-
PDF
- Split View
-
Views
-
Cite
Cite
Susan M. Millard, Liping Wang, Lalita Wattanachanya, Dylan O’Carroll, Aaron J. Fields, Joyce Pang, Galateia Kazakia, Jeffrey C. Lotz, Robert A. Nissenson, Role of Osteoblast Gi Signaling in Age-Related Bone Loss in Female Mice, Endocrinology, Volume 158, Issue 6, 1 June 2017, Pages 1715–1726, https://doi.org/10.1210/en.2016-1365
- Share Icon Share
Abstract
Age-related bone loss is an important risk factor for fractures in the elderly; it results from an imbalance in bone remodeling mainly due to decreased bone formation. We have previously demonstrated that endogenous G protein–coupled receptor (GPCR)-driven Gi signaling in osteoblasts (Obs) restrains bone formation in mice during growth. Here, we launched a longitudinal study to test the hypothesis that Gi signaling in Obs restrains bone formation in aging mice, thereby promoting bone loss. Our approach was to block Gi signaling in maturing Obs by the induced expression of the catalytic subunit of pertussis toxin (PTX) after the achievement of peak bone mass. In contrast to the progressive cancellous bone loss seen in aging sex-matched littermate control mice, aging female Col1(2.3)+/PTX+ mice showed an age-related increase in bone volume. Increased bone volume was associated with increased bone formation at both trabecular and endocortical surfaces as well as increased bending strength of the femoral middiaphyses. In contrast, male Col1(2.3)+/PTX+ mice were not protected from age-related bone loss. Our results indicate that Gi signaling markedly restrains bone formation at cancellous and endosteal bone surfaces in female mice during aging. Blockade of the relevant Gi-coupled GPCRs represents an approach for the development of osteoporosis therapies—at least in the long bones of aging women.
Primary osteoporosis is predominantly associated with aging and is believed to occur largely because of an age-related decrease in bone formation (1). Mature osteoblasts (Obs) are critical for producing and mineralizing the bone matrix (2, 3). Therefore, studying mechanisms that limit bone formation by Obs in the aging skeleton may lead to the discovery of important anabolic targets to increase bone mass and repair age-related fragility fractures.
G protein–coupled receptors (GPCRs) are the largest family of cell surface receptors and signal through a select number of pathways, including Gs and Gi pathways that, respectively, increase and decrease intracellular cyclic adenosine 5’-monophosphate levels. Among the GPCRs in Obs, the parathyroid hormone (PTH) receptor 1 has been extensively studied and recombinant PTH has been administered to treat age- and osteoporosis-related bone loss. However, aging also results in a defect in the stimulatory G protein coupling to adenylyl cyclase, which limits the bone’s response to PTH treatment (4). PTH receptor expression and the stimulatory effects of PTH on mesenchymal stem cells also decline with aging (5). The factors and mechanisms dampening the anabolic effect of PTH in the aging bone environment are still not clear.
Because the classical downstream effector of Gi activation is antagonism of Gs-mediated increases in adenylyl cyclase activity (6), endogenous Gi signaling may play an important role regulating bone homeostasis (7). Our recent studies manipulating Gi-GPCR signaling in mouse Obs in vivo support the notion that Gi activity within Obs suppresses bone formation in adult animals. For example, Ob-specific expression of a constitutively active Gi-coupled engineered GPCR leads to marked osteopenia, resulting from a reduction of cancellous bone formation (8). Also, loss of apelin, a ligand of a Gi-GPCR (9), leads to an increase in bone formation at both cortical and cancellous bone surfaces in young and older mice (10). Moreover, Ob-specific blockade of Gi-coupled signaling increases periosteal bone formation and cortical bone thickness in young male and female mice and increases fractional bone volume (BV) at the distal femur seen in female mice only (11). Bone formation decreases with advancing age; therefore, we hypothesized that endogenous Gi signaling in Obs may limit bone formation during aging, thereby contributing to age-related bone loss.
We have successfully pursued a strategy of Ob-specific blockade of Gi-coupled signaling by expressing the catalytic subunit of pertussis toxin (PTX) under the control of 2.3 kb-Col1 promoter (11). This catalytic subunit acts to adenosine 5’-diphosphate-ribosylate the α subunits of heterotrimeric Gi proteins, and a subset of Go proteins, thereby preventing the dissociation of the Gi/oα-subunit from the βγ subunits in response to GPCR activation (12). The PTX blockade strategy is expected to prevent the inhibition of adenylate cyclase function by Giα-subunit and effector functions driven by the βγ subunits.
In this study, we elucidated the role of this signaling pathway in regulating Ob function during aging independent of the developmental effects of Gi signaling blockade. This was achieved by suppressing expression of the PTX transgene until the mice reached adulthood and then characterizing the bone phenotype of these mice during aging after the induction of PTX expression.
Materials and Methods
Animals
All transgenic mouse studies were approved by and performed in accordance with the Institutional Animal Care and Use Committee at the Veterans Affairs Medical Center, San Francisco, and at the University of California, San Francisco.
The mice with a collagen type 1α 2.3-kb promoter fragment driving the tetracycline transactivator (tTA; “TET-Off” system) driver transgene, Col1(2.3)-tTA mice (line 139) [FVB/NJ-Tg(Col1a1-tTA)139Niss/Mmmh; Mutant Mouse Resource & Research Centers accession no. 030758-MU] and the mice with the TetO-PTX transgene, TetO-PTX mice [FVB-Tg(teto-ptxA)1Conk/Mmmh; Mutant Mouse Resource & Research Centers accession no. 014241-MU] have both been previously described. Heterozygous Col1(2.3)-tTA mice have been shown to be indistinguishable from wild-type mice (8, 11). The Col1(2.3)+/PTX+ mice and Col1(2.3)-tTA control littermates used in this study were generated by crossing mice heterozygous for the tetO-PTX transgene with mice that were true breeding for the Col1(2.3)-tTA transgene. All animals were maintained on the FVB/N background. Transgene expression was suppressed with the doxycycline-impregnated mouse chow (DoxDiet, 200 mg/kg; BioServ, Flemington, NJ). Transgene expression was activated by switching the mice to the regular mouse chow without doxycycline at the age of 8 weeks. The full transgene expression was expected to occur within 2 weeks after doxycycline withdrawal, based on prior data (8).
The average litter size for the FVB/N mouse breeding pair in this study was eight pups (range, 4 to 11). Mice were housed up to five per cage in controlled conditions with room temperature of 22°C ± 1°C and a light-dark cycle of 12:12 hours, and the mice were given water and mouse chow ad libitum.
Five litters of mice were assigned to the study for longitudinal monitoring of BV during aging, with a further three litters of mice assigned to end-point analysis only. Eight mice were excluded at the outset of the study because of an absence of sex-matched control mice within the litter. Four mice were excluded over the course of the 12-month study because of the development of malocclusions. At the experimental end point, bones were assigned to varied end-point analysis. Flushed humeri were fresh frozen in liquid nitrogen for later RNA extraction or Fourier transform infrared spectroscopy composition analysis. The fifth lumbar (L5) vertebral bones were collected for microcomputed tomography (microCT) and biomechanical testing. Femurs were either wrapped in saline-soaked gauze and fresh frozen at −20°C for later microCT and biomechanical testing, or were formalin fixed before microCT analysis and methyl-methacrylate (Sigma-Aldrich, St. Louis, MO) embedding for trabecular or cortical histomorphometry. For each mouse, two of three possible femoral analysis techniques were conducted. In some instances, tissue damage that occurred during methyl-methacrylate embedding prevented samples from being histomorphometrically analyzed.
