-
PDF
- Split View
-
Views
-
Cite
Cite
Han Zhang, Christian Klausen, Hua Zhu, Hsun-Ming Chang, Peter C. K. Leung, BMP4 and BMP7 Suppress StAR and Progesterone Production via ALK3 and SMAD1/5/8-SMAD4 in Human Granulosa-Lutein Cells, Endocrinology, Volume 156, Issue 11, 1 November 2015, Pages 4269–4280, https://doi.org/10.1210/en.2015-1494
- Share Icon Share
Adequate production of progesterone by the corpus luteum is critical to the successful establishment of pregnancy. In animal models, bone morphogenetic protein (BMP) 4 and BMP7 have been shown to suppress either basal or gonadotropin-induced progesterone production, depending on the species examined. However, the effects of BMP4 and BMP7 on progesterone production in human granulosa cells are unknown. In the present study, we used immortalized (SVOG) and primary human granulosa-lutein cells to investigate the effects of BMP4 and BMP7 on steroidogenic acute regulatory protein (StAR) expression and progesterone production and to examine the underlying molecular mechanism. Treatment of primary and immortalized human granulosa cells with recombinant BMP4 or BMP7 decreased StAR expression and progesterone accumulation. In SVOG cells, the suppressive effects of BMP4 and BMP7 on StAR expression were blocked by pretreatment with inhibitors of activin receptor-like kinase (ALK)2/3/6 (dorsomorphin) or ALK2/3 (DMH1) but not ALK4/5/7 (SB-431542). Moreover, small interfering RNA-mediated depletion of ALK3, but not ALK2 or ALK6, reversed the effects of BMP4 and BMP7 on StAR expression. Likewise, BMP4- and BMP7-induced phosphorylation of SMAD 1/5/8 was reversed by treatment with DMH1 or small interfering RNA targeting ALK3. Knockdown of SMAD4, the essential common SMAD for BMP/TGF-β signaling, abolished the effects of BMP4 and BMP7 on StAR expression. Our results suggest that BMP4 and BMP7 down-regulate StAR and progesterone production via ALK3 and SMAD1/5/8-SMAD4 signaling in human granulosa-lutein cells.
Luteinization, the process whereby granulosa cells and theca cells differentiate into luteal cells, is regulated by LH. The main function of luteal cells is steroid hormone secretion, especially progesterone, to maintain pregnancy or regulate the estrus cycle. Progesterone is derived from cholesterol, which is mainly obtained from blood as low-density lipoprotein and transferred into cells by receptor-mediated endocytosis (1). Steroidogenic acute regulatory protein (StAR) governs the process by which cholesterol is transported from the outer to the inner mitochondrial membrane, in which cytochrome P450 side-chain cleavage enzyme (P450scc) catalyzes the first step in progesterone synthesis (2, 3). After its conversion from cholesterol by mitochondrial P450scc, pregnenolone is then converted to progesterone by 3β-hydroxysteroid dehydrogenase in the smooth endoplasmic reticulum (4). After ovulation, increasing theca cell vascularization brings more cholesterol to luteinized granulosa cells and high LH levels induce low-density lipoprotein receptor, StAR, P450scc and 3β-hydroxysteroid dehydrogenase expression, all of which result in elevated progesterone production to maintain corpus luteum function (5–8). Throughout this process, growth factors from oocyte, theca and/or granulosa cells exert important paracrine/autocrine regulatory roles on luteinization and progesterone production.
Bone morphogenetic proteins (BMPs) belong to the TGF-β superfamily. Like other TGF-β superfamily members, such as activins, inhibins, growth differentiation factors, and anti-Müllerian hormone, BMPs exert their functions by binding type I and type II receptors, which regulate downstream gene expression by phosphorylating SMAD transcription factors (9). The human genome encodes seven type I receptors known as activin receptor-like kinases (ALK1–7). BMPs are thought to bind ALK2/3/6 leading to the phosphorylation of SMAD1/5/8, whereas TGF-β superfamily members that bind ALK4/5/7 generally phosphorylate SMAD2/3 (10). BMPs and their receptors are well known to be expressed in the reproductive system and to exert important roles in steroidogenesis (11, 12). In particular, BMP4 and BMP7 have been detected in theca/stromal cells in both rat and human ovaries (11, 13). BMP4 was found to suppress both basal and FSH-stimulated progesterone production in cow granulosa cells and to down-regulate FSH-induced StAR and P450scc in sheep granulosa cells (14, 15). Interestingly, BMP4 and BMP7 suppressed FSH-induced, but not basal, progesterone production in rat granulosa cells (12, 16). Although accumulating evidence from animal studies suggest that BMP4 and BMP7 can regulate progesterone production, their roles in human granulosa cell steroidogenesis are poorly understood, and the molecular mediators involved have yet to be defined in any species. Therefore, in this study, we examined the effects of recombinant BMP4 and BMP7 on progesterone biosynthesis and investigated the underlying mechanisms in human granulosa-lutein cells.
Materials and Methods
Culture of primary and immortalized human granulosa-lutein cells
Primary human granulosa-lutein cells were obtained with informed patient consent after the approval from the University of British Columbia Research Ethics Board. Follicular aspirates were obtained from nine women undergoing in vitro fertilization, and the controlled ovarian stimulation protocol was as previously described (17). Granulosa cells were purified by density centrifugation from follicular aspirates as previously described (18). Briefly, each patient’s follicular aspirate was centrifuged at 400 × g for 20 minutes, and the cell pellet was resuspended in 4 mL phenol-red free DMEM/F12 medium (Invitrogen, Life Technologies) containing 10% charcoal/dextran-treated fetal bovine serum (Hyclone, GE Healthcare Life Sciences), 1× antibiotic-antimycotic (Gibco, Life Technologies), and 1× Gluta MAX (Gibco, Life Technologies). Cell suspensions were layered on 8 mL Ficoll-Paque Plus (GE Healthcare Life Sciences) and centrifuged at 600 × g for 20 minutes. The granulosa-lutein cell layer was removed from the Ficoll-Paque column, washed with medium, and resuspended in 5 mL medium. The nontumorigenic immortalized human granulosa cell line (SVOG) was previously produced by transfecting human granulosa-lutein cells with the Simian virus 40 large T antigen (19). These cells retain much of their steroidogenic capacity, including basal and cAMP- or human chorionic gonadotropin-induced progesterone production (19); however, they have reduced levels of FSH receptor and aromatase (20). SVOG cells were passaged using trypsin-EDTA solution (0.05% trypsin, 0.5 mM EDTA; Gibco, Life Technologies) when they reached 90% confluence. All cells were counted with a hemocytometer and cell viability was assessed by Trypan blue (0.04%) exclusion. Primary granulosa cells were seeded (1–2 × 105 per well) in 12-well plates, whereas SVOG cells were seeded (2–4 × 105 per well) in six-well plates. Unless otherwise indicated, cells were precultured for 24 hours and then maintained in serum-free medium for 24 hours prior to treatment. Culture medium was changed every other day in all experiments, and the cells were cultured in a humidified atmosphere containing 5% CO2–95% air at 37°C.
