-
PDF
- Split View
-
Views
-
Cite
Cite
Yolanda Macías, Marcos C de Almeida, Justin T Tretter, Robert H Anderson, Diane E Spicer, Timothy J Mohun, Damián Sánchez-Quintana, Jerónimo Farré, Eduardo Back Sternick, Miniseries 1—Part II: the comparative anatomy of the atrioventricular conduction axis, EP Europace, Volume 24, Issue 3, March 2022, Pages 443–454, https://doi.org/10.1093/europace/euab291
- Share Icon Share
Abstract
The arrangement of the conduction axis is markedly different in various mammalian species. Knowledge of such variation may serve to question the validity of using animals as prospective models for design of systems for clinical use.
We compared the arrangement of the atrioventricular conduction axis in human, murine, canine, porcine, and bovine hearts, examining serially sectioned datasets from 20 human, 16 murine, 3 porcine, 5 canine, and 1 bovine hearts. We also analysed computed tomographic datasets obtained from bovines and one human heart. Unlike the situation in the human heart, there is no formation of an atrioventricular fibrous membranous septum in the murine, canine, porcine, nor bovine hearts. Canine, porcine, and bovine hearts also lack an infero-septal recess, when defined as a fibrous plate supporting the buttress of the atrial septum. In these species, half of the non-coronary leaflet is directly opposed to the ventricular septal surface.
There is a long right-sided non-branching component of the axis, which skirts the attachment of the non-coronary sinus of the aortic root. In the bovine heart, moreover, the left bundle branch usually extends intramyocardially as a solitary tape before surfacing and ramifying on the left ventricular septal surface. The difference in the atrioventricular conduction axis between species may influence the anatomical substrates for atrioventricular re-entry tachycardia, as well as providing inferences for assessing the risks of transcatheter implantation of the aortic valve. Further studies are now needed to assess these possibilities.
The arrangement of the atrioventricular conduction axis is markedly different in other animal species when compared to the arrangement found in the human heart.
The non-branching atrioventricular bundle is much longer in the animal species, skirting the hinges of the leaflets of the aortic valve.
The differences in the atrioventricular node may influence the substrates for atrioventricular nodal re-entry.
Introduction
It is now more than 100 years since Sunao Tawara published the results of his stellar investigation establishing the arrangement of the atrioventricular conduction axis.1 It is significant that, prior to the appearance of the monograph, as distinguished an anatomist and histologist as Arthur Keith had questioned the very existence of the conducting tract.2 This was despite the information provided in the initial publication of Wilhelm His Jr,3 which with the aid of the retrospectroscope we can now appreciate was remarkably accurate.4 The discussions regarding the significance of the pathway clarified by Tawara had been confused by the incorrect claim made by Kent that multiple conducting tracts were to be found crossing the atrioventricular junctions.5 The monograph of Tawara, however, despite its excellence, is not easy to digest. It is written in German, with the illustrations grouped together in plates hidden at the back of the book. This makes it very difficult to correlate the multiple items of interest identified and discussed by Tawara.6 These potential problems have now been overcome, to a significant extent. by the availability of an English translation, with the majority of the illustrations appropriately placed within the text.7 Careful examination of the illustrations provided by Tawara now provides new insights pertinent to some of the controversies that have persisted over the more than hundred years that have passed since the original publication. For a brief period in the middle of the 20th century, for example, a group of investigators denied the existence of the conduction axis.8 An ingenious report using ‘motion pictures’ served to validate the accuracy of the original descriptions provided by Tawara.9 Then, in the latter decades of the 20th century, Racker misinterpreted the arrangement of the conduction axis in the dog heart, proposing the presence of not one, but two atrioventricular bundles.10 As was pointed out in a counter publication,11 careful examination of the illustrations of Tawara would have served to defuse this particular controversy (Figure 1).