In vivo microcomputed tomography
In vivo microCT scanning was conducted on mice anesthetized with inhaled isoflurane (1.5% to 2% in oxygen) and scanned at predetermined times (i.e., 10 weeks, and 6, 9, and 12 months) using a Scanco VivaCT 40 (Scanco Medical, Bruttisellen, Switzerland). In vivo images of the distal femur and tibiofibular junction (TFJ) were obtained at an x-ray energy of 55 kV, voxel size of 10.5 μm, and integration time of 250 ms. The region of interest (ROI) for the distal femur was defined as the cancellous bone compartment beginning 0.6 mm proximal to the most proximal point of the growth plate and extending proximally 1.0 mm. A global thresholding protocol with segmentation values (i.e., sigma/support/threshold) of 0.8/1/240 was applied to distinguish mineralized bone from bone marrow and soft tissue (11). The ROI for the TFJ was defined as the region beginning 0.02 mm proximal to the bifurcation of the TFJ and extending proximally 0.4 mm. A global thresholding protocol with segmentation values of 0.8/1/365 was used in the assessment of cortical bone (11). Nomenclature was used and calculations of microCT indices were performed according to Bouxsein et al. (13).
Ex vivo microCT
Ex vivo scanning and analysis of distal femur, femoral midshaft, and the L5 vertebral bodies were conducted using a Scanco VivaCT 40 (Scanco Medical) microCT scanner. Bones to be used subsequently in biomechanical testing were immersed in phosphate-buffered saline during scanning, without fixation or dehydration. Bones to be used subsequently in histology were fixed for 1 to 2 days in 10% neutral buffered formalin (Fisher Scientific, Pittsburgh, PA) and dehydrated in 70% ethanol before scanning. Images were obtained at an x-ray energy of 55 kV, voxel size of 10.5μm, and integration time of 1000 ms. Quantitative assessment of tissue volume (TV), BV, medullary volume, and cortical thickness of the femoral diaphyseal cortex was conducted using a 0.4-mm–thick ROI at the midfemur. A much broader region of the femoral midshaft was scanned, allowing retrospective assessment of the moment of inertia over a 0.1-mm region spanning the location where fracture occurred during biomechanical testing. The L5 vertebral body was scanned after the removal of posterior elements and end plates. The entire trabecular structure within the vertebral bodies was assessed using a manually drawn ROI excluding cortical shell. Global thresholding protocols with segmentation values of 0.8/1/365 and 0.4/1/270 were used in the assessment of ex vivo scans of the femoral midshaft and the L5 vertebral bodies, respectively.
Histomorphometry
Mice were injected with 20 mg/kg calcein (Sigma-Aldrich) 21 and 7 days before euthanasia and with 15 mg/kg demeclocycline (Sigma-Aldrich) 2 days before euthanasia, to allow for dynamic histomorphometric assessment. Isolated femurs were fixed in 10% neutral buffered formalin, dehydrated, and embedded in methyl methacrylate (Sigma-Aldrich), as previously described (14). Assessment of cancellous bone in the distal femur was performed on longitudinal sections from the left femur. Coronal sections of distal femur were cut and stained with Von Kossa/tetrachome (for assessment of trabecular structure and OB surface) or TRAP/aniline blue (for assessment of osteoclast surface) or left unstained for the visualization of fluorochrome-labeled surfaces. Assessment of cortical bone was performed on 10-µm–thick unstained cross-sections at the midpoint of the right femur. Images were captured with a Zeiss Axioplan Imager M1 microscope (Carl Zeiss MicroImaging, Jena, Germany) and analyzed using specialized image analysis software (Bioquant Image Analysis Corp., Nashville, TN), as previously described (11). The dynamic indices of bone formation that were measured include mineralizing surface to bone surface (MS/BS), mineral apposition rate (MAR), and surface-based bone formation rate (BFR) (15, 16).
Biomechanical testing
Load-to-failure tests were performed to determine whole-bone behavior at anatomic sites consisting of only cortical bone (femoral diaphysis) and corticotrabecular bone (lumbar vertebra). The right femora were tested in three-point bending. Each femur was placed on two supports (7-mm span) and a transverse force was applied to the middiaphysis under displacement control at a rate of 0.03 mm/s (17). The L5 vertebrae were tested in compression at a rate of 0.01 mm/s. Before testing, the posterior elements and end plates were removed from the vertebrae, resulting in vertebral bodies with plano-parallel ends. All tests were performed at room temperature using a voice coil-based load frame (ElectroForce 3200; Bose, Eden Prairie, MN). Femoral strength and vertebral strength were defined as the maximum force sustained during the tests. Vertebral stiffness was defined as the maximum slope of the force deformation curve.
RNA extraction and reverse transcription-quantitative polymerase chain reaction
Tissue samples were isolated and kept frozen in liquid nitrogen until processing. Before freezing, epiphyses were removed and bone marrow flushed from humeral bone samples. RNA extraction and reverse transcription-quantitative polymerase chain reaction (PCR) analysis was conducted as previously described (11). Primer sequences (Supplemental Table 1) have been published before (10, 18). All reactions were performed in triplicate, and target gene expression displayed was normalized to the housekeeping gene, Gapdh, in the same sample.
Statistical analysis
All data are presented as means ± standard deviation. Two-tailed Student t tests and analysis of variance were performed as indicated using GraphPad Prism 5.04 (GraphPad Software, La Jolla, CA). Statistical significance was taken as P < 0.05.
Results
Doxycycline suppressed the developmental phenotype of Col1(2.3)+/PTX+ mice
This study was designed to assess the effects of Ob-specific Gi signaling blockade in adult mice, independent of the developmental effects of such a signaling blockade that we previously reported (11). This was achieved by suppressing PTX expression from the TetO-PTX transgene in Col1(2.3)+/PTX+ mice by maintaining these mice on a doxycycline diet until 8 weeks of age, at which time they were transferred to standard chow [Fig. 1(a)]. Doxycycline suppression of transgene expression gradually wanes over 2 weeks, as the doxycycline reservoirs within bone are slowly cleared (8). As expected, this protocol suppressed the runting that we have reported in Col1(2.3)+/PTX+ mice maintained in the absence of doxycycline from conception (11). Femoral lengths of Col1(2.3)+/PTX+ mice maintained on a doxycycline diet to adulthood were unchanged compared with the age- and sex-matched Col1(2.3)+-tTA+ control littermates at 12 weeks of age [Fig. 1(b)].