Antibodies and reagents
Detailed information for all primary antibodies is listed in Table 1. Horseradish peroxidase-conjugated goat antirabbit and goat antimouse IgG were obtained from Bio-Rad Laboratories. Recombinant human BMP4, recombinant human BMP7, dorsomorphin dihydrochloride, and DMH1 were obtained from R&D Systems. SB-431542 was obtained from Sigma.
Peptide/Protein Target . | Antigen Sequence (If Known) . | Name of Antibody . | Manufacturer, Catalog Number, and/or Name of Individual Providing the Antibody . | Species Raised (Monoclonal or Polyclonal) . | Dilution Used . |
---|---|---|---|---|---|
StAR | Amino acids 1-285 representing full-length StAR of human origin | StAR antibody (FL-285) | Santa Cruz Biotechnology, sc-25806 | Rabbit polyclonal | 1:1000 |
SMAD1/5/8 | A peptide mappin to N terminus of SMAD1 of human origin | Smad1/5/8 (N-18)-R | Santa Cruz Biotechnology, sc-6031-R | Rabbit polyclonal | 1:1000 |
α-Tubulin | Sarkosyl-resistant ribbons from sperm axonemes of sea urchin orgin | α-Tubulin (B-5-1-2) | Santa Cruz Biotechnology, sc-23948 | Mouse monoclonal | 1:2000 |
Phospho-SMAD1/5/8 | Synthetic phosphopeptide corresponding to residues surrounding Ser463/465 of human SMAD5 | Phospho-Smad1 (Ser463/465)/Smad5 (Ser463/465)/Smad9 (Ser465/467) | Cell Signaling Technology, number 9511 | Rabbit polyclonal | 1:1000 |
SMAD4 | A synthetic peptide corresponding to the residues surrounding Pro278 of human SMAd4 | Smad4 antibody | Cell Signaling Technology, number 9515 | Rabbit polyclonal | 1:1000 |
Peptide/Protein Target . | Antigen Sequence (If Known) . | Name of Antibody . | Manufacturer, Catalog Number, and/or Name of Individual Providing the Antibody . | Species Raised (Monoclonal or Polyclonal) . | Dilution Used . |
---|---|---|---|---|---|
StAR | Amino acids 1-285 representing full-length StAR of human origin | StAR antibody (FL-285) | Santa Cruz Biotechnology, sc-25806 | Rabbit polyclonal | 1:1000 |
SMAD1/5/8 | A peptide mappin to N terminus of SMAD1 of human origin | Smad1/5/8 (N-18)-R | Santa Cruz Biotechnology, sc-6031-R | Rabbit polyclonal | 1:1000 |
α-Tubulin | Sarkosyl-resistant ribbons from sperm axonemes of sea urchin orgin | α-Tubulin (B-5-1-2) | Santa Cruz Biotechnology, sc-23948 | Mouse monoclonal | 1:2000 |
Phospho-SMAD1/5/8 | Synthetic phosphopeptide corresponding to residues surrounding Ser463/465 of human SMAD5 | Phospho-Smad1 (Ser463/465)/Smad5 (Ser463/465)/Smad9 (Ser465/467) | Cell Signaling Technology, number 9511 | Rabbit polyclonal | 1:1000 |
SMAD4 | A synthetic peptide corresponding to the residues surrounding Pro278 of human SMAd4 | Smad4 antibody | Cell Signaling Technology, number 9515 | Rabbit polyclonal | 1:1000 |
Peptide/Protein Target . | Antigen Sequence (If Known) . | Name of Antibody . | Manufacturer, Catalog Number, and/or Name of Individual Providing the Antibody . | Species Raised (Monoclonal or Polyclonal) . | Dilution Used . |
---|---|---|---|---|---|
StAR | Amino acids 1-285 representing full-length StAR of human origin | StAR antibody (FL-285) | Santa Cruz Biotechnology, sc-25806 | Rabbit polyclonal | 1:1000 |
SMAD1/5/8 | A peptide mappin to N terminus of SMAD1 of human origin | Smad1/5/8 (N-18)-R | Santa Cruz Biotechnology, sc-6031-R | Rabbit polyclonal | 1:1000 |
α-Tubulin | Sarkosyl-resistant ribbons from sperm axonemes of sea urchin orgin | α-Tubulin (B-5-1-2) | Santa Cruz Biotechnology, sc-23948 | Mouse monoclonal | 1:2000 |
Phospho-SMAD1/5/8 | Synthetic phosphopeptide corresponding to residues surrounding Ser463/465 of human SMAD5 | Phospho-Smad1 (Ser463/465)/Smad5 (Ser463/465)/Smad9 (Ser465/467) | Cell Signaling Technology, number 9511 | Rabbit polyclonal | 1:1000 |
SMAD4 | A synthetic peptide corresponding to the residues surrounding Pro278 of human SMAd4 | Smad4 antibody | Cell Signaling Technology, number 9515 | Rabbit polyclonal | 1:1000 |
Peptide/Protein Target . | Antigen Sequence (If Known) . | Name of Antibody . | Manufacturer, Catalog Number, and/or Name of Individual Providing the Antibody . | Species Raised (Monoclonal or Polyclonal) . | Dilution Used . |
---|---|---|---|---|---|
StAR | Amino acids 1-285 representing full-length StAR of human origin | StAR antibody (FL-285) | Santa Cruz Biotechnology, sc-25806 | Rabbit polyclonal | 1:1000 |
SMAD1/5/8 | A peptide mappin to N terminus of SMAD1 of human origin | Smad1/5/8 (N-18)-R | Santa Cruz Biotechnology, sc-6031-R | Rabbit polyclonal | 1:1000 |
α-Tubulin | Sarkosyl-resistant ribbons from sperm axonemes of sea urchin orgin | α-Tubulin (B-5-1-2) | Santa Cruz Biotechnology, sc-23948 | Mouse monoclonal | 1:2000 |
Phospho-SMAD1/5/8 | Synthetic phosphopeptide corresponding to residues surrounding Ser463/465 of human SMAD5 | Phospho-Smad1 (Ser463/465)/Smad5 (Ser463/465)/Smad9 (Ser465/467) | Cell Signaling Technology, number 9511 | Rabbit polyclonal | 1:1000 |
SMAD4 | A synthetic peptide corresponding to the residues surrounding Pro278 of human SMAd4 | Smad4 antibody | Cell Signaling Technology, number 9515 | Rabbit polyclonal | 1:1000 |