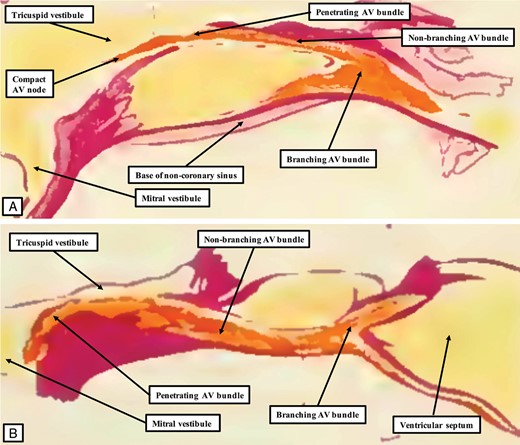
The illustrations are taken from the monograph of Sunao Tawara.1 They have been reorientated to match the majority of the illustrations to be included in our review showing the arrangement of the conduction axis in various animal species. The atrial chambers are seen to the left hand, with the right-sided cavities uppermost. The figures provided by Tawara show the markedly different arrangement of the axis in the dog heart (A) compared with man (B). Our own findings endorse the significance of the differences shown by Tawara.15
Even in the 21st century, however, investigators seem unaware of the markedly different arrangement of the conduction axis in various different mammalian species. Knowledge of these differences are now the more important. They serve to question the validity of using animals as prospective models for design of systems used for pacing the atrioventricular bundle.12 The difference in the atrial connections of the atrioventricular node between species may influence the anatomical substrates for atrioventricular nodal re-entry.13 Current interpretations in this regard depend to a large extent on inferences made from experiments carried out in the animal laboratory.14 To the best of our own knowledge, there is no recent review that has explored the significance of the disposition of the atrioventricular conduction axis in different animal species, particularly those used for animal experiments, or in molecular biological or immunohistochemical investigations. With this shortcoming in mind, and being aware of the potential clinical significance of any differences, we have compared the arrangement of the atrioventricular conduction axis in human, murine, canine, porcine, and bovine hearts. We have recently provided a more detailed account of the specific differences between the canine and human arrangements.15
Methods
For the purposes of our review, we have taken advantage of the large number of datasets related to the atrioventricular conduction axis available to us through our previous investigations, supplementing this evidence with new material prepared specifically for the purpose of this review. Thus, we have re-examined the serially sectioned datasets prepared from 20 human hearts used to establish the atrial connections of the atrioventricular node.13 We have also examined a computed tomographic dataset obtained from a living human, using this to put the location of the conduction axis into the context of the living heart. To determine the arrangement of the conduction axis in the murine heart, we re-examined multiple datasets prepared by the technique of episcopic microscopy.16 We examined 10 datasets from foetal mice sacrificed during the nineteenth day of gestation, along with 3 datasets for each of the first 3 days of postnatal life. To supplement these findings, we prepared serial histological sections in the three orthogonal planes of the intact murine heart, preparing three datasets for each of the planes. We then studied, also serial histological sections taken through the entirety of the conduction axis, three porcine and five canine hearts. In these animals, the block of tissue containing the conduction axis had been prepared in the same fashion as the human hearts used to identify the atrial connections of the atrioventricular node.13 The findings in the canine heart had formed the basis for a recent more extensive study specifically comparing the arrangements as seen in the human heart.15 We also examined a solitary bovine heart using the same technique, with these findings supplementing our knowledge of the conduction axis gained from our previous investigation using computed tomographic analysis of bovine hearts. In our earlier study, the sheaths of the ventricular components of the axis had been injected with either Indian ink or lead.17
Results
Findings in the human heart
One of the major features of the atrioventricular conduction axis in the human heart is the manner of its transition between the atrial and ventricular components, in other words the site of penetration of the axis through the plane of atrioventricular insulation.1 In the human heart, the transition is found at the site where the apex of the inferior pyramidal space overlaps the inferior extent of the infero-septal recess of the left ventricle (Figure 2). This arrangement permits the atrial inputs of the atrioventricular node, formed as inferior extensions within the vestibules of the tricuspid and mitral valves, to merge and form the compact atrioventricular node at the apex of the inferior pyramidal space (Figure 3A). The compact node, having formed from the merging inferior extensions, can be carried either on a fibrous plate formed at the apex of the inferior pyramidal space, as shown in Figure 3A, or be supported on the rightward surface of an area of fibrous continuity between the leaflets of the tricuspid and mitral valves. In the majority of the human hearts, this area provides the support for the base of the antero-inferior buttress of the atrial septum. This buttress itself is the true second atrial septum. It is the cardiomyocytes of the atrial septum that provide the septal inputs to the atrioventricular node (Figure 4A). Taken together, it is the presence of these connections from the atrial septum, together with the extensions into the atrial vestibules, that provide the human compact atrioventricular node with its characteristic features (Figure 4A).
