-
PDF
- Split View
-
Views
-
Cite
Cite
Serge Korjian, Syed Hassan A Kazmi, Gerald Chi, Arzu Kalayci, Jane J Lee, Usama Talib, Samuel D Wright, Danielle Duffy, Bronwyn A Kingwell, Roxana Mehran, Paul M Ridker, C Michael Gibson, Biological basis and proposed mechanism of action of CSL112 (apolipoprotein A-I [human]) for prevention of major adverse cardiovascular events in patients with myocardial infarction, European Heart Journal - Cardiovascular Pharmacotherapy, Volume 9, Issue 4, June 2023, Pages 387–398, https://doi.org/10.1093/ehjcvp/pvad014
- Share Icon Share
Abstract
Despite current standard of care treatment, the period shortly after acute myocardial infarction (AMI) is associated with high residual cardiovascular (CV) risk, with high rates of recurrent AMI and CV death in the first 90 days following the index event. This represents an area of high unmet need that may be potentially addressed by novel therapeutic agents that optimize high-density lipoprotein cholesterol (HDL-C) function rather than increase HDL-C concentrations. Apolipoprotein A-I (apoA-I) is the major constituent of HDL and a key mediator of cholesterol efflux from macrophages within atherosclerotic plaque, a property especially relevant during the high-risk period immediately following an AMI when cholesterol efflux capacity is found to be reduced. CSL112 is a novel formulation of human plasma-derived apolipoprotein A-I (apoA-I), currently being evaluated in a Phase 3 clinical trial (AEGIS-II) for the reduction of major adverse CV events in the 90-day high-risk period post-AMI. In this review, we provide an overview of the biological properties of CSL112 that contribute to its proposed mechanism of action for potential therapeutic benefit. These properties include rapid and robust promotion of cholesterol efflux from cells abundant in atherosclerotic plaque, in addition to anti-inflammatory effects, which together, may have a stabilizing effect on atherosclerotic plaque. We provide a detailed overview of these mechanisms, in addition to information on the composition of CSL112 and how it is manufactured.
Introduction
Epidemiological studies, including the multigenerational Framingham Heart Study, have demonstrated that higher levels of high-density lipoprotein cholesterol (HDL-C) are associated with lower rates of adverse cardiovascular (CV) outcomes.1–3 These findings underpinned the hypothesis that raising HDL-C might reduce CV events and led to the development of several therapies aimed at reducing the rate of CV events by raising levels of HDL-C. However, large-scale randomized clinical trials of pharmacological agents, such as niacin and inhibitors of cholesteryl ester transfer protein, have shown that raising the serum concentration of endogenous HDL-C is not associated with an improvement in CV outcomes [major adverse CV event (MACE) reduction and all-cause and coronary heart disease (CHD)-related mortality reduction].4–6 This has changed the discussion in relation to HDL and cardiovascular disease (CVD) risk.
Rather than raising the concentration of HDL-C, recent studies have highlighted the importance of improving the quality or function of HDL particles, a key measure of which is cholesterol efflux capacity (CEC).7–9 The field has begun to pivot away from the ‘HDL Hypothesis’, which focused on HDL cholesterol concentration (HDL-C) to one centred on the ability of the HDL to accept cholesterol from cells, i.e. CEC. High CEC has been inversely related to the incidence of CV events.10 Additionally, and of particular interest, CEC is significantly impaired following acute myocardial infarction (AMI),11 which represents a potential therapeutic target to reduce rates of MACE following an AMI event, particularly in the early, high-risk period.12,13 CSL112, a novel, infusible formulation of human apolipoprotein A-I (apoA-I), (the major protein constituent of HDL) significantly elevates CEC.14–19 Whether this improvement in CEC resulting from CSL112 infusion reduces the risk of MACE after AMI is the subject of an ongoing multicentre, Phase 3, randomized clinical trial20 (AEGIS-II; ClinicalTrials.gov: NCT03473223).
This review article will discuss the proposed mechanism of action, the manufacturing process, safety, tolerability, and potential future clinical applications of CSL112, a novel investigational pharmacotherapy aimed at reducing residual CV risk in high-risk post-AMI patients.
CSL112: a novel investigational pharmacotherapy designed to improve high-density lipoprotein function
CSL112 is a novel formulation of human apoA-I, formulated for intravenous infusion. The apoA-I is purified from human plasma and is combined with phosphatidylcholine (PC) as a structural component to yield disc-shaped HDL particles. The discs are lyophilized using sucrose as a stabilizer.21,22 Formulation of apoA-I with PC allows a volume of distribution and clearance rate comparable with endogenous apoA-I,22 which is around 48 h.23 Key components of CSL112 are shown in Table 1. Compared with other previously studied infusible HDL therapies, CSL112 is not a mimetic or recombinant agent but purified wild-type apoA-I from human plasma. A comprehensive list of infusible HDL and HDL-mimetic therapies previously studied is provided in Table 2.
Component . | Nominal contents per vial (g) . | Nominal concentration (g/L)a . |
---|---|---|
ApoA-I | 2.0 | 35 |
Phosphatidylcholine | 2.65 | 47.1 |
Cholate | 0.04 | 0.71 |
Sucrose | 3.28 | 58.4b |
Component . | Nominal contents per vial (g) . | Nominal concentration (g/L)a . |
---|---|---|
ApoA-I | 2.0 | 35 |
Phosphatidylcholine | 2.65 | 47.1 |
Cholate | 0.04 | 0.71 |
Sucrose | 3.28 | 58.4b |
After reconstitution with 50 mL WFI.
Corresponds to 56.7 g sucrose/kg solution.
ApoA-I, apolipoprotein A-I.
Component . | Nominal contents per vial (g) . | Nominal concentration (g/L)a . |
---|---|---|
ApoA-I | 2.0 | 35 |
Phosphatidylcholine | 2.65 | 47.1 |
Cholate | 0.04 | 0.71 |
Sucrose | 3.28 | 58.4b |
Component . | Nominal contents per vial (g) . | Nominal concentration (g/L)a . |
---|---|---|
ApoA-I | 2.0 | 35 |
Phosphatidylcholine | 2.65 | 47.1 |
Cholate | 0.04 | 0.71 |
Sucrose | 3.28 | 58.4b |
After reconstitution with 50 mL WFI.
Corresponds to 56.7 g sucrose/kg solution.