Reverse transcription-quantitative real-time PCR (RT-qPCR)
Total RNA was extracted with TRIzol reagent (Invitrogen, Life Technologies), and 2 μg was reverse transcribed into first-strand cDNA with random primers and Moloney murine leukemia virus reverse transcriptase (Promega). SYBR Green or TaqMan RT-qPCR was performed on an Applied Biosystems 7300 Real-Time PCR system equipped with 96-well optical reaction plates. Each 20-μl SYBR Green RT-qPCR reaction contained 1× SYBR Green PCR master mix (Applied Biosystems), 20 ng cDNA, and 250 nM of each specific primer. The primers used were as follows: STAR (efficiency [E] = 108%), 5′-AAA CTT ACG TGG CTA CTC AGC ATC-3′ (sense) and 5′-GAC CTG GTT GAT GCT CTTG-3′ (antisense); SMAD4 (SMAD family member 4; E = 112%), 5′-TGG CCC AGG ATC ACC AGA AG-3′ (sense) and 5′-CAT CAA CAC CAA TTC CAG CA-3′ (antisense); steroidogenic factor 1 (SF-1; E = 105%), 5′-CAG CCT GGA TTT GAA GTT TCC-3′ (sense) and 5′-TTC GAT GAG CAG GTT GC-3′ (antisense); and glyceraldehyde-3-phosphate dehydrogenase (GAPDH; E = 111%), 5′-GAG TCA ACG GAT TTG GTC GT-3′ (sense) and 5′-GAC AAG CTT CCC GTT CTC AG-3′ (antisense). The specificity of each assay was validated by dissociation curve analysis and agarose gel electrophoresis of PCR products. Alternatively, FAM-MGB TaqMan gene expression assays for ACVR1 (ALK2; E = 100%; Hs00153836_m1), BMPR1A (ALK3; E = 100%; Hs01034913_g1), BMPR1B (ALK6; E = 101%; Hs00176144_m1), and GAPDH (E = 97%; Hs02758991_g1) were performed on corresponding cDNA samples. Each 20-μL TaqMan RT-qPCR reaction contained 1 × TaqMan gene expression master mix (Applied Biosystems), 1× specific TaqMan gene expression assay (Applied Biosystems), and 20 ng cDNA. The performance of each SYBR Green or TaqMan assay was validated by evaluating amplification efficiencies by means of calibration curves and ensuring that the plot of log input amount vs ΔCq has a slope with an absolute value less than 0.1. Three separate experiments were performed on different cultures and each sample was assayed in triplicate. A mean value was used for the determination of mRNA levels by the comparative Cq method (2—ΔΔCq) with GAPDH as the reference.
Western blot analysis
Cells were seeded, treated as described, and lysed with cell lysis buffer (Cell Signaling Technology) containing protease inhibitor cocktail (Sigma-Aldrich). Extracts were centrifuged at 20 000 × g for 10 minutes at 4°C to remove cellular debris, and protein concentrations were quantified using the DC protein assay (Bio-Rad Laboratories). Equal amounts of protein (50 μg) were separated by 10% SDS-PAGE and transferred to polyvinylidene fluoride membranes. After blocking for 1 hour with 5% nonfat dried milk in Tris-buffered saline containing 0.1% Tween 20 (TBST), membranes were incubated overnight at 4°C with primary antibodies diluted 1:1000 in TBST with 5% nonfat dried milk. After washing, membranes were incubated for 1 hour with appropriate peroxidase-conjugated secondary antibody diluted 1:5000 in TBST with 5% nonfat dried milk. Immunoreactive bands were detected using an enhanced chemiluminescence reagent or SuperSignal West Femto chemiluminescence substrate (Pierce, Thermo Scientific), followed by exposure to CL-XPosure film (Thermo Scientific). Membranes were stripped with stripping buffer (50 mM Tris-HCl, pH 7.6; 10 mmol/L β-mercaptoethanol; and 1% sodium dodecyl sulfate) at 50°C for 30 minutes and reprobed with anti-α-tubulin or anti-SMAD1/5/8 as a loading control. Immunoreactive band intensities were quantified by densitometry (Scion Image Software, Scion Corp), target levels were normalized to those of the relevant loading control, and the results are expressed as fold change relative to the respective control.
Small interfering RNA (siRNA) transfection
To knock down endogenous ALK2, ALK3, ALK6, or SMAD4, cells were seeded (0.5–1 × 105 per well in six well plates) and transfected the day after seeding with 25 nM ON-TARGETplus SMARTpool siRNAs targeting human ALK2, ALK3, ALK6 or SMAD4 (Dharmacon, GE Healthcare Life Sciences) using LipofectamineRNA iMAX (Invitrogen, Life Technologies) according to the manufacturer’s instructions. ON-TARGETplus nontargeting control pool siRNA (25 nM) was used as a transfection control in all experiments. Knockdown efficiency was confirmed by RT-qPCR (ALK2, ALK3, ALK6, and SMAD4) and Western blot (SMAD4).
Measurement of progesterone
Cells were seeded and treated as described, and conditioned medium was stored at −80°C until assayed. Progesterone levels in conditioned medium were measured as per the manufacturer’s instructions using a competitive enzyme immunoassay kit (Cayman Chemical). The intra- and interassay coefficients of variation were 6.8% and 9.7%, respectively, and the detection limit was 0.01 ng/mL. All samples were measured in triplicate and normalized to total cellular protein content.