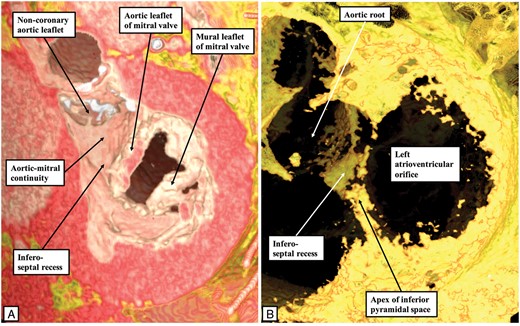
These virtual dissections made using a living dataset prepared from an individual undergoing computed tomographic investigation reveal how the apex of the inferior pyramidal space, shown in the right hand panel (B) by the adipose tissue found in the space between the atrial and ventricular walls, overlaps the inferior extent of the recess below the aortic root. The infero-septal recess of the left ventricular outflow tract undermines the base of the atrial septum. Its roof is formed by an area of fibrous continuity between the leaflets of the tricuspid and mitral valves, this area being continuous with the area of fibrous continuity between the leaflets of the aortic and mitral valves.

The images show serial sections through a histological dataset prepared from a human heart in which the atrioventricular (AV) node was formed at the apex of the inferior pyramidal space (A). The sections are orientated with the atrial chambers seen to the right side, and the right-sided cavities to the top, as for the images provided by Tawara shown in Figure 1. Figure 4A is from the same series, and shows how the node achieves its connections with the cardiomyocytes of the atrial septum. (B) The key feature of insulation of the axis by the formation of a tongue of fibrous tissue between the leaflets of the mitral and tricuspid valves. This tongue insulates the conduction pathway from the atrial septum. The fibrous tissue also forms the roof and rightward wall of the infero-septal recess. Having penetrated, the axis then has a non-branching portion of varying length before it gives rise to the fascicles of the left bundle branch, as shown in (C). The axis then is carried on the crest of the muscular ventricular septum, before giving rise to the right bundle branch at the level of the membranous septum, as shown in (D). In this particular heart, the right bundle branch extends intramyocardially before surfacing in the right ventricle beneath the site of the medial papillary muscle. The axis itself then continues as a dead-end tract.
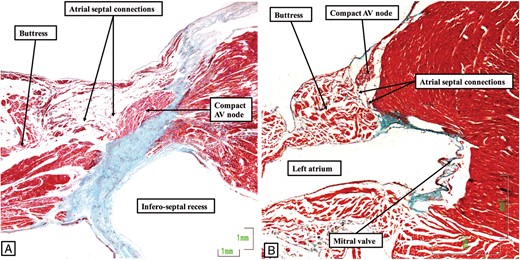
The images show how the conduction axis in both the human heart (A) and the murine heart (B) receive connections from the buttress of the atrial septum before penetrating to become the non-branching atrioventricular (AV) bundle. The image in (A) is from the same dataset as shown in Figure 3, while the image in (B) is from the dataset of the murine heart to be shown in Figure 5. In both datasets, the connections between the atrial septum and the axis are relatively tenuous. They are the final communication between the atrial walls and the axis before it becomes the penetrating component.
In the human heart, having passed through the fibrous tissue which provides the roof and right wall of the infero-septal recess (Figure 3B), the axis takes a non-branching course of variable length before giving off the fascicles of the left bundle branch (Figure 3C). The fascicles of the left bundle take their origin over a length of the branching bundle. They cascade down the left ventricular surface of the septum, branching into superior, septal, and inferior divisions. The axis then gives off the right bundle branch, which is typically cord-like. This can arise directly on the septal surface, or can run intramyocardially as shown in Figure 3D before surfacing in the right ventricle beneath the site of the medial papillary muscle. The fascicles of the left bundle, as they extend into the cavity of the left ventricle, course towards the supero-lateral and infero-medial papillary muscles. The superior fascicle has a variable relationship to the nadir of attachment of the right coronary aortic valvar leaflet. The precise distances between the nadir of this leaflet and the superior extent of the left bundle branch have still to be established. This variability could prove to be one of the key features determining the vulnerability of the conduction axis subsequent to transcatheter replacement of the aortic valve.18 The portion of the conduction axis related to the base of the atrial septum, however, is inferior to the aortic root itself. It should not, in the usual arrangement as described above, be at risk during insertion of a prosthesis within the root. As we will see, this vulnerability could vary according to the fashion in which the components of the root are supported within the outflow tract of the left ventricle.
