-
PDF
- Split View
-
Views
-
Cite
Cite
Jiarong Li, Aimée-Lee Luco, Benoît Ochietti, Ibtihal Fadhil, Anne Camirand, Timothy A. Reinhardt, René St-Arnaud, William Muller, Richard Kremer, Tumoral Vitamin D Synthesis by CYP27B1 1-α-Hydroxylase Delays Mammary Tumor Progression in the PyMT-MMTV Mouse Model and Its Action Involves NF-κB Modulation, Endocrinology, Volume 157, Issue 6, 1 June 2016, Pages 2204–2216, https://doi.org/10.1210/en.2015-1824
- Share Icon Share
Biologically active vitamin D (1,25-dihydroxycholecalciferol or 1,25(OH)2D) is synthetized from inactive prohormone 25-hydroxycholecalciferol (25(OH)D) by the enzyme CYP27B1 1-α-hydroxylase in kidney and several extrarenal tissues including breast. Although the development of breast cancer has been linked to inadequate vitamin D status, the importance of bioactive vitamin D production within tumors themselves is not fully understood. To investigate the role of tumoral vitamin D production in mammary epithelial cell progression to breast cancer, we conducted a Cre-loxP-mediated Cyp27b1 gene ablation in the mammary epithelium of the polyoma middle T antigen-mouse mammary tumor virus (PyMT-MMTV) mouse breast cancer model. Targeted ablation of Cyp27b1 was accompanied by significant acceleration in initiation of spontaneous mammary tumorigenesis. In vivo, cell proliferation, angiogenesis, cell cycle progression, and survival markers were up-regulated in tumors by Cyp27b1 ablation, and apoptosis was decreased. AK thymoma (AKT) phosphorylation and expression of several components of nuclear factor κB (NF-κB), integrin, and signal transducer and activator of transcription 3 (STAT3) signaling pathways were increased in Cyp27b1-ablated tumors compared with nonablated controls. In vitro, 1,25(OH)2D treatment induced a strong antiproliferative action on tumor cells from both ablated and nonablated mice, accompanied by rapid disappearance of NF-κB p65 from the nucleus and segregation in the cytoplasm. In contrast, treatment with the metabolic precursor 25(OH)D was only effective against cells from nonablated mice. 25(OH)D did not inhibit growth of Cyp27b1-ablated cells, and their nuclear NF-κB p65 remained abundant. Our findings demonstrate that in-tumor CYP27B1 1-α-hydroxylase activity plays a crucial role in controlling early oncogene-mediated mammary carcinogenesis events, at least in part by modulating tumoral cell NF-κB p65 nuclear translocation.
People who live in northern latitudes suffer a higher incidence of chronic diseases than populations living further south (1). Reduced exposure to solar radiation has been similarly associated to higher risk for colon, prostate and breast cancers that are accompanied by vitamin D3 deficiency (2–5). Not surprisingly, improved vitamin D nutritional status was observed to decrease all-cancer risk in postmenopausal women from northern climates (6). However, the role played in cancer development by bioactive vitamin D synthesized within the tumors themselves has not been fully understood.
Humans produce vitamin D precursors in their skin through sun exposure, and can also ingest the precursors in their diet. The liver then synthesizes the 25-hydroxycholecalciferol (25(OH)D) prohormone through the action of the 25-hydroxylase enzyme cytochrome P450 (CYP) 2R1 (7). 25(OH)D is the main circulating form of vitamin D but is biologically inert and must be activated through hydroxylation to 1,25-dihydroxycholecalciferol (1,25(OH)2D) by the 1-α-hydroxylase enzyme (CYP27B1) present in renal and many extrarenal tissues including breast. The production and action of bioactive vitamin D follow 2 distinct pathways depending on the tissue (8). Kidneys present an endocrine route where the 1-α-hydroxylase enzyme is regulated by plasma calcium, phosphorus and parathyroid hormone, and the active 1,25(OH)2D is released to produce systemic calcemic effects. In contrast, extrarenal tissues possess an autocrine/paracrine pathway where the activity of 1-α-hydroxylase is substrate dependent and produces active 1,25(OH)2D that does not appear to be secreted (9) but exerts local effects (10). Active 1,25(OH)2D from renal and other sources acts as a classical steroid hormone and binds the vitamin D receptor (VDR) in target organs (11). VDR forms a dimeric complex with the retinoid X receptor (12), recruits coactivators/corepressors (13), and interacts with discrete vitamin D-responsive elements in DNA as a ligand-activated transcription factor. As a protection against excessive 1,25(OH)2D levels, the molecule can be inactivated to 1,24,25(OH)3D by the action of 24-hydroxylase (CYP24A1) (14, 15).
The classical action of vitamin D on bone metabolism and mineral homeostasis is well known, and its nonclassical but important implication in tumorigenesis has been widely reported. 1,25(OH)2D displays antiproliferative effects in several cancers including breast (16–26) where it induces apoptosis (19, 20, 27) and decreases in vitro invasion and angiogenesis (28–31). Several components of the vitamin D pathway are suspected of playing a role in breast oncogenic events. CYP24A1, which degrades 1,25(OH)2D, would be expected to decrease the local anticancer protection effect of 1,25(OH)2D in extrarenal tissues. Indeed, the Cyp24 gene is often amplified in human breast cancer and is considered a candidate oncogene (32). Similarly, VDR has been observed to modulate oncogene-induced mammary tumorigenesis in vivo in mouse mammary tumor virus (MMTV)-neu mice (33) and to regulate the c-MYC (myelocytomatosis oncogene) pathway (34, 35). A low level of VDR expression has also been proposed as part of a novel biomarker signature for triple-negative breast cancer and VDR ablation reduces carcinogen-induced tumorigenesis in mammary gland (36, 37). Another potentially important component of the vitamin D pathway in breast cancer cells is the CYP27B1 hydroxylase whose inhibition would result in inadequate levels of 1,25(OH)2D for VDR binding. Complex regulation mechanisms apply to CYP27B1, because its mRNA is up-regulated in some types of cancer and down-regulated in others (10, 26), and splice variants causing catalytically inactive enzymes occur frequently (38, 39). As mentioned above, CYP27B1 functions differently in renal and extrarenal tissues, with enzymes from extrarenal locations such as breast displaying autocrine/paracrine action for their locally synthesized active 1,25(OH)2D which is mostly not released in the circulation except in granulomatous conditions (40). The growing body of evidence linking vitamin D and breast cancer prompts speculation on the role played by locally produced 1,25(OH)2D in breast tumoral development and the exact molecular mechanisms for a potential antineoplastic action.
The nuclear factor κB (NF-κB) family of nuclear transcription factors includes p65 (RelA), p105/p50, p100/p52, RelB, and c-Rel proteins. These factors are involved in the regulation of growth, inflammation, apoptosis, cell survival, and cancer development (41, 42). In its canonical presentation, NF-κB is composed of a p65/p50 heterodimer sequestered in the cytoplasm as an inactive complex through its association with the inhibitor IkB (inhibitor of kappa B kinase). When extracellular signals stimulate the activation of IkB kinases, IkB is released from the NF-κB dimer and degraded, allowing NF-κB to translocate to the nucleus where it can activate target genes. In the noncanonical pathway, IKK kinase catalyzes the processing of NF-κB p100 to p52 and the translocation of the p52/RelB dimer to the nucleus (41). NF-κB is known to bind to transcription factor signal transducer and activator of transcription 3 (STAT3) and coact in the control of interactions between malignant cells and their microenvironment (43). NF-κB and Stat3 are key regulators of epithelial tumorigenesis and are among the most commonly activated transcription factors in cancer (44). The metabolism of NF-κB and vitamin D interacts at several points in a complex and sometimes contradictory manner (41, 45). For example, 1,25(OH)2D can either activate or inactivate NF-κB action depending on the cell system (41). In cancer situations, vitamin D and derivatives inhibit NF-κB action (46, 47), with consequences on reduction of inflammation, angiogenesis, apoptosis, invasion and migration (21).