![Doxycycline (doxy)-mediated suppression of PTX transgene expression allowed for induction of PTX transgene expression in young adult Col1(2.3)+/PTX+ mice that were indistinguishable from control littermates. (a) Schematic diagram of the experimental protocol for the investigation of the effects of PTX-mediated Gi blockade in Obs during aging, independent of the effects of transgene expression during development. (b) Femoral lengths of Col1(2.3)+/PTX+ mice maintained on a doxy diet to adulthood (PTX, induced; n = 3) were unchanged compared with sex-matched control littermates (CTL; n = 10) at 12 weeks of age. This is in contrast to Col1(2.3)+/PTX+ mice that expressed the transgene throughout development (PTX, off doxy; n = 10). ***P < 0.001 vs sex-matched control mice. (c) Quantitative PCR analysis of mRNA isolated from humeri demonstrated the successful induction of PTX expression in these PTX-induced mice (n = 3). **P < 0.01 vs sex-matched control mice; ***P < 0.001 vs sex-matched control mice; (c) P < 0.001 vs baseline measurement. (d, e) Blockade of endogenous Gi signaling in mature Obs did not affect body weight in the Col1(2.3)+/PTX+ transgenic mice during aging [(d) male mice, n = 7 per group; (e) female mice, n = 8 per group]. All data are presented as mean ± standard deviation.](https://oup.silverchair-cdn.com/oup/backfile/Content_public/Journal/endo/158/6/10.1210_en.2016-1365/3/m_en.2016-1365f1.jpeg?Expires=1750303261&Signature=kWiEA-yoZ7jn4a~gILZXXBIbM3THpvFgvcR5HUACye8mVmNNeLRK7zA7PO22KS~Xrf2PTWphxdodvjLc-AQIz0uOarHIMY3CqcFc-kaGWRLJc~kPx66uxU50XH04zajaVH1IGm42bxMPc3Phyw-vw3GJmL76BXOqI4ux436VDPCQdv5d7otvvGpR03FCl0MPLvqTkvsqGSgTmHtaK2bdY3hkwC~MQhjtrfrF8w8xAgW4kxpxnkgElIE5681vnB3GuyBnOiGha1svRYaginKSeuorbKw47FZothPsS2y2qKwZsfTMIgPibDOApreJlL69cAR3RBU8fZDzEcz1PRqQ7w__&Key-Pair-Id=APKAIE5G5CRDK6RD3PGA)
Doxycycline (doxy)-mediated suppression of PTX transgene expression allowed for induction of PTX transgene expression in young adult Col1(2.3)+/PTX+ mice that were indistinguishable from control littermates. (a) Schematic diagram of the experimental protocol for the investigation of the effects of PTX-mediated Gi blockade in Obs during aging, independent of the effects of transgene expression during development. (b) Femoral lengths of Col1(2.3)+/PTX+ mice maintained on a doxy diet to adulthood (PTX, induced; n = 3) were unchanged compared with sex-matched control littermates (CTL; n = 10) at 12 weeks of age. This is in contrast to Col1(2.3)+/PTX+ mice that expressed the transgene throughout development (PTX, off doxy; n = 10). ***P < 0.001 vs sex-matched control mice. (c) Quantitative PCR analysis of mRNA isolated from humeri demonstrated the successful induction of PTX expression in these PTX-induced mice (n = 3). **P < 0.01 vs sex-matched control mice; ***P < 0.001 vs sex-matched control mice; (c) P < 0.001 vs baseline measurement. (d, e) Blockade of endogenous Gi signaling in mature Obs did not affect body weight in the Col1(2.3)+/PTX+ transgenic mice during aging [(d) male mice, n = 7 per group; (e) female mice, n = 8 per group]. All data are presented as mean ± standard deviation.
After the 2-week doxycycline wash-out period, quantitative PCR analysis of mRNA isolated from humeri demonstrated the successful induction of PTX expression to comparable levels in male and female mice [Fig. 1(c)]. Furthermore, baseline measurements of 10-week-old mice that underwent the induction protocol revealed no differences between Col1(2.3)+/PTX+ mice and control littermates in body weight [Fig. 1(d) and 1(e)], fractional bone volume to tissue volume (BV/TV) at the distal femur [Fig. 2(a) and 2(c)], or cortical bone at the TFJ) (Figs. 3 and 4). As mice that underwent the induction protocol aged, there was no divergence in body weights of Col1(2.3)+/PTX+ mice and sex-matched littermate control mice: all groups gained weight with age [Fig. 1(d) and 1(e)]. From 10 weeks to 12 months of age, this increase was from 26 ± 5 g to 35 ± 8 g in male control mice, from 25 ± 3 g to 33 ± 6 g in male Col1(2.3)+/PTX+ mice, from 21 ± 4 g to 29 ± 5 g in female control mice, and from 19 ± 3 g to 27 ± 5 g in female Col1(2.3)+/PTX+ mice. In addition, by visual examination, no obvious physical defects had been observed in the double transgenic mice. Hence, we confirmed that the protocol outlined in Fig. 1(a) successfully prevented the runting and skeletal effects that are observed when blockade of endogenous Gi signaling in Obs is allowed throughout development. This protocol was used for all further experiments.
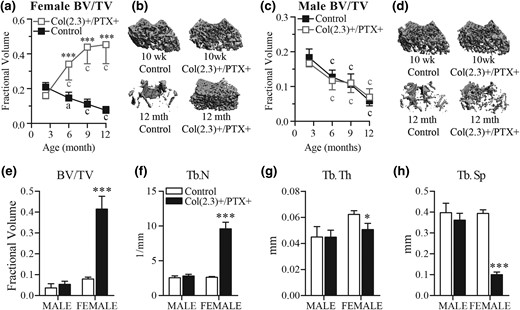
MicroCT assessment of cancellous bone at the distal femur. Col1(2.3)+/PTX+ and Col1(2.3)+ littermate control mice were transferred from a doxycycline diet to a doxycycline-free diet at 8 weeks of age to induce PTX transgene expression in Col1(2.3)+/PTX+ mice and were aged to 12 months. (a–d) Longitudinal in vivo microCT assessment of age-related changes in cancellous BV/TV at the distal femur in Col1(2.3)+/PTX+ and littermate control mice (male mice, n = 7 per group; female mice, n = 8 per group). (b, d) Three-dimensional image reconstruction of cancellous bone at the distal femurs at baseline and at 12 months of age. (e–h) Ex vivo microCT assessment of distal femur in 12-month-old mice that did not undergo in vivo microCT (male mice, n = 6 per group; female mice, n = 4 per group). All data are presented as mean ± standard deviation. *P < 0.05 vs age-matched control mice; ***P < 0.001 vs age-matched control mice; (a) P < 0.05; (c) P < 0.001 vs baseline measurement.
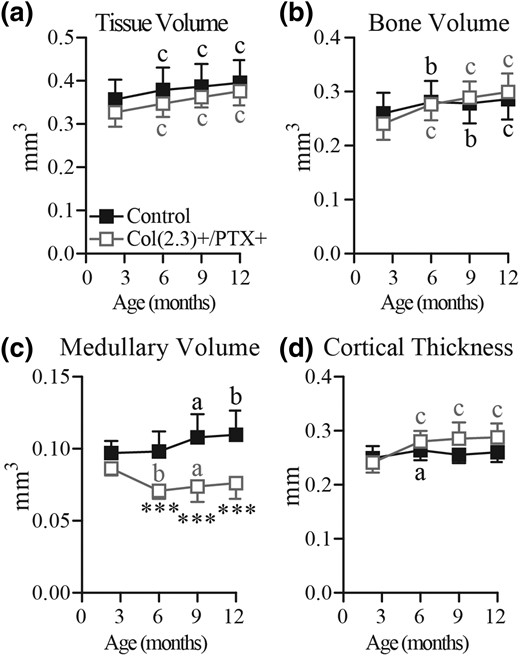
Longitudinal in vivo microCT assessment of age-related changes in cortical bone in female mice. Col1(2.3)+/PTX+ and Col1(2.3)+ littermate control mice were transferred from a doxycycline diet to a doxycycline-free diet at 8 weeks of age to induce PTX transgene expression in Col1(2.3)+/PTX+ mice. In vivo microCT was used to assess cortical bone at the TFJ at 12 weeks, and at 6, 9, and 12 months of age. The reported parameters are (a) TV, (b) BV, (c) medullary volume, and (d) cortical thickness. All data are presented as mean ± standard deviation (n = 8 per group). Statistical comparisons were made vs baseline measurement. ***P < 0.001 vs littermate control mice; (a) P < 0.05; (b) P < 0.01; (c) P < 0.001.