Findings in the murine heart
The arrangement as seen in the human heart is paralleled to a large extent in the murine heart, but with subtle differences. In the murine heart, as in the human heart, there is an infero-septal recess of the left ventricle which undermines the base of the atrial septum (Figure 5). The presence of the infero-septal recess means that, as in the human heart, the conduction axis penetrates directly from the apex of the inferior pyramidal space to reach the crest of the muscular ventricular septum (Figure 6). Having penetrated, the non-branching component of the axis is positioned on the crest of the muscular ventricular septum, in the right wall of the infero-septal recess. Unlike the human heart, however, there is no formation in the mouse of an interventricular fibrous membranous septum. The axis in the murine heart, therefore, continues within the area of fibrous continuity between the leaflets of the mitral and tricuspid valves, giving off the radiating fascicles of the left bundle branch on the septal aspect of the ventricular septum. The right bundle branch is then given off from the axis in the region of the interleaflet fibrous triangle between the right coronary and non-coronary sinuses of the aortic root. At this location, the left bundle branch is directly beneath the nadir of the hinge of the right coronary leaflet of the aortic valve (Figure 6D).
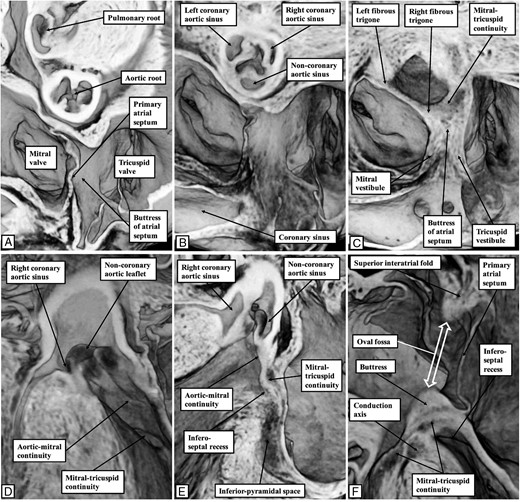
The images show the relationship between the base of the atrial septum and the infero-septal recess of the left ventricular outflow tract in the murine heart. All the images are taken from a three-dimensional dataset of a foetal mouse at embryonic cay E18.5, just prior to birth, and prepared using the technique of episcopic microscopy.15 (A) A view of the base of the heart as seen from the atrial aspect. (B) Is in the same plane, but cut closer to the atrioventricular junction, with (C) again in the same plane, but cut to show the buttress of the atrial septum. It is directly adjacent to the area of fibrous continuity between the leaflets of the mitral and tricuspid valves, which straddles the crest of the muscular ventricular septum. (D) A frontal view of the infero-septal recess of the left ventricle. It extends between the aortic leaflet of the mitral valve and the surface of the ventricular septum. (E) The ‘en face’ view of the septal surface, with the recess seen excavating beneath the area of mitral-to-tricuspid valvar continuity which provides the support for the buttress of the atrial septum. (F) Is a ‘four chamber’ cut. It shows the support provided to the buttress, and also demonstrates that the superior margin of the oval fossa, often described as the ‘septum secundum’, is an infolding of the atrial roof. The buttress is the true second atrial septum. As such, it is able to provide the input to the atrioventricular node via the atrial septal cardiomyocytes, as shown in Figure 4.
