-
PDF
- Split View
-
Views
-
Cite
Cite
Jill P J M Hikspoors, Yolanda Macías, Justin T Tretter, Robert H Anderson, Wouter H Lamers, Timothy J Mohun, Damián Sánchez-Quintana, Jerónimo Farré, Eduardo Back Sternick, Miniseries 1—Part I: the Development of the atrioventricular conduction axis, EP Europace, Volume 24, Issue 3, March 2022, Pages 432–442, https://doi.org/10.1093/europace/euab287
- Share Icon Share
Abstract
Despite years of research, many details of the formation of the atrioventricular conduction axis remain uncertain. In this study, we aimed to clarify the situation. We studied three-dimensional reconstructions of serial histological sections and episcopic datasets of human embryos, supplementing these findings with assessment of material housed at the Human Developmental Biological Resource. We also examined serially sectioned human foetal hearts between 10 and 30 weeks of gestation. The conduction axis originates from the primary interventricular ring, which is initially at right angles to the plane of the atrioventricular canal, with which it co-localizes in the lesser curvature of the heart loop. With rightward expansion of the atrioventricular canal, the primary ring bends rightward, encircling the newly forming right atrioventricular junction. Subsequent to remodelling of the outflow tract, part of the primary ring remains localized on the crest of the muscular ventricular septum. By 7 weeks, its atrioventricular part has extended perpendicular to the septal parts. The atrioventricular node is formed at the inferior transition between the ventricular and atrial parts, with the transition itself marking the site of the penetrating atrioventricular bundle. Only subsequent to muscularization of the true second atrial septum does it become possible to recognize the definitive node. The conversion of the developmental arrangement into the definitive situation as seen postnatally requires additional remodelling in the first month of foetal development, concomitant with formation of the inferior pyramidal space and the infero-septal recess of the subaortic outflow tract.
The origins of all the developmental components of the atrioventricular conduction axis remain to be clarified.
The basis of the axis is the ring of specialized cardiomyocytes that encircles the primary interventricular foramen.
The atrioventricular node is not completed until after muscularisation of the true second atrial septum.
The atrioventricular node and bundle do not achieve their definitive positions until after formation of the inferior pyramidal space and the infero-septal recess of the subaortic outflow tract.
Introduction
Much has been written regarding the development of the conducting tissues of the heart. Despite these works, there remains much that remains to be clarified with regard to the subtleties of formation of the atrioventricular conduction axis. For example, arguments have raged regarding the role of the various rings between the components of the developing heart in forming the axis,1–3 yet little has been written regarding the manner of formation of the connections between the atrioventricular node and the atrial septum. It is these connections, surely, which provide the so-called ‘fast pathway’ into the node. In similar fashion, little attention has been paid to the manner in which the axis becomes sequestrated from the atrial myocardium so as to become the penetrating atrioventricular bundle. It was this feature that was nominated by Tawara as the distinguishing feature between the node and the bundle in his seminal monograph.4 Perhaps of equal importance in the current era, when attention is directed to the vulnerability of the axis during transcatheter replacement of the aortic valve,5 little is known as to how the aortic root becomes committed to the left ventricle. It is this process that likely regulates the relationship between the conduction axis and the leaflets of the aortic valve. We have previously had the opportunity to explore the role of remodelling of the so-called primary ring during the expansion of the atrioventricular canal, with this process producing the inlet of the morphologically right ventricle.2 We are now able to supplement this information by access to reconstructions of human embryos over the key period of embryonic development, with these reconstructions now being available in the form of interactive three-dimensional pd files.6 We also have access to a series of human embryos prepared using the technique of episcopic microscopy.7 The information available from these datasets is then further enhanced by our access to the material housed in the human developmental biological resource.8 All of this information has then been expanded by our access to serially sectioned human foetal hearts covering the period of development from 10 to 30 weeks of gestation. Based on all these materials, we are now able to provide an account of development of the human atrioventricular conduction axis, providing new information on all the topics emphasized above.