ApoA-I, apolipoprotein A-I.
Type of reconstituted HDL agent . | Formulation . | Particle size [nanometers (nm)] . | Citation . | Tested human population (n) . | Study type . | NCT identifier . | Primary outcome . | Results . |
---|---|---|---|---|---|---|---|---|
ProApoA1 | Recombinant human proapolipoprotein A-I/liposomes (1:1.25 molar ratio) | 7–30 | Carlson et al.82 | Subjects with low HDL cholesterol (n = 4) | Single dose (Phase 1) | –– | Effect of a single infusion of recombinant human proapolipoprotein AI liposomes (synthetic HDL) on plasma lipoproteins in patients with low HDL | Increased levels of serum apoA-I subsequent to infusion; half-life of apoA-I <24 h |
Eriksson et al.83 | Subjects with familial hypercholesterolemia (n = 4) | Single dose (Phase 1) | –– | Determination of role of apoA-I in reverse cholesterol transport and biliary excretion (fecal steroid excretion) | Increased fecal excretion of cholesterol (sterols and bile acids; mean increase, +39% and +30%, respectively) | |||
ETC-216 | Recombinant apoA-I Milano/palmitoyloleoylphosphatidyl choline (1:1 molar ratio) | 7–30 | Bisgaier et al.84 | Healthy subjects (n = 32) | Single dose (Phase 1) | –– | Pharmacokinetics, safety, and tolerability | Well tolerated |
Nissen et al.85 | ACS patients (n = 57) | Multiple doses (Phase 2) | –– | % change in atheroma volume by IVUS | Significant reduction in atheroma volume recorded. Two adverse events in high-dose group resulting in study withdrawal | |||
MDCO-216 | ApoA-I Milano/palmitoyloleoylphosphatidyl choline (1:1 molar ratio) | 7–30 | Kempen et al.91 | Healthy subjects (n = 24) and patients with stable coronary artery disease (n = 24) | Multiple doses (Phase 2) | –– | Effects on HDL subfractions | Dose-dependent increase in pre-β1, α1, and α2 HDL; decrease in α3 and α4 HDL |
Nicholls et al.92 | Subjects with acute coronary syndrome (n = 126) | MILANO-PILOT study (multiple doses—Phases 1 and 2) | NCT02678923 | IVUS assessed % change in atheroma volume, efficacy, pharmacokinetics, safety, and tolerability | A similar proportion of patients in the MDCO-216 and placebo group showed regression in plaque volume | |||
CER-001 | Recombinant apoA-I/egg derived sphingomyelin/dipalmitoyl-sn-glycero-3-phosphorylglycerol (1:2.7:0.1 molar ratio) | 7–13 | Keyserling et al.86 | Healthy subjects (n = 32) | Single dose (0.25–45 mg/kg—Phase 1) | –– | Pharmacokinetics, safety, and tolerability | Well tolerated |
Tardif et al.87 | ACS patients (n = 504) | CHI-SQUARE study (Phase 2) | NCT01201837 | IVUS-assessed change in total coronary plaque volume | CER-001 infusions did not reduce coronary atherosclerosis on IVUS and quantitative coronary angiography | |||
Hovingh et al.88 | Heterozygous familial hypercholesterolemia (n = 30) | MODE study (Phase 2) | NCT01412034 | MRI assessed % change in total carotid plaque volume | ApoA-I increased from 114.8 ± 20.7 mg/dL to 129.3 ± 23.0 mg/dL. After 24 weeks, mean vessel wall area decreased from 17.23 to 16.75 mm2 (P = 0.008) | |||
Nicholls et al.89 | ACS patients (n = 292) | CARAT study (Phase 2) | NCT02484378 | IVUS-assessed % change in coronary atheroma volume | CER-001 3 mg/kg did not reduce % atheroma volume vs. placebo (−0.09% vs. −0.41%; P = 0.15) | |||
Cerenis Therapeutics90 | Familial hypoalpha-lipoproteinemia (n = 23) | TANGO study (Phase 3) | NCT02697136 | 3TMRI-assessed change in mean vessel wall area of the carotid artery | Early study termination due to lack of efficacy | |||
CSL111 | Purified wild type apoA-I from human plasma complexed with phosphatidylcholine (soy source; 1:150 molar ratio) | 7–30 | Van Oostrom et al.93 | Subjects with type 2 diabetes (n = 7) | Single dose (80 mg/kg—Phase 1) | –– | Effect of raising HDL levels by intravenous infusion of rHDL on endothelial progenitor cells (EPCs) | ApoA-I increased from 1.2 ± 0.2 (baseline) to 2.8 ± 0.6 g/L (t = 7 h, P < 0.001); increase in the number of EPCs in blood (480±85 mL at baseline vs. 1060 ± 347/mL at t = 7d, P < 0.05) |
Patel et al.76 | Subjects with type 2 diabetes (n = 13) | Single dose (80 mg/kg—Phase 1) | –– | Effect of rHDL on anti-inflammatory properties of endogenous HDL and ex vivo CEC | VCAM and ICAM-1 inhibition proportional to increase in endogenous HDL (up to 25%, P < 0.01); reduced expression of CD11b and neutrophil adhesion 72 h post-rHDL infusion (P = 0.02); increased CEC 1–72 h post-rHDL (40–60%, P < 0.05) | |||
Shaw et al.94 | Patients with claudication scheduled for percutaneous revascularization (n = 20) | Single dose (80 mg/kg—Phase 1) | –– | Determination of acute changes in plaque characteristic following rHDL infusions | Lower VCAM-1 expression vs. placebo (28 ± 3% vs. 50 ± 3%; P < 0.05) and a reduction in lipid content in the plaque of HDL-treated subjects compared with placebo | |||
Tardif et al.97 | ACS patients (n = 183) | ERASE study multiple doses (Phase 2) | NCT00225719 | Determination of % change in atheroma volume, plaque volume, plaque characterization index assessed by IVUS and QCA | rHDL infusions resulted in no significant reductions in atheroma volume (−3.4% with CSL111 and −1.6% for placebo, P = 0.48); significant improvement in plaque characterization index (−0.0097 for CSL111 and 0.0128 with placebo, P = 0.01) | |||