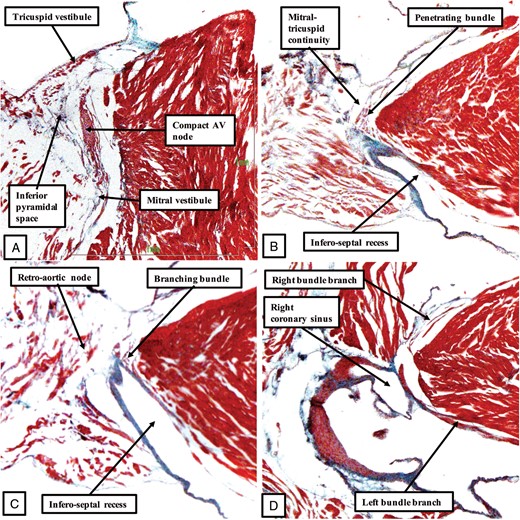
The images show the features of the conduction axis in the murine heart, prepared from a serially sectioned histological dataset, and presented in a fashion comparable to that used to produce Figure 3 for the human heart. As in the human heart, inferior extensions from the tricuspid and mitral vestibules come together at the apex of the inferior pyramidal space to form the compact atrioventricular (AV) node (A). As was shown in Figure 4B, and again as in the human heart (Figure 4A), the compact node in the murine heart receives connections from the buttress of the atrial septum before passing beneath the area of mitral-to-tricuspid valvar continuity to become the penetrating bundle (B). Replicating the situation in the human heart, this is a short non-branching component before the axis which gives off the fascicles of the left bundle branch (C). Again as in the human heart, the axis then gives off the right bundle branch on the crest of the muscular ventricular septum (D). Unlike the human heart, however, the murine heart does not have a membranous interventricular septum.
Findings in the canine, porcine, and bovine hearts
The atrioventricular conduction axis in the remaining species we have examined resembles the arrangement in the human and murine hearts in penetrating through the area of fibrous continuity between the leaflets of the mitral and tricuspid valves, but in all other aspects shows significant differences. The differences relate to the arrangement of hinging of the non-coronary leaflet of the aortic root within the outflow tract of the left ventricle, with our preliminary findings suggesting that this feature might also be found as a variation in some human hearts (Figure 7). We interpret this attachment of half of the non-coronary leaflet of the aortic valve directly to the septal structures, along with the rightward end of fibrous continuity between the leaflets of the aortic and mitral valves, as being related to the markedly different arrangement of the conduction axis observed in the canine heart, and illustrated by Tawara (Figure 1A). Our own more recent findings15 have endorsed the features shown by Tawara,1 which in turn had been endorsed by the previous description by Ho et al.11 The atrioventricular node is formed from the inferior extension carried within the vestibular myocardium of the tricuspid valve. It has direct connections from the right side of the atrial septum, but no obvious input from the mitral vestibular myocardium, such as are observed in the human and murine hearts (Figure 8A). At the level of the attachment of the aortic leaflet of the mitral valve to the left side of the septal surfaces, the axis separates itself from the atrial cardiomyocytes, and passes through the area of fibrous continuity between the leaflets of the mitral and tricuspid valves (Figure 8B). As we have recently emphasized,15 due to the absence of an infero-septal recess supporting the base of the atrial septum in the canine heart, the axis is carried as a long non-branching component on the right side of the ventricular septum, with the bundle skirting the attachment of the non-coronary sinus of the aortic root to the septal structures, as shown in Figure 7A. The bundle gives off the fascicles of the left bundle in the region of the interleaflet triangle between the non-coronary and right coronary sinuses of the aortic root (Figure 8C), with the right bundle branch also taking origin from the axis in this region (Figure 8D). In the region of the interleaflet triangle, the fascicles of the left bundle branch are directly beneath the hinges of the leaflets of the aortic valve (Figure 8C and D).
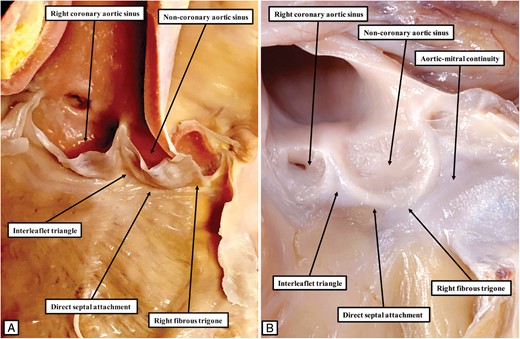
The image to the left hand (A) shows the typical arrangement of the aortic root in the canine heart. The ascending part of the hinge of the non-coronary leaflet, bordering the interleaflet triangle between the non-coronary and right coronary aortic valvar sinuses, is attached directly to the septal surface. In consequence of this feature, the rightward area of fibrous continuity between the leaflets of the aortic and mitral valves, known as the right fibrous trigone, is also attached directly to the septal surface. This is the exception rather than the rule in the human heart. The right fibrous trigone in the human heart is usually formed within the leftward margin of the infero-septal recess (Figure 2A). In some human hearts, however, the arrangement can parallel the situation as found in the canine heart, as shown in (B). Because of the direct attachment of the trigone to the septal surface, there is no formation of an infero-septal recess. The significance of this finding with regard to the arrangement of the conduction axis has yet to be established.