To investigate the role of tumoral CYP27B1 1-α-hydroxylase in breast cancer, we deleted the Cyp27b1 gene in the mammary epithelium (ME) of the MMTV promoter-driven polyoma middle T antigen (PyMT) oncoprotein (PyMT-MMTV) mouse. The PyMT-MMTV model of breast cancer displays highly aggressive spontaneous mammary tumors closely mimicking the human disease in its progression from premalignant to malignant stage followed by high metastatic frequency, and in its expression of poor outcome biomarkers (loss of estrogen and progesterone receptors and integrin 1 at later stages, and persistent expression of ErbB2 and cyclinD1) (48, 49) PyMT-MMTV is related to the human luminal cell phenotype and is similar to human HER+/ER− breast cancer (50). Although the Cyp27b1-ablated mice presented normal mammary gland development, their spontaneous mammary cancer was accelerated, and more numerous as well as larger tumors were observed compared with nonablated PyMT-MMTV controls. Tumors from ablated mice showed increased expression of cell proliferation and angiogenesis markers, decreased apoptosis, and the ablated tumor cells lost the capacity to segregate NF-κB p65 in the cytoplasm in response to 25(OH)D treatment.
Materials and Methods
Conditional ablation of the Cyp27b1 gene in mouse ME
The Cyp27b1flox/flox mice on C57/BL6 background were derived from animals obtained from Dr René St-Arnaud. In the original mice, Cyp27b1 gene exon 8 (containing the hemebinding region essential to biological activity) was floxed (51). Cyp27b1flox/flox animals were backcrossed 12 generations to friend virus B-type mouse (FVB) mice to obtain a 99% FVB/NJ background. The mice were bred to MMTV-PyMT transgenic mice (strain 634) on an FVB background (48) and to MMTV-Cre (Cre7 type) on an FVB background (52) obtained from Dr William Muller. The MMTV promoter allows ME targeting of the selected gene. This produced PyMT-MMTV;Cyp27b1flox/flox, PyMT-MMTV;Cyp27b1flox/+, MMTV-Cre;Cyp27b1flox/flox; Cre, and MMTV-Cre;Cyp27b1flox/+. Further crossing produced PyMT-MMTV;Cyp27b1flox/flox;Cre+ (homozygous), PyMT-MMTV;Cyp27b1flox/+;Cre+ (heterozygous), and PyMT-MMTV;Cyp27b1flox/flox;Cre− (control) mice (Supplemental Figure 1).
Cultured cells and treatments
Cells were isolated from spontaneous mammary tumors derived from Cyp27b1-ablated (Cre+) and nonablated (Cre−) animals as follows: tumors were harvested, minced and incubated in DMEM (no fetal bovine serum) containing 2.4-mg/mL collagenase B and dispase II (Roche) at 37°C for 2 hours. Floating cells were washed, pelleted, resuspended, and filtered through a 40-μm sterile nylon mesh strainer. Cells were propagated in complete DMEM with 5% FBS for 2 passages to remove fibroblasts. Cells were tested for reactivity with cytokeratin 8, mT and the Cre protein to confirm their epithelial origin. Cells were stored at −80°C or in liquid nitrogen for later analysis. For proliferation assays, 24-well plates were seeded with 5000 cells/well, incubated for 24 hours then serum starved for 6 hours. After serum starvation, the cells were treated with either 1,25(OH)2D (10−10M to 10−7M) or 25(OH)D (10−9M to 10−7M) for various periods of time, trypsinized, and counted on a Z1 Coulter Counter (Beckman), or used for immunofluorescence (IF) detection. In some tests, Presto Blue (Invitrogen/Life Technologies) was used for viability assays: 10-μL undiluted Presto blue regent was added to individual wells (containing 100-μL DMEM), incubated at 37°C for 2 hours and the change in fluorescence measured with a SPECTRA-max Gemini microplate spectrophotometer (Molecular Devices 5) using 544 nm (excitation) and 590 nm (emission) wavelengths. 1,25(OH)2D and 25(OH)D used for treatment were from Sigma.
Mammary fat pad injections
Cells were isolated from spontaneous mammary tumors derived from Cyp27b1-ablated (Cre+) and nonablated (Cre−) animals, then injected (100 000 cells) into the mammary fat pad of syngeneic FVB virgin mice (2 groups of 10 animals each).
Tumor palpation and excision
Whole-tissue and histology staining
Whole mammary glands were prepared from 3-week old control and CYP27B1flox/flox MMTV-Cre+ female mice according to Ackler et al (53). Glands were spread on a glass slide, fixed in 4% formalin, delipidated and stained with 0.5% Neutral Red (Sigma-Aldrich). Breast tumor tissues were fixed, embedded and stained with hematoxylin-eosin (H and E) using standard protocols. The SK-4100 kit (Victor) was used for immunohistochemistry. Photomicrographs of H and E-stained hyperplasia slides were analyzed with ImageJ software (https://imagej.nih.gov/ij/).
Antibodies for immunohistochemistry and IF
Antibodies used are described in the Appendix.
Measurement of 1,25(OH)2D and 25(OH)D
Tumors were minced and homogenized in 95% ethanol (1:10 wt/vol), centrifuged (10 min at 10 000g), and the supernatant frozen until assay. Blood was collected at killing, serum was separated, aliquoted and frozen until RIAs for 1,25(OH)2D and 25(OH)D as described in Ref. 25. Briefly, extracts and standards were dried in tubes, mixed with 1:1 water:acetonitrile, centrifuged, and the supernatant transferred to a new tube. One sample volume of 12 mmol/L sodium metaperiodate was added and incubated 60 minutes at room temperature. The metaperiodate destroys metabolites with vicinal diols, ie, 1,24,25(OH)3D and 24,25(OH)2D. The 1,25(OH)2D was further purified from remaining metabolites on Bond-Flut C18-OH columns. Calculated assay precision for within and between assay variation was 6% and 16%, respectively, for 25(OH)D assays and 8% and 18% for 1,25(OH)2D assays. The goat anti-25(OH)D antibody was a gift from Dr Bruce Hollis, Department of Pediatrics, Medical University of South Carolina, Charleston, SC. 125I-25(OH)D3 was from Diasorin.
Measurement of calcium levels
Serum calcium was determined with a Synchron 67 autoanalyzer (Beckman). Corrected plasma calcium was calculated using the formula: plasma total calcium + {(40 − plasma albumin)} × 0.02.
NF-κB sequestration analysis
Cultured cells were fixed on slides, stained with anti-NF-κB p65 antibody (Novus) and 4′,6-diamidino-2-phenylindole (DAPI) (Life Technologies) and analyzed with an LSM 510 Meta confocal microscope (Carl Zeiss Microimaging).