Statistical analysis
Results are presented as the mean ± SEM of at least three independent experiments performed on separate cultures. PRISM software (GraphPad Software Inc) was used to perform a one-way ANOVA followed by a Tukey’s test for multiple comparisons of means. Means were considered statistically different at a value of P < .05 and are indicated by different letters.
Results
BMP4 and BMP7 suppress StAR expression in human granulosa cells
To examine the effects of BMP4 and BMP7 on granulosa cell steroidogenesis, we first used RT-qPCR and Western blot to examine their effects on the expression of StAR, which regulates the rate-limiting step in steroid biosynthesis. StAR mRNA and protein levels were measured in SVOG immortalized human granulosa cells after treatment with vehicle control or 30 ng/mL recombinant human BMP4 or BMP7 for 3, 6, 12, or 24 hours. The RT-qPCR results show that treatment with either BMP4 (Figure 1A) or BMP7 (Figure 1B) down-regulated StAR mRNA levels after 12 and 24 hours. The suppressive effects of BMP4 and BMP7 on StAR protein levels were further confirmed by Western blot analysis (Figure 1, C and D). To evaluate the concentration-dependent effects of BMP4 and BMP7 on StAR expression, SVOG cells were treated for 24 hours with varying concentrations of each protein (1, 10, 30, or 100 ng/mL), and StAR mRNA and protein levels were measured by RT-qPCR and Western blot, respectively. Treatment with either BMP4 or BMP7 down-regulated StAR mRNA (Figure 2, A and B) and protein (Figure 2, C and D) levels in a concentration-dependent manner. Interestingly, treatment with 1 ng/mL BMP4 induced significant decreases in StAR expression, whereas only concentrations of 30 or 100 ng/mL of BMP7 yielded significant effects at both the mRNA and protein level.
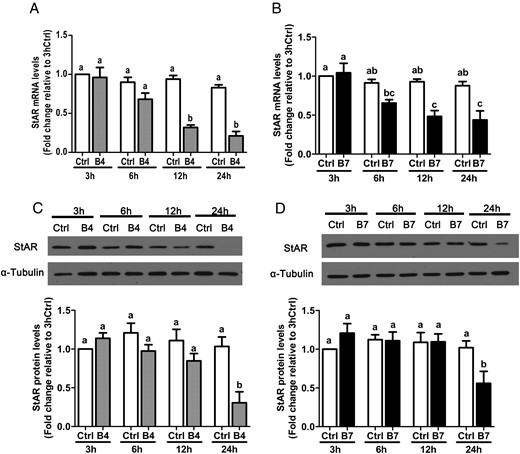
Time-dependent effects of BMP4 and BMP7 on StAR expression in SVOG immortalized human granulosa cells. Cells were treated with vehicle control (Ctrl) or 30 ng/mL BMP4 (B4; A and C) or BMP7 (B7; B and D) for 3, 6, 12, or 24 hours, and StAR mRNA (A and B) and protein (C and D) levels were examined by RT-qPCR and Western blot, respectively. Results are expressed as the mean ± SEM of at least three independent experiments and values without common letters are significantly different (P < .05).
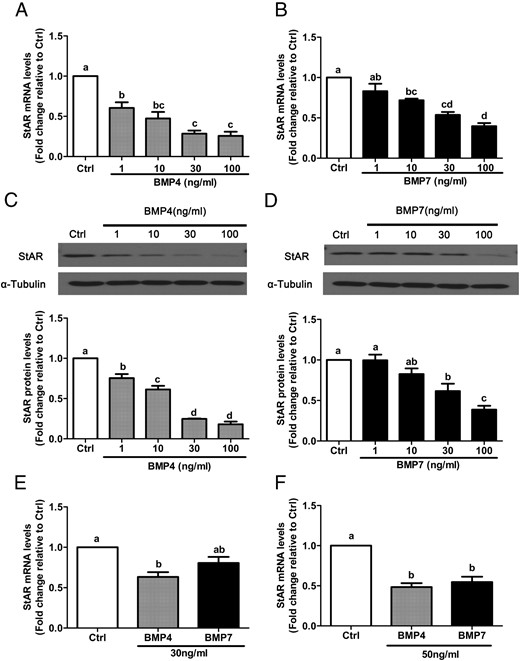
BMP4 and BMP7 down-regulate StAR expression in a concentration-dependent manner in primary and immortalized human granulosa cells. A–D, SVOG immortalized human granulosa cells were treated for 24 hours with vehicle control (Ctrl) or increasing concentrations (1, 10, 30, or100 ng/mL) of BMP4 (A and C) or BMP7 (B and D), and StAR mRNA (A and B) and protein (C and D) levels were examined by RT-qPCR and Western blot, respectively. E and F, Primary human granulosa cells were treated for 24 hours with 30 ng/mL (E) or 50 ng/mL (F) BMP4 or BMP7, and StAR mRNA levels were examined by RT-qPCR. Results are expressed as the mean ± SEM of at least three independent experiments, and values without common letters are significantly different (P < .05).
To further confirm the suppressive effects of BMP4 and BMP7 on StAR expression, primary human granulosa-lutein cells were treated for 24 hours with 30 or 50 ng/mL BMP4 or BMP7. As shown in Figure 2E, treatment with 30 ng/mL BMP4 significantly reduced StAR mRNA levels, whereas treatment with BMP7 yielded reductions approaching statistical significance. However, when the treatment concentrations were increased to 50 ng/mL, both BMP4 and BMP7 significantly reduced StAR mRNA levels (Figure 2F).
BMP4 and BMP7 decrease progesterone production in human granulosa cells
Next, the effects of BMP4 and BMP7 on granulosa cell progesterone production were examined in SVOG cells treated for 24 hours with vehicle control or increasing concentrations (1, 10, 30, or 100 ng/mL) of BMP4 or BMP7. The mean level of progesterone in conditioned medium from control SVOG cells was 3.6 ± 0.6 ng/mL. Treatment with either BMP4 (Figure 3A) or BMP7 (Figure 3B) down-regulated progesterone accumulation in conditioned medium in a concentration-dependent manner. Consistent with the findings for StAR expression, BMP4 induced significant decreases at all concentrations tested, whereas only treatment with 30 or 100 ng/mL BMP7 yielded significant effects on progesterone production. Next, we investigated the effects of BMP4 and BMP7 on progesterone production in primary human granulosa-lutein cells. The mean level of progesterone in conditioned medium from control primary human granulosa-lutein cells was 297 ± 228 ng/mL. As shown in Figure 3C, treatment for 24 hours with 50 ng/mL BMP4 or BMP7 significantly decreased progesterone accumulation in conditioned medium.