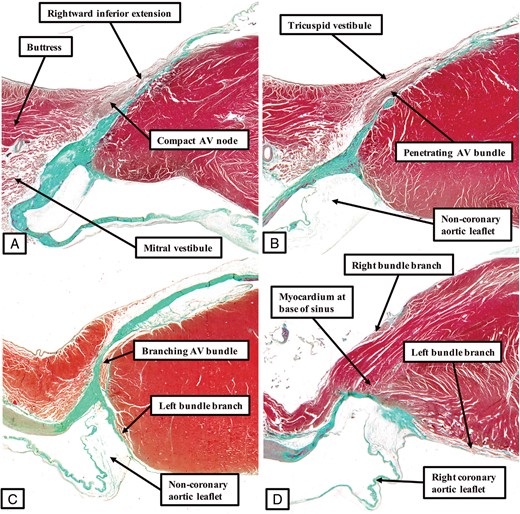
The panels, prepared in comparable fashion to the figures showing the arrangement of the axis in the human (Figure 3) and murine (Figure 5) hearts, show the features of the atrioventricular conduction axis as found in the canine heart. The compact atrioventricular node is formed from the rightward inferior extension at the site of attachment of the right fibrous trigone to the septal structure (A). The axis then becomes isolated from the atrial myocardium (B). As shown by Tawara, it then extends as a long non-branching bundle along the right side of the crest of the muscular ventricular septum (Figure 1A). It gives off the fascicles of the left bundle branch directly beneath the hinge of the non-coronary leaflet of the aortic valve (C) before branching to give of the right bundle branch, with the superior fascicle of the left bundle positioned directly beneath the hinge of the right coronary aortic valvar leaflet (D).
The arrangement as seen in the canine heart is then paralleled in both the porcine and bovine hearts, albeit with differences in the bovine heart relative to the origin of the fascicles of the left bundle branch. In both species, the non-coronary leaflet of the aortic valve is hinged directly from the left side of the septal structures (Figures 9 and 10). In both species, furthermore, the atrioventricular node is formed on the basis of the extension found in the tricuspid vestibule, with minimal contributions from the mitral vestibule (Figure 11). As was the case in the canine heart,1,15 the axis takes an extensive non-branching course on the right side of the ventricular septum, skirting the area of attachment of the non-coronary sinus on the left side of the septum (Figure 1A). In the porcine heart, the axis then branches on the crest of the muscular septum, albeit in the absence of any formation of a membranous septum. The fascicles of the left bundle branch are directly beneath the hinges of the leaflets of the aortic valve as they cascade down the left ventricular septal surface (Figure 11D). This feature is different from the arrangement found in the bovine heart, where the usual arrangement is for the left bundle branch to extend intramyocardially as a solitary tape, before surfacing and ramifying on the left ventricular septal surface (Figure 10A).19 In some instances, nonetheless, the left bundle branch in the bovine heart can also arise at the level of the crest of the muscular ventricular septum.
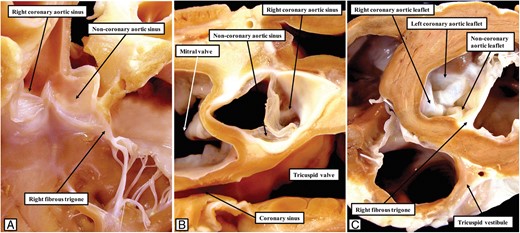
The images show the features of the left ventricular outflow tract in the porcine heart. As shown in (A), reflecting the arrangement in the canine heart (Figure 7A), the half of the non-coronary leaflet of the aortic valve adjacent to the right coronary sinus is attached, along with the right fibrous trigone, directly to the left surface of the septal structures. This is shown in (B), which is prepared by removing the non-coronary leaflet and showing the junction from the atrial aspect, and in (C), which shows the short axis of the junction from the ventricular aspect.