CSL112 | Purified wild-type apoA-I from human plasma complexed with phosphatidylcholine (soy source; 1:50 molar ratio) | 7–13 | Gille et al.14 | Healthy subjects (n = 57) | Single ascending dose (Phase 1) | NCT01129661 | Quantification of rise in apoA-I, pre-β1 HDL, ABCA1-dependent and total CEC following CSL112 infusion | CSL112 infusions lead to rapid dose-dependent rise in serum apoA-I, HDL, LCAT activity, and 2.9-fold elevation in total CEC and ≤5.8-fold elevation in ABCA1-dependent CEC |
Easton et al.95 | Healthy subjects (n = 36) | Multiple doses (Phase 1) | NCT01281774 | Assessment of safety, tolerability, and PK/PD | CSL112 infusions lead to rapid dose-dependent rise in serum apoA-I and PC. All AEs were mild to moderate and resolved by end of reporting period, no clinically relevant or dose-dependent increase in ALT, AST, bilirubin, deaths or SAEs | |||
Tricoci et al.15 | Subjects with stable atherosclerotic disease (n = 45) | Single ascending dose (1.7, 3.4, or 6.8 g—Phase 2a) | NCT01499420 | Assessment of safety, tolerability, and PK/PD | No clinically relevant elevations in AST, ALT. No SAEs. Rapid (Tmax ≈ 2 h) and dose-dependent increases in apoA-I (145% increase in the 6.8 g group) and total cholesterol efflux (up to 3.1-fold higher than placebo; P < 0.001) | |||
Tortorici et al.80 and Gille et al.96 | Healthy subjects with and without moderate renal impairment (n = 32) | Single ascending dose (2 and 6 g—Phase 1) | NCT02427035 | Assessment of safety, tolerability, and PK/PD | Moderate renal impairment did not impact the PK and PD of CSL112, and was well tolerated in these patients | |||
Gibson et al.16 | Subjects with acute coronary syndrome (n = 1258) | AEGIS-I study multiple doses (2 and 6 g—Phase 2b) | NCT02108262 | Assessment of safety, tolerability, and PK/PD | No association with CSL112 and alterations in either liver or kidney function. Dose-dependent elevation in both apoA-I and CEC. CSL112 2 g: 1.29-, 1.87-, and 3.67-fold elevations in apoA-I, and total and ABCA1-dependent CEC, respectively. CSL112 6 g: 2.06-, 2.45-, and 4.30-fold elevations in apoA-I, and total and ABCA1-dependent CEC, respectively | |||
Gibson et al.18 | AMI patients with stage 3 CKD | Multiple doses (6 g—Phase 2) | NCT02742103 | Assessment of safety, tolerability, and PK/PD | Well tolerated in patients with both AMI and moderate renal impairment | |||
Ongoing | Subjects with acute coronary syndrome, multivessel disease, high-risk population (n = 17 400) | AEGIS-II study (Phase 3) | NCT03473223 | Efficacy and safety of CSL112 in reduction of MACE | Estimated completion date: June 2022 |
Type of reconstituted HDL agent . | Formulation . | Particle size [nanometers (nm)] . | Citation . | Tested human population (n) . | Study type . | NCT identifier . | Primary outcome . | Results . |
---|---|---|---|---|---|---|---|---|
ProApoA1 | Recombinant human proapolipoprotein A-I/liposomes (1:1.25 molar ratio) | 7–30 | Carlson et al.82 | Subjects with low HDL cholesterol (n = 4) | Single dose (Phase 1) | –– | Effect of a single infusion of recombinant human proapolipoprotein AI liposomes (synthetic HDL) on plasma lipoproteins in patients with low HDL | Increased levels of serum apoA-I subsequent to infusion; half-life of apoA-I <24 h |
Eriksson et al.83 | Subjects with familial hypercholesterolemia (n = 4) | Single dose (Phase 1) | –– | Determination of role of apoA-I in reverse cholesterol transport and biliary excretion (fecal steroid excretion) | Increased fecal excretion of cholesterol (sterols and bile acids; mean increase, +39% and +30%, respectively) | |||
ETC-216 | Recombinant apoA-I Milano/palmitoyloleoylphosphatidyl choline (1:1 molar ratio) | 7–30 | Bisgaier et al.84 | Healthy subjects (n = 32) | Single dose (Phase 1) | –– | Pharmacokinetics, safety, and tolerability | Well tolerated |
Nissen et al.85 | ACS patients (n = 57) | Multiple doses (Phase 2) | –– | % change in atheroma volume by IVUS | Significant reduction in atheroma volume recorded. Two adverse events in high-dose group resulting in study withdrawal | |||
MDCO-216 | ApoA-I Milano/palmitoyloleoylphosphatidyl choline (1:1 molar ratio) | 7–30 | Kempen et al.91 | Healthy subjects (n = 24) and patients with stable coronary artery disease (n = 24) | Multiple doses (Phase 2) | –– | Effects on HDL subfractions | Dose-dependent increase in pre-β1, α1, and α2 HDL; decrease in α3 and α4 HDL |
Nicholls et al.92 | Subjects with acute coronary syndrome (n = 126) | MILANO-PILOT study (multiple doses—Phases 1 and 2) | NCT02678923 | IVUS assessed % change in atheroma volume, efficacy, pharmacokinetics, safety, and tolerability | A similar proportion of patients in the MDCO-216 and placebo group showed regression in plaque volume | |||
CER-001 | Recombinant apoA-I/egg derived sphingomyelin/dipalmitoyl-sn-glycero-3-phosphorylglycerol (1:2.7:0.1 molar ratio) | 7–13 | Keyserling et al.86 | Healthy subjects (n = 32) | Single dose (0.25–45 mg/kg—Phase 1) | –– | Pharmacokinetics, safety, and tolerability | Well tolerated |
Tardif et al.87 | ACS patients (n = 504) | CHI-SQUARE study (Phase 2) | NCT01201837 | IVUS-assessed change in total coronary plaque volume | CER-001 infusions did not reduce coronary atherosclerosis on IVUS and quantitative coronary angiography | |||
Hovingh et al.88 | Heterozygous familial hypercholesterolemia (n = 30) | MODE study (Phase 2) | NCT01412034 | MRI assessed % change in total carotid plaque volume | ApoA-I increased from 114.8 ± 20.7 mg/dL to 129.3 ± 23.0 mg/dL. After 24 weeks, mean vessel wall area decreased from 17.23 to 16.75 mm2 (P = 0.008) | |||