Methods
Our knowledge of the major temporal changes occurring during the remodelling of the atrioventricular canal and the embryonic interventricular communication are based on analysis of the interactive pd files made from the series of human embryos from Carnegie stage 9 through Carnegie stage 23.6 The location of the so-called primary interventricular ring was incorporated into these reconstructions as based on our earlier work in which the cardiomyocytes making up the ring were identified according to their reaction with an antibody raised against the nodose ganglion of the chick.2 This material remained available for re-examination for the purposes of this study. Episcopic datasets7 were available for human hearts between Carnegie stages 12 and 20, with 18 datasets being available for interrogation. The episcopic data were further enhanced by examination of the serial histological sections available from human hearts as archived in the human developmental biology resource.8 For this purpose, we had access to 47 datasets from Carnegie stage 11 through Carnegie stage 23. The serially sectioned foetal hearts covered the period from 11 weeks of gestation to 32 weeks. In all, 11 datasets were available for examination.
Results
The heart first becomes recognizable as a straight tube, with venous and arterial connections, at ∼26 days after fertilization. The temporal morphological changes taking place during embryonic development, which last for the first 8 weeks after fertilization, are described on the basis of the stages identified by those who studied the serially sectioned human embryos housed in the archive of the Carnegie institution. The stage corresponding to 26 days of development is #9, with Stage #23 identified at the end of embryonic development.6 In the days that immediately follow Stage 9, the heart begins to beat. By Carnegie stage 12, it is possible to recognize the atrial component of the heart, which communicates through the atrioventricular canal with the ventricular loop. The inlet component of the loop then provides the basis for formation of the left ventricle, with the apical component of the right ventricle developing from the outlet component of the ventricular loop. It is the stages that follow on from Stage 12 as identified in the Carnegie system that are key for appreciation of the formation of the atrioventricular conduction axis. It is during these stages that it also becomes possible to recognize the appearance of the apical ventricular septum, concomitant with the formation of the apical components of the ventricles (Figure 1). Carnegie stages #13 and #14 encompass the period of 32 through 34 days subsequent to fertilization. During this period, furthermore, conduction velocity is known to increase within the developing ventricular walls, with ventricular activation changing from a base-to-apex to an apex-to-base direction. The ring of cardiomyocytes identified by their reaction to the nodose ganglion of the chick is recognizable at Carnegie stage 13 (Figure 1A). This is the primary ring (Figure 1B).
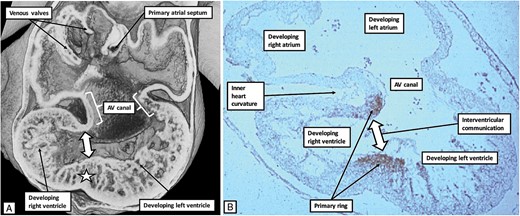
The images show, to the left hand, an episcopic dataset (A), and to the right hand a histological section prepared to react to an antibody derived from the nodose ganglion of the chick (B). The heart used to prepare the episcopic dataset was graded as representing Carnegie stage 14, whereas the heart from which the section was made was graded at Carnegie stage 13. They are showing the same basic stage of development, which represents ∼34 days subsequent to fertilization. The antibody to the nodose ganglion has marked the cardiomyocytes of the primary ring, which encircle the embryonic interventricular communication. At the same time, the cardiomyocytes occupy the rightward margin of the atrioventricular canal (see Figure 3). At this early stage of development, the atrioventricular canal, along with the atrioventricular cushions, is supported exclusively above the cavity of the developing left ventricle.
Comparison with the episcopic datasets then permits recognition of the extent of the atrioventricular canal myocardium (Figure 1). Whilst the primary ring encircles the embryonic interventricular communication, its dorsal component also occupies the rightward margin of the atrioventricular canal myocardium. At this initial stage, the circumference of the atrioventricular canal is supported by the myocardium of the primary heart tube that has ballooned out to form the apical component of the developing right ventricle. This means that the blood entering the right atrium from the venous tributaries must pass through the cavity of the developing left atrium, thence through the atrioventricular canal, and finally through the primary ring before it can enter the developing outflow tract, which is supported exclusively by the cavity of the developing right ventricle. Within the next few days, the atrioventricular canal has expanded rightwards so as to produce a direct connection between the cavities of the right atrium and the right ventricle, thus providing the right ventricle with its inlet component (Figure 2).