Western blot analyses
Proteins were extracted from tissues using standard protocols using protease inhibitor cocktail (Roche). Nuclear protein extracts were prepared with NP-002 Nuclear Protein Extraction kit (ZmTech Scientifique). Samples of 30–50 μg were fractionated by SDS-PAGE electrophoresis, transferred to polyvinylidene difluoride membranes, reacted with primary and secondary antibodies, and developed by enhanced chemiluminescence according to standard methods.
Terminal deoxynucleotidyl transferase 2′-deoxyuridine 5′-diphosphate nick end labeling (TUNEL) assay
TUNEL assay for apoptotic cells was conducted with the In Situ Cell Death Detection kit (Roche Diagnostics) according to the manufacturer's protocol.
Quantitative real-time PCR (qRT-PCR)
Total RNA extraction was performed using the RNAeasy kit (QIAGEN) with on-column deoxyribonuclease treatment. RNA quality was assessed by UV spectrophotometry. First-strand cDNA synthesis was performed on 500-ng total RNA with the RT2 First Strand kit (QIAGEN). The cDNA template was combined with the RT2 Real-Time SYBR Green/Rox master mix and RNAse-free water (SuperArray Bioscience). A final 25-μL reaction volume was added to each well of the mouse NF-κB signaling (PAMM-025Z) RT2 Profiler PCR Array, mouse focal adhesion signaling (PAMM-145Z) array or Janus kinase (JAK)/STAT (PAMM-039Z) arrays from QIAGEN, and amplification was conducted on a 7300 Real-Time PCR system (Applied Biosystems) according to manufacturer's instructions. Housekeeping genes as well as reverse transcription and positive controls were included.
Statistical analysis
All results are expressed as mean ± SE. Statistical comparisons were made using the unpaired Student's t test (P < .05 was considered significant). The statistical difference of tumor onset rate of the animals was determined by Kaplan-Meier analysis. For statistical analysis of tumor progression and tumor growth, numerical data are presented as mean ± SD. Data were analyzed by ANOVA followed by a Bonferri post hoc test to determine the statistical significance of the differences. All statistical analyses were performed using Instat Software (GraphPad Software), and P < .05 was considered statistically significant. Specific tests are mentioned in the text. For qRT-PCR data, the RT2 Profiler PCR Array software package was used, and statistical analyses were performed (n = 3). This package uses ΔΔCT (delta delta threshold cycle)-based fold change calculations and the Student's t test to calculate 2-tail, equal variance P values. P < .05 and a fold change greater than 1.5 were considered to be a significant dysregulation.
Study approval
These animal studies were approved the McGill University Animal Compliance Office. All experiments were carried out in compliance with regulations of the McGill University institutional animal care committee. All animal surgeries were conducted in accordance with principles and procedures dictated by the highest standards of humane animal care.
Results
Cre-mediated ME-targeted ablation of Cyp27b1 gene in PyMT-MMTV mice reduces 1,25(OH)2D concentrations in mammary tumors but does not affect normal mammary development
To clarify the role of vitamin D 1-α-hydroxylase (CYP27B1) in oncogene-driven breast tumorigenesis, we generated PyMT-MMTV mice with a Cre-loxP-mediated (52) homozygous Cyp27b1 gene ablation specifically targeted to the ME (Supplemental Figure 1). These Cyp27b1flox/flox; Cre+ mice are ablated for the vitamin D 1-α-hydroxylase and hereafter designated Cre+. Cyp27b1flox/flox:Cre− (nonablated mice, hereafter designated Cre−) and Cyp27b1wt/wt;Cre+ mice were used as controls to test potential artifactual effects caused by expression of the Cre recombinase but showed no difference from other controls throughout all experiments. In normal mammary glands, CYP27B1 expression was confirmed to colocalize with cytokeratin 8, a marker for mammary epithelial cells (Figure 1A) (54). The Cre+ animals displayed a significant reduction in the level of expression of the 1-α-hydroxylase enzyme (CYP27B1) in normal mammary tissue with respect to Cre− tissue (Figure 1A). Expression is not completely eliminated because Cre-mediated ablation commonly results in a small residual expression of the targeted gene (52, 55). The Cre gene expression is known to be under the control of the MMTV long terminal repeat and its ME-specific expression is detectable starting at 6 days postpartum (Supplemental Figure 2A) (56). Consequently, normal mammary development was observed in Cyp27b1-ablated female mice (Figure 1B) and the mice reproduced and lactated normally.
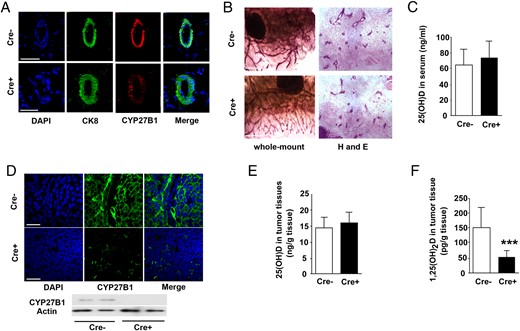
Cre-mediated ME-targeted ablation of the Cyp27b1 gene in PyMT-MMTV mice reduces tumor 1,25(OH)2D concentrations but does not affect normal mammary development. A, IF staining of CYP27B1 in normal ME of PyMT-MMTV mice; control mice (Cre−) (top) and Cyp27b1-ablated mice (Cre+) (bottom). CK8 staining for luminal cells (green), CYP27B1 staining (red), and DAPI (blue). B, Whole-mount staining of mammary glands: neutral red (left) and H and E (right). Cre− (control, top) and Cre+ (Cyp27b1-ablated, bottom) at 4 weeks. C, 25(OH) levels in serum at 11 weeks (Cre− n = 25, Cre+ n = 28, P = .128). D, IF staining of CYP27B1 in mammary tumors at 11 weeks; control mice (Cre−) (top) and Cyp27b1-ablated mice (Cre+) (bottom). Below, Western blotting for CYP27B1 (representative of 6 animals). E, 25(OH) levels in mammary tumors (Cre− n = 25, Cre+ n = 28; P = .136). F, 1,25(OH)2D levels in tumors from control (Cre−) and Cyp27b1-ablated (Cre+) animals (Cre− n = 25, Cre+ n = 28; ***, P < .0001). Scale bars, 50 μm (A and D) and 5 μm (B).
The levels of circulating 25(OH)D in serum were assessed and found to be unaffected by ablation (Figure 1C). Similarly unaffected were the serum calcium levels: average for Cre−, 2.333 ± 0.0667 mmol/L; average for Cre+, 2.416 C 0.0536 mmol/L; average for FVB mice, 2.347 ± 0.007 mmpl/L (P = .4045; n = 49), suggesting that there is no change in circulating 1,25(OH)2D consequent to tumoral Cyp27b1 ablation. Mammary tumors from Cre+ (ablated) animals at 11 weeks displayed a substantial reduction of CYP27B1 protein (Figure 1D). Although tumor 25(OH)D levels were unaffected by ablation, 1,25(OH)2D levels within tumors were decreased by two thirds (Figure 1, E and F). The expression level of the CYP24A1 24-hydroxylase enzyme which degrades biologically active 1,25(OH)2D was not changed in tumor tissues by Cyp27b1 ablation (Supplemental Figure 2B).
These results indicate that ME-targeted ablation of the Cyp27b1 gene greatly reduces the expression of CYP27B1 1-α-hydroxylase in mammary tumors of PyMT-MMTV mice and consequently reduces local tumor 1,25(OH)2D concentrations without affecting circulating 25(OH)D and calcium levels. This substantial decrease in CYP27B1 nevertheless allows normal mammary development and does not affect expression of CYP24.