Nicholls et al.89 | ACS patients (n = 292) | CARAT study (Phase 2) | NCT02484378 | IVUS-assessed % change in coronary atheroma volume | CER-001 3 mg/kg did not reduce % atheroma volume vs. placebo (−0.09% vs. −0.41%; P = 0.15) | |||
Cerenis Therapeutics90 | Familial hypoalpha-lipoproteinemia (n = 23) | TANGO study (Phase 3) | NCT02697136 | 3TMRI-assessed change in mean vessel wall area of the carotid artery | Early study termination due to lack of efficacy | |||
CSL111 | Purified wild type apoA-I from human plasma complexed with phosphatidylcholine (soy source; 1:150 molar ratio) | 7–30 | Van Oostrom et al.93 | Subjects with type 2 diabetes (n = 7) | Single dose (80 mg/kg—Phase 1) | –– | Effect of raising HDL levels by intravenous infusion of rHDL on endothelial progenitor cells (EPCs) | ApoA-I increased from 1.2 ± 0.2 (baseline) to 2.8 ± 0.6 g/L (t = 7 h, P < 0.001); increase in the number of EPCs in blood (480±85 mL at baseline vs. 1060 ± 347/mL at t = 7d, P < 0.05) |
Patel et al.76 | Subjects with type 2 diabetes (n = 13) | Single dose (80 mg/kg—Phase 1) | –– | Effect of rHDL on anti-inflammatory properties of endogenous HDL and ex vivo CEC | VCAM and ICAM-1 inhibition proportional to increase in endogenous HDL (up to 25%, P < 0.01); reduced expression of CD11b and neutrophil adhesion 72 h post-rHDL infusion (P = 0.02); increased CEC 1–72 h post-rHDL (40–60%, P < 0.05) | |||
Shaw et al.94 | Patients with claudication scheduled for percutaneous revascularization (n = 20) | Single dose (80 mg/kg—Phase 1) | –– | Determination of acute changes in plaque characteristic following rHDL infusions | Lower VCAM-1 expression vs. placebo (28 ± 3% vs. 50 ± 3%; P < 0.05) and a reduction in lipid content in the plaque of HDL-treated subjects compared with placebo | |||
Tardif et al.97 | ACS patients (n = 183) | ERASE study multiple doses (Phase 2) | NCT00225719 | Determination of % change in atheroma volume, plaque volume, plaque characterization index assessed by IVUS and QCA | rHDL infusions resulted in no significant reductions in atheroma volume (−3.4% with CSL111 and −1.6% for placebo, P = 0.48); significant improvement in plaque characterization index (−0.0097 for CSL111 and 0.0128 with placebo, P = 0.01) | |||
CSL112 | Purified wild-type apoA-I from human plasma complexed with phosphatidylcholine (soy source; 1:50 molar ratio) | 7–13 | Gille et al.14 | Healthy subjects (n = 57) | Single ascending dose (Phase 1) | NCT01129661 | Quantification of rise in apoA-I, pre-β1 HDL, ABCA1-dependent and total CEC following CSL112 infusion | CSL112 infusions lead to rapid dose-dependent rise in serum apoA-I, HDL, LCAT activity, and 2.9-fold elevation in total CEC and ≤5.8-fold elevation in ABCA1-dependent CEC |
Easton et al.95 | Healthy subjects (n = 36) | Multiple doses (Phase 1) | NCT01281774 | Assessment of safety, tolerability, and PK/PD | CSL112 infusions lead to rapid dose-dependent rise in serum apoA-I and PC. All AEs were mild to moderate and resolved by end of reporting period, no clinically relevant or dose-dependent increase in ALT, AST, bilirubin, deaths or SAEs | |||
Tricoci et al.15 | Subjects with stable atherosclerotic disease (n = 45) | Single ascending dose (1.7, 3.4, or 6.8 g—Phase 2a) | NCT01499420 | Assessment of safety, tolerability, and PK/PD | No clinically relevant elevations in AST, ALT. No SAEs. Rapid (Tmax ≈ 2 h) and dose-dependent increases in apoA-I (145% increase in the 6.8 g group) and total cholesterol efflux (up to 3.1-fold higher than placebo; P < 0.001) | |||
Tortorici et al.80 and Gille et al.96 | Healthy subjects with and without moderate renal impairment (n = 32) | Single ascending dose (2 and 6 g—Phase 1) | NCT02427035 | Assessment of safety, tolerability, and PK/PD | Moderate renal impairment did not impact the PK and PD of CSL112, and was well tolerated in these patients | |||
Gibson et al.16 | Subjects with acute coronary syndrome (n = 1258) | AEGIS-I study multiple doses (2 and 6 g—Phase 2b) | NCT02108262 | Assessment of safety, tolerability, and PK/PD | No association with CSL112 and alterations in either liver or kidney function. Dose-dependent elevation in both apoA-I and CEC. CSL112 2 g: 1.29-, 1.87-, and 3.67-fold elevations in apoA-I, and total and ABCA1-dependent CEC, respectively. CSL112 6 g: 2.06-, 2.45-, and 4.30-fold elevations in apoA-I, and total and ABCA1-dependent CEC, respectively | |||
Gibson et al.18 | AMI patients with stage 3 CKD | Multiple doses (6 g—Phase 2) | NCT02742103 | Assessment of safety, tolerability, and PK/PD | Well tolerated in patients with both AMI and moderate renal impairment | |||
Ongoing | Subjects with acute coronary syndrome, multivessel disease, high-risk population (n = 17 400) | AEGIS-II study (Phase 3) | NCT03473223 | Efficacy and safety of CSL112 in reduction of MACE | Estimated completion date: June 2022 |
ABCA1, ATP-binding cassette transporter A1; ACS, acute coronary syndrome; ALT, alanine aminotransferase; AMI, acute myocardial infarction; apoA-I, apolipoprotein A-I; AST, aspartate transaminase; CEC, cholesterol efflux capacity; HDL, high-density lipoprotein; ICAM-1, intercellular adhesion molecule-1; IVUS, intravascular ultrasound; PD, pharmacodynamics; PK pharmacokinetics; rHDL, reconstituted high-density lipoprotein cholesterol; SAE, serious adverse event; Tmax, time to maximum concentration; and VCAM, vascular cell adhesion molecule.