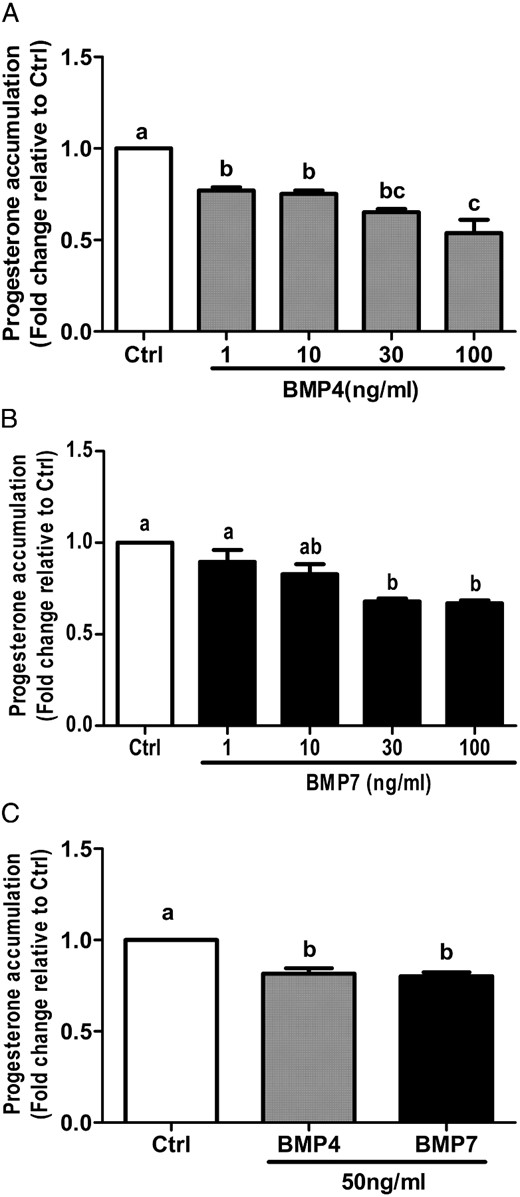
BMP4 and BMP7 suppress progesterone accumulation in primary and immortalized human granulosa cells. A and B, SVOG immortalized human granulosa cells were treated for 24 hours with vehicle control (Ctrl) or increasing concentrations (1, 10, 30, or 100 ng/mL) of BMP4 (A) or BMP7 (B), and progesterone accumulation in conditioned medium was measured by an ELISA. C, Primary human granulosa cells were treated with 50 ng/mL BMP4 or BMP7 for 24 hours, and progesterone accumulation was measured by ELISA. Results are expressed as the mean ± SEM of at least three independent experiments and values without common letters are significantly different (P < .05).
BMP4 and BMP7 down-regulate StAR via ALK3 in SVOG cells
Although both type I and type II receptors are required for BMP signaling, signaling specificity is primarily determined by BMP type I receptors (ALK2, ALK3, and/or ALK6; [10, 21]). To determine which ALK(s) is required for the suppression of StAR expression by BMP4 and BMP7 in human granulosa cells, SVOG cells were treated for 24 hours with BMP4 or BMP7 (30 ng/mL) in the presence or absence of dorsomorphin (10 μM), DMH1 (0.25 μM), or SB-431542 (10 μM). Dorsomorphin is a selective inhibitor of ALK2/3/6 (22), DMH1 is a specific inhibitor of ALK2/3 (23), and SB-431542 is a selective inhibitor ALK4/5/7 (activin/TGF-β type I receptors [24]). The suppressive effects of BMP4 and BMP7 on StAR mRNA and protein levels were abolished by pretreatment with either dorsomorphin (Figure 4, A and D) or DMH1 (Figure 4, B and E) but not SB-431542 (Figure 4, C and F). These results suggest that BMP type I receptors ALK2 or ALK3, but not ALK6, are involved in the inhibitory effects of BMP4 and BMP7 on StAR expression in human granulosa cells.
![The effects of BMP4 and BMP7 on StAR expression are abolished by BMP type I receptor inhibitors dorsomorphin and DMH1 but not by TGF-β type I receptor inhibitor SB-431542. SVOG immortalized human granulosa cells were treated for 24 hours with vehicle control (Ctrl) or 30 ng/mL BMP4 or BMP7 in the absence (dimethylsulfoxide [DMSO]) or presence of 10 μM dorsomorphin (A and D), 0.25 μM DMH1 (B and E), or 10 μM SB-431542 (C and F). StAR mRNA (A–C) and protein (D–F) levels were examined by RT-qPCR and Western blot, respectively. Results are expressed as the mean ± SEM of at least three independent experiments and values without common letters are significantly different (P < .05).](https://oup.silverchair-cdn.com/oup/backfile/Content_public/Journal/endo/156/11/10.1210_en.2015-1494/3/m_zee9991581970004.jpeg?Expires=1747879692&Signature=34gOrNynrkjDxZk-chETmT05gwCi-9ipaT8d5FRRpWPJL1woSx9rrXcf262M~cxB7B2uVtRYYyf1UtzROVLY6E6rQEU1mBiNYce~drQhlOfoMbWUq6nJFNCascJsE2Rl2uGyDBZjf~ch3XSnk86RHftBm4i7H9Gf8Z-AoBtFqzzgtAwNKSULzkxDQj8UkGK3-vSJrEncHqzBGmoKZ5VoQgDhM~sN6dIeHFIxZ0XKkP67hX5Xz7KvvGlOQBCz~-C5gPuwlTVciNRhfkDkxlmZQ2gQE-4L3GWOBxRbVSVATvVdHY-4Ct3DIyGzJRPl9-U3USzCC8yd9YBpTkwivrFSnA__&Key-Pair-Id=APKAIE5G5CRDK6RD3PGA)
The effects of BMP4 and BMP7 on StAR expression are abolished by BMP type I receptor inhibitors dorsomorphin and DMH1 but not by TGF-β type I receptor inhibitor SB-431542. SVOG immortalized human granulosa cells were treated for 24 hours with vehicle control (Ctrl) or 30 ng/mL BMP4 or BMP7 in the absence (dimethylsulfoxide [DMSO]) or presence of 10 μM dorsomorphin (A and D), 0.25 μM DMH1 (B and E), or 10 μM SB-431542 (C and F). StAR mRNA (A–C) and protein (D–F) levels were examined by RT-qPCR and Western blot, respectively. Results are expressed as the mean ± SEM of at least three independent experiments and values without common letters are significantly different (P < .05).