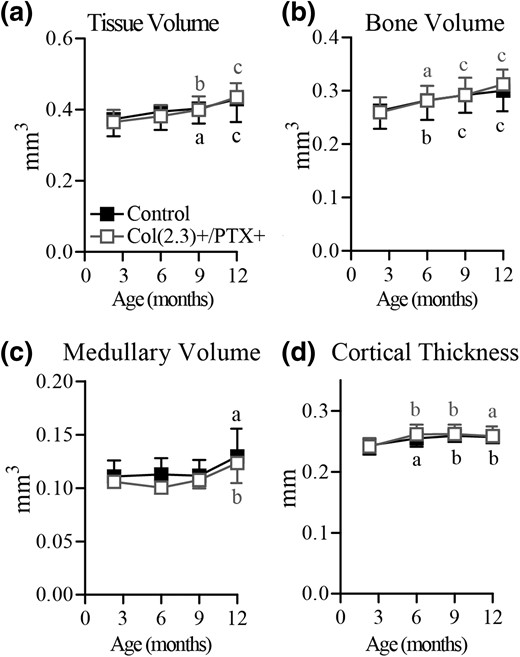
Longitudinal in vivo microCT assessment of age-related changes in cortical bone in male mice. Col1(2.3)+/PTX+ and Col1(2.3)+ littermate control mice were transferred from a doxycycline diet to a doxycycline-free diet at 8 weeks of age to induce PTX transgene expression in Col1(2.3)+/PTX+ mice. In vivo microCT was used to assess cortical bone at the TFJ at 12 weeks, and at 6, 9, and 12 months of age. The reported parameters are (a) TV, (b) BV, (c) medullary volume, and (d) cortical thickness. All data are presented as mean ± standard deviation (n = 7 per group). Statistical comparisons were made vs baseline measurement. (a) P < 0.05; (b) P < 0.01; (c) P < 0.001.
Blockade of Gi signaling in osteoblasts increases cancellous bone in female mice during aging
To assess age-related changes in trabecular bone volume in Col1(2.3)+/PTX+ mice, cancellous bone at the distal femur was examined longitudinally by in vivo microCT scanning with a baseline scan at 10 weeks of age and subsequent scans at 6, 9, and 12 months. During this time, cancellous BV/TV gradually decreased by >50% in both female and male control mice (Fig. 2). In contrast, cancellous BV/TV in the female Col1(2.3)+/PTX+ mice increased with advancing age and, by the end of 12 months of age, there was a 2.8-fold increase in cancellous BV/TV at the distal femur as compared with the 10-week baseline scan, such that at 12 months of age, female Col1(2.3)+/PTX+ mice had fivefold higher BV/TV than age- and sex-matched control mice [Fig. 2(a) and 2(b)]. Surprisingly, the effect of PTX expression in mature Obs was sex specific, with age-related cancellous bone loss being observed in male Col1(2.3)+/PTX+ mice at a comparable rate to control mice [Fig. 2(c) and 2(d)].
Ex vivo microCT scanning was conducted on the same ROI in femurs from a separate cohort of 12-month-old mice that had not undergone in vivo microCT scanning. This confirmed the increase in cancellous BV/TV in female mice only, and allowed for more detailed assessment of trabecular structure [Fig. 2(e–h)]. A 3.7-fold increase in trabecular number (Tb.N) and markedly decreased trabecular spacing (Tb.Sp), associated with a modest (18%) reduction in trabecular thickness (Tb.Th) were demonstrated [Fig. 2(e–h)].
Blockade of Gi signaling stimulated cancellous BFR in aged female Col1(2.3)+/PTX+ mice
Histomorphometry conducted on the 12-month-old femurs confirmed the previous microCT observations and provided further insights about the age-related changes of bone structure with PTX blocking the endogenous Gi signaling in Obs. Compared with sex-matched littermate control mice, female Col1(2.3)+/PTX+ mice showed a 13-fold increase in trabecular BV/TV, a 17-fold increase in Tb.N, and a 23-fold decrease in Tb.Sp (Table 1). A trend toward a modest decrease in trabecular width was not statistically significant. Although Ob surface per bone surface was unaltered in female Col1(2.3)+/PTX+ mice, both MS/BS and MAR were increased, leading to a greater than threefold increase in trabecular BFR compared with littermate control mice. Typical dynamic labeling patterns in trabecular bone of female control and Col1(2.3)+/PTX+ mice are shown in Fig. 5(a) and 5(b). Male Col1(2.3)+/PTX+ mice showed no significant differences in static or dynamic histomorphometric parameters when compared with sex-matched littermate control mice (Table 1).
. | M-ctl (n = 9) . | M-PTX (n = 8) . | F-ctl (n = 4) . | F-PTX (n = 8) . |
---|---|---|---|---|
BV/TV, % | 1.6 ± 0.8 | 2.4 ± 1.2 | 1.9 ± 0.4 | 25.1 ± 7.6a |
Tb.N, 1/mm | 0.65 ± 0.21 | 0.98 ± 0.38 | 0.52 ± 0.11 | 8.98 ± 2.64a |
Tb.Th, µm | 24.2 ± 5.2 | 24.2 ± 4.5 | 36.0 ± 7.1 | 28.7 ± 6.7 |
Tb.Sp, mm | 1.7 ± 0.5 | 1.2 ± 0.5 | 2.1 ± 0.6 | 0.09 ± 0.05b |
ObS/BS, % | 10 ± 5 | 17 ± 8 | 17 ± 9 | 20 ± 5 |
OcS/BS, % | 3.1 ± 3.2 | 2.5 ± 1.2 | 3.0 ± 2.5 | 6.0 ± 2.6 |
MS/BS, % | 19 ± 9 | 23 ± 10 | 37 ± 12 | 64 ± 4c |
MAR, µm/d | 0.8 ± 0.8 | 1.2 ± 0.3 | 1.1 ± 0.8 | 2.5 ± 0.5b |
BFR, µm3/µm2/d | 0.19 ± 0.21 | 0.47 ± 0.33 | 0.47 ± 0.33 | 1.59 ± 0.41b |
. | M-ctl (n = 9) . | M-PTX (n = 8) . | F-ctl (n = 4) . | F-PTX (n = 8) . |
---|---|---|---|---|
BV/TV, % | 1.6 ± 0.8 | 2.4 ± 1.2 | 1.9 ± 0.4 | 25.1 ± 7.6a |
Tb.N, 1/mm | 0.65 ± 0.21 | 0.98 ± 0.38 | 0.52 ± 0.11 | 8.98 ± 2.64a |
Tb.Th, µm | 24.2 ± 5.2 | 24.2 ± 4.5 | 36.0 ± 7.1 | 28.7 ± 6.7 |
Tb.Sp, mm | 1.7 ± 0.5 | 1.2 ± 0.5 | 2.1 ± 0.6 | 0.09 ± 0.05b |
ObS/BS, % | 10 ± 5 | 17 ± 8 | 17 ± 9 | 20 ± 5 |
OcS/BS, % | 3.1 ± 3.2 | 2.5 ± 1.2 | 3.0 ± 2.5 | 6.0 ± 2.6 |
MS/BS, % | 19 ± 9 | 23 ± 10 | 37 ± 12 | 64 ± 4c |
MAR, µm/d | 0.8 ± 0.8 | 1.2 ± 0.3 | 1.1 ± 0.8 | 2.5 ± 0.5b |
BFR, µm3/µm2/d | 0.19 ± 0.21 | 0.47 ± 0.33 | 0.47 ± 0.33 | 1.59 ± 0.41b |
Data are given as mean ± standard deviation. Comparisons with sex- and age-matched control mice were conducted by unpaired t test.