Type of reconstituted HDL agent . | Formulation . | Particle size [nanometers (nm)] . | Citation . | Tested human population (n) . | Study type . | NCT identifier . | Primary outcome . | Results . |
---|---|---|---|---|---|---|---|---|
ProApoA1 | Recombinant human proapolipoprotein A-I/liposomes (1:1.25 molar ratio) | 7–30 | Carlson et al.82 | Subjects with low HDL cholesterol (n = 4) | Single dose (Phase 1) | –– | Effect of a single infusion of recombinant human proapolipoprotein AI liposomes (synthetic HDL) on plasma lipoproteins in patients with low HDL | Increased levels of serum apoA-I subsequent to infusion; half-life of apoA-I <24 h |
Eriksson et al.83 | Subjects with familial hypercholesterolemia (n = 4) | Single dose (Phase 1) | –– | Determination of role of apoA-I in reverse cholesterol transport and biliary excretion (fecal steroid excretion) | Increased fecal excretion of cholesterol (sterols and bile acids; mean increase, +39% and +30%, respectively) | |||
ETC-216 | Recombinant apoA-I Milano/palmitoyloleoylphosphatidyl choline (1:1 molar ratio) | 7–30 | Bisgaier et al.84 | Healthy subjects (n = 32) | Single dose (Phase 1) | –– | Pharmacokinetics, safety, and tolerability | Well tolerated |
Nissen et al.85 | ACS patients (n = 57) | Multiple doses (Phase 2) | –– | % change in atheroma volume by IVUS | Significant reduction in atheroma volume recorded. Two adverse events in high-dose group resulting in study withdrawal | |||
MDCO-216 | ApoA-I Milano/palmitoyloleoylphosphatidyl choline (1:1 molar ratio) | 7–30 | Kempen et al.91 | Healthy subjects (n = 24) and patients with stable coronary artery disease (n = 24) | Multiple doses (Phase 2) | –– | Effects on HDL subfractions | Dose-dependent increase in pre-β1, α1, and α2 HDL; decrease in α3 and α4 HDL |
Nicholls et al.92 | Subjects with acute coronary syndrome (n = 126) | MILANO-PILOT study (multiple doses—Phases 1 and 2) | NCT02678923 | IVUS assessed % change in atheroma volume, efficacy, pharmacokinetics, safety, and tolerability | A similar proportion of patients in the MDCO-216 and placebo group showed regression in plaque volume | |||
CER-001 | Recombinant apoA-I/egg derived sphingomyelin/dipalmitoyl-sn-glycero-3-phosphorylglycerol (1:2.7:0.1 molar ratio) | 7–13 | Keyserling et al.86 | Healthy subjects (n = 32) | Single dose (0.25–45 mg/kg—Phase 1) | –– | Pharmacokinetics, safety, and tolerability | Well tolerated |
Tardif et al.87 | ACS patients (n = 504) | CHI-SQUARE study (Phase 2) | NCT01201837 | IVUS-assessed change in total coronary plaque volume | CER-001 infusions did not reduce coronary atherosclerosis on IVUS and quantitative coronary angiography | |||
Hovingh et al.88 | Heterozygous familial hypercholesterolemia (n = 30) | MODE study (Phase 2) | NCT01412034 | MRI assessed % change in total carotid plaque volume | ApoA-I increased from 114.8 ± 20.7 mg/dL to 129.3 ± 23.0 mg/dL. After 24 weeks, mean vessel wall area decreased from 17.23 to 16.75 mm2 (P = 0.008) | |||
Nicholls et al.89 | ACS patients (n = 292) | CARAT study (Phase 2) | NCT02484378 | IVUS-assessed % change in coronary atheroma volume | CER-001 3 mg/kg did not reduce % atheroma volume vs. placebo (−0.09% vs. −0.41%; P = 0.15) | |||
Cerenis Therapeutics90 | Familial hypoalpha-lipoproteinemia (n = 23) | TANGO study (Phase 3) | NCT02697136 | 3TMRI-assessed change in mean vessel wall area of the carotid artery | Early study termination due to lack of efficacy | |||
CSL111 | Purified wild type apoA-I from human plasma complexed with phosphatidylcholine (soy source; 1:150 molar ratio) | 7–30 | Van Oostrom et al.93 | Subjects with type 2 diabetes (n = 7) | Single dose (80 mg/kg—Phase 1) | –– | Effect of raising HDL levels by intravenous infusion of rHDL on endothelial progenitor cells (EPCs) | ApoA-I increased from 1.2 ± 0.2 (baseline) to 2.8 ± 0.6 g/L (t = 7 h, P < 0.001); increase in the number of EPCs in blood (480±85 mL at baseline vs. 1060 ± 347/mL at t = 7d, P < 0.05) |
Patel et al.76 | Subjects with type 2 diabetes (n = 13) | Single dose (80 mg/kg—Phase 1) | –– | Effect of rHDL on anti-inflammatory properties of endogenous HDL and ex vivo CEC | VCAM and ICAM-1 inhibition proportional to increase in endogenous HDL (up to 25%, P < 0.01); reduced expression of CD11b and neutrophil adhesion 72 h post-rHDL infusion (P = 0.02); increased CEC 1–72 h post-rHDL (40–60%, P < 0.05) | |||
Shaw et al.94 | Patients with claudication scheduled for percutaneous revascularization (n = 20) | Single dose (80 mg/kg—Phase 1) | –– | Determination of acute changes in plaque characteristic following rHDL infusions | Lower VCAM-1 expression vs. placebo (28 ± 3% vs. 50 ± 3%; P < 0.05) and a reduction in lipid content in the plaque of HDL-treated subjects compared with placebo | |||
Tardif et al.97 | ACS patients (n = 183) | ERASE study multiple doses (Phase 2) | NCT00225719 | Determination of % change in atheroma volume, plaque volume, plaque characterization index assessed by IVUS and QCA | rHDL infusions resulted in no significant reductions in atheroma volume (−3.4% with CSL111 and −1.6% for placebo, P = 0.48); significant improvement in plaque characterization index (−0.0097 for CSL111 and 0.0128 with placebo, P = 0.01) | |||