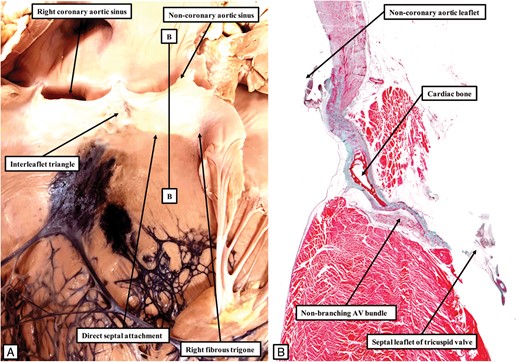
The left hand panel (A) shows the aortic root in the bovine heart, with Indian ink injected into the sheaths of the conduction axis so as to reveal the ramifications of the left bundle branch, seen in blue-black. As in the canine and porcine hearts (Figure 7A and 9A), the half of the non-coronary leaflet of the aortic valve adjacent to the right coronary aortic leaflet is attached directly to the septal surface, along with the right fibrous trigone. Note that the left bundle branch emerges from the ventricular septum at a distance from its crest. The line B–B in (A) shows the level and orientation of the histological section shown in (B). The non-branching bundle has a long course, skirting the attachment of the non-coronary leaflet to the septum, and paralleling the arrangement found in the canine heart (Figure 1A).
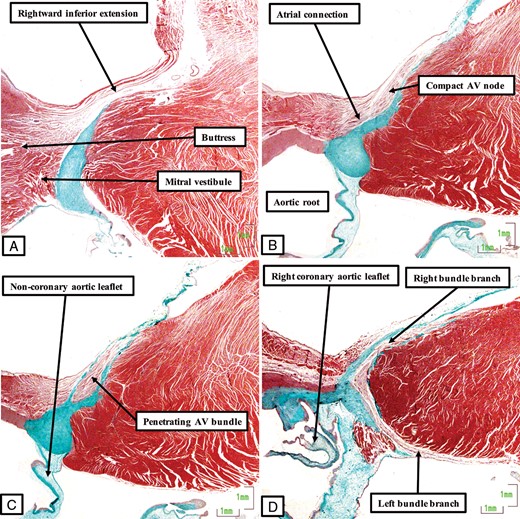
The images, prepared from a histological dataset in the same fashion as for the other species, show the arrangement of the conduction axis in the porcine heart. (A) The inferior extension of the atrioventricular node in the vestibule of the tricuspid valve. It has connections with the atrial myocardium and the level of attachment of the right fibrous trigone to the septum, but minimal input from the myocardium of the mitral vestibule (B). Having penetrated through the area of fibrous continuity between the leaflets of the mitral and tricuspid valves (C), the axis runs a long non-branching course on the right side of the ventricular septum before branching in the region of the interleaflet triangle between the non-coronary and right coronary leaflets of the aortic valve. The left bundle branch is directly adjacent to the hinge of the right coronary leaflet (D).
Discussion
To the best of our knowledge, little attention has been paid to the marked differences between species in the architecture of the atrioventricular conduction axis. This is surprising, since all of the features described in our review are readily discernable in the illustrations and descriptions provided by Tawara when he clarified the arrangement of the atrioventricular conduction axis.1 As Keith commented when acknowledging his own initial deficiencies with recognizing the atrioventricular bundle, ‘with the discovery of the conducting system of Tawara, heart research entered a new epoch’.20 The basic arrangement of the axis does have comparable features in all the species, in that the atrial myocardium comes together in the region of the base of the atrial septum to form the atrioventricular node. The node then penetrates through the plane of atrioventricular fibrous insulation to become the atrioventricular bundle, with the axis then having a non-branching component before giving rise to the right and left bundle branches. The axis can then continue as the so-called ‘dead-end tract’.21 This latter part of the axis, however, was not always recognizable in postnatal hearts of all the species we examined. Despite these similarities to be found in all the species, in particular the fashion in which the node becomes the penetrating bundle by passing beneath the area of fibrous continuity between the leaflets of the mitral and tricuspid valves, there are fundamental differences in the overall arrangement. These relate primarily to the fashion in which the non-branching component of the bundle is related to the non-coronary leaflet of the aortic valve.