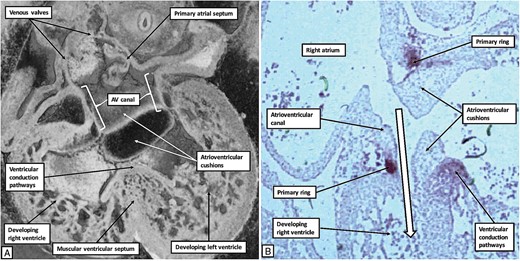
The images show the changes that take place during cardiac development in the human heart as the developing right ventricle achieves its inlet component. The left hand panel (A) is a ‘four chamber’ section through an episcopic dataset of an embryo graded at Carnegie stage 16. The right hand panel (B) is a histological section incubated with the antibody to the nodose ganglion of the chick from another human embryo also graded at Carnegie stage 16. Expansion of the atrioventricular canal has provided the right ventricle with its inlet component, as shown by the white arrow with black borders in (B). As shown in (B), the primary ring now encircles the right atrioventricular junction, occupying the parietal margin of the right atrioventricular canal. The part of the ring on the crest of the newly formed muscular ventricular septum will form the basis of the ventricular conduction pathways.
Of necessity, the expansion of the atrioventricular canal will also remodel the location of the primary ring. Subsequent to expansion, the caudal component of the ring retains its location on the crest of the developing muscular ventricular septum. The dorsal margin of the ring, however, has expanded concomitant with the expansion of the atrioventricular canal. It now encircles the parietal part of the newly formed right atrioventricular junction. It continues, nonetheless, to occupy the caudal margin of the right side of the atrioventricular canal myocardium (Figures 2B and 3).
The interventricular foramen itself has also remodelled concomitant with these changes. It remains bounded caudally by the crest of the muscular ventricular septum, with the caudal component of the ring marking the septal crest (Figures 2B and 3). The cranial margin is now the undersurface of the atrioventricular cushions. These structures have by this stage separated the canal itself into the right and left atrioventricular orifices. The ring itself has bent such that its components are perpendicular to each other. Part surrounds the persisting interventricular component of the original foramen, while the other part encircles the right atrioventricular junction. Eventually, the cranial component will become the outflow tract for the left ventricle (Figure 4, dotted arrow). Note that even after the right ventricle has achieved its own inlet, the entirety of the outflow tract remains supported above this ventricle. It is, therefore, the cranial part of the interventricular foramen that represents the outlet for the developing left ventricle (Figures 3 and 4).
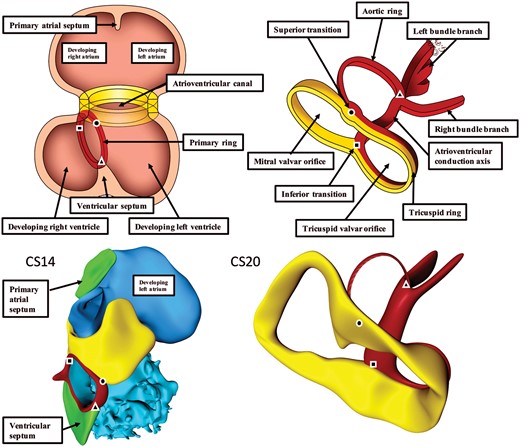
The influence of expansion of the atrioventricular canal (yellow) on the disposition of the primary ring (red). Square—inferior transition; circle—superior transition; and triangle—transition to dead-end tract. At Carnegie stages 13 and 14, the plane of the orifice of the primary ring is itself at right angles to the plane of the atrioventricular junction (left column). With rightward expansion of the atrioventricular canal, the primary ring itself bends at a right angle, with the dorsal part then encircling the newly formed right atrioventricular junction as part of the right atrioventricular canal. The right column has been reorientated to show the view of the atrioventricular junctions as seen from the right side with the ventricular apex pointing to the left. The dorsal part retains its position on the muscular ventricular septum, while the cranial part expands leftward to encircle the outflow tract of the left ventricle (aortic ring).