CSL112 | Purified wild-type apoA-I from human plasma complexed with phosphatidylcholine (soy source; 1:50 molar ratio) | 7–13 | Gille et al.14 | Healthy subjects (n = 57) | Single ascending dose (Phase 1) | NCT01129661 | Quantification of rise in apoA-I, pre-β1 HDL, ABCA1-dependent and total CEC following CSL112 infusion | CSL112 infusions lead to rapid dose-dependent rise in serum apoA-I, HDL, LCAT activity, and 2.9-fold elevation in total CEC and ≤5.8-fold elevation in ABCA1-dependent CEC |
Easton et al.95 | Healthy subjects (n = 36) | Multiple doses (Phase 1) | NCT01281774 | Assessment of safety, tolerability, and PK/PD | CSL112 infusions lead to rapid dose-dependent rise in serum apoA-I and PC. All AEs were mild to moderate and resolved by end of reporting period, no clinically relevant or dose-dependent increase in ALT, AST, bilirubin, deaths or SAEs | |||
Tricoci et al.15 | Subjects with stable atherosclerotic disease (n = 45) | Single ascending dose (1.7, 3.4, or 6.8 g—Phase 2a) | NCT01499420 | Assessment of safety, tolerability, and PK/PD | No clinically relevant elevations in AST, ALT. No SAEs. Rapid (Tmax ≈ 2 h) and dose-dependent increases in apoA-I (145% increase in the 6.8 g group) and total cholesterol efflux (up to 3.1-fold higher than placebo; P < 0.001) | |||
Tortorici et al.80 and Gille et al.96 | Healthy subjects with and without moderate renal impairment (n = 32) | Single ascending dose (2 and 6 g—Phase 1) | NCT02427035 | Assessment of safety, tolerability, and PK/PD | Moderate renal impairment did not impact the PK and PD of CSL112, and was well tolerated in these patients | |||
Gibson et al.16 | Subjects with acute coronary syndrome (n = 1258) | AEGIS-I study multiple doses (2 and 6 g—Phase 2b) | NCT02108262 | Assessment of safety, tolerability, and PK/PD | No association with CSL112 and alterations in either liver or kidney function. Dose-dependent elevation in both apoA-I and CEC. CSL112 2 g: 1.29-, 1.87-, and 3.67-fold elevations in apoA-I, and total and ABCA1-dependent CEC, respectively. CSL112 6 g: 2.06-, 2.45-, and 4.30-fold elevations in apoA-I, and total and ABCA1-dependent CEC, respectively | |||
Gibson et al.18 | AMI patients with stage 3 CKD | Multiple doses (6 g—Phase 2) | NCT02742103 | Assessment of safety, tolerability, and PK/PD | Well tolerated in patients with both AMI and moderate renal impairment | |||
Ongoing | Subjects with acute coronary syndrome, multivessel disease, high-risk population (n = 17 400) | AEGIS-II study (Phase 3) | NCT03473223 | Efficacy and safety of CSL112 in reduction of MACE | Estimated completion date: June 2022 |
Type of reconstituted HDL agent . | Formulation . | Particle size [nanometers (nm)] . | Citation . | Tested human population (n) . | Study type . | NCT identifier . | Primary outcome . | Results . |
---|---|---|---|---|---|---|---|---|
ProApoA1 | Recombinant human proapolipoprotein A-I/liposomes (1:1.25 molar ratio) | 7–30 | Carlson et al.82 | Subjects with low HDL cholesterol (n = 4) | Single dose (Phase 1) | –– | Effect of a single infusion of recombinant human proapolipoprotein AI liposomes (synthetic HDL) on plasma lipoproteins in patients with low HDL | Increased levels of serum apoA-I subsequent to infusion; half-life of apoA-I <24 h |
Eriksson et al.83 | Subjects with familial hypercholesterolemia (n = 4) | Single dose (Phase 1) | –– | Determination of role of apoA-I in reverse cholesterol transport and biliary excretion (fecal steroid excretion) | Increased fecal excretion of cholesterol (sterols and bile acids; mean increase, +39% and +30%, respectively) | |||
ETC-216 | Recombinant apoA-I Milano/palmitoyloleoylphosphatidyl choline (1:1 molar ratio) | 7–30 | Bisgaier et al.84 | Healthy subjects (n = 32) | Single dose (Phase 1) | –– | Pharmacokinetics, safety, and tolerability | Well tolerated |
Nissen et al.85 | ACS patients (n = 57) | Multiple doses (Phase 2) | –– | % change in atheroma volume by IVUS | Significant reduction in atheroma volume recorded. Two adverse events in high-dose group resulting in study withdrawal | |||
MDCO-216 | ApoA-I Milano/palmitoyloleoylphosphatidyl choline (1:1 molar ratio) | 7–30 | Kempen et al.91 | Healthy subjects (n = 24) and patients with stable coronary artery disease (n = 24) | Multiple doses (Phase 2) | –– | Effects on HDL subfractions | Dose-dependent increase in pre-β1, α1, and α2 HDL; decrease in α3 and α4 HDL |
Nicholls et al.92 | Subjects with acute coronary syndrome (n = 126) | MILANO-PILOT study (multiple doses—Phases 1 and 2) | NCT02678923 | IVUS assessed % change in atheroma volume, efficacy, pharmacokinetics, safety, and tolerability | A similar proportion of patients in the MDCO-216 and placebo group showed regression in plaque volume | |||
CER-001 | Recombinant apoA-I/egg derived sphingomyelin/dipalmitoyl-sn-glycero-3-phosphorylglycerol (1:2.7:0.1 molar ratio) | 7–13 | Keyserling et al.86 | Healthy subjects (n = 32) | Single dose (0.25–45 mg/kg—Phase 1) | –– | Pharmacokinetics, safety, and tolerability | Well tolerated |
Tardif et al.87 | ACS patients (n = 504) | CHI-SQUARE study (Phase 2) | NCT01201837 | IVUS-assessed change in total coronary plaque volume | CER-001 infusions did not reduce coronary atherosclerosis on IVUS and quantitative coronary angiography | |||
Hovingh et al.88 | Heterozygous familial hypercholesterolemia (n = 30) | MODE study (Phase 2) | NCT01412034 | MRI assessed % change in total carotid plaque volume | ApoA-I increased from 114.8 ± 20.7 mg/dL to 129.3 ± 23.0 mg/dL. After 24 weeks, mean vessel wall area decreased from 17.23 to 16.75 mm2 (P = 0.008) | |||