Ablation of Cyp27b1 gene for 1-α-hydroxylase in PyMT-MMTV mice accelerates hyperplasia appearance
Cyp27b1 ablation significantly accelerated the appearance of spontaneous mammary hyperplasia (Figure 2, A and B). At 3 weeks, none of the Cre− control mice (0/11) presented hyperplasia, in contrast to Cre+ animals (6/11 positive mice). Kaplan-Meier analysis showed that Cre− mice remained tumor-free significantly longer than Cyp27b1-ablated animals (P value for tumor onset = .034) (Figure 2C). Primary mammary tumors appeared earlier and were larger in Cyp27b1-ablated animals than in control mice at the same age (Figure 2D). When tumor cells were isolated from ablated and nonablated mice and injected into the mammary fat pad of FVB mice, xenografts from ablated cells grew significantly faster than xenografts from nonablated controls (Supplemental Figure 3). These results indicate that near-elimination of the 1-α-hydroxylase gene expression in the ME significantly affects tumor latency and accelerates initiation of primary mammary tumorigenesis in PyMT-MMTV mice.
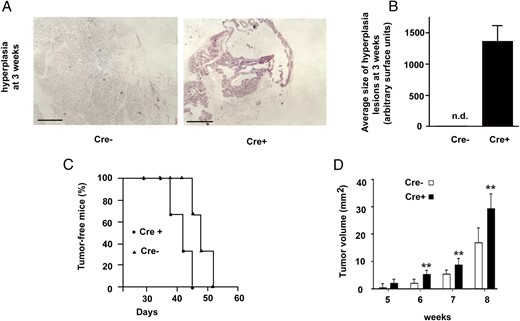
Ablation of Cyp27b1 gene in PyMT-MMTV mice accelerates early stages of mammary tumorigenesis in vivo. A, H and E staining of mammary tissue at 3 weeks in control (Cre−) and Cyp27b1-ablated (Cre+) animals. B, Quantification of hyperplasia surface in control (Cre−) and Cyp27b1-ablated mice (Cre+) (n = 11/group), n.d., not detectable. C, Kaplan-Meier curve (log rank test) for appearance of palpable mammary tumors in control (Cre−, n = 37) or ablated (Cre+, n = 18) mice (P = .034). All (100%) mice are affected. D, Comparison of tumor volumes in control (Cre−) and Cyp27b1-ablated (Cre+) animals at killing; n = 30/group; **, P < .01.
Cyp27b1 ablation modifies apoptosis, cell proliferation, cell cycle, and angiogenesis
Cyp27b1 ablation in ME cells was accompanied by an increase in levels of the apoptotic regulator B-cell lymphoma-2 (Bcl2) and a decrease in apoptotic cell numbers (TUNEL) (Figure 3, A and B). Ablation also caused an increase in cell proliferation marker KI-67 (Kiel-67), cell cycle progression regulator cyclin D1, and angiogenesis markers factor VIII and fms macrophage colony-stimulating factor-related tyrosine kinase (FLT)1 (Figure 3, C–F). These data suggest a general acceleration of cell division events and angiogenesis capacity as well as a reduction in apoptosis in tumor tissues when 1,25(OH)2D local supply is diminished due to tumoral Cyp27b1 ablation.
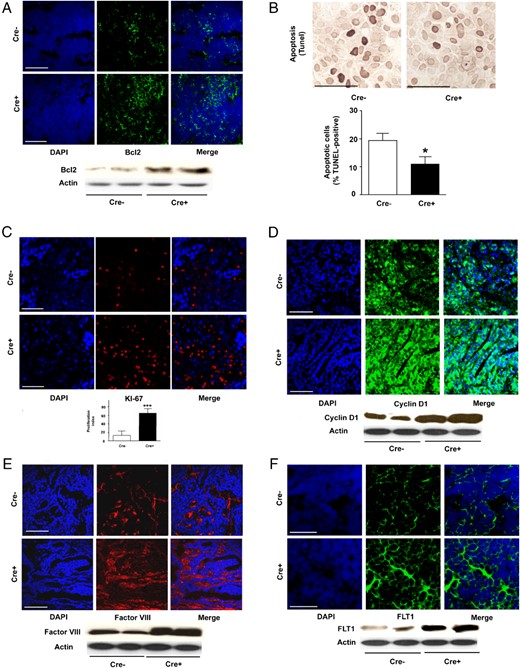
Cyp27b1 ablation in ME modifies apoptosis, proliferation, cell cycle, and angiogenesis markers in mammary tumor tissue. A, IF staining for Bcl2 apoptosis regulator in (below) Western blotting. B, TUNEL staining for apoptosis and quantification histogram (n = 7 in each group; *, P = .045). C, IF staining of KI-67 proliferation marker. Below, Quantification. D, IF staining for cyclin D1 cell division. Below, Western blotting. E, IF staining for factor VIII angiogenesis marker. Below, Western blotting. F, IF staining for FLT1 angiogenesis marker. Below, Western blotting. All Western blottings illustrate a selection from 6 mice. For all, Cre− are control and Cre+ are Cyp27b1-ablated animals. Scale bars, 50 μm (A and C–F) and 200 μm (B).
Cyp27b1 ablation increases tumoral expression of signaling molecules in integrin β4, NF-κB, and STAT3 pathways, as well as phosphorylation of AKT1
Cyp27b1 ablation was accompanied by an increase in the tumoral expression of integrin β4 (Figure 4A). Although protein levels of AK thymoma (AKT) were unaffected by ablation, phosphorylation levels (serine 473) were increased in tumors from ablated mice (Figure 4B). Expression of NF-κB p105/50 and STAT3 also accompanies Cyp27b1 ablation (Figure 4, C and D) although changes in STAT3 protein levels are not reflected in gene expression (see below). To confirm the signaling mechanisms involved downstream of CYP27B1, qRT-PCR analysis of gene expression modulation was conducted for genes related to the integrin/focal adhesion, NF-κB, and JAK/STAT signaling pathways. Profiler PCR array analysis indicates that Cyp27b1 ablation is accompanied by up-regulation of (among others) Bcl2l, Bcl3, Ikbkϵ, Map3k, Raf1, Tnf, and Traf2. Ablation down-regulates Jak1 and Jak2, and Stat1. NFkb p65 levels are unaffected (a list of selected genes appears in Supplemental Table 1, A–C). These results indicate that loss of 1,25(OH)2D production in the ME-derived tumors is accompanied by a significant modulation in the expression or activation of several molecules from the NF-κB, focal adhesion/integrin, and JAK/STAT pathways known as critical control points for oncogenic progression and that the AKT pathway is involved in tumoral vitamin D signaling.