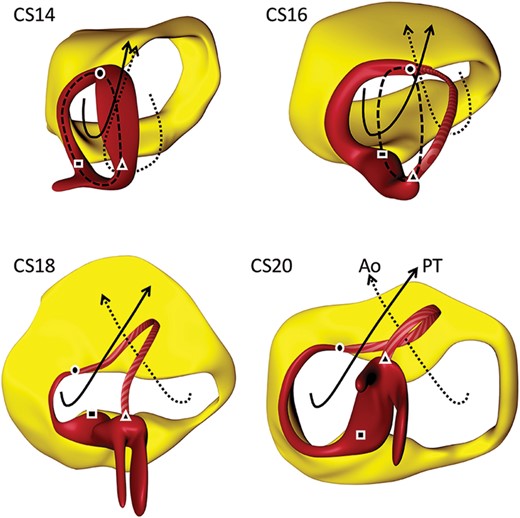
Three-dimensional views on the remodelling of the interventricular foramen. The coding colours are as shown in Figure 3. The dashed black line shows the orientation if the primary ring, itself shown in red, prior to remodelling. It can be divided into three parts. That part between the square and triangle is located on the crest of the developing muscular ventricular septum. The part between the circle and square is incorporated into the right atrioventricular junction, and will expend concomitant with the expansion of the atrioventricular canal. The part between the triangle and circle, which can be considered to represent the aortic ring, will expand leftward along with the formation of the left ventricular outflow tract. Rightward remodelling takes place in the dorsal part of primary ring, while the leftward remodelling takes place in the cranial part. The square identifies the site of the inferior atrioventricular transition. The ring in this area will eventually provide the basis for the formation of the atrioventricular node. The circle marks the superior atrioventricular transition. This part persists as the retro-aortic node, although in some malformations it can persist as a connecting anomalous atrioventricular node. The solid and dotted lines show the changes occurring with the systemic and pulmonary circulations that occur subsequent to the remodelling of the primary foramen. Ao, aorta; CS, Carnegie stage; PT, pulmonary trunk.
At CS14, the atrial septum has yet to form. The communications between the primary ring and the developing atrial myocardium, therefore, are found at the superior and inferior transitions of the two components of the initial ring (Figure 3). Already at this stage, nonetheless, the caudal component of the ring has established continuity with cardiomyocytes formed within the apical trabeculations of both developing ventricles. These changes make it possible to recognize the primordiums of the developing ventricular conduction pathways draped across the crest of the newly formed muscular ventricular septum (Figures 3 and 4). At the inferior transition, furthermore, the cardiomyocytes within the primary ring continue rightward as an encircling component that surrounds the developing tricuspid valvar orifice.9 With subsequent development of the plane of atrioventricular insulation, the atrioventricular canal myocardium will be sequestrated within the terminal parts of the atrial vestibules.9 After the establishment of atrioventricular insulation, the persisting atrioventricular myocardial transitions will be found inferiorly and superiorly relative to the persisting interventricular communication. It is only the inferior transition that persists, and this is found initially at the crux of the developing ventricular mass. This arrangement is seen at Carnegie stage 17, when the embryo has developed through ∼40 days subsequent to fertilization (Figure 5).
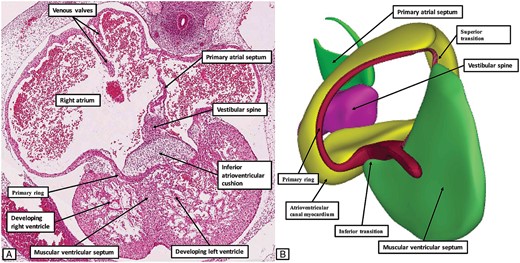
The images show the arrangement of the primary ring at Carnegie stage 17. The left hand panel (A) is a histological section. It shows the inferior transition from the ventricular to the atrial components of the ring. The right hand panel (B), a reconstruction from an embryo at the same stage of development, shows the view of the developing atrioventricular canal from the right ventricular aspect, having removed the atrial and ventricular myocardial walls and the atrioventricular cushions. At this stage, the developing ring is in communication only with the dorsal walls of the developing atrial chambers. The developing base of the atrial septum at this stage is formed by the mesenchymal tissues of the vestibular spine, along with a mesenchymal cap carried on the leading edge of the primary atrial septum.
At this stage of development, therefore, the cardiomyocytes in the inferior atrioventricular transition of the primary ring are in communication with the developing vestibules of both the right and left atrial chambers. The cranial component of the primary ring, however, is present only within the right-sided vestibule. The atrial septum, furthermore, has yet to form. It is at this stage that the primary atrial foramen is being closed by the apposition of the mesenchymal cap carried on the leading edge of the primary septum with the atrial surfaces of the atrioventricular cushions, which by now have themselves fused. The process of closure is reinforced by the growth of the vestibular spine through the rightward rim of the dorsal mesocardium.10 By Carnegie stage 18, therefore, the primary atrial foramen is closed. It is at this stage that it becomes possible to recognize the beginning of the insulation between the atrial and ventricular myocardial walls. This requires fusion of the external tissues of the atrioventricular grooves with the atrioventricular cushions formed within the lumen of the atrioventricular canal. It is the fusion of the two mesenchymal components that sequestrates the atrioventricular canal myocardium, including the encircling component of the primary ring on the right side, as the vestibular components of the right and left atrioventricular junctions.9 Even at this stage, however, there is no direct communication between the cardiomyocytes of the primary ring and the developing atrial septum (Figure 6).

