-
PDF
- Split View
-
Views
-
Cite
Cite
Christophe Chassard, Eve Delmas, Céline Robert, Annick Bernalier-Donadille, The cellulose-degrading microbial community of the human gut varies according to the presence or absence of methanogens, FEMS Microbiology Ecology, Volume 74, Issue 1, October 2010, Pages 205–213, https://doi.org/10.1111/j.1574-6941.2010.00941.x
- Share Icon Share
Cellulose-degrading microorganisms involved in the breakdown of plant cell wall material in the human gut remain rather unexplored despite their role in intestinal fermentation. Microcrystalline cellulose-degrading bacteria were previously identified in faeces of methane-excreting individuals, whereas these microorganisms were undetectable in faecal samples from non-methane excretors. This suggested that the structure and activity of the cellulose-degrading community differ in methane- and non-methane-excreting individuals. The purpose of this study was to characterize in depth this cellulose-degrading community in individuals of both CH4 statuses using both culture-dependent and molecular methods. A new real-time PCR analysis was developed to enumerate microcrystalline cellulose-degrading ruminococci and used to confirm the predominance of these hydrolytic ruminococci in methane excretors. Culture-dependent methods using cell wall spinach (CWS) residue revealed the presence of CWS-degrading microorganisms in all individuals. Characterization of CWS-degrading isolates further showed that the main cellulose-degrading bacteria belong essentially to Bacteroidetes in non-methane-excreting subjects, while they are predominantly represented by Firmicutes in methane-excreting individuals. This taxonomic diversity was associated with functional diversity: the ability to degrade different types of cellulose and to produce H2 from fermentation differed depending on the species. The structure of the cellulolytic community was shown to vary depending on the presence of methanogens in the human gut.
Introduction
Dietary fibres, i.e. indigestible plant residues, are important in maintaining healthy gastrointestinal functions. These fibres enter the human colon, where they are fermented by the gut microbial community (Flint et al., 2007). Microbial fermentation of dietary fibres is now recognized as providing human health benefits, for example regulation of digestive transit and a preventive role in cardiovascular diseases and cancers through the production of short-chain fatty acids and release of plant polymer-associated bioactive compounds with antioxidant activity (Schweizer & Edwards, 1991). Plant cell wall polysaccharides, mainly cellulose, hemicelluloses and pectin, found in cereals, vegetables and fruits, represent one of the most important fractions of dietary fibres. The daily consumption of plant cell wall polysaccharides varies from 10 to 25 g in European adults or about 30% of the total dietary fibre ingested (Lairon et al., 2003, 2005). Despite the potential health benefits associated with microbial digestion of these substrates, studies on gut bacterial communities involved in the breakdown and fermentation of these insoluble polysaccharides are lacking. Attempts to elucidate the cellulose-degrading microbial community have only been partially successful as only a restricted number of individuals appear to harbour such cellulose-degrading organisms (Bétian et al., 1977; Montgomery, 1988; Wedekind et al., 1988). We demonstrated previously that the presence or absence of microcrystalline cellulose-degrading bacteria was related to the methane status of volunteers (Robert & Bernalier-Donadille, 2003). This population was found in the faecal samples of the majority of methane-excreting subjects (CH4+) studied, while it was not detected in specimens from non-methane-excreting individuals (CH4−). The dominant microcrystalline cellulose-degrading species isolated from CH4+ subjects belong to new Ruminococcus sp. and some Enterococcus sp. closely related to Enterococcus faecalis (Robert & Bernalier-Donadille, 2003). These microcrystalline cellulose-degrading species were shown to produce high amounts of H2 from cellulose fermentation.
The absence of cultivable microcrystalline cellulose-degrading organisms in faecal samples from CH4− individuals does not reflect the total absence of cellulose-degrading bacteria in this non-methane-producing ecosystem as both cellulase and xylanase activities could be detected in this faecal microbial community (Robert & Bernalier-Donadille, 2003). A unique isolate described as Bacteroides cellulosilyticus (Robert et al., 2007), a new Bacteroides sp. able to grow with pure celluloses, was isolated recently from a CH4− subject. We therefore hypothesize that the structure and activity of the cellulolytic community from CH4− individuals may differ from that of CH4+ individuals. The objective of the present study was to characterize in depth the cellulose-degrading community from the gut of CH4− and CH4+ individuals using both culture-dependent and molecular approaches. Populations of microcrystalline cellulose-degrading ruminococci and methanogens were first investigated using real-time PCR analysis in a large number of subjects (n=49). Culture-dependent studies were further performed using different types of pure celluloses and cell wall spinach (CWS) residue in order to identify cellulose-degrading organisms, especially in CH4− individuals.
