identification of the isolates
The six selected isolates obtained from the enrichment cultures were grown in LB medium, and a 1500-bp fragment of the 16S rRNA gene was amplified by PCR and sequenced. Homology searches with the blast server of the National Centre for Biotechnology Information showed that the isolates originating from the same host species were closely related (97.1%, 99.1% and 98.9% identity between the two strains isolated from sturgeon, sea bass and prawn, respectively). Isolates S4 and S7 (from sturgeon) were closely related to Acidovorax spp. (99.0% and 96.9% identity, respectively); B7 and B12 (from sea bass) were closely related to Acinetobacter spp. (99.4% and 99.2% identity, respectively); and M13 and M15 (from prawn) were closely related to Ochrobactrum spp. (99.5% and 99.3% identity, respectively).
Acylhomoserine lactone (AHL) inactivation assay
AHL degradation by the selected isolates was screened by growing them in LB medium containing 10 mg L−1 HHL. After 48 h of incubation, the remaining HHL in filter-sterilized supernatants was measured in an agar diffusion assay with the AHL reporter strain C. violaceum CV026. In this assay, the presence of AHLs in the supernatant is manifested by purple pigment production by the reporter strain. The supernatants of isolate S7 did not induce pigment production in the reporter, indicating complete degradation of HHL (Fig. 3). For the other isolates, a purple zone with approximately the same diameter as the non-HHL-degrading control occurred, indicating no or very little HHL inactivation.
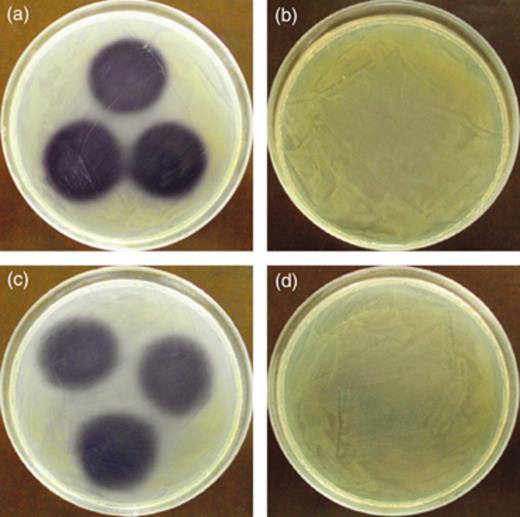
HHL degradation by the isolates. Ten microliters filter-sterilized supernatants of cultures grown for 48 h in LB medium supplemented with 10 mg L−1 HHL were spotted on LB agar covered with a lawn of the AHL reporter strain Chromobacterium violeceum CV026. The presence of HHL in the supernatants is manifested by purple pigment production in the reporter strain. (a) Negative control (P3/pME6000), (b) positive control (P3/pME6863), (c) isolate S4 and (d) isolate S7.
Effect of the isolates on the survival of brine shrimp nauplii challenged to pathogenic V. campbellii
In order to obtain a first indication of the potential of the selected isolates to protect cultured organisms from infection, we performed challenge tests in a model system with gnotobiotic brine shrimp (Artemia) nauplii. In a first series of challenge tests, the protective effect of PHB degradation by the isolates against infection with pathogenic V. campbellii was investigated. The PHB-degrading isolates were added to the culture water either with or without 100 mg L−1 PHB. This PHB concentration was chosen because previous research at our laboratories showed that it was suboptimal in protecting Artemia against infection and that the addition of a PHB-degrading bacterium could enhance the protective effect of PHB at this concentration (Defoirdt et al., 2007b). When added in combination with PHB, isolates B7, B12, M13 and M15 significantly increased the survival of Artemia nauplii when compared with untreated challenged nauplii (Fig. 4). For isolates S4 and S7, the survival was not significantly different. Therefore, these strains were not used in further challenge tests. The addition of the isolates without PHB did not result in a significant difference in survival compared with the challenged control without PHB, except for isolate B12.