The images show the completion of atrial septation, but still with the base of the septum remaining mesenchymal. Panels (A) and (B) are serial histological sections showing the transition of the primary ring from the atrial to the ventricular myocardial compartments. The white arrows with black borders show how the insulating tissues of the atrioventricular grooves are producing separation between the atrial and ventricular muscle masses. Panel (C) shows a reconstruction of a separate heart at this stage of development, revealing how both arterial roots remain supported by the developing right ventricle.
Although having no septal connections, the primary ring is in communication with the inferior atrial walls as it emerges from beneath the inferior atrioventricular cushion (Figure 6A and B). Since the base of the atrial septum remains mesenchymal, there is still no direct myocardial continuity with the component of the primary ring carried on the crest of the muscular ventricular septum. This connection does not take place until the vestibular spine, along with the mesenchymal cap carried on the leading edge of the primary atrial septum, have muscularized to form the true second atrial septum.10 It is often stated that the ‘septum secundum’ is located cranially. This is a mistake. The superior rim of the oval fossa is no more than an infolding between the walls of the right and left atrial chambers. It is the muscularization of the developing true second atrial septum, which forms the buttress that binds the septum to the atrial aspect of the atrioventricular cushions, that is the key to formation of the definitive atrioventricular node. Once the connection has been established between the atrial septal myocardium and the primary ring, the initial inferior transition from the ventricular to the atrial components of the ring can then be recognized as the site of penetration of the conduction axis. The insulation from the atrial septum is formed at the point where the axis emerges between the diverging inferior limbs of the inferior atrioventricular cushion. These changes take place during Carnegie stages 22 and 23, which is ∼56 days after fertilization (Figure 7).
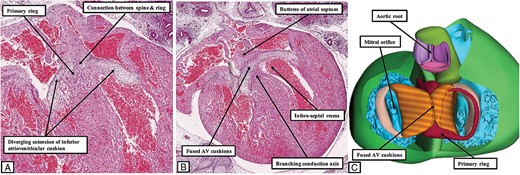
The images show the arrangement of the atrioventricular conduction axis at the end of the embryonic period, ∼8 weeks after fertilization. The left hand panels (A and B) are serial histological sections from a human embryo at Carnegie stage 22. They show how that primary ring emerges between the diverging inferior margins of the fused atrioventricular cushions to make contact with the cardiomyocytes of the newly muscularized inferior buttress of the atrial septum. Panel (C) is a reconstruction of a different human embryo at Carnegie stage 23. The base of the ventricular mass is shown as viewed from the atrial aspect having removed the atrial myocardium. The atrial component of the primary ring can be seen emerging from beneath the atrioventricular cushions, and encircling the orifice of the tricuspid valve. Note that the aortic root, although now in direct communication with the cavity of the left ventricle, remains positioned above the cavity of the right ventricle. Its developing leaflets are separated at this stage from the developing leaflets of the mitral valve by the atrioventricular canal myocardium, which will eventually be converted into the area of aortic-to-mitral fibrous continuity.
By this stage, the embryonic interventricular communication seen at Carnegie stage 16, when both developing arterial roots, were supported by the right ventricle, has itself remodelled to become the definitive outflow tract of the left ventricle. This process is achieved by fusion of the proximal outflow cushions, creating a shelf in the roof of the right ventricle to tunnel the aortic root via the interventricular communication to the cavity of the left ventricle. Once the shelf is built, tubercles derived from the rightward margins of the fused atrioventricular cushions are able to close the persisting communication between the base of the aortic root and the cavity of the right ventricle. It is these tubercles that subsequently remodel to become the membranous part of the ventricular septum. At the stage at which the channel between the aortic root and the right ventricle is closed, with this process placing the aortic root in direct communication with the cavity of the left ventricle, the root remains supported above the cavity of the right ventricle (Figure 7C).