Nicholls et al.89 | ACS patients (n = 292) | CARAT study (Phase 2) | NCT02484378 | IVUS-assessed % change in coronary atheroma volume | CER-001 3 mg/kg did not reduce % atheroma volume vs. placebo (−0.09% vs. −0.41%; P = 0.15) | |||
Cerenis Therapeutics90 | Familial hypoalpha-lipoproteinemia (n = 23) | TANGO study (Phase 3) | NCT02697136 | 3TMRI-assessed change in mean vessel wall area of the carotid artery | Early study termination due to lack of efficacy | |||
CSL111 | Purified wild type apoA-I from human plasma complexed with phosphatidylcholine (soy source; 1:150 molar ratio) | 7–30 | Van Oostrom et al.93 | Subjects with type 2 diabetes (n = 7) | Single dose (80 mg/kg—Phase 1) | –– | Effect of raising HDL levels by intravenous infusion of rHDL on endothelial progenitor cells (EPCs) | ApoA-I increased from 1.2 ± 0.2 (baseline) to 2.8 ± 0.6 g/L (t = 7 h, P < 0.001); increase in the number of EPCs in blood (480±85 mL at baseline vs. 1060 ± 347/mL at t = 7d, P < 0.05) |
Patel et al.76 | Subjects with type 2 diabetes (n = 13) | Single dose (80 mg/kg—Phase 1) | –– | Effect of rHDL on anti-inflammatory properties of endogenous HDL and ex vivo CEC | VCAM and ICAM-1 inhibition proportional to increase in endogenous HDL (up to 25%, P < 0.01); reduced expression of CD11b and neutrophil adhesion 72 h post-rHDL infusion (P = 0.02); increased CEC 1–72 h post-rHDL (40–60%, P < 0.05) | |||
Shaw et al.94 | Patients with claudication scheduled for percutaneous revascularization (n = 20) | Single dose (80 mg/kg—Phase 1) | –– | Determination of acute changes in plaque characteristic following rHDL infusions | Lower VCAM-1 expression vs. placebo (28 ± 3% vs. 50 ± 3%; P < 0.05) and a reduction in lipid content in the plaque of HDL-treated subjects compared with placebo | |||
Tardif et al.97 | ACS patients (n = 183) | ERASE study multiple doses (Phase 2) | NCT00225719 | Determination of % change in atheroma volume, plaque volume, plaque characterization index assessed by IVUS and QCA | rHDL infusions resulted in no significant reductions in atheroma volume (−3.4% with CSL111 and −1.6% for placebo, P = 0.48); significant improvement in plaque characterization index (−0.0097 for CSL111 and 0.0128 with placebo, P = 0.01) | |||
CSL112 | Purified wild-type apoA-I from human plasma complexed with phosphatidylcholine (soy source; 1:50 molar ratio) | 7–13 | Gille et al.14 | Healthy subjects (n = 57) | Single ascending dose (Phase 1) | NCT01129661 | Quantification of rise in apoA-I, pre-β1 HDL, ABCA1-dependent and total CEC following CSL112 infusion | CSL112 infusions lead to rapid dose-dependent rise in serum apoA-I, HDL, LCAT activity, and 2.9-fold elevation in total CEC and ≤5.8-fold elevation in ABCA1-dependent CEC |
Easton et al.95 | Healthy subjects (n = 36) | Multiple doses (Phase 1) | NCT01281774 | Assessment of safety, tolerability, and PK/PD | CSL112 infusions lead to rapid dose-dependent rise in serum apoA-I and PC. All AEs were mild to moderate and resolved by end of reporting period, no clinically relevant or dose-dependent increase in ALT, AST, bilirubin, deaths or SAEs | |||
Tricoci et al.15 | Subjects with stable atherosclerotic disease (n = 45) | Single ascending dose (1.7, 3.4, or 6.8 g—Phase 2a) | NCT01499420 | Assessment of safety, tolerability, and PK/PD | No clinically relevant elevations in AST, ALT. No SAEs. Rapid (Tmax ≈ 2 h) and dose-dependent increases in apoA-I (145% increase in the 6.8 g group) and total cholesterol efflux (up to 3.1-fold higher than placebo; P < 0.001) | |||
Tortorici et al.80 and Gille et al.96 | Healthy subjects with and without moderate renal impairment (n = 32) | Single ascending dose (2 and 6 g—Phase 1) | NCT02427035 | Assessment of safety, tolerability, and PK/PD | Moderate renal impairment did not impact the PK and PD of CSL112, and was well tolerated in these patients | |||
Gibson et al.16 | Subjects with acute coronary syndrome (n = 1258) | AEGIS-I study multiple doses (2 and 6 g—Phase 2b) | NCT02108262 | Assessment of safety, tolerability, and PK/PD | No association with CSL112 and alterations in either liver or kidney function. Dose-dependent elevation in both apoA-I and CEC. CSL112 2 g: 1.29-, 1.87-, and 3.67-fold elevations in apoA-I, and total and ABCA1-dependent CEC, respectively. CSL112 6 g: 2.06-, 2.45-, and 4.30-fold elevations in apoA-I, and total and ABCA1-dependent CEC, respectively | |||
Gibson et al.18 | AMI patients with stage 3 CKD | Multiple doses (6 g—Phase 2) | NCT02742103 | Assessment of safety, tolerability, and PK/PD | Well tolerated in patients with both AMI and moderate renal impairment | |||
Ongoing | Subjects with acute coronary syndrome, multivessel disease, high-risk population (n = 17 400) | AEGIS-II study (Phase 3) | NCT03473223 | Efficacy and safety of CSL112 in reduction of MACE | Estimated completion date: June 2022 |
ABCA1, ATP-binding cassette transporter A1; ACS, acute coronary syndrome; ALT, alanine aminotransferase; AMI, acute myocardial infarction; apoA-I, apolipoprotein A-I; AST, aspartate transaminase; CEC, cholesterol efflux capacity; HDL, high-density lipoprotein; ICAM-1, intercellular adhesion molecule-1; IVUS, intravascular ultrasound; PD, pharmacodynamics; PK pharmacokinetics; rHDL, reconstituted high-density lipoprotein cholesterol; SAE, serious adverse event; Tmax, time to maximum concentration; and VCAM, vascular cell adhesion molecule.