Materials and methods
Human subjects
Forty-nine healthy subjects, aged 20–54 years, were recruited for the present study. All consumed a diversified Western diet including 10–15 g of fibre per day. No vegetarians were included in the study. All volunteers had a normal body mass index (BMI between 18 and 25 kg m−2). None had received antibiotics during the 3 months preceding the study and none had any previously diagnosed gastrointestinal disease. All participants gave informed consent to the protocol, which was approved by the local ethics committee.
Breath methane concentration was determined using a Microlyser-SC gas chromatograph (Quintron Instrument, Biotech, France). Twenty-nine volunteers, who did not expire any CH4, were selected as non-methane excretors (CH4−). The other 20 subjects, selected as methane excretors (CH4+), excreted CH4 at a concentration in excess of at least 1 p.p.m. of the ambient atmosphere (Bond et al., 1971).
Collection and preparation of faecal samples for analyses
Freshly voided faeces were obtained from all subjects, stored at 4 °C under anaerobic conditions and processed within a few hours. Each sample was mixed, and five aliquots were stored at −80 °C for DNA extraction and quantitative real-time PCR analysis. One gram of faecal sample was collected from 18 selected volunteers (nine CH4− and nine CH4+) and was 10-fold (wet w/v) diluted in an anaerobic mineral solution. Serial 10-fold dilutions to 10−11 were then carried out for culture-dependent analysis.
Quantitative real-time PCR analysis
Population levels of microcrystalline cellulose-degrading ruminococci and methanogens were evaluated in faecal samples from CH4+ (n=20) and CH4− individuals (n=29) using real-time PCR (LightCycler™ system, Roche Molecular Biochemicals Mannheim, Germany).
Primers specifically targeting the 16S rRNA gene of the new cellulose-degrading Ruminococcus phylum (Robert & Bernalier-Donadille, 2003) were designed and validated (RumcelF: GTTCAGTCCTTCGGGACACAA, RumcelR: TAGCAATTCCGACTTCAT, amplicon=336 pb). Primers were initially selected based on the sequence alignment of cellulose-degrading human strains, Ruminococcus sp. from clostridial cluster IV and XIVa, while the specificity of the primers was checked with the Probe match function of the Ribosome Database Project II. Primer specificity was verified using a panel of >20 strains belonging to the major bacterial genera found in humans and two major cellulose-degrading Ruminococcus sp. (Ruminococcus albus and Ruminococcus flavefaciens) from the rumen ecosystem (Table 1). Amplification was performed in a total volume of 25 μL with a primer concentration of 0.5 μmol L−1 each according to the following protocol: one cycle of denaturation (95 °C for 10 min), 35 cycles of amplification (95 °C for 15 s, 60 °C for 10 s and 72 °C for 30 s), one melting curve programme (65–95 °C with a heating rate of 0.1 °C s−1) and a cooling step to 45 °C. Standard template DNA was prepared from the 16S rRNA gene of Ruminococcus sp. strain 18P13 (DSMZ 18848) by amplification with F8 (5′-AGAGTTTGATCMTGGCTC-3′) and 1492R (5′-GNTACCTTGTTACGACTT-3′) primers and purification as described previously (Mosoni et al., 2007). Standard curves were prepared with five standard concentrations from 10-fold serial dilutions in water, ranging from 108 to 104 copies. Methanogens were quantified using the primers described previously and following the conditions of analysis defined by Ohene-Adjei et al. (2007).
Validation of 16S rRNA gene primers for real-time PCR analysis of human cellulolytic ruminococci
+, species detected; −, species not detected.
+, reported as cellulose-degrading species (Chesson & Forsberg, 1988; Robert & Bernalier-Donadille, 2003; Robert et al., 2007); −, not reported as cellulose-degrading species.
¶This study.
Validation of 16S rRNA gene primers for real-time PCR analysis of human cellulolytic ruminococci
+, species detected; −, species not detected.
+, reported as cellulose-degrading species (Chesson & Forsberg, 1988; Robert & Bernalier-Donadille, 2003; Robert et al., 2007); −, not reported as cellulose-degrading species.
¶This study.
Media and enumeration procedures
All liquid and solid media were prepared, dispensed and inoculated using strictly anaerobic techniques (Hungate, 1969), with 100% O2-free CO2 gas. Total anaerobes, cellulose and CWS-degrading bacteria, and methanogens were enumerated using the most probable number (MPN) estimation (Clarke & Owens, 1983). Three tubes were inoculated per dilution for each cellulosic substrate and for total anaerobe enumeration.
Total anaerobes were enumerated in a complex medium containing clarified rumen fluid (Leedle & Hespell, 1980) and bacterial growth was recorded in culture tubes of each dilution inoculated (10−7–10−11) after 48 h of incubation at 37 °C.
Cellulose- and CWS-degrading bacteria were enumerated in basal cellulolytic (BC) medium as described previously (Robert & Bernalier-Donadille, 2003). Two pure celluloses (Whatman no. 1 filter paper cellulose and Sigmacell® 101 cellulose) and a CWS extract were used as substrates. CWS residue was obtained by pebble milling fresh spinach in liquid nitrogen as described previously (Robert et al., 2007). A strip of 50 mg Whatman no. 1 filter paper cellulose was added to each culture tube before the addition of the prereduced BC medium (10 mL per tube). After incubation at 37 °C for 21 days, cellulose utilization in the cultures obtained from each dilution inoculated (10−3–10−9) was detected visually by noting the degradation of the piece of filter paper. Sigmacell® 101 cellulose and cell wall spinach were rehydrated in distilled water and solutions of each substrate (2%) were pebble milled for one night before addition to the BC medium at a final concentration of 0.4%. After incubation at 37 °C for 15 days, the presence of endoglucanase activity, the carboxymethyl cellulase (CMCase), was examined in each culture tube inoculated with faecal dilutions (from 10−3 to 10−9). CMCase activity was detected on agar plates containing the following mixture: 50 mM sodium phosphate buffer (pH 6.8), 0.3% carboxymethylcellulose (Sigma Chemicals Co., St. Louis) and 1.5% bacteriologic agar type A (Biokar Diagnostics). Supernatant fluids (0.2 mL) of each culture were deposited in a hole formed in the agar mixture and plates were incubated for 10 h at 37 °C. The CMCase activity was visualized with Congo red after extensive washing of the agar plates with 1M NaCl (Forano et al., 1994).
H2-utilizing methanogens were enumerated as described by Doré (1995). Briefly, faecal sample dilutions from 10−2 to 10−9 were inoculated into broth culture medium. After inoculation, culture tubes were pressurized with H2/CO2 (80/20 v/v, 202 kPa). Vials with CH4 levels above 0.1% after 15 days of incubation at 37 °C were considered positive.
Isolation of CWS-degrading bacteria from faecal samples
Enrichments of CWS-degrading bacteria were obtained from the highest dilution tubes in MPN estimation in CWS–BC medium, showing CMCase activity. Two to three weekly subcultures were performed in CWS-BC broth medium before transferring the enriched cultures into roll tubes containing the same medium with 2% Bacto agar and 0.7% hydrated CWS residue. Colonies developing in agar medium were transferred from roll tubes into a CWS–BC broth medium. The CMCase activity of the culture was then checked on the agar mixture as described above. Two to five successive subcultures on roll tubes and broth BC medium were carried out to obtain a pure culture of isolates showing CMCase activity. Isolates were then examined for purity, morphology and Gram staining by bright-field microscopy, in CWS- and glucose (2 g L−1)-grown cultures.
Degradation of CWS and pure celluloses by isolates
CWS-degrading isolates were cultivated in a liquid BC medium (10 mL per tube) containing 100 mg of Avicel® or Sigmacell® 101 cellulose or 100 mg of CWS as the sole energy source. Inocula were composed of 0.3 mL of 5-day-old cultures on a CWS–BC medium. Three culture tubes were inoculated for each incubation time and each strain studied. Substrate degradation by the isolates was determined by measuring the dry weight of the substrate remaining in the culture tubes after 10 days of incubation at 37 °C (Chassard et al., 2005).
Fermentation of pure celluloses and CWS by isolates
Fermentation end-products of each isolate were determined in the 10-day-old cultures obtained with the different pure celluloses or CWS as the sole energy source. Headspace gases were analysed by gas-phase chromatography (Chassard et al., 2005), and short-chain fatty acid production was quantified by 1D 1H NMR (Chassard et al., 2008).
Sequencing of the 16S rRNA gene and phylogenetic placement of CWS-degrading isolates
Sequencing of the 16S rRNA gene and phylogenetic assignment of isolates were performed as described previously (Chassard et al., 2007). The 16S rRNA gene (c. 1500 bp) was amplified using the universal primers F8 and 1492R. Two PCR reactions were performed per strain. PCR products were purified using the Strataprep® PCR Purification kit (Ozyme, St Quentin en Yvelines, France) according to the manufacturer's instructions. The concentration of amplified DNA was estimated in ethidium bromide-stained agarose gels by densitometry and c. 50 ng of each product was included in a 20-μL sequencing reaction. Amplicons were sequenced to full length in both directions using an ABI Prism BigDie terminator cycle sequencing kit (Perkin Elmer Corporation, Norwalk, CT) with appropriate primers by the ABI Prism 310 genetic analyzer (Perkin Elmer Corporation).
Data and statistical analysis
The results of bacterial counts are reported as log10 microorganisms per gram wet weight of faeces. Data were expressed as mean±SE (n=number of faecal samples studied). Statistical analyses were performed with instat 3.0 graphpad software using Student's t-test. All tests were two-tailed and the level used to establish significance was P<0.05. The statistical correlation between bacterial population levels was studied using the linear Pearson correlation.
Results
Quantification of population levels of human microcrystalline cellulose-degrading ruminococci and methanogens in faecal samples from CH4+ and CH4− individuals
Newly designed primers for human cellulose-degrading ruminococci enumeration were validated successfully for specificity (Table 1). A broad range of dominant species including Ruminococcus sp. with a human origin and major cellulose-degrading Ruminococcus sp. from the rumen ecosystem (R. albus and R. flavefaciens) were tested. All microcrystalline cellulose-degrading Ruminococcus strains previously isolated from the human gut (Robert & Bernalier-Donadille, 2003) were enumerated using RumcelF and RumcelR primers. Amplification was observed neither with other human intestinal bacteria nor with cellulose-degrading Ruminococcus sp. of ruminal origin (Table 1). As expected, the population levels of methanogens were significantly higher in CH4+ individuals than in CH4− ones (log 7.8±1.2 vs. log 3.2±0.8 g faeces, respectively). The population of human microcrystalline cellulose-degrading ruminococci was detected in the majority of subjects regardless of the methane excretion status (Fig. 1), and this population level ranged from log 6.1 to log 9.2 g faeces depending on the subject considered. The level of this ruminococci population was, however, significantly higher (P<0.0001) in CH4+ individuals (log 8.1±0.6 g faeces) than in CH4− individuals (log 7.2±0.5 g faeces). A correlation (r2=0.463) was observed between the population levels of human microcrystalline cellulose-degrading ruminococci and methanogens in faecal samples of CH4+ and CH4− subjects (Fig. 1). In CH4+ subjects, a high population level of methanogens was determined to correlate significantly (P=0.008) with a high population level of human cellulose-degrading ruminococci.
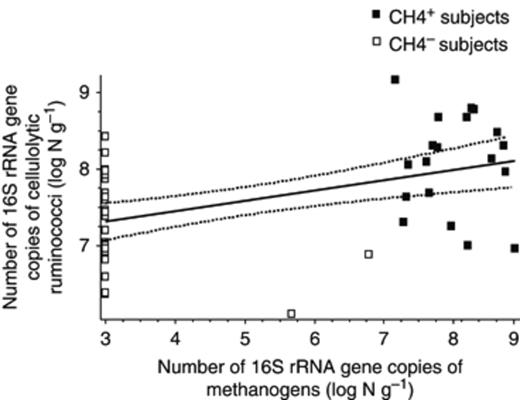
Correlation between human cellulolytic ruminococci and methanogen populations in faecal samples from methane-producing (CH4+) and non-methane-producing (CH4−) faecal samples (n=49), estimated by quantitative real-time PCR.
Evaluation of the cellulose- and CWS-degrading population in faecal samples from CH4+ and CH4− individuals
The population levels of cultivable cellulose- and CWS-degrading communities were estimated in faecal samples using different types of substrates. The results of these bacterial counts are shown in Table 2. As reported previously (Robert & Bernalier-Donadille, 2003), microcrystalline (filter paper) cellulose-degrading bacteria could not be cultivated from CH4− faecal samples while the level of this population reached 106–107 g−1 faeces in CH4+ faecal specimens. The use of Sigmacell®101 cellulose allowed the detection of cellulose-degrading organisms in all CH4+ faecal samples as well as in six of the nine CH4− individuals studied. The Sigmacell®101 cellulose-degrading community was detected at similar levels in CH4+ and in the six CH4− individuals, with the average number of these bacteria nearly reaching 107 g−1 faeces. The Sigmacell®101 cellulose-degrading population, however, remained undetectable (<103 g−1 faeces) in three CH4− faecal samples. By contrast, CWS-degrading bacteria were found in all faecal samples from both CH4+ and CH4− subjects. The average number of the CWS-degrading population was not significantly different between CH4+ and CH4− individuals, with the population levels of this community varying from 106 to 109 bacteria g−1 faeces, depending on the faecal sample considered. The CWS-degrading population was detected at higher levels than either the filter paper- or the Sigmacell® 101-degrading communities, in both CH4− and CH4+ individuals.
Distribution of the cellulose- and cell wall spinach-degrading populations in faecal samples from methane-excreting (CH4+) and non-methane-excreting (CH4−) individuals (n=18)
Subjects | Microbial counts (log10 N g−1 faeces) | ||||
Total anaerobes | Filter paper-degrading bacteria | Sigmacell® 101-degrading bacteria | Cell wall spinach-degrading bacteria | Methanogenic archaea | |
CH4+ | 11.3 ± 0.9 | 6.3 ± 0.9 | 7.7 ± 1.6 | 8.6 ± 0.8 | 8.8 ± 1.1 |
n=9 | [9.9–12.2] | [5.7–7.7] | [5.3–9.5] | [7.9–9.5] | [7.0–9.9] |
CH4− | 10.7 ± 0.6 | <3.0 | 6.6 ± 2.9 | 7.8 ± 0.9 | 4.5 ± 1.8 |
n=9 | [9.9–11.9] | [3.0–9.9] | [6.2–9.2] | [3.0–7.6] | |
P | NS | 0.001 | NS | NS | 0.001 |
Subjects | Microbial counts (log10 N g−1 faeces) | ||||
Total anaerobes | Filter paper-degrading bacteria | Sigmacell® 101-degrading bacteria | Cell wall spinach-degrading bacteria | Methanogenic archaea | |
CH4+ | 11.3 ± 0.9 | 6.3 ± 0.9 | 7.7 ± 1.6 | 8.6 ± 0.8 | 8.8 ± 1.1 |
n=9 | [9.9–12.2] | [5.7–7.7] | [5.3–9.5] | [7.9–9.5] | [7.0–9.9] |
CH4− | 10.7 ± 0.6 | <3.0 | 6.6 ± 2.9 | 7.8 ± 0.9 | 4.5 ± 1.8 |
n=9 | [9.9–11.9] | [3.0–9.9] | [6.2–9.2] | [3.0–7.6] | |
P | NS | 0.001 | NS | NS | 0.001 |
NS, not statistically different (P>0.05).
Distribution of the cellulose- and cell wall spinach-degrading populations in faecal samples from methane-excreting (CH4+) and non-methane-excreting (CH4−) individuals (n=18)
Subjects | Microbial counts (log10 N g−1 faeces) | ||||
Total anaerobes | Filter paper-degrading bacteria | Sigmacell® 101-degrading bacteria | Cell wall spinach-degrading bacteria | Methanogenic archaea | |
CH4+ | 11.3 ± 0.9 | 6.3 ± 0.9 | 7.7 ± 1.6 | 8.6 ± 0.8 | 8.8 ± 1.1 |
n=9 | [9.9–12.2] | [5.7–7.7] | [5.3–9.5] | [7.9–9.5] | [7.0–9.9] |
CH4− | 10.7 ± 0.6 | <3.0 | 6.6 ± 2.9 | 7.8 ± 0.9 | 4.5 ± 1.8 |
n=9 | [9.9–11.9] | [3.0–9.9] | [6.2–9.2] | [3.0–7.6] | |
P | NS | 0.001 | NS | NS | 0.001 |
Subjects | Microbial counts (log10 N g−1 faeces) | ||||
Total anaerobes | Filter paper-degrading bacteria | Sigmacell® 101-degrading bacteria | Cell wall spinach-degrading bacteria | Methanogenic archaea | |
CH4+ | 11.3 ± 0.9 | 6.3 ± 0.9 | 7.7 ± 1.6 | 8.6 ± 0.8 | 8.8 ± 1.1 |
n=9 | [9.9–12.2] | [5.7–7.7] | [5.3–9.5] | [7.9–9.5] | [7.0–9.9] |
CH4− | 10.7 ± 0.6 | <3.0 | 6.6 ± 2.9 | 7.8 ± 0.9 | 4.5 ± 1.8 |
n=9 | [9.9–11.9] | [3.0–9.9] | [6.2–9.2] | [3.0–7.6] | |
P | NS | 0.001 | NS | NS | 0.001 |
NS, not statistically different (P>0.05).
Isolation of CWS-degrading bacteria from faecal samples
Enrichments of CWS-degrading bacteria were obtained from seven faecal samples of subjects harbouring high levels (107–108) of CWS-degrading bacteria. The highest dilution cultures from MPN estimation exhibiting carboxymethylcellulase activity were used for the isolation of CWS-degrading organisms (Table 3). These cultures were transferred two to three times on CWS–BC medium. A total of 14 CWS-degrading strains were then obtained after three to four successive isolations on agar roll tubes with CWS as a substrate.
Origin and phylogenic analysis of CWS-degrading strains isolated from seven human faecal samples according to the methane status
The population level is expressed as log N g−1 faeces.
Origin and phylogenic analysis of CWS-degrading strains isolated from seven human faecal samples according to the methane status
The population level is expressed as log N g−1 faeces.
The seven isolates obtained from three CH4− faecal samples were Gram-negative coccobacillus (Table 3) and expressed CMCase activity in CWS-broth cultures. Seven different strains were obtained from CH4+ faecal samples and all exhibited positive or variable Gram staining (Table 3). Three strains were identified as facultative anaerobes (strains 7SE20, 8SE23, 8SE26), while only one strain was identified as a Gram-positive coccus (strain 9SE51). Three other strains (11SE37, 11SE38 and 11SE39) were identified as Gram-variable curved rods. All isolates expressed CMCase activity in CWS broth medium.
Sequencing of the 16S rRNA gene of CWS-degrading isolates
A nearly complete sequence of the 16S rRNA gene (around 1400 nucleotides) was determined for the 14 CWS-degrading isolates. Sequence similarity analysis showed that all Gram-negative coccobacilli isolated from CH4− faecal samples belonged to the Bacteroides taxon (Eckburg et al., 2005) (Table 3). Phylogenetic analysis further demonstrated that these isolates could be classified into only one subgroup that was closely related (at least 98% of 16S rRNA gene sequence similarity) to B. cellulosilyticus, the cellulose-degrading Bacteroides sp. described previously by Robert et al. (2007). The 16S rRNA gene sequences of these Bacteroides isolates were deposited in the EMBL database (AJ583244, AJ583245, AJ583246, AJ583247, AJ583248 and AJ583249).
16S rRNA gene sequence analysis of the three facultative anaerobes (strains 7SE20, 8SE23, and 8SE26) demonstrated that these isolates could be assigned to Enterococcus sp., as their sequence showed 99% similarity to E. faecalis (Table 3). Strain 9SE51 was assigned to the Ruminococcus genus (cluster IV of Clostridiacaea), with the closest relative of this isolate corresponding to the microcrystalline cellulose-degrading Ruminococcus sp. described previously by Robert & Bernalier-Donadille (2003). The 16S rRNA gene sequence of this Ruminococcus isolate was deposited in the EMBL database (FM954974). The three identified Gram-variable curved rods (strains 11SE37, 11SE38 and 11SE39) were assigned to the genus Roseburia (Table 3), with their sequences (EMBL accession numbers FM954975, FM954976 and FM954977) showing 99% similarity to the type strain of Roseburia faecis.
Ability of different CWS-degrading isolates to degrade and ferment pure celluloses and CWS
Four CWS-degrading species, all representatives of the various phylogenetic groups of isolates described by Robert & Bernalier-Donadille (2003) and identified in this study, were analysed for their ability to degrade and ferment both celluloses and CWS (Fig. 2). Bacteroides isolates degraded different types of pure cellulose such as Avicel® pH-101 in addition to CWS, but were unable to degrade microcrystalline (filter paper) cellulose. In contrast, Roseburia isolates degraded CWS, but not the pure celluloses tested (Avicel® pH-101, Sigmacell® 101 and filter paper). Enterococcus and Ruminococcus isolates degraded all different types of celluloses including filter paper cellulose. Furthermore, these Gram-positive species were also particularly efficient in degrading CWS (Fig. 2).
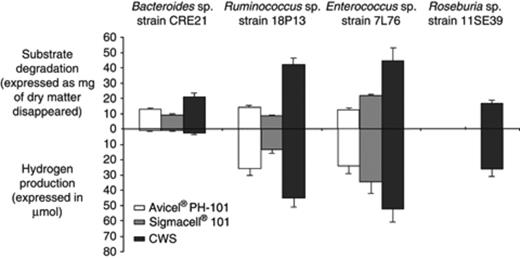
Cellulose or CWS disappearance and production of H2 by Bacteroides sp., Ruminococcus sp., Enterococcus sp. and Roseburia sp. after 10 days of incubation at 37°C. Substrate degradation is expressed as mg of dry matter disappeared and H2 production in μmol.
Metabolite production and ratios varied depending on the strain considered. Bacteroides sp. produced mainly acetate, propionate and succinate during cellulose metabolism, while Enterococcus and Ruminococcus isolates synthesized mostly acetate and succinate and Roseburia sp. formed butyrate (data not shown). The production of hydrogen also varied considerably depending on the species (Fig. 2). Bacteroides sp. produced only small amounts of hydrogen in cellulose-grown cultures, while Enterococcus, Ruminococcus and Roseburia sp. produced large amounts of this gas from cellulose and/or CWS fermentation.
Discussion
The present study demonstrates that cellulose-degrading organisms are present in the gut microbiota of all individuals, but that the structure of this cellulose-degrading community differs largely depending on the CH4 status of the subject. The dominant cellulose degraders isolated from non-methane-excreting subjects belong mainly to Bacteroidetes, while they are predominantly composed of Firmicutes in methane-excreting individuals.
Enumeration of cellulose-degrading organisms in human faecal samples appears to be dependent on the type of cellulose used in the culture medium. This hypothesis stems from our previous results (Robert & Bernalier-Donadille, 2003), demonstrating that microcrystalline cellulose-degrading bacteria could only be detected in methane-excreting subjects. The relationship between a detectable microcrystalline cellulose-degrading population and the CH4 status of the volunteers was confirmed in the present study. We further demonstrate here that the population level of the main microcrystalline cellulose-degrading Ruminococcus sp. identified previously (Robert & Bernalier-Donadille, 2003) correlates with the population levels of methanogens in the gut. While this Ruminococcus group was detected in all faecal samples, this bacterial group was determined to represent a subdominant community in CH4− individuals. However, no microcrystalline cellulose-degrading Ruminococcus sp. could be cultivated from CH4− subjects, suggesting that some members of this phylogenetic subgroup could be non-cellulose-degraders such as Ruminococcus bromii, another member of the cluster IV subgroup capable of degrading resistant starch, but not cellulose.
The use of crystalline Sigmacell® 101 cellulose was not as successful in detecting the cellulose-degrading community in faeces from both CH4+ and CH4− subjects. The presence of the Sigmacell® 101 cellulose-degrading population was not related to the CH4 status of volunteers in contrast to the microcrystalline cellulose-degrading population. However, Sigmacell® 101 cellulose-degrading organisms could not be found in three of the nine CH4− faecal samples examined. In contrast, CWS-degrading populations were detected in all subjects, regardless of the methane excretion status. CWS enrichments were therefore selected for further isolation of CWS-degrading organisms. CWS was determined to be an effective substrate for the exploration of the bacterial diversity of cellulose-degrading microorganisms in humans. All bacterial species isolated previously from CH4+ subjects using microcrystalline (filter paper) cellulose (Robert & Bernalier-Donadille, 2003) were also identified using CWS as a substrate. Several more species belonging to the Bacteroides and Roseburia genera were also identified using CWS as a substrate. All seven CWS-degrading strains isolated from CH4− individuals were determined to be closely related to the cellulose-degrading Bacteroides sp., B. cellulosilyticus (Robert et al., 2007). These different Bacteroides isolates were determined as belonging to the same Bacteroides phylotype despite being isolated from three different subjects.
Bacteroides represents a predominant taxon in the colon (Eckburg et al., 2005; Chassard et al., 2008). Several species within this taxon have been shown to express xylanolytic activity (Bacteroides ovatus, Bacteroides fragilis, Bacteroides xylanisolvens) (Hespell & Whitehead, 1990; Chassard et al., 2007); however, very few species exhibited clear cellulose-degrading activity. Only B. cellulolysiticus (Robert et al., 2007) and one other Bacteroides-like strain isolated from human faeces by Bétian et al. (1977) have been reported to be capable of degrading pure cellulose. Identification of the B. cellulosilyticus subgroup through our enrichment cultures suggests that this subgroup may represent an important cellulose-degrading Bacteroides phylotype of the human gut. This finding further supports the hypothesis that the predominant Bacteroidetes contribute to plant cell wall degradation in the human gut through hydrolysis of main polysaccharides such as xylan and cellulose (Macfarlane & Macfarlane, 2006). Our attempt to specifically quantify the B. cellulosilyticus subgroup by real-time PCR in faecal samples remains elusive. Primer sets designed for this Bacteroides subgroup were capable of detecting the targeted cellulose-degrading species; however, other non-cellulose-degrading Bacteroides sp. were also quantified. This is likely attributed to the close phylogenetic relationship between B. cellulosilyticus and some predominant Bacteroides sp. found in the human gut such as Bacteroides intestinalis (Robert et al., 2007).
While CWS-degrading isolates from CH4− faecal samples were determined as belonging to the Bacteroidetes phylum, CWS-degrading isolates obtained from faecal samples of CH4+ subjects were identified as Firmicutes and could be assigned to different bacterial genera including Ruminococcus, Enterococcus and Roseburia. This result supports and extends the previous hypothesis (Robert & Bernalier-Donadille, 2003) of Gram-positive cellulose-degrading organisms dominating in CH4+ individuals. Although Ruminococcus and Enterococcus isolates were mainly obtained using microcrystalline cellulose as a substrate (Robert & Bernalier-Donadille, 2003), CWS represented a more favourable substrate for the growth and isolation of Enterococcus sp. and Roseburia sp. The distribution of these two bacterial subgroups in CH4+ and CH4− subjects was not evaluated in this study. Roseburia sp. are an important group of butyrate-producing bacteria that predominate in the gut of all healthy human individuals (Aminov et al., 2006; Duncan et al., 2006), while Enterococcus sp. are also indigenous, but subdominant bacterial species. The role of Enterococcus sp. and Roseburia sp. in cellulose breakdown in the gut has not been explored extensively in contrast to the Ruminococcus sp., which are predominant cellulose degraders in the rumen (Flint et al., 2008).
The taxonomic diversity of the cellulose-degrading community found in CH4+ and CH4− individuals strongly correlated with functional diversity. The ability to degrade and ferment different types of cellulose varied considerably depending on the bacterial species considered. Bacteroides isolates degraded pure celluloses, but not microcrystalline filter paper, while Ruminococcus sp. and Enterococcus sp. efficiently hydrolysed all types of substrates (Robert & Bernalier-Donadille, 2003). Roseburia sp. expressed CMCase activity when grown on CWS, but were unable to grow on pure cellulose. Roseburia sp. were also shown to express high xylanase activity (Chassard et al., 2007), suggesting that this bacterial species was selected on CWS medium because of its ability to use other complex polysaccharides present in CWS. Roseburia sp. could thus potentially interact with cellulose-degrading microorganisms to ensure efficient degradation of complex substrates such as spinach in the human gut.
While the different Bacteroides isolates did not produce H2 from cellulose fermentation, large amounts of this gas were released by the dominant cellulose-degrading organisms isolated from CH4+ individuals (Robert & Bernalier-Donadille, 2003; Chassard et al., 2005). Thus, different cellulose-degrading species may contribute differently to H2 production during fibre fermentation in the human gut. The presence of H2-producing cellulolytic species in CH4+ subjects, but not in CH4− individuals, further implies that the structure of this fibrolytic community may vary according to the presence or absence of methanogens in the gut ecosystem.
Cross-feeding between H2-producing and H2-consuming microorganisms is a crucial mechanism, essential for the maintenance of efficient degradation and fermentation of organic matter. Hydrogen produced at high levels by several hydrolytic and saccharolytic species in CH4+ subjects should be used by methanogens, to ensure an optimal fermentation of substrates (Robert et al., 2001). This bacterial equilibrium could, however, be impaired in certain situations, leading to lower or higher energy salvage of the gut microbiota from the diet (Samuel & Gordon, 2006; Turnbaugh et al., 2006). The disturbance of this equilibrium could further impact the ability of the host to harvest and store calories. Further studies are needed to elucidate the potential role of bacterial populations and/or interactions in diseases such as obesity. In this context, the role of the different H2-producing and non-H2-producing cellulose-degrading species and their interactions with hydrogenotrophic microorganisms on gut health should be explored.
In conclusion, the composition and activity of the cellulose-degrading population were shown to vary considerably according to the presence or absence of methanogens in the human gut, further suggesting that microbial factors might influence the CH4 status and affect host energy balance in humans.
Acknowledgements
C.C. and C.R. were supported by a fellowship from the French Ministère de la Recherche et de l'Enseignement Supérieur. The authors thank Dr Marcus Yaffee and Dr Amanda Payne for critically reading the manuscript.
References
Author notes
Editor: Julian Marchesi