Abbreviations: ctl, control mice; F, female; M, male; ObS/BS, osteoblast surface per bone surface.
P < 0.001.
P < 0.01.
P < 0.05.
. | M-ctl (n = 9) . | M-PTX (n = 8) . | F-ctl (n = 4) . | F-PTX (n = 8) . |
---|---|---|---|---|
BV/TV, % | 1.6 ± 0.8 | 2.4 ± 1.2 | 1.9 ± 0.4 | 25.1 ± 7.6a |
Tb.N, 1/mm | 0.65 ± 0.21 | 0.98 ± 0.38 | 0.52 ± 0.11 | 8.98 ± 2.64a |
Tb.Th, µm | 24.2 ± 5.2 | 24.2 ± 4.5 | 36.0 ± 7.1 | 28.7 ± 6.7 |
Tb.Sp, mm | 1.7 ± 0.5 | 1.2 ± 0.5 | 2.1 ± 0.6 | 0.09 ± 0.05b |
ObS/BS, % | 10 ± 5 | 17 ± 8 | 17 ± 9 | 20 ± 5 |
OcS/BS, % | 3.1 ± 3.2 | 2.5 ± 1.2 | 3.0 ± 2.5 | 6.0 ± 2.6 |
MS/BS, % | 19 ± 9 | 23 ± 10 | 37 ± 12 | 64 ± 4c |
MAR, µm/d | 0.8 ± 0.8 | 1.2 ± 0.3 | 1.1 ± 0.8 | 2.5 ± 0.5b |
BFR, µm3/µm2/d | 0.19 ± 0.21 | 0.47 ± 0.33 | 0.47 ± 0.33 | 1.59 ± 0.41b |
. | M-ctl (n = 9) . | M-PTX (n = 8) . | F-ctl (n = 4) . | F-PTX (n = 8) . |
---|---|---|---|---|
BV/TV, % | 1.6 ± 0.8 | 2.4 ± 1.2 | 1.9 ± 0.4 | 25.1 ± 7.6a |
Tb.N, 1/mm | 0.65 ± 0.21 | 0.98 ± 0.38 | 0.52 ± 0.11 | 8.98 ± 2.64a |
Tb.Th, µm | 24.2 ± 5.2 | 24.2 ± 4.5 | 36.0 ± 7.1 | 28.7 ± 6.7 |
Tb.Sp, mm | 1.7 ± 0.5 | 1.2 ± 0.5 | 2.1 ± 0.6 | 0.09 ± 0.05b |
ObS/BS, % | 10 ± 5 | 17 ± 8 | 17 ± 9 | 20 ± 5 |
OcS/BS, % | 3.1 ± 3.2 | 2.5 ± 1.2 | 3.0 ± 2.5 | 6.0 ± 2.6 |
MS/BS, % | 19 ± 9 | 23 ± 10 | 37 ± 12 | 64 ± 4c |
MAR, µm/d | 0.8 ± 0.8 | 1.2 ± 0.3 | 1.1 ± 0.8 | 2.5 ± 0.5b |
BFR, µm3/µm2/d | 0.19 ± 0.21 | 0.47 ± 0.33 | 0.47 ± 0.33 | 1.59 ± 0.41b |
Data are given as mean ± standard deviation. Comparisons with sex- and age-matched control mice were conducted by unpaired t test.
Abbreviations: ctl, control mice; F, female; M, male; ObS/BS, osteoblast surface per bone surface.
P < 0.001.
P < 0.01.
P < 0.05.
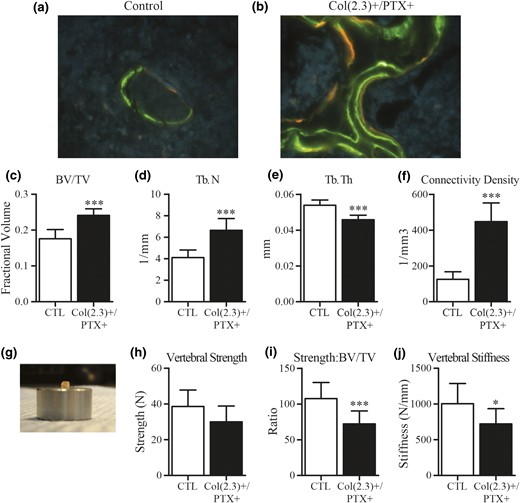
Dynamic labeling of cancellous bone at distal femur, and ex vivo assessment of L5 vertebral bodies from 12-month old female Col1(2.3)+/PTX+ and littermate control mice. Col1(2.3)+/PTX+ and Col1(2.3)+ littermate control mice were transferred from a doxycycline diet to a doxycycline-free diet at 8 weeks of age to induce PTX transgene expression in Col1(2.3)+/PTX+ mice and were aged to 12 months. (a, b) Representative images of dynamic histomorphometry of the distal femur in the female Col1(2.3)+/PTX+ and littermate control mice (green, calcein labeling given at −21 and −7 days; orange, demeclocycline labeling given at −2 days). (c–f) Ex vivo microCT assessment of the L5 vertebra. (g–j) Biomechanical properties of the L5 vertebral body assessed by compression testing. Before compression testing, the posterior elements and end plates of the vertebrae were removed to yield a vertebral body with plano-parallel ends, as imaged. All data are presented as mean ± standard deviation (n = 8 per group). *P < 0.05 vs control mice; ***P < 0.001 vs control mice.
Effects of blockade of Gi signaling on biomechanical strength of L5 vertebral bone from aged female Col(2.3)+/PTX+ mice
We assessed whether the biomechanical strength of cancellous-rich vertebral bone was enhanced in female Col1(2.3)+/PTX+ mice, by conducting compression testing on L5 vertebral bodies collected from 12-month-old mice. Before testing, we conducted ex vivo microCT scanning on the L5 vertebral bodies to confirm whether the dramatic effects of Ob-specific Gi signaling blockade observed in cancellous bone of the peripheral skeleton were also present in the axial skeleton. A similar, but less pronounced, phenotype was observed with a 1.4-fold increase in fractional BV driven by a 1.6-fold increase in Tb.N and associated with a 3.5-fold increase in connectivity density of trabeculae as compared with age- and sex-matched littermate control mice (Fig. 5). Although these increases in trabecular volume fraction, Tb.N, and connectivity would normally be expected to improve biomechanical properties, Ob-specific Gi signaling blockade also caused a modest 15% reduction in Tb.Th [Fig. 5(e)], which could diminish biomechanical properties. Indeed, no difference in vertebral compressive strength (load to failure) was observed between control mice and Col1(2.3)+/PTX+ mice [Fig. 5(g) and 5(h)], and both bone strength relative to fractional bone volume and vertebral stiffness were reduced in Col1(2.3)+/PTX+ L5 vertebrae [Fig. 5(i) and 5(j)]. This finding suggests that the biomechanical effect of increased trabecular BV/TV and connectivity is offset by the effects of reduced Tb.Th, or that changes in bone tissue material properties and structural properties are responsible for the impaired strength and stiffness of the Col1(2.3)+/PTX+ cancellous bone.