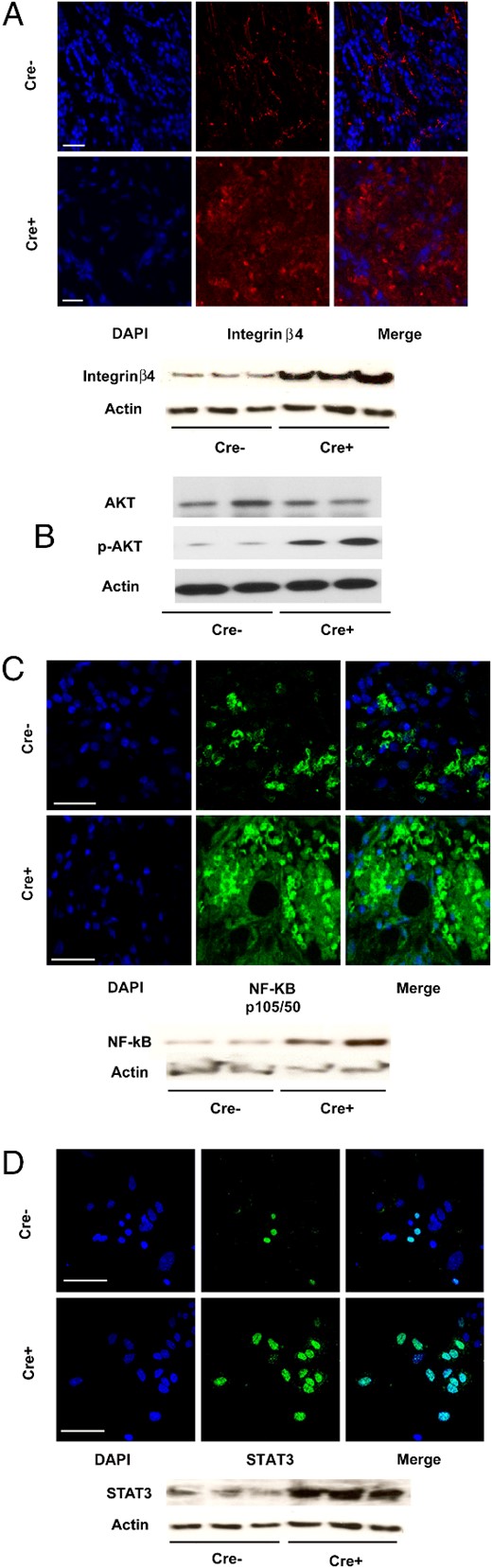
Cyp27b1 ablation increases expression of integrin and NF-κB-related signaling molecules. A, IF staining for integrin β4. Below, Western blotting. B, Western blottings for AKT whole protein, and serine 473 phosphorylation levels in tumor tissue. C, IF staining for NF-κB p105/p50 transcription factor in tumor tissue. Below, Western blotting. D, IF staining for STAT3 transcription factor. Below, Western blotting. All Western blottings illustrate a selection from 6 mice. For all, Cre− are control and Cre+ are Cyp27b1-ablated animals. Scale bars, 50 μm (A, C, and D).
Cyp27b1-ablated tumor cells do not respond to the antiproliferative action of exogenous 25(OH)D prohormone treatment in vitro
In vitro, a 72-hour treatment with 25(OH)D prohormone (10−10M to 10−7M) of isolated cultured control tumor cells (unablated, Cre−) caused up to 30% inhibition in proliferation with respect to untreated Cre− cells (Figure 5A). A similar treatment with the active 1,25(OH)2D resulted in 88% inhibition of proliferation (Figure 5B). In Cre+ cells where Cyp27b1 was ablated, 25(OH)D treatment was ineffective (Figure 5C), whereas 1,25(OH)2D retained inhibitory property (80%) (Figure 5D).
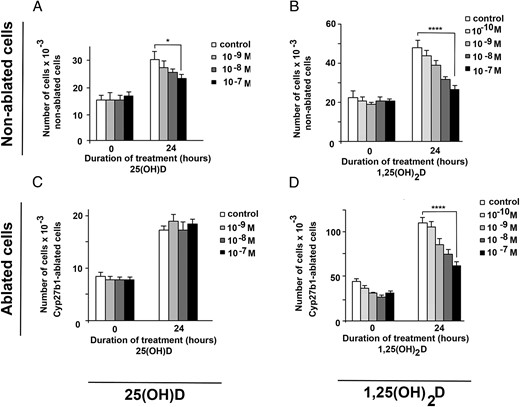
Cyp27b1-ablated cells cannot respond to 25(OH)D growth-inhibition treatment in vitro. In vitro proliferation pattern of nonablated (A and B) and Cyp27b1-ablated tumor cells (C and D) treated for 72 hours with 10−10M to 10−7M 25(OH)D (A and C) or 1,25(OH)2D (B and D). P values at 72 hours: *, P ≤ .05; **, P ≤ .01; ***, P < .001; ****, P < .0001.
NF-κB p65 remains sequestered in the cytoplasm of tumor cells if 1,25(OH)2D is externally provided or locally synthesized
In vitro cultured tumor cells from nonablated (Cre−) and ablated cells (Cre+) that were stained for NF-κB p65 showed substantial nuclear presence of the NF-κB p65 protein at T0 (Figure 6, A–D, top row, white arrows). Treatment (4 or 24 h) with 1,25(OH)2D (10−7M) elicited rapid disappearance of p65 from the nucleus in nonablated and ablated cells (Figure 6, B and D). Prohormone 25(OH)D treatment of nonablated tumor cells in culture caused a similar cytoplasmic sequestration of p65 (Figure 6A and Supplemental Figure 4). However, because prohormone 25(OH)D needs to be activated to 1,25(OH)2D by CYP27B1, 25(OH)D did not cause cytoplasmic sequestration in ablated tumor cells, and p65 remained present in the nucleus of these cells after treatment (Figure 6C, yellow arrows). These data indicate that NF-κB p65 is rapidly sequestered in the cytoplasm of tumor cells if 1,25(OH)2D is externally provided or locally synthesized. If local 25(OH)D activation into 1,25(OH)2D is impossible due to Cyp27b1 ablation, tumor cells retain p65 in their nucleus, allowing gene transcription.
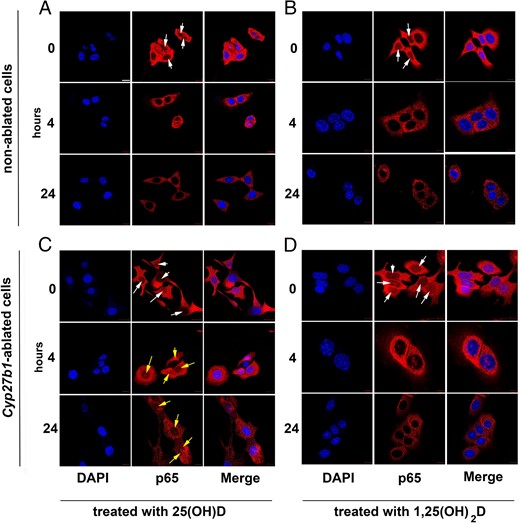
NF-κB p65 is sequestered in the cytoplasm of tumor cells if active 1,25(OH)2D is externally provided or locally synthesized from exogenous 25(OH)D. IF staining pattern (blue, DAPI; red, p65) of nonablated (A and B) and Cyp27b1-ablated (C and D) in vitro cultured tumor cells treated for 0, 4, or 24 hours with 10−7M 25(OH)D (A and C) or 1,25(OH)2D (B and D). White arrows, nuclear p65 at T0. Yellow arrows, remaining nuclear p65 after 25(OH)D treatment. Scale bar, 50 μm.
Discussion
Vitamin D has long been suspected of providing a protective effect against carcinogenesis although epidemiologic studies including metaanalyses on vitamin D benefits in human cancer survival have been inconclusive (16, 57, 58). Nevertheless, breast cancer patients who display higher levels of circulating 25(OH)D appear to have better disease-free survival prediction (59, 60). The fact that the 25(OH)D prohormone requires activation to 1,25(OH)2D by the CYP27B1 α-1-hydroxylase suggests a potentially important role for this enzyme, but the relative antineoplastic importance of renal-derived circulating 1,25(OH)2D vs tumor-synthesized 1,25(OH)2D is unclear. We previously demonstrated the critical role of CYP27B1 in the growth restriction of transformed keratinocytes in vitro and in vivo (61). We now show that tumoral 1-α-hydroxylase is involved in delaying mammary tumor initiation and progression in the MMTV-PyMT mouse, a cancer model that mimics several features of human breast cancer progression (48, 49), and that vitamin D action proceeds at least in part through NF-κB signaling inactivation.