The primary ring by this stage, as it emerges from beneath the diverging inferior horns of the fused atrioventricular cushions, is in direct communication with the newly formed myocardium of the buttress of the atrial septum. Even at this stage of development, however, when the connection between the cardiomyocytes of the primary ring and those of the atrial septum has made it possible to recognize an atrioventricular node with both septal and vestibular connections, there has been minimal expansion of the atrioventricular orifices. These changes will not take place until the foetal stages of development. By the end of 8 weeks of embryonic development, nonetheless, it is possible to recognize the ventricular components of the axis, derived from the primary ring, on the crest of the muscular ventricular septum. The transition from the ventricular components of the axis to the newly formed atrioventricular node is found at the point where the ring emerges from beneath the inferior extent of the fused atrioventricular cushions. At the end of the embryonic period, this transition remains close to the inferior margin of the base of the ventricular mass (Figure 7C). The atrioventricular node, which now includes part of the atrial component of the primary ring, has connections through the ring with the tricuspid vestibular myocardium, and through the atrioventricular canal myocardium with the mitral vestibule. The node by now has also achieved its direct atrial septal connections thanks to the myocardialization of the vestibular spine and the mesenchymal cap (Figure 7A and B).10 Conversion of this basic arrangement into the definitive situation as seen in the postnatal heart requires further expansion of the inferior margins of both atrioventricular junctions. It is this process that produces the inferior pyramidal space.11 It is also necessary for the aortic root to be wedged between the superior margin of the left atrioventricular junction and the leftward surface of the ventricular septum. This requires conversion of the initial myocardial inner heart curvature into the fibrous continuity found postnatally between the leaflets of the aortic and mitral valves. Both these changes take place rapidly within the 2–3 weeks that follow the end of the embryonic period of development. Thus, by 11–12 weeks of gestation it is possible to recognize that atrioventricular node formed at the apex of the inferior pyramidal space, with the cardiomyocytes of the node continuous inferior with the vestibules of both the tricuspid and mitral valves (Figure 8).

The sections are from a human foetus stillborn at 11–12 weeks of gestation. In this figure, and also in Figure 9, which shows further sections from this foetus, the sections are shown in ‘four chamber’ orientation, with the atrial chambers on top of their respective ventricles. When traced from inferior (A) to superior (E), the sections show how the vestibules provide the inferior extensions from the atrioventricular (AV) node (A and B). The compact node thus formed then receives connections from the base of the atrial septum (C and D) before becoming the penetrating atrioventricular bundle as it is insulated from the atrial septal myocardium by the fibrous continuity between the leaflets of the mitral and tricuspid valves (E).
As the components derived from the vestibules of the atrial chambers come together to form the compact node, it is carried on a fibrous plate formed at the apex of the inferior pyramidal space (Figure 8B). Due to the muscularization of the buttress of the atrial septum, connections are now recognizable between the atrial septal cardiomyocytes and the cardiomyocytes of the compact node (Figure 8C). The axis then becomes the bundle of His as it passes beneath an insulating tongue formed between the hinges of the mitral and tricuspid valves (Figure 8D and E). At this point, the axis is now found within the fibrous tissues forming the right wall of the infero-septal recess of the left ventricle,12 the latter space having been formed concomitant with the eventual wedging of the aortic root between the leaflets of the mitral valve and the septal surface of the left ventricle (Figure 8E). When traced further ventrally, the axis is then seen to branch on the crest of the muscular part of the ventricular septum, wedged between the muscular and membranous septal components (Figure 9).

The images show the continuation of the conduction axis in the heart of the stillborn human foetus shown in Figure 8. Panel (A) shows how, having penetrated, the axis branches on the crest of the muscular ventricular septum, first giving off the fascicles of the left bundle branch. It then gives rise to the right bundle branch (B), with the axis then being within the circumference of the aortic root (C). Note that, at this stage of development, it is still possible to recognize the remnants of outflow tract myocardium within which the arterial roots were formed during embryonic development. It is also possible to recognize the retro-aortic node, which remains at the site of the superior transition of the primary ring.
The manner of formation of the node, and the insulation of the penetrating atrioventricular bundle, are further endorsed by analysis of a foetal heart obtained from a stillbirth after 21 weeks of gestation (Figure 10).