Blocking Gi signaling in Obs increased cortical bone in female mice during aging
Age-related changes in cortical BV in Col1(2.3)+/PTX+ mice were assessed at TFJ by in vivo microCT, with a baseline scan at 10 weeks of age and subsequent scans at 6, 9, and 12 months. The baseline scans demonstrated that there were no differences in cortical bone TV at the TFJ between Col1(2.3)+/PTX+ mice and littermate control mice for either female (Fig. 3) or male (Fig. 4) mice. In female control mice, advancing age resulted in increasing TV and medullary volume, with maintenance of BV and cortical thickness, whereas in female Col1(2.3)+/PTX+ mice, increased cortical TV with advancing age was accompanied by an increase in BV and cortical thickness, and a decrease in medullary volume (Fig. 3). Medullary volume was statistically decreased in female Col1(2.3)+/PTX+ mice compared with control mice from 6 months of age [Fig. 3(c)]. Ex vivo microCT conducted at the midshaft of 12-month-old femurs demonstrated similar changes in Col1(2.3)+/PTX+ female mice at this alternate cortical site [Fig. 6(a–d)]. Compared with littermate control mice, femoral midshaft cortical thickness and BV were increased with a concomitant decrease in medullary volume [Fig. 6(a–d)].
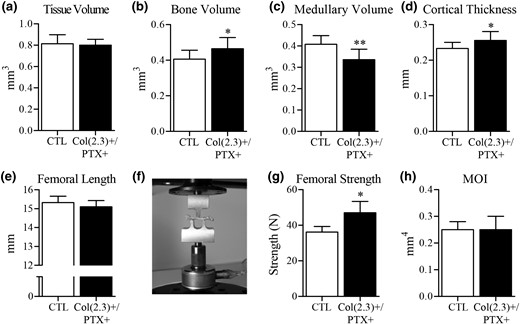
Ex vivo microCT and mechanical testing of cortical bone at the femoral midshaft. Col1(2.3)+/PTX+ and Col1(2.3)+ littermate control mice were transferred from a doxycycline diet to a doxycycline-free diet at 8 weeks of age to induce PTX transgene expression in Col1(2.3)+/PTX+ mice and were aged to 12 months. Ex vivo microCT assessment of midshaft of femur yielded measurements of (a) TV, (b) BV, (c) medullary volume, and (d) cortical thickness. (e) Femoral length was measured before (f) conducting three-point bend testing. (g) Femoral strength was the maximum force sustained during the test. (h) MOI was calculated from microCT images. All data are presented as mean ± standard deviation (n = 8 per group). *P < 0.05 vs control mice; **P < 0.01 vs control mice. MOI, moment of inertia.
The pattern of age-related changes in cortical bone structure observed at the TFJ was identical between male Col1(2.3)+/PTX+ mice and control mice (Fig. 4). TV and BV consistently increased with aging, whereas cortical thickness was increased at 6 months and maintained thereafter and medullary volume was only increased above baseline at 12 months of age. These data indicate that blockade of endogenous Gi signaling in Obs of the mature skeleton influences age-related cortical remodeling in female mice but not male mice.
Blockade of Gi signaling stimulated endocortical bone formation in aged female Col1(2.3)+/PTX+ mice
In female Col1(2.3)+/PTX+ mice, the observed age-related changes in cortical structure of increasing cortical thickness and BV, with markedly decreasing medullary volume (Figs. 3 and 6), are suggestive of changes in endocortical bone turnover. Dynamic histomorphometry carried out at the midshaft of 12-month-old femurs indicated a 14-fold higher endocortical BFR in female Col1(2.3)+/PTX+ mice as compared with control mice (Table 2). This resulted from increases in both MS/BS (threefold) and MAR (fivefold). No differences were observed in periosteal BFR in female mice, consistent with the comparable tissue volume by microCT [Fig. 6(a)] and periosteal perimeter (Table 2) in female Col1(2.3)+/PTX+ mice and littermate control mice. No differences in any of these cortical parameters were observed between male Col1(2.3)+/PTX+ mice and littermate control mice (Table 2).
. | M-ctl (n = 8) . | M-PTX (n = 4) . | F-ctl (n = 5) . | F-PTX (n = 7) . |
---|---|---|---|---|
Ps.Pm, mm | 6.9 ± 0.4 | 6.8 ± 0.4 | 6.2 ± 0.6 | 6.2 ± 0.4 |
Ps.MS, % | 39 ± 13 | 20 ± 4 | 48 ± 18 | 29 ± 23 |
Ps.MAR, μm/d | 0.50 ± 0.18 | 0.44 ± 0.09 | 0.44 ± 0.09 | 0.46 ± 0.14 |
Ps.BFR, μm3/μm2/d | 0.21 ± 0.14 | 0.09 ± 0.03 | 0.26 ± 0.11 | 0.14 ± 0.12 |
Ec.Pm, mm | 5.0 ± 0.4 | 5.3 ± 0.5 | 4.4 ± 0.4 | 5.4 ± 0.6a |
Ec.MS, % | 18 ± 6 | 22 ± 4 | 17 ± 6 | 54 ± 24a |
Ec.MAR, μm/d | 0.62 ± 0.37 | 0.55 ± 0.40 | 0.21 ± 0.19 | 1.09 ± 0.25b |
Ec.BFR, μm3/μm2/d | 0.12 ± 0.08 | 0.13 ± 0.12 | 0.04 ± 0.04 | 0.59 ± 0.28a |
. | M-ctl (n = 8) . | M-PTX (n = 4) . | F-ctl (n = 5) . | F-PTX (n = 7) . |
---|---|---|---|---|
Ps.Pm, mm | 6.9 ± 0.4 | 6.8 ± 0.4 | 6.2 ± 0.6 | 6.2 ± 0.4 |
Ps.MS, % | 39 ± 13 | 20 ± 4 | 48 ± 18 | 29 ± 23 |
Ps.MAR, μm/d | 0.50 ± 0.18 | 0.44 ± 0.09 | 0.44 ± 0.09 | 0.46 ± 0.14 |
Ps.BFR, μm3/μm2/d | 0.21 ± 0.14 | 0.09 ± 0.03 | 0.26 ± 0.11 | 0.14 ± 0.12 |
Ec.Pm, mm | 5.0 ± 0.4 | 5.3 ± 0.5 | 4.4 ± 0.4 | 5.4 ± 0.6a |
Ec.MS, % | 18 ± 6 | 22 ± 4 | 17 ± 6 | 54 ± 24a |
Ec.MAR, μm/d | 0.62 ± 0.37 | 0.55 ± 0.40 | 0.21 ± 0.19 | 1.09 ± 0.25b |
Ec.BFR, μm3/μm2/d | 0.12 ± 0.08 | 0.13 ± 0.12 | 0.04 ± 0.04 | 0.59 ± 0.28a |
Data are given as mean ± standard deviation. Comparisons with sex- and age-matched control micewere conducted by unpaired t test.