1,25(OH)2D displays a complex pleiotropic regulatory role in cancer cells and induces cell type-specific and stage-specific gene expression changes (21). In the present model of oncogene-driven mammary tumorigenesis, the absence of tumor-synthesized 1,25(OH)2D significantly accelerates the early stages of neoplasia. This agrees with our previous observations that exogenous 25(OH)D and 1,25(OH)2D treatment of MMTV-PyMT mice affects mostly early neoplasia (25), and with the report that vitamin D analog Ro3582 is more effective against early premalignant MCF10AT1 cells than against malignant metastatic MCF10CA1a cells (63). Interestingly, our previous observations also showed 25(OH)D and 1,25(OH)2D treatments of MMTV-PyMT mice as equipotent on tumor growth (25), and indicated that 1,25(OH)2D accumulation in tumor tissues was similar in 25(OH)D and 1,25(OH)2D-treated animals. This is in sharp contrast with the in vitro data of the present study where 1,25(OH)2D has much higher potency on cell growth. The mechanism behind the lower efficacy of 25(OH)D compared with 1,25(OH)2D in nonablated cells (30% [Figure 5A] vs 88% [Figure 5B]) in vitro remains elusive but may be due the relative inefficacy of the extra metabolic step (transformation of 25(OH)D to active 1,25(OH)2D). This inefficient conversion in vitro could also explain the absence of significant 25(OH)D inhibitory action resulting from residual Cyp27B1 in ablated cells (Figure 5C). We speculate that if Cre− cells (where 100% of Cyp27B1 activity is present) are very slow in producing 1,25(OH)2D from exogenous 25(OH)D in culture (Figure 5A), then cells where only a residual amount of enzyme is present will be even less efficient (Figure 5C). Overall, it would appear that the efficacy of the prohormone conversion involves additional mechanisms present only in vivo.
Human cancer biopsies reveal increasing expression of VDR and CYP27B1 in initial tumor development stages, followed by a decline as malignancy sets in (21). Overall, this suggests that vitamin D is better suited to early stage intervention in breast cancer. The expression of CYP24, the enzyme which catabolizes 1,25(OH)2D and a putative oncogene itself (32), is not affected by Cyp27b1 ablation suggesting that CYP27B1 is the main actor in vitamin D action on oncogenic progression in the present system. The observed effects of Cyp27b1 ablation on tumor initiation agree with a local autocrine/intracrine mechanism and strongly support the epidemiological data on the efficacy of vitamin D against breast cancer (64).
Cyp27b1 ablation in ME is accompanied in vivo by increases in expression of several control molecules for cell proliferation, angiogenesis, cell cycle progression and loss of apoptotic capacity (KI-67, factor VIII, Flt1, cyclin D1, and Bcl2), as well as by elevated AKT phosphorylation (prosurvival indicator). Molecules in the NF-κB pathway are particularly affected, with an up-regulation of Raf1, Map3k, as well as a decrease in expression of Crk and IKK (Chuk) which point to Cyp27b1 ablation as prooncogenic. The increase in expression levels of Nfkb2 (encoding P100) in Cyp27b1-ablated tumors suggests that the signaling of the noncanonical NF-κB pathway (42) is also enhanced in absence of tumoral 1,25(OH)D synthesis. Cyp27b1 ablation modulates the expression of key signaling molecules such as Stat3 as well. NF-κB and Stat3 are among the most commonly activated transcriptional factors in cancer and control the expression of antiapoptotic, cell cycle and proliferative genes, and cooperate to facilitate the appearance and progression of several types of cancers (43). Cyp27b1 ablation also causes a change in expression of several integrins, adhesion molecules involved in cell-cell and cell-extracellular matrix interactions. In particular, the increase in expression of integrins α6 and β4 known to possess a procarcinogenic role (65) agrees with the acceleration of tumor initiation observed to accompany Cyp27b1 ablation here.
In addition to the transcriptional consequences of Cyp27b1 ablation, we observed in vitro rapid action of vitamin D treatment on nuclear translocation as a nontranscriptional mechanism. Treatment with 1,25(OH)2D prevents NF-κB p65 migration to the nucleus and confirms results reported for prostate and breast cancer cells in vitro (66, 67). The interesting observation here is that treatment with 25(OH)D also restricts p65 to the cytoplasm except in Cre+ cells where the ablation of CYP27B1 prevents transformation of 25(OH)D into bioactive 1,25(OH)2D, resulting in remaining nuclear presence of p65 that allows gene transcription leading to cell proliferation. This demonstrates a mechanistic link between locally produced 1,25(OH)2D and mammary cancer development, in agreement with the epidemiological data supporting the inverse relationship between circulating 25(OH)D levels and breast cancer occurrence.
Accumulating evidence points to a link between the lack of CYP27B1 activity in tumors and the progression of colon (68), melanoma (69) and pancreatic (62) cancers. We show that for the present system of mammary tumor progression, tumoral presence of the Cyp27b1 gene is crucial to delay very early neoplasia events, as locally produced 1,25(OH)2D restricts tumor growth in an autocrine/intracrine fashion. Locally synthesized vitamin D has both rapid (NF-κB translocation) effects and longer-term (transcriptional) effects on multiple signaling pathways that result in a delay in early events of mammary tumor development. Our work opens the door to therapies aimed at modulating CYP27B1 expression and activity.