In the human and murine hearts, the hinge of the non-coronary leaflet is lifted away from the crest of the muscular ventricular septum by virtue of the presence of an extensive inferior recess of the left ventricular outflow tract. The recess interposes between the aortic leaflet of the mitral valve and the left ventricular septal surface, and supports the base of the atrial septum.22 Its right wall is formed by the atrioventricular septum. Initially described by Sibson,23 the left ventricular wall of the recess was emphasized by McAlpine in his atlas of cardiac anatomy as the ‘posterior–superior process’.24 It is paradoxical that, when discussing the process, and although McAlpine had promoted the importance of attitudinally appropriate nomenclature, he reverted to describing the heart as though removed from the body and standing on its apex. When the heart is viewed as seen in the living individual, the recess can be appreciated to be infero-septal (Figure 2A). It is the presence of the infero-septal recess, with its roof supporting the base of the atrial septum, and with the atrioventricular septum forming its right wall, which permits the conduction axis to pass directly onto the crest of the muscular ventricular septum in the human and murine hearts. Even in these species, however, there is a difference between the species, since the murine heart lacks the presence of an extensive fibrous interventricular membranous septum.25 The hearts of all the other species examined also lacked the presence of such an interventricular membranous septum. And even in the normal heart, it is questionable whether the membranous septum: is as extensive as it is often described. The septum is formed from the right tubercles of the embryonic atrioventricular endocardial cushions, which close the persisting communication between the aortic root and the ventricular cavities subsequent to the transfer, during embryonic development, of the aorta to the left ventricle. The tissues provided by the atrioventricular cushions, however, are much more extensive than the part which forms the membranous septum. In the context of the aortic root, the cushions give rise to the extensive area of fibrous continuity between the leaflets of the mitral and tricuspid valves that provides the support for the base of the atrial septum. And, as discussed above, it is this fibrous tissue that forms the roof of the infero-septal recess. If we are fully to appreciate the role of the infero-septal recess in determining the course of the conduction axis, therefore, it is necessary to distinguish between the area of mitral-to-tricuspid continuity, particularly the part that insulated the axis as it passes from the atrial to the ventricular components of the heart, and the true atrioventricular component of the membranous septum. This is because the area perforated by the conduction axis is overlaid on its right atrial aspect by the atrial myocardium of the tricuspid vestibule. This area, therefore, is best described as being mitral-to-tricuspid fibrous continuity. It is the ‘naked’ area of fibrous tissue that interposes between the subaortic outflow tract and the right atrial cavity on the atrial aspect of the hinge of the septal leaflet of the tricuspid valve that is the true atrioventricular membranous septum.25 Such a septum is lacking in the murine heart, and also in the porcine, canine, and bovine hearts.
The key difference between the murine and human hearts, therefore, and the bovine, porcine, and canine hearts, is the presence or absence of an infero-septal recess. In the absence of this recess, as seen in the bovine, porcine, and canine hearts, there is a long non-branching component of the axis. This part of the axis skirts the direct attachment, in the left ventricle, of the non-coronary leaflet of the aortic valve to the septal structures. We have now observed such an attachment of the non-coronary leaflet directly to the septal structures in two hearts obtained from infants.15 In these hearts, there was separation between the area of mitral-to-tricuspid fibrous continuity and the interleaflet triangle between the right and non-coronary aortic valvar sinuses (Figure 7). As yet, we have not explored the influence that these changes may have on the disposition of the atrioventricular conduction axis. The differences that might ensue, however, may well prove to be significant in establishing the vulnerability of the conduction axis known to exist during transcatheter implantation of the aortic valve.18 The findings we have observed may also prove to be of value in determining the anatomical substrates of atrioventricular re-entry tachycardia.13 All of these ramifications will require further investigation, taking note of the major differences in species revealed by our current findings. In this respect, we are aware that the rabbit is one of the species used most frequently in previous investigations on the electrophysiological behaviour of the atrioventricular conduction axis, and that we have not discussed the findings in the rabbit heart. This is because, as yet, we are not in possession of datasets that would have permitted us to make direct comparisons with the other species examined. It is our intention to remedy this deficiency in the very near future.
Conflict of interest: none declared.
References
His Jr W.