Abbreviations: Ec.BFR, endosteal bone formation rate; Ec.MAR, endosteal mineral apposition rate; Ec.MS, endosteal mineralizing surface per bone surface; Ec.Pm, endosteal perimeter; Ps.BFR, periosteal bone-formation rate; Ps.MAR, periosteal mineral apposition rate; Ps.MS, periosteal mineralizing surface per bone surface; Ps.Pm, periosteal perimeter.
P < 0.01.
P < 0.001.
. | M-ctl (n = 8) . | M-PTX (n = 4) . | F-ctl (n = 5) . | F-PTX (n = 7) . |
---|---|---|---|---|
Ps.Pm, mm | 6.9 ± 0.4 | 6.8 ± 0.4 | 6.2 ± 0.6 | 6.2 ± 0.4 |
Ps.MS, % | 39 ± 13 | 20 ± 4 | 48 ± 18 | 29 ± 23 |
Ps.MAR, μm/d | 0.50 ± 0.18 | 0.44 ± 0.09 | 0.44 ± 0.09 | 0.46 ± 0.14 |
Ps.BFR, μm3/μm2/d | 0.21 ± 0.14 | 0.09 ± 0.03 | 0.26 ± 0.11 | 0.14 ± 0.12 |
Ec.Pm, mm | 5.0 ± 0.4 | 5.3 ± 0.5 | 4.4 ± 0.4 | 5.4 ± 0.6a |
Ec.MS, % | 18 ± 6 | 22 ± 4 | 17 ± 6 | 54 ± 24a |
Ec.MAR, μm/d | 0.62 ± 0.37 | 0.55 ± 0.40 | 0.21 ± 0.19 | 1.09 ± 0.25b |
Ec.BFR, μm3/μm2/d | 0.12 ± 0.08 | 0.13 ± 0.12 | 0.04 ± 0.04 | 0.59 ± 0.28a |
. | M-ctl (n = 8) . | M-PTX (n = 4) . | F-ctl (n = 5) . | F-PTX (n = 7) . |
---|---|---|---|---|
Ps.Pm, mm | 6.9 ± 0.4 | 6.8 ± 0.4 | 6.2 ± 0.6 | 6.2 ± 0.4 |
Ps.MS, % | 39 ± 13 | 20 ± 4 | 48 ± 18 | 29 ± 23 |
Ps.MAR, μm/d | 0.50 ± 0.18 | 0.44 ± 0.09 | 0.44 ± 0.09 | 0.46 ± 0.14 |
Ps.BFR, μm3/μm2/d | 0.21 ± 0.14 | 0.09 ± 0.03 | 0.26 ± 0.11 | 0.14 ± 0.12 |
Ec.Pm, mm | 5.0 ± 0.4 | 5.3 ± 0.5 | 4.4 ± 0.4 | 5.4 ± 0.6a |
Ec.MS, % | 18 ± 6 | 22 ± 4 | 17 ± 6 | 54 ± 24a |
Ec.MAR, μm/d | 0.62 ± 0.37 | 0.55 ± 0.40 | 0.21 ± 0.19 | 1.09 ± 0.25b |
Ec.BFR, μm3/μm2/d | 0.12 ± 0.08 | 0.13 ± 0.12 | 0.04 ± 0.04 | 0.59 ± 0.28a |
Data are given as mean ± standard deviation. Comparisons with sex- and age-matched control micewere conducted by unpaired t test.
Abbreviations: Ec.BFR, endosteal bone formation rate; Ec.MAR, endosteal mineral apposition rate; Ec.MS, endosteal mineralizing surface per bone surface; Ec.Pm, endosteal perimeter; Ps.BFR, periosteal bone-formation rate; Ps.MAR, periosteal mineral apposition rate; Ps.MS, periosteal mineralizing surface per bone surface; Ps.Pm, periosteal perimeter.
P < 0.01.
P < 0.001.
Blockade of Gi signaling increased biomechanical strength of cortical bone from aged female Col(2.3)+/PTX+ mice
Cortical thickness and TV are both key determinants of cortical bone strength (19, 20). Given the increased cortical thickness in female Col(2.3)+/PTX+ mice, we examined femoral strength by three-point bend testing. Curiously, despite changes in cortical structure and increased femoral strength in the three-point bend test, the moment of inertia along the test axis was unchanged in female Col(2.3)+/PTX+ mice as compared with littermate control mice (Fig. 6(e) and 6(f)]. As a result, we examined further structural parameters that may explain increased femoral strength, as could be determined from microCT images of the femurs. We observed a trend toward higher section modulus in female Col(2.3)+/PTX+ mice (0.27 ± 0.02 mm3 vs 0.30 ± 0.03 mm3; P = 0.11), which was associated with decreased medullary volume, presumably due to the observed increases in endosteal bone formation (Table 2). To determine whether blocking Gi signaling improved bone material properties, another potential explanation for increased femoral strength, we assessed humeral cortical bone by Fourier transform infrared spectroscopy. However, no material differences between bone from Col(2.3)+/PTX+ mice and control littermates were elucidated (data not shown).
Blockade of Gi signaling did not affect serum markers of bone turnover
We also performed serum analysis on the global bone formation marker PINP and the resorption marker PYD and did not find any statistically significant difference for both sexes between Col1(2.3)+/PTX+ mice and their littermate mice (Supplemental Fig. 1).
Molecular mechanism of blockade of Gi signaling in Obs on bone formation
To elucidate the molecular mechanism of endogenous Gi signaling restraining Obs during aging, RNA was extracted from the 12-month-old mice. Real-time PCR demonstrated that specific expression of the PTX gene in Obs dramatically increased the level of both early osteoblastic marker genes (Runx2 and Osterix) and mature osteoblast markers (collagen type I and osteocalcin) in the female Col1(2.3)+/PTX+ mice only (Supplemental Fig. 2). The increase in Ob marker gene expression in female Col1(2.3)+/PTX+ mice compared with female control mice was similar in magnitude to the increase in trabecular BV in these mice, and likely reflects an increase in the proportion of Obs present in the whole bone preparation.
To reveal whether PTX expression affects the remodeling activity, expression of RANKL, OPG, and the ratio of RANKL to OPG were measured. Although PTX expression upregulated the RANKL and OPG mRNA levels individually in the female Col1(2.3)+/PTX+ mice, the ratio of RANKL to OPG remained unchanged (Supplemental Fig. 2). Meanwhile, the level of calcitonin receptor mRNA, a gene highly expressed in osteoclasts, was increased in the female transgenic mice only. These findings suggest that the altered bone resorption might be secondary to an increase in bone formation (10). We examined expression of two Wnt-pathway inhibitors that are known negative regulators of bone formation, Dkk1 and sclerostin (Supplemental Fig. 2). Whereas Dkk1 expression was increased in female COL1(2.3)+/PTX+ mice, similar to markers of bone cells (Obs and osteoclasts), sclerostin expression was not. This suggests that mechanisms to increase sclerostin expression may be blunted in female mice with osteoblastic PTX expression.