Appendix
Peptide/Protein Target . | Antigen Sequence (if Known) . | Name of Antibody . | Manufacturer, Catalog Number, and/or Name of Individual Providing the Antibody . | Species Raised in; Monoclonal or Polyclonal . | Dilution Used . |
---|---|---|---|---|---|
Akt1 | C terminus | Antimouse D17 | Santa Cruz Biotechnology, Inc, SC-7126 | Goat; polyclonal | 1:100 |
Bcl-2 | Anti-M,H D 17C4 | Cell Signaling Technology, 3498 | Rabbit; monoclonal | 1:100 | |
Cre | Antimouse | Abcam, ab40011 | Rabbit; polyclonal | 1:100 | |
cyclin D1 | C terminus | Antimouse C-20 | Santa Cruz Biotechnology, Inc, SC-717 | Rabbit; polyclonal | 1:100 |
CYP24A1 | Antimouse | Abnova, NBP1-85495 | Rabbit; polyclonal | 1:100 | |
CYP27B1 | C internal | Antimouse G-20 | Santa Cruz Biotechnology, Inc, SC-49644 | Goat; polyclonal | 1:100 |
Cytokeratin 8 | Anti-M,H | Progen Biotecnik, GP-K8 | Guinea pig; polyclonal | 1:100 | |
Factor VIII | Anti-D,H,M,P,R | AbBiotech LLC, Q06194 | Rabbit; polyclonal | 1:100 | |
Integrin β4 | Anti-H,M,R | Novus, NBP2-13954 | Rabbit; polyclonal | 1:100 | |
KI-67 | Antimouse M-19 | Santa Cruz Biotechnology, Inc, SC-7846 | Goat; polyclonal | 1:100 | |
NF-κB p105 | Anti-H,M | Novus, NBP1-77395 | Rabbit; polyclonal | 1:100 | |
NF-κB p65 | Anti-H,M,R | Novus, NB 100-2176 | Rabbit; polyclonal | 1:100 | |
mT | N terminus | Antipolyoma virus | Abcam, ab15085 | Rat; monoclonal | 1:100 |
PCNA | Anti-M,R,H, etc | Abcam, PC10 ab29 | Mouse; monoclonal | 1:100 | |
Phospho-Akt1 | Ser473 | Antimouse | Abcam, ab66138 | Rabbit; polyclonal | 1:100 |
STAT3 | Antimouse C-20 | Santa Cruz Biotechnology, Inc, SC-482 | Rabbit; polyclonal | 1:100 | |
VDR | Antimouse C-20 | Santa Cruz Biotechnology, Inc, SC-1008 | Rabbit; polyclonal | 1:100 | |
25(OH)D | Anti-25(OH)D | Gift from Dr Bruce Hollis | Goat; polyclonal | 1:100 | |
IgG | Secondary | Goat antimouse Alexa Fluor 555 conjugated | Invitrogen, A-28180 | Goat; polyclonal | 1:200 |
IgG | Secondary | Goat antirabbit Alexa Fluor 488 conjugated | Invitrogen, A-11034 | Goat; polyclonal | 1:200 |
IgG | Secondary | Rabbit antigoat Alexa Fluor 555 conjugated | Invitrogen, A-21431 | Rabbit; polyclonal | 1:200 |
IgG | Secondary | Rabbit antigoat Alexa Fluor 488 conjugated | Invitrogen, A-11078 | Rabbit; polyclonal | 1:200 |
IgG | Secondary | Donkey antimouse Alexa Fluor 488 conjugated | Abcam, ab150105 | Donkey; polyclonal | 1:200 |
IgG | Secondary | Donkey antirabbit Alexa Fluor 488 conjugated | Abcam, ab150073 | Donkey; polyclonal | 1:200 |
IgG | Secondary | Donkey antigoat Alexa Fluor 488 conjugated | Abcam, ab150129 | Donkey; polyclonal | 1:200 |
Peptide/Protein Target . | Antigen Sequence (if Known) . | Name of Antibody . | Manufacturer, Catalog Number, and/or Name of Individual Providing the Antibody . | Species Raised in; Monoclonal or Polyclonal . | Dilution Used . |
---|---|---|---|---|---|
Akt1 | C terminus | Antimouse D17 | Santa Cruz Biotechnology, Inc, SC-7126 | Goat; polyclonal | 1:100 |
Bcl-2 | Anti-M,H D 17C4 | Cell Signaling Technology, 3498 | Rabbit; monoclonal | 1:100 | |
Cre | Antimouse | Abcam, ab40011 | Rabbit; polyclonal | 1:100 | |
cyclin D1 | C terminus | Antimouse C-20 | Santa Cruz Biotechnology, Inc, SC-717 | Rabbit; polyclonal | 1:100 |
CYP24A1 | Antimouse | Abnova, NBP1-85495 | Rabbit; polyclonal | 1:100 | |
CYP27B1 | C internal | Antimouse G-20 | Santa Cruz Biotechnology, Inc, SC-49644 | Goat; polyclonal | 1:100 |
Cytokeratin 8 | Anti-M,H | Progen Biotecnik, GP-K8 | Guinea pig; polyclonal | 1:100 | |
Factor VIII | Anti-D,H,M,P,R | AbBiotech LLC, Q06194 | Rabbit; polyclonal | 1:100 | |
Integrin β4 | Anti-H,M,R | Novus, NBP2-13954 | Rabbit; polyclonal | 1:100 | |
KI-67 | Antimouse M-19 | Santa Cruz Biotechnology, Inc, SC-7846 | Goat; polyclonal | 1:100 | |
NF-κB p105 | Anti-H,M | Novus, NBP1-77395 | Rabbit; polyclonal | 1:100 | |
NF-κB p65 | Anti-H,M,R | Novus, NB 100-2176 | Rabbit; polyclonal | 1:100 | |
mT | N terminus | Antipolyoma virus | Abcam, ab15085 | Rat; monoclonal | 1:100 |
PCNA | Anti-M,R,H, etc | Abcam, PC10 ab29 | Mouse; monoclonal | 1:100 | |
Phospho-Akt1 | Ser473 | Antimouse | Abcam, ab66138 | Rabbit; polyclonal | 1:100 |
STAT3 | Antimouse C-20 | Santa Cruz Biotechnology, Inc, SC-482 | Rabbit; polyclonal | 1:100 | |
VDR | Antimouse C-20 | Santa Cruz Biotechnology, Inc, SC-1008 | Rabbit; polyclonal | 1:100 | |
25(OH)D | Anti-25(OH)D | Gift from Dr Bruce Hollis | Goat; polyclonal | 1:100 | |
IgG | Secondary | Goat antimouse Alexa Fluor 555 conjugated | Invitrogen, A-28180 | Goat; polyclonal | 1:200 |
IgG | Secondary | Goat antirabbit Alexa Fluor 488 conjugated | Invitrogen, A-11034 | Goat; polyclonal | 1:200 |
IgG | Secondary | Rabbit antigoat Alexa Fluor 555 conjugated | Invitrogen, A-21431 | Rabbit; polyclonal | 1:200 |
IgG | Secondary | Rabbit antigoat Alexa Fluor 488 conjugated | Invitrogen, A-11078 | Rabbit; polyclonal | 1:200 |
IgG | Secondary | Donkey antimouse Alexa Fluor 488 conjugated | Abcam, ab150105 | Donkey; polyclonal | 1:200 |
IgG | Secondary | Donkey antirabbit Alexa Fluor 488 conjugated | Abcam, ab150073 | Donkey; polyclonal | 1:200 |
IgG | Secondary | Donkey antigoat Alexa Fluor 488 conjugated | Abcam, ab150129 | Donkey; polyclonal | 1:200 |
Peptide/Protein Target . | Antigen Sequence (if Known) . | Name of Antibody . | Manufacturer, Catalog Number, and/or Name of Individual Providing the Antibody . | Species Raised in; Monoclonal or Polyclonal . | Dilution Used . |
---|---|---|---|---|---|
Akt1 | C terminus | Antimouse D17 | Santa Cruz Biotechnology, Inc, SC-7126 | Goat; polyclonal | 1:100 |
Bcl-2 | Anti-M,H D 17C4 | Cell Signaling Technology, 3498 | Rabbit; monoclonal | 1:100 | |