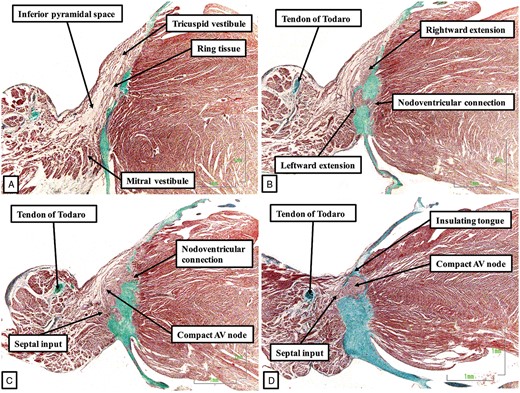
The images, shown in attitudinally appropriate fashion, with the atrial chambers seen to the left of their corresponding ventricles, are from a dataset prepared from a 21 week human foetus. They confirm that the compact atrioventricular node is formed by conjunction of extensions from the tricuspid and mitral vestibules. The axis is insulated as it passes beneath the fibrous continuity between the leaflets of the mitral and tricuspid valves. Note that, in this foetal heart, connections persist between the developing node and the crest of the muscular ventricular septum.
At this stage of foetal development, however, the fibrous insulation between the conduction axis and the crest of the muscular ventricular septum is incomplete, so that extensive nodoventricular connections are present (Figure 10B–D). In the heart shown in Figure 10, the connections between the conduction axis and the crest of the ventricular septum are confined to the area of the developing atrioventricular node. In another stillborn foetus, however, which survived until 29 weeks of gestation, extensive connections were also present between the developing ventricular components of the axis (Figure 11).
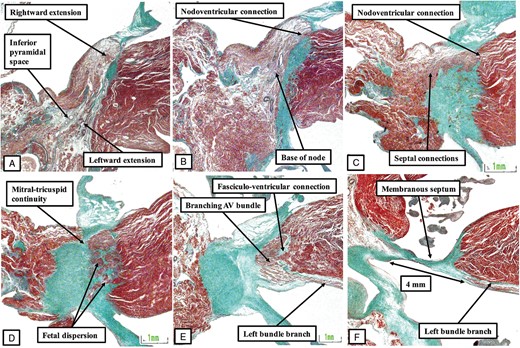
The images show serial histologic sections (panels A through F), again shown in attitudinally appropriate fashion, from a stillborn foetus of 29 weeks of gestation. The make-up of the node is as shown in Figures 8 and 10. In this foetus, however, not only are then extensive nodoventricular connections, as shown in Figure 10, but also fasciculo-ventricular connections (E). At the transition from the node to the penetrating bundle, it is also possible to recognize the feature known as foetal dispersion (D).
Analysis of the foetal hearts shows significant variation in the precise relationship of the conduction axis to the virtual basal ring of the aortic root (Figure 11F). In some of the hearts, the left bundle branch, when traced towards the right coronary leaflet, is within 1 mm of the nadir of the leaflet. In other foetuses, however, in which there is more extensive outflow tract myocardium supporting the leaflet, there is a much larger distance between the left bundle branch and the level of the virtual basal ring of the aortic root. The image shown in Figure 12 is from another foetus stillborn at 22 weeks of gestation.
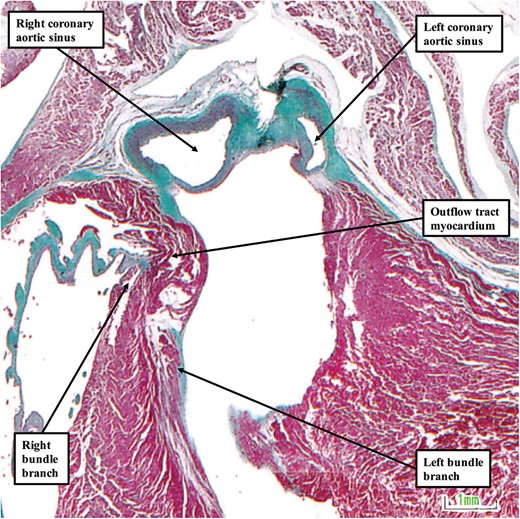
The image shows the relationship between the left bundle branch and the aortic sinuses of the aortic root in a foetus stillborn at 22 weeks of gestation. The image is shown in ‘four chamber’ orientation, with the atrial chambers on top of their respective ventricles. In this heart, there is an extensive collar of outflow tract myocardium that lifts the aortic root away from the base of the heart.