Next, we used an siRNA-based approach to confirm these findings and further specify which ALK(s) mediates the effects of BMP4 and BMP7 on StAR expression. SVOG cells were pretreated for 48 hours with siRNA targeting ALK2, ALK3, or ALK6, treated for a further 24 hours with 30 ng/mL BMP4 or BMP7, and StAR mRNA and protein levels were measured by RT-qPCR and Western blot. Transfection with each siRNA significantly reduced the mRNA levels of only the targeted ALK (Figure 5, A–C). Interestingly, the suppressive effects of BMP4 and BMP7 on StAR mRNA and protein levels were abolished by the knockdown of ALK3 but not ALK2 or ALK6 (Figure 5, D–I). Taken together, these results indicate that ALK3 is required for the down-regulation of StAR expression by BMP4 and BMP7 in SVOG cells.
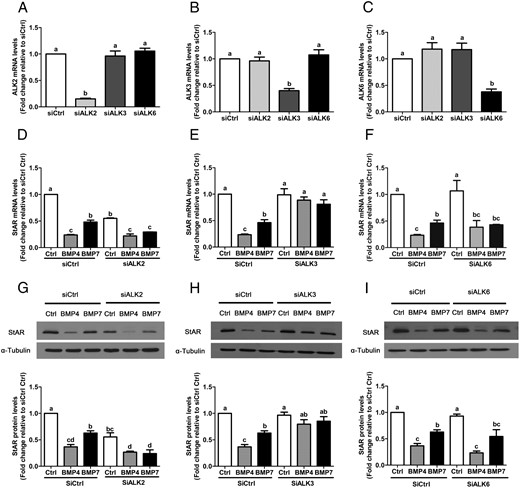
BMP4 and BMP7 down-regulate StAR via ALK3 in SVOG immortalized human granulosa cells. Cells were transfected for 48 hours with 25 nM control siRNA (siCtrl), ALK2 siRNA (siALK2), ALK3 siRNA (siALK3), or ALK6 siRNA (siALK6) and then treated for a further 24 hours with vehicle control (Ctrl) or 30 ng/mL BMP4 or BMP7. The efficiency and specificity of knockdown for each siRNA was evaluated by RT-qPCR (A–C). The mRNA (D–F) and protein (G–I) levels of StAR were examined by RT-qPCR and Western blot, respectively. Results are expressed as the mean ± SEM of at least three independent experiments and values without common letters are significantly different (P < .05).
SMAD1/5/8-SMAD4 signaling is required for BMP4- and BMP7-induced down-regulation of StAR in SVOG cells
We next investigated whether canonical SMAD1/5/8-SMAD4 signaling mediates the effects of BMP4 and BMP7 on StAR expression. First, we used Western blot to measure changes in the levels of phosphorylated SMAD1/5/8 in relation to those of total SMAD1/5/8 in SVOG cells treated for 60 minutes with BMP4 or BMP7 (30 ng/mL) in the presence or absence of DMH1 (0.25 μM). As shown in Figure 6A, pretreatment with DMH1 inhibited BMP4- and BMP7-induced SMAD1/5/8 phosphorylation. Next, we examined BMP4- and BMP7-induced SMAD1/5/8 phosphorylation in SVOG cells pretreated for 48 hours with or without ALK3 siRNA. As shown in Figure 6B, knockdown of ALK3 reversed the phosphorylation of SMAD1/5/8 by BMP4 and BMP7.
![SMAD1/5/8-SMAD4 signaling is required for BMP4- and BMP7-induced the down-regulation of StAR in SVOG immortalized human granulosa cells. A, Cells were treated for 60 minutes with vehicle control (Ctrl) or 30 ng/mL BMP4 or BMP7 in the absence (dimethylsulfoxide [DMSO]) or presence of DMH1 (0.25 μM). Western blot was used to measure the levels of phosphorylated SMAD1/5/8 (p-SMAD1/5/8) in relation to their total levels (SMAD1/5/8), and the results are expressed as fold change relative to control. B, Cells were transfected for 48 hours with 25 nM control siRNA (siCtrl) or ALK3 siRNA (siALK3), treated for 60 minutes with 30 ng/mL BMP4 or BMP7, and changes in phosphorylated SMAD1/5/8 protein levels were examined by Western blot as described above. C and D, Cells were transfected for 48 hours with 25 nM control siRNA (siCtrl) or SMAD4 siRNA (siSMAD4) and then treated for a further 24 hours with BMP4 or BMP7 (30 ng/mL). SMAD4 and StAR mRNA (C) and protein (D) levels were examined by RT-qPCR and Western blot, respectively. Results are expressed as the mean ± SEM of at least three independent experiments and values without common letters are significantly different (P < .05).](https://oup.silverchair-cdn.com/oup/backfile/Content_public/Journal/endo/156/11/10.1210_en.2015-1494/3/m_zee9991581970006.jpeg?Expires=1747879692&Signature=djbqWNin-5A4sSlU67Cwb12iz9s8Qx~QcKZc1E7v3HIFIWYjDdBuGIglxHpUYu7-iCTQ57Ny~7dMmF5HVCiJE2lfj48SuCBUUyqzK~VQvO8zi-KcvEiQMnXqsQzUOSZPB5-NjEWSwlHviezmcCEDbt3l9rie6D1VsGhQWldr4lyKnGCjqnCi6WzJTUMfLLRmIQW8qN~TN7XQtr6W9txMsQddvsMXHlG9QGB2cZdkI~vFf7l53jDT8gAg2qFYHY4MKPKTH4nJuciet8cVYDbSrvGyLTLE0QPTdVADQnvDaecXJYV9b8fy9oDpvMpnS9UZT2V44lGJ2xPMTcanWHDxww__&Key-Pair-Id=APKAIE5G5CRDK6RD3PGA)
SMAD1/5/8-SMAD4 signaling is required for BMP4- and BMP7-induced the down-regulation of StAR in SVOG immortalized human granulosa cells. A, Cells were treated for 60 minutes with vehicle control (Ctrl) or 30 ng/mL BMP4 or BMP7 in the absence (dimethylsulfoxide [DMSO]) or presence of DMH1 (0.25 μM). Western blot was used to measure the levels of phosphorylated SMAD1/5/8 (p-SMAD1/5/8) in relation to their total levels (SMAD1/5/8), and the results are expressed as fold change relative to control. B, Cells were transfected for 48 hours with 25 nM control siRNA (siCtrl) or ALK3 siRNA (siALK3), treated for 60 minutes with 30 ng/mL BMP4 or BMP7, and changes in phosphorylated SMAD1/5/8 protein levels were examined by Western blot as described above. C and D, Cells were transfected for 48 hours with 25 nM control siRNA (siCtrl) or SMAD4 siRNA (siSMAD4) and then treated for a further 24 hours with BMP4 or BMP7 (30 ng/mL). SMAD4 and StAR mRNA (C) and protein (D) levels were examined by RT-qPCR and Western blot, respectively. Results are expressed as the mean ± SEM of at least three independent experiments and values without common letters are significantly different (P < .05).