Cre | Antimouse | Abcam, ab40011 | Rabbit; polyclonal | 1:100 | |
cyclin D1 | C terminus | Antimouse C-20 | Santa Cruz Biotechnology, Inc, SC-717 | Rabbit; polyclonal | 1:100 |
CYP24A1 | Antimouse | Abnova, NBP1-85495 | Rabbit; polyclonal | 1:100 | |
CYP27B1 | C internal | Antimouse G-20 | Santa Cruz Biotechnology, Inc, SC-49644 | Goat; polyclonal | 1:100 |
Cytokeratin 8 | Anti-M,H | Progen Biotecnik, GP-K8 | Guinea pig; polyclonal | 1:100 | |
Factor VIII | Anti-D,H,M,P,R | AbBiotech LLC, Q06194 | Rabbit; polyclonal | 1:100 | |
Integrin β4 | Anti-H,M,R | Novus, NBP2-13954 | Rabbit; polyclonal | 1:100 | |
KI-67 | Antimouse M-19 | Santa Cruz Biotechnology, Inc, SC-7846 | Goat; polyclonal | 1:100 | |
NF-κB p105 | Anti-H,M | Novus, NBP1-77395 | Rabbit; polyclonal | 1:100 | |
NF-κB p65 | Anti-H,M,R | Novus, NB 100-2176 | Rabbit; polyclonal | 1:100 | |
mT | N terminus | Antipolyoma virus | Abcam, ab15085 | Rat; monoclonal | 1:100 |
PCNA | Anti-M,R,H, etc | Abcam, PC10 ab29 | Mouse; monoclonal | 1:100 | |
Phospho-Akt1 | Ser473 | Antimouse | Abcam, ab66138 | Rabbit; polyclonal | 1:100 |
STAT3 | Antimouse C-20 | Santa Cruz Biotechnology, Inc, SC-482 | Rabbit; polyclonal | 1:100 | |
VDR | Antimouse C-20 | Santa Cruz Biotechnology, Inc, SC-1008 | Rabbit; polyclonal | 1:100 | |
25(OH)D | Anti-25(OH)D | Gift from Dr Bruce Hollis | Goat; polyclonal | 1:100 | |
IgG | Secondary | Goat antimouse Alexa Fluor 555 conjugated | Invitrogen, A-28180 | Goat; polyclonal | 1:200 |
IgG | Secondary | Goat antirabbit Alexa Fluor 488 conjugated | Invitrogen, A-11034 | Goat; polyclonal | 1:200 |
IgG | Secondary | Rabbit antigoat Alexa Fluor 555 conjugated | Invitrogen, A-21431 | Rabbit; polyclonal | 1:200 |
IgG | Secondary | Rabbit antigoat Alexa Fluor 488 conjugated | Invitrogen, A-11078 | Rabbit; polyclonal | 1:200 |
IgG | Secondary | Donkey antimouse Alexa Fluor 488 conjugated | Abcam, ab150105 | Donkey; polyclonal | 1:200 |
IgG | Secondary | Donkey antirabbit Alexa Fluor 488 conjugated | Abcam, ab150073 | Donkey; polyclonal | 1:200 |
IgG | Secondary | Donkey antigoat Alexa Fluor 488 conjugated | Abcam, ab150129 | Donkey; polyclonal | 1:200 |
Peptide/Protein Target . | Antigen Sequence (if Known) . | Name of Antibody . | Manufacturer, Catalog Number, and/or Name of Individual Providing the Antibody . | Species Raised in; Monoclonal or Polyclonal . | Dilution Used . |
---|---|---|---|---|---|
Akt1 | C terminus | Antimouse D17 | Santa Cruz Biotechnology, Inc, SC-7126 | Goat; polyclonal | 1:100 |
Bcl-2 | Anti-M,H D 17C4 | Cell Signaling Technology, 3498 | Rabbit; monoclonal | 1:100 | |
Cre | Antimouse | Abcam, ab40011 | Rabbit; polyclonal | 1:100 | |
cyclin D1 | C terminus | Antimouse C-20 | Santa Cruz Biotechnology, Inc, SC-717 | Rabbit; polyclonal | 1:100 |
CYP24A1 | Antimouse | Abnova, NBP1-85495 | Rabbit; polyclonal | 1:100 | |
CYP27B1 | C internal | Antimouse G-20 | Santa Cruz Biotechnology, Inc, SC-49644 | Goat; polyclonal | 1:100 |
Cytokeratin 8 | Anti-M,H | Progen Biotecnik, GP-K8 | Guinea pig; polyclonal | 1:100 | |
Factor VIII | Anti-D,H,M,P,R | AbBiotech LLC, Q06194 | Rabbit; polyclonal | 1:100 | |
Integrin β4 | Anti-H,M,R | Novus, NBP2-13954 | Rabbit; polyclonal | 1:100 | |
KI-67 | Antimouse M-19 | Santa Cruz Biotechnology, Inc, SC-7846 | Goat; polyclonal | 1:100 | |
NF-κB p105 | Anti-H,M | Novus, NBP1-77395 | Rabbit; polyclonal | 1:100 | |
NF-κB p65 | Anti-H,M,R | Novus, NB 100-2176 | Rabbit; polyclonal | 1:100 | |
mT | N terminus | Antipolyoma virus | Abcam, ab15085 | Rat; monoclonal | 1:100 |
PCNA | Anti-M,R,H, etc | Abcam, PC10 ab29 | Mouse; monoclonal | 1:100 | |
Phospho-Akt1 | Ser473 | Antimouse | Abcam, ab66138 | Rabbit; polyclonal | 1:100 |
STAT3 | Antimouse C-20 | Santa Cruz Biotechnology, Inc, SC-482 | Rabbit; polyclonal | 1:100 | |
VDR | Antimouse C-20 | Santa Cruz Biotechnology, Inc, SC-1008 | Rabbit; polyclonal | 1:100 | |
25(OH)D | Anti-25(OH)D | Gift from Dr Bruce Hollis | Goat; polyclonal | 1:100 | |
IgG | Secondary | Goat antimouse Alexa Fluor 555 conjugated | Invitrogen, A-28180 | Goat; polyclonal | 1:200 |
IgG | Secondary | Goat antirabbit Alexa Fluor 488 conjugated | Invitrogen, A-11034 | Goat; polyclonal | 1:200 |
IgG | Secondary | Rabbit antigoat Alexa Fluor 555 conjugated | Invitrogen, A-21431 | Rabbit; polyclonal | 1:200 |
IgG | Secondary | Rabbit antigoat Alexa Fluor 488 conjugated | Invitrogen, A-11078 | Rabbit; polyclonal | 1:200 |
IgG | Secondary | Donkey antimouse Alexa Fluor 488 conjugated | Abcam, ab150105 | Donkey; polyclonal | 1:200 |
IgG | Secondary | Donkey antirabbit Alexa Fluor 488 conjugated | Abcam, ab150073 | Donkey; polyclonal | 1:200 |
IgG | Secondary | Donkey antigoat Alexa Fluor 488 conjugated | Abcam, ab150129 | Donkey; polyclonal | 1:200 |
Acknowledgments
This work was supported by the Canadian Institute for Health Research Grant MOP 10839 and by the Susan G. Komen Foundation Grant KG 100766.
Disclosure Summary: The authors have nothing to disclose.
Abbreviations
- AKT
AK thymoma
- Bcl2
B-cell lymphoma-2
- CYP
cytochrome P450
- DAPI
4′,6-diamidino-2-phenylindole
- FLT
fms macrophage colony-stimulating factor-related tyrosine kinase
- FVB
friend virus B-type mouse
- H and E
hematoxylin-eosin
- IF
immunofluorescence
- IkB
inhibitor of kappa B kinase
- JAK
Janus kinase
- KI-67
Kiel-67
- ME
mammary epithelium
- MMTV
mouse mammary tumor virus
- NF-κB
nuclear factor κB
- 25(OH)D
25-hydroxycholecalciferol
- 1,25(OH)2D
1,25-dihydroxycholecalciferol
- PyMT
polyoma middle T antigen
- qRT-PCR
quantitative real-time PCR
- STAT3
signal transducer and activator of transcription 3
- TUNEL
terminal deoxynucleotidyl transferase 2′-deoxyuridine 5′-diphosphate nick end labeling
- VDR
vitamin D receptor.