The CSL112 manufacturing process involves the isolation of precipitate from pooled plasma.21,24 Upon reconstitution with water for injection, CSL112 is a pale yellow, clear to slightly opalescent, practically particle-free solution suitable for infusion.
Mechanism of action
To understand the mechanism of action of CSL112 and the potential benefit it may provide AMI patients, it is important to understand the function of human HDL, the pathways of cholesterol efflux, and HDL dysfunction in patients with AMI.
Cholesterol efflux
High-density lipoprotein has a critical role in reverse cholesterol transport, in addition to its anti-inflammatory, anti-oxidative, and endothelial protective functions.25–27 The apoA-I component of HDL is the major determinant of its functionality in terms of reverse cholesterol transport, and apoA-I-initiated cholesterol efflux from lipid-laden cells is the first step of reverse cholesterol transport.28 Recent mechanistic insights into cholesterol efflux have shown that the efflux of intracellular cholesterol from lipid-laden macrophages can be quantified and interpreted using in vitro assays of CEC. These assays utilize cell lines as donors of labelled cholesterol and measure its fractional release to various acceptors such as isolated apoA-I or patient plasma. This extracellular transport of cholesterol can occur via two energy-independent, bidirectional, passive pathways (by simple diffusion and mediated by scavenger receptor class B type 1 [SR-B1]), and two energy-dependent, unidirectional, active pathways mediated by the transport proteins adenosine triphosphate-binding cassette (ABC) A1 and G1.29
Adenosine triphosphate-binding cassette A1-driven cholesterol efflux, cholesterol efflux capacity, and acute myocardial infarction
Adenosine triphosphate-binding cassette A1-mediated cholesterol efflux is of special significance when it comes to AMI patients, since this protein is known to be highly upregulated in lipid-laden macrophages (foam cells) within atherosclerotic plaques.30 This increased expression results from activation of sterol-sensing nuclear transcription factors called liver X receptor-retinoid X receptor (LXR-RXR), which respond to the presence of excess cellular cholesterol in foam cells.31,32 LXR-RXR in turn drives transcription of genes such as ABCA1, which act to rid the cells of excess cholesterol.33,34 Therefore, the upregulation of ABCA1 transmembrane protein expression in atherosclerotic arteries allows this efflux pathway to potentially act as the major route for cholesterol efflux from foam cells in the presence of lipid-poor apoA-I.
A key property of ABCA1 is that it must have an acceptor in the surrounding fluid in order for it to export cholesterol from cells. Neither LDL particles nor mature, spherical HDL can serve as an acceptor; rather, ABCA1 is specific for lipid-poor apoA-I (also called pre-β1 HDL),28 and the availability of lipid-poor apoA-I is most likely the main limiting factor in the export of cellular cholesterol. The specificity of ABCA1 for lipid-poor apoA-I further explains the disconnect often seen between the plasma concentration of HDL-C and the level of CEC: Most of the cholesterol measured in a standard HDL-C assay resides in the large HDL2 and HDL3 species. Therefore, effective reverse cholesterol transport is dependent on a continual supply of cholesterol-poor pre-β1 HDL entering the circulation and acting as a cholesterol acceptor for ABCA1. However, this presents a challenge in patients’ post-AMI, as individuals with atherosclerotic CVD may have an accelerated clearance of endogenous apoA-I due to altered HDL metabolism and dysregulated lipolysis.35 In addition, the tissue damage and stress of an MI provoke a strong inflammatory response, which can impact CEC.36–39 The suppression of CEC coupled with an ongoing inflammatory process contributes to atherosclerotic plaque progression and disruption40–42 predisposing patients presenting with an AMI to a high risk of recurrent MACE.
CSL112 induces high-density lipoprotein remodelling
The primary benefit derived from infusion of CSL112 most likely relates to the generation of small and very small cholesterol-poor HDL sub-species, produced by spontaneous remodelling of endogenous HDL upon CSL112 infusion. These sub-species function as more potent acceptors of cholesterol than CSL112 itself (Figure 1).43 The generation of small lipid-poor HDL particles secondary to HDL remodelling induced upon infusion of CSL112 correlate with an increase in CEC, indicating that these small lipid-poor HDL particles may be responsible for the elevated capacity of plasma to induce cholesterol efflux via ABCA1. During the remodelling process, CSL112 fuses with native HDL particles, and the discoidal morphology (high surface-to-core ratio) of CSL112 causes subsequent fission leading to the generation of small lipid-poor pre-β1 HDL particles, which can now act as highly efficient acceptors of unesterified cholesterol from plaque. A further study of the remodelling process induced by CSL112 showed that nascent HDL discs are relatively poor acceptors of cholesterol, but the interaction between discs and larger HDL spheres upon CLS112 infusion leads to a more than additive elevation in CEC.44 This is reflected as a rapid dose-dependent increase in CEC upon CSL112 infusion [6 g CSL112 infusion producing a 2.45-fold elevation in total CEC (%/4 h) and a 4.30-fold elevation in ABCA1-dependent CEC (%/4 h)].16
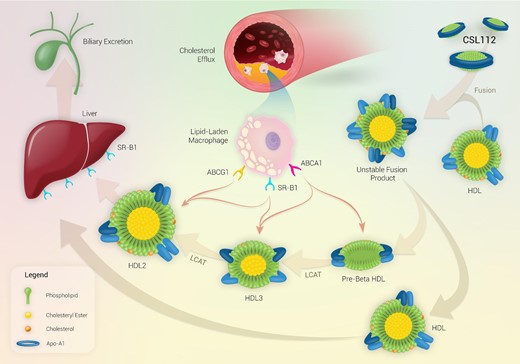
Mechanism of action of CSL112 and reverse cholesterol transport. Upon infusion, CSL112 fuses with native HDL and causes a rapid dose-dependent increase in lipid-poor pre-β HDL concentrations, which increases ABCA1 driven cholesterol efflux. CSL112 also causes an increase in the rate of LCAT-driven esterification of free cholesterol to form cholesteryl ester. The hydrophobic cholesteryl esters move to the interior of the HDL particle causing the pre-β HDL particles to form larger more mature spherical HDL3 and HDL2 particles which transport cholesterol to the liver for excretion in bile. Abbreviations: ABCA1/G1, ATP-binding cassette transporter A1/G1; HDL, high-density lipoprotein; HL, hepatic lipase; LCAT, lecithin-cholesterol acyltransferase; SR-B1, scavenger receptor