Upon phosphorylation/activation, SMAD1/5/8 binds with common SMAD4 to form heteromeric complexes that regulate target gene transcription. To determine whether the effects of BMP4 and BMP7 on StAR expression are SMAD dependent, siRNA was used to down-regulate SMAD4 prior to treatment for 24 hours with BMP4 or BMP7 (30 ng/mL). Pretreatment for 48 hours with SMAD4 siRNA significantly reduced SMAD4 mRNA and protein levels and abolished BMP4- and BMP7-induced down-regulation of StAR mRNA and protein (Figure 6, C and D). Taken together, these results indicate that SMAD1/5/8-SMAD4 signaling is involved in the suppression of StAR expression by BMP4 and BMP7 in SVOG cells.
Discussion
We describe for the first time the effects of recombinant human BMP4 or BMP7 on StAR expression and progesterone production in primary and immortalized human granulosa-lutein cells. Specifically, treatment with BMP4 or BMP7 down-regulated the rate-limiting regulatory protein StAR and suppressed progesterone accumulation in conditioned medium. After ovulation, granulosa cells become part of the corpus luteum, which produces large amounts of progesterone that are required for establishing and maintaining early pregnancy. However, elevated progesterone levels prior to ovulation can result in premature luteinization which adversely affects oocyte quality and endometrial receptivity (25, 26). Although pituitary gonadotropins are key regulators of luteal steroidogenesis (27–29), important paracrine or autocrine regulation has also been described for local growth factors, such as IGF, TGF-β, and activins/inhibins (30, 31). In addition to the well-characterized roles of oocyte-derived factors (eg, BMP15 and growth differentiation factor 9 [32, 33]), increasing evidence suggests that theca cell-derived factors also play important roles in follicular development and granulosa cell function.
The present results suggest BMP4 and BMP7 may act as inhibitors of granulosa cell luteinization prior to or after ovulation. Indeed, injection of BMP7 into the ovarian bursa of rats significantly reduces serum progesterone levels and ovulation rate (16). Studies in cow and sheep granulosa cells have demonstrated suppressive effects of BMP4 and BMP7 on basal progesterone production (14, 34–37), whereas in rat granulosa cells both no effects (12) and suppression (35) have been reported. In addition to regulating basal progesterone synthesis, BMP4 and BMP7 have been shown to suppress the production of progesterone in response to FSH in rat granulosa cells (12), IGF-I in cow granulosa cells (34), and cAMP in KGN human granulosa tumor cells (38). Moreover, treatment with BMP4 suppresses FSH-induced progesterone production in sheep granulosa cells (14). Future studies will be required to investigate the effects of BMP4 and BMP7 on gonadotropin-induced progesterone production in human granulosa cells. Unlike oocyte-derived inhibitors of luteinization, theca cell-derived factors would not be withdrawn upon ovulation. Little is known about the functional roles of BMPs in the luteal phase, especially as it relates to corpus luteum formation, function and regression. Interestingly, treatment of cow theca cells with BMP4 and BMP7 enhances basal, but not LH-induced, progesterone production (39). Whether the differential effects of BMP4 and BMP7 on progesterone production are maintained in granulosa- vs theca-luteal cells of the corpus luteum would be an interesting question for future investigation.
Animal as well as in vitro human studies suggest intraovarian roles for BMP4 and BMP7; however, little is known about their potential dysregulation in human ovarian reproductive disorders and infertility. Early studies suggested that women with polycystic ovary syndrome (PCOS) may have reduced serum BMP7 levels compared with healthy controls (39 ± 4 vs 75 ± 21 pg/mL, respectively [40]). However in another cohort of PCOS patients, serum BMP4 levels were undetectable in all samples and only 3 of 20 women had detectable serum BMP7 (range 42–58 pg/ml [41]). Similarly, previous studies measuring serum BMP4 (42) or BMP7 (43–45) found levels approaching or below the lower limits of detection of these Quantikine assays. Alternatively, analyzing follicular fluid BMP4 or BMP7 levels may be more informative than serum levels in the context of ovarian reproductive disorders, infertility, and assisted reproductive technology. Primary human granulosa cells have been shown to express BMP4 and BMP7 mRNA; however, their levels were not significantly different between women with PCOS and healthy controls (46). Interestingly, concentrations of BMP7 in human follicular fluid (50–100 ng/mL [47]) are at least 3 orders of magnitude higher than serum levels measured using the same assay (40, 41, 43–45). However, BMP7 levels did not correlate with those of estrogen or progesterone in follicular fluid from 24 women with unexplained infertility or tubal factors (47).
In contrast, follicular fluid BMP4 concentrations (1–20 pg/mL [48]) are similar to those previously measured in serum (42) and appear to be at least 3 orders of magnitude lower than those of BMP7. Detailed in situ hybridization studies in rats suggest that BMP4 is localized to theca externa cells and an outer layer of theca interna cells, whereas BMP7 is localized to an inner layer of theca interna cells close to the basal lamina (11). This pattern of expression could account for the differences in follicular fluid levels and might suggest a more important role for BMP7 in regulating granulosa cell function, whereas BMP4 may be a more significant autocrine/paracrine regulator of theca cell function. Future studies will be required to clearly define the cell types expressing BMP4 and BMP7 during folliculogenesis in humans. In addition, studies examining follicular fluid concentrations of BMP4 and BMP7 in women with PCOS or other reproductive disorders will be of interest.