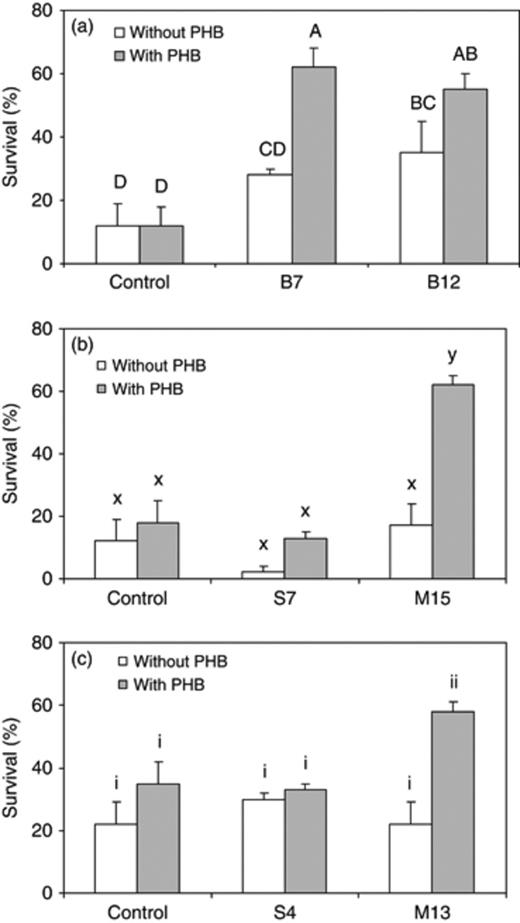
Percentage survival of Artemia nauplii after a 48-h challenge with Vibrio campbellii LMG 21363 (mean±SE of three replicates). The three different panels represent different challenge tests (performed at different times). The challenged nauplii were either untreated (control) or treated with a PHB-degrading isolate, with or without 100 mg L−1 PHB. Within each panel, treatments with a different letter are significantly different (P<0.05).
In a last challenge test, in order to confirm that the increased survival upon addition of the isolates was caused by PHB degradation and not by nutritional properties of the isolates, we studied the effect of adding the isolates dead to the culture water vs. adding them alive. The addition of live isolates resulted in a significantly higher survival of the Artemia when compared with the addition of the corresponding dead isolates, except for isolate B7 (Fig. 5). All the living isolates resulted in a higher survival than the challenged control, although the differences were not always significant.
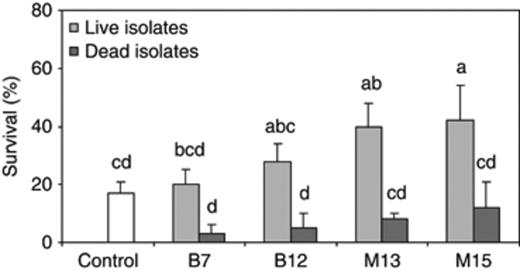
Percentage survival of Artemia nauplii after a 48-h challenge with Vibrio campbellii LMG 21363 (mean±SE of three replicates). The challenged nauplii were either not treated (control) or treated with living or dead isolates, in combination with 100 mg L−1 PHB. Treatments with a different letter are significantly different (P<0.05).
Discussion
PHB has been shown to increase pathogen resistance and to beneficially influence the growth of aquatic animals in a number of studies (Defoirdt et al., 2007b; Halet et al., 2007; De Schryver et al., 2009; Nhan et al., 2010). A method to increase these beneficial effects could be the addition of extracellular PHB-degrading bacteria (Defoirdt et al., 2009). PHB-degrading bacteria have been isolated from various environments such as soil, fresh and marine water, estuarine sediment and air (Jendrossek & Handrick, 2002). Bacteria that are used to beneficially affect a certain host need to persist and function in the specific ecological niche of the host gastrointestinal tract and, consequently, it is advisable to isolate them from this environment (or from a similar niche) in order to increase the chance of success for colonization (Reid et al., 2003). Here, we isolated PHB-degrading bacteria from intestinal microbiota of Siberian sturgeon, European sea bass and giant river prawn that had received PHB in their diets. To our knowledge, this was the first attempt to isolate PHB-degrading strains from the intestinal tract of higher animals.
PHB-degrading pure strains were isolated from PHB-degrading enrichment cultures from the intestinal microbiota of the animals and their capability to degrade PHB was confirmed in at least two of three setups: (1) growth in minimal medium containing PHB as the sole C source, (2) production of clearing zones on minimal agar containing PHB as the sole C source and (3) degradation of PHB (as determined by HPLC analysis) in 10% LB medium containing PHB. The isolates originating from sea bass and giant river prawn grew well in the minimal medium containing PHB. The sturgeon isolates were not tested in this setup because they are growing as flocs in liquid medium (and thus growth could not be measured accurately by plating). However, their PHB-degrading capability was confirmed by the production of clearing zones on agar plates containing PHB particles. All isolates were able to degrade PHB in the last setup (although to a different extent), which simulated the presence of other, easily degradable, C sources as is the case in the gastrointestinal tract of animals. Interestingly, the isolates from sturgeon showed a lower PHB-degrading capacity than the mixed bacterial community from sea bass, suggesting that the cooperation between different strains might enhance the degradation rate. The PHB-degrading isolates originating from sturgeon belonged to the genus Acidovorax. Extracellular PHB-depolymerase-producing members of this genus have been isolated previously from pond water, river water and soil (Kobayashi et al., 1999). The isolates from sea bass and giant river prawn belonged to the genus Acinetobacter and Ochrobactrum, respectively. Both genera have not been documented before to contain PHB-degrading species.