Surprisingly, we found that the level of expression of the PTX transgene was much lower in the aged male mice compared with female mice (Supplemental Fig. 2). This may reflect both the presence of fewer Obs in the aged male mouse and the modestly lower levels of PTX expression in Obs at the time of induction [Fig. 1(c)]. The pattern is consistent with the previous observation of changes in BV at the distal femur and cortical bone and changes of osteogenic marker genes (11). However, whether the endogenous Gi signaling in the male Obs in terms of the aged male mouse unaltered bone phenotype during aging is effectively blocked by the PTX transgene remains to be elucidated.
Discussion
In the mouse, bone mass peaks at 2 to 3 months of age and declines thereafter during aging (21, 22). The goal of the current study was to determine the role of Ob Gi signaling in age-related bone loss. Our previous studies in mice demonstrated that blockade of Ob Gi signaling during growth and development results in increased cortical thickness (in both sexes) and increased fractional cancellous BV (female mice only) (11). In the current study, we prevented developmental effects by delaying PTX expression until ∼10 weeks (2.5 months) of age. Assessment of 12-week-old mice demonstrated that transfer to a doxycycline-free diet induced similar levels of PTX gene expression in both male and female Col1(2.3)+/PTX+ mice. Surprisingly, the delayed expression of PTX led to a gradual increase of BV in female Col1(2.3)+/PTX+ mice with advancing age. These results support the hypothesis that endogenous of Gi signaling in Obs restrains bone formation and promotes age-related bone loss in female mice.
In young mice, expression of PTX in Obs resulted in a regional increase in periosteal BFR without affecting endocortical bone formation (11). In contrast, we demonstrated in the current study that blockade of Gi signaling caused a significant increase in bone formation at the endosteal surface without affecting periosteal bone formation in aging mice. These results support the idea that Obs at periosteal and endocortical surfaces may be distinguished from each other either by the complement of Gi-coupled GPCRs that they express or by differential exposure to Gi-GPCR ligands (11). Cortical bone size and thickness are key determinants of bone strength and fracture risk (19, 20). Advancing age is associated with a decrease in periosteal apposition and an increase in endocortical resorption (23). Therefore, the increase in biomechanical strength of the femur in adult female Col1(2.3)+/PTX+ mice was likely due to increased bone formation by endosteal osteoblasts.
Bone resorption and formation are two coupled aspects of the remodeling cycle and advancing age is associated with a decline in the volume of bone formed by each basic multicellular unit. Blockade of Gi signaling in Obs in female mice led to a significant increase in bone formation on trabecular surfaces with some maintenance of coupling. The ratio of expression levels of the osteoclastogenic regulators RANKL and OPG and the bone resorption marker PYD were unchanged in Col1(2.3)+/PTX+ mice as compared with control littermates. In addition, histomorphometric assessment also revealed that osteoclast surface per bone surface was maintained in these mice. Expression of the osteoclastic marker gene calcitonin receptor showed a comparable, approximately fivefold increase as was observed for Ob marker genes (i.e., Runx2, Osterix, Collagen Type 1, and Osteocalcin) compared with sex-matched littermate control mice. Despite these indicators of increased osteoclast quantity, the progressive increase in BV suggests an imbalance in the amount of bone formation and bone resorption, and suggests that Gi signaling in Obs contributes to age-related bone loss by restraining the activity of Obs in the cancellous compartment and at the endocortical bone surface.
Our study used genetic approaches to specifically abolish the Gi pathway in Obs. However, redundancy of the multiple Gαi subunits and the large number of GPCRs pose substantial challenges to target the specific GPCRs and ligands contributing the bone loss during aging, as seen in the female Col1(2.3)+/PTX+ mice. Emerging evidence has revealed some promising candidates for osteoporosis. For instance, apelin, an adipokine, plays an important role in bone metabolism and restrains bone formation in aged mice by decreasing Ob surfaces and numbers (10). Sphingosine 1-phosphate receptor 1 is another Gi protein-coupled receptor and the level of plasma sphingosine 1-phosphate is inversely correlated with bone mineral density (24, 25). It will be essential to identify the role of specific Gi-GPCRs in Obs in suppressing bone formation in female mice.
There are some caveats in this study. First, the effect of blocking endogenous of Gi signaling in Obs seen in female transgenic mice was not observed in male mice during aging. The basis for the sex difference in the skeletal response to PTX expression during aging is not clear. It is possible that Gi signaling (or the osteoblast response to it) is more prominent in aging female mice than in aging male mice. We currently have no direct evidence to support this notion. Another possibility is that the PTX transgene might not have been as effective in blocking Gi signaling in Obs from aging male mice vs those from female mice. In support of this possibility, the level of expression of the PTX transgene was much lower in adult male mice compared with age-matched female mice. Unfortunately, we have not been successful in developing an experimental approach to unambiguously confirm successful blockade of Gi signaling in Obs from aging male mice.
Second, blocking Gi signaling in Obs resulted in a striking bone anabolic effect in female Col1(2.3)+/PTX+ mice with aging, in which the BV gain was mainly associated with an increased Tb.N and connectivity. But there was a decrease in Tb.Th at both the distal femur and lumbar vertebrae in the Col1(2.3)+/PTX+ mice. The decreased vertebral stiffness suggests that the beneficial effects on bone stiffness expected to result from increased BV and Tb.N are not sufficient to offset the negative biomechanical effects resulting from decreased trabecular thickness. Alternatively, there may be concomitant changes in bone material properties that offset the increase in BV, because other factors such as tissue material properties or mechanical stress (weight bearing) contribute to bone strength (26,–28).
In conclusion, our results indicate that Gi signaling restrains bone formation at endosteal and trabecular surfaces in a sex-dependent manner and blockade of Gi signaling with a specific expression of PTX transgene in Obs stimulates bone formation, increases long-bone strength, and prevents age-related bone loss in the adult female Col1(2.3)+/PTX+ mice. Therefore, therapy designed to inhibit GPCR-mediated Gi activation in Obs could be useful in the treatment of age-related bone loss in women, provided that deleterious effects on the appendicular skeleton can be circumvented.
Abbreviations:
- BFR
bone formation rate
- BV
bone volume
- BV/TV
bone volume to tissue volume
- GPCR
G protein–coupled receptor
- L5
fifth lumbar
- MAR
mineral apposition rate
- microCT
microcomputed tomography
- MS/BS
mineralizing surface to bone surface
- Ob
osteoblast
- PCR
polymerase chain reaction
- PTH
parathyroid hormone
- PTX
pertussis toxin
- ROI
region of interest
- Tb.N
trabecular number
- Tb.Sp
trabecular spacing
- Tb.Th
trabecular thickness
- TFJ
tibiofibular junction
- TV
tissue volume.
Acknowledgments
We thank Dr. Bruce R. Conklin (Gladstone Institute of Cardiovascular Disease, University of California, San Francisco) for generously providing the TetO-PTX transgenic mice, and we thank San Francisco VA Medical Center Bone Core for its technical assistance.
These studies were supported by National Institutes of Health Grants R01 DK072071 and P30 AR066262 and by Veterans Affairs Merit Review Program Grant BX001496-01.
Disclosure Summary: The authors have nothing to disclose.
References
Author notes
Address all correspondence and requests for reprints to: Robert A. Nissenson, PhD, VA Medical Center (111 N-MB), University of California, 1700 Owens Street, San Francisco, California 94158. E-mail: [email protected].