BMP4 and BMP7 inhibit progesterone production in human granulosa cells by down-regulating the mRNA and protein levels of StAR. In rat granulosa cells, treatment with BMP7 suppressed FSH-induced StAR mRNA levels but did not alter basal mRNA levels (16). BMP4 has been shown to suppress basal StAR mRNA levels in cow granulosa cells (36) as well as basal and FSH-induced StAR mRNA and protein levels in sheep granulosa cells (14). In KGN cells, BMP4 and BMP7 were shown to reduce basal and cAMP-induced StAR mRNA levels as well as basal STAR promoter activity (38). Although BMP4 and BMP7 can regulate StAR expression, the mechanisms underlying these effects remain poorly defined. We have demonstrated that the effects of BMP4 and BMP7 on StAR expression are SMAD dependent; however, the time-course (≥12 h) does not rule out indirect effects via induction/suppression of other transcription factors. This is in keeping with the fact that no consensus SMAD binding elements have been described for the STAR promoter. However, a multitude of associations with diverse DNA-binding factors is critical to selective and high-affinity interactions of SMAD complexes with target gene promoters (49, 50).
Previous studies in sheep granulosa cells demonstrated that the induction of STAR promoter activity by SF-1 overexpression was inhibited by cotransfection with Smad1 (14). Although it suggests a negative crosstalk between Smad1 and SF-1, coimmunoprecipitation studies failed to detect any direct binding between Smad1 and SF-1 (14). In addition, the down-regulation of SF-1 expression is not likely to contribute to the effects of BMP4 or BMP7 on StAR expression because neither BMP altered SF-1 mRNA levels in SVOG cells (Supplemental Figure 1). Regulatory SMADs have also been shown to interact with transcription factors such as activator protein 1 and CCAAT enhancer binding protein β (50), both of which have also been implicated in the regulation of STAR promoter activity (51). In addition, BMP4 has been shown to reduce the levels of acetylated histone H3 in the STAR promoter at 48 and 72 hours in cow granulosa cells (36). Clarifying the mechanism(s) by which SMAD complexes regulate StAR expression in human granulosa cells is of increasing interest, given that, in addition to the present findings for BMP4 and BMP7, studies have implicated BMP2 (52), BMP15 (33), activins (53), and TGF-β1 (20) in the regulation of StAR.
In addition to characterizing the effects of BMP4 and BMP7 on StAR expression and progesterone production, we used pharmacological and siRNA-based approaches to demonstrate that ALK3 is required for the down-regulation of StAR expression in human granulosa cells. These results are consistent with our previous studies showing that ALK3 mediates the effects of BMP15 on StAR expression in human granulosa cells (33). However, they differ from our recent findings that differential subsets of BMP type I receptors mediate the down-regulation of pentraxin 3 by BMP4 (ALK3/6) and BMP7 (ALK3/2) in human granulosa cells (48). Together these findings suggest that human granulosa cells have the capacity to signal via multiple type I receptors in response to BMP4 or BMP7 but that different receptor(s) may somehow be coupled to different target genes and/or functions. Previous studies in diverse cell types have described differing type I receptor binding affinities for BMP4 and BMP7 (54, 55). Our results appear to support differences in receptor binding characteristics as BMP4 produced significant reductions in StAR expression and progesterone production at 1 ng/mL, whereas concentrations between 30 and 100 ng/mL were required for BMP7. Nevertheless, previous studies in KGN cells have likewise demonstrated differences in type I receptor dependence between multiple promoter-luciferase constructs (38). In particular, forced-expression of ALK3 or ALK6 enhanced the effects of BMP4, BMP7 or BMP6 on the 3TP-luciferase construct. Overexpression of ALK3 or ALK6 also enhanced the effects of BMP7 or BMP6 on the Id1- and Tlx2-luciferase constructs, whereas the effects of BMP4 on the Tlx2-luciferase construct were only enhanced by ALK3, and its effects on the Id1-luciferase construct were not enhanced by overexpression of ALK2, ALK3, or ALK6 (38).
Future studies investigating putative differential coupling of BMP type I receptors to specific cellular responses, as well as the mechanisms involved, are warranted because they could lead to novel pharmacological strategies for clinical use. Although selective antagonists or agonists of individual BMP type I receptors have yet to be fully developed, they might be used to selectively target specific biological processes. For example, in granulosa cells, specific targeting of ALK6 could alter the effects of BMP4, but not BMP7, on pentraxin 3 (and possibly cumulus expansion) without altering the effects of either protein on StAR expression or progesterone production. In addition, dysregulated receptor expression could contribute to reproductive disorders and infertility by altering the relative potency/efficacy of endogenous ligands. Indeed, conditional deletion of Bmpr1a (Alk3) in granulosa cells causes reduced fertility, fecundity, and spontaneous ovulation as well as progressive infertility (56). Interestingly, granulosa cells from women with PCOS have elevated levels of ALK3 mRNA, which could enhance the effects of BMP4 and BMP7, even in the absence of changes in their mRNA levels (46).
In summary, the present study demonstrates that BMP4 and BMP7 suppress progesterone production by down-regulating the expression of StAR in human granulosa-lutein cells. Furthermore, our results in SVOG immortalized granulosa cells suggest the suppressive effects of BMP4 and BMP7 are mediated by ALK3 and most likely involve SMAD1/5/8/-SMAD4 signaling. These findings provide important insights into the functional roles and molecular mechanisms of theca cell-derived BMPs in the periovulatory period.
Acknowledgments
We thank the patients, Dr Elizabeth L. Taylor, and the team at the Olive Fertility Centre for providing the follicular aspirates used for the purification of primary human granulosa-lutein cells.
This work was supported by an operating grant from the Canadian Institutes of Health Research (to P.C.K.L.). H.Z. is supported by the China Scholarship Council.
Disclosure Summary: The authors have nothing to disclose.
Abbreviations
- ALK
activin receptor-like kinase
- BMP
bone morphogenetic protein
- E
efficiency
- GAPDH
glyceraldehyde-3-phosphate dehydrogenase
- PCOS
polycystic ovary syndrome
- P450scc
P450 side-chain cleavage enzyme
- RT-qPCR
reverse transcription-quantitative real-time PCR
- SF-1
steroidogenic factor 1
- siRNA
small interfering RNA
- StAR
steroidogenic acute regulatory protein
- TBST
Tris-buffered saline containing Tween 20.