Previous research at our laboratories has shown that PHB can protect brine shrimp (A. franciscana) larvae from infection caused by the virulent pathogen V. campbellii LMG 21363 (Defoirdt et al., 2007b; Halet et al., 2007). However, in order to exert its beneficial effect, the polymer needs to be degraded into water-soluble products. The main end product of microbial PHB degradation is β-hydroxybutyrate (Jendrossek & Handrick, 2002), which has been reasoned to protect aquaculture animals from bacterial infections in two ways: (1) by providing energy to the intestinal mucosa, thereby increasing intestinal health and resistance to infections, and (2) by decreasing the growth and/or the virulence of the pathogens (Defoirdt et al., 2009). The results presented in this study showed that the PHB-degrading activity of the selected isolates increased the survival of brine shrimp larvae challenged to the pathogenic V. campbellii strain. Indeed, the addition of the isolates together with 100 mg L−1 PHB generally resulted in a higher survival than the addition of the isolates without PHB, and the addition of live isolates with 100 mg L−1 PHB resulted in a higher survival than the addition of the corresponding dead isolates with 100 mg L−1 PHB. Only strains S4 and S7 (isolated from sturgeon) were not able to protect the brine shrimp larvae from the pathogen, which can be ascribed to two factors: (1) both strains were growing as flocs in liquid medium and most of them were too large to be ingested by the brine shrimp larvae. Indeed, brine shrimp graze on particles with a diameter preferentially smaller than 50 μm (Sorgeloos et al., 1986) and (2) these strains were isolated from a freshwater environment and were probably not active in the brine shrimp cultures (which were performed in seawater). Indeed, these isolates did not grow in LB medium with a high salt concentration (35 g L−1). In contrast, the isolates from sea bass and giant river prawn were euryhaline (able to grow between 5 and 35 g L−1), which might make them useful under a broad range of environmental conditions.
Quorum-sensing, bacterial cell-to-cell communication with small signal molecules has been shown to regulate the virulence of several pathogenic bacteria, including aquaculture pathogens (Defoirdt et al., 2004). Several types of signal molecules have been identified, with the best-studied ones being AHLs. Previous research at our laboratories has shown that AHL-degrading bacteria can readily be isolated from the intestinal microbiota of aquatic animals and that these bacteria can protect cultured animals from bacterial infections (Dang et al., 2009). Therefore, AHL degradation by the selected isolates was screened by growing them in LB medium containing 10 mg L−1 HHL. This experiment revealed that isolate S7 was able to degrade HHL. No AHL-degrading bacteria belonging to the genus Acidovorax have been reported thus far. However, the 16S rRNA gene of isolate S7 showed 92.6% identity with the sequence of Variovorax paradoxus S110 (GenBank accession number CP001635.1) and V. paradoxus is a known AHL-degrading species (Leadbetter & Greenberg, 2000). Hence, strain S7 displays two beneficial anti-infective characteristics (PHB degradation and quorum-sensing disruption), and consequently, although it did not reveal any positive effect toward brine shrimp larvae in this study, it certainly is worthwhile to evaluate its beneficial potential in its original host.
To conclude, this research was the first to report the isolation of PHB-degrading bacteria from a gastrointestinal environment. The addition of selected PHB-degrading isolates together with PHB significantly increased the survival of brine shrimp larvae infected with the virulent pathogen V. campbellii LMG 21363. The effects noted in the challenge tests represent an increased survival by a factor 2–3, which is highly relevant for practice. Moreover, the dose of the PHB involved (100 mg L−1) is economically feasible. Finally, the isolates involved are not related to aquaculture disease-associated genera and are easy to propagate. Hence, they offer possibilities for production at a technically relevant scale. Detailed studies on the use of the strains as probiotic feed components for the animals from which they were isolated will be the subject of our further research. These studies will reveal whether the isolates are active in different hosts and whether they can establish and maintain themselves in the intestinal tract of these hosts or whether repeated addition is necessary.
Authors' contribution
Y.L. and P.D.S. contributed equally to this work.
Acknowledgements
This work was performed and funded within the frame of the Research Foundation of Flanders (FWO) project ‘Probiont-induced functional responses in aquatic organisms’ and the European FP7 project ‘Promicrobe – Microbes as positive actors for more sustainable aquaculture’ (project reference: 227197). Additional financial support was obtained from a Master Grant from Ghent University to Y.L. and an FWO Postdoc Grant to T.D.
References
Author notes
Editor: Julian Marchesi