Discussion
So as to provide information regarding the development of the atrioventricular conduction axis, we have been able to examine large numbers of serially sectioned human embryos covering the key periods of 5–8 weeks of embryonic development. These sections are available for general scrutiny in the website of the Human Developmental Biology Resource.8 We have been able to supplement our examination of the histological sections by interrogating three-dimensional reconstructions prepared from the original Carnegie series of embryos,6 and also additional embryos prepared using the technique of episcopic microscopy.7 The findings from the embryonic period were then extended by our access to further series of serial histological sections prepared from stillborn human foetuses covering the period from the end of embryonic development to 30 weeks of gestation. Taken together, our findings show that the conduction axis does not approach its definitive postnatal form until the buttress of the atrial septum is muscularized towards the end of the embryonic period of development.10 Prior to this stage, it is possible to recognize the primordium of the conduction axis, which is derived from the ring of cardiomyocytes that encircles the embryonic interventricular communication at the stage of initial appearance of the muscular ventricular septum.2 And even at the stage at which the atrioventricular junction is supported above the developing left ventricle, it is known that that the heart is able to generate an ‘adult type’ electrocardiogram. It is likely, therefore, that both the cardiomyocytes making up the primary ring, and those which constitute the myocardium of the atrioventricular canal, are incorporated into the definitive atrioventricular node. The node as recognized in the postnatal heart, however, is not complete until it also receives direct input from the cardiomyocytes of the atrial septum. In this regard, therefore, it is inappropriate to describe a definitive atrioventricular node until after the buttress of the atrial septum has been formed by muscularization of the vestibular spine and the mesenchymal cap carried on the leading edge of the ventricular septum.3 An analogue of the node, of course, does exist in the absence of these contributions, as seen for example in the setting of atrioventricular septal defect with common atrioventricular junction.13 It is unlikely to be coincidental, therefore, that the known arrangement of the conduction axis in this congenital cardiac malformation is directly comparable to the situation found in the developing human heart prior to the establishment of the atrial septal connections.
Even after the contributions provided to the nodal primordiums by muscularization of the vestibular spine and mesenchymal cap, the anatomical arrangement remains markedly different from that found in the postnatal heart. This is because, at the end of the embryonic period of development, there has been scant formation of the inferior pyramidal space.11 And the aortic root, even though tunnelled to the cavity of the left ventricle, remains aligned with the cavity of the right ventricle. It is the subsequent remodelling that takes place during the first month of foetal development that sees the formation of both the inferior pyramidal space and the infero-septal recess of the left ventricle.12 It is also during these stages of foetal development that the insulating tissues develop so as to separate the atrioventricular node from the penetrating atrioventricular bundle. It is passage of the axis beneath the tongue of tissue derived from the inferior atrioventricular cushion, which produces fibrous continuity between the leaflets of the tricuspid and mitral valves, which marks the boundary between the node and the bundle. This boundary is to be found from the outset of development. It represents the inferior transition of the primary ring from the ventricular to what will become the atrial component of the developing heart. The findings observed during the foetal period of development are then pertinent to the pathways known to exist in the postnatal heart as arrhythmic substrates. Thus, in the early stage of foetal development, connections are present between both the atrial and ventricular components of the conduction axis and the crest of the muscular ventricular septum. It is again unlikely to be coincidental that such connections between the atrial component of the axis and the crest of the ventricular septum are frequent in the setting of Ebstein’s malformation.14 This lesion is known to represent abnormal formation of the right ventricular inlet. And, as we have now shown elsewhere in this supplement,15 fasciculo-ventricular connections as described initially by Mahaim are much more frequent in otherwise normal hearts than had previously been thought. Our findings are also potentially of significance to determining the vulnerability of the ventricular component of the conduction axis during transcatheter replacement of the aortic valve. Although we have been able to examine only a limited number of foetal hearts, we have found significant differences in the relationship of the left bundle branch to the nadir of attachment of the right coronary aortic leaflet. This difference, at first sight, seems related to the amount of outflow tract myocardium transferred to the left ventricle along with the aortic root. Other features, however, could well be significant, notably the precise mechanism of transfer of the root relative to the infero-septal recess of the left ventricle. Further work is now needed to explore further these possible surrogates for the vulnerability of the aortic root during transcatheter valvar replacement.
Acknowledgement
The human histology images were provided by the Joint MRC/Wellcome Trust (MR/R006237/1) Human Developmental Biology Resource (www.hdbr.org).8
Conflict of interest: none declared.
References
Author notes
Jill P.J.M. Hikspoors and Yolanda Macías contributed equally to the study.
- cardiac myocytes
- myocardium
- pregnancy
- atrioventricular node
- bundle of his
- atrium
- decompression sickness
- embryo
- fetal heart
- heart ventricle
- housing
- reconstructive surgical procedures
- embryology
- fetal development
- heart
- interventricular septum
- interatrial septum
- atrioventricular canal
- atrioventricular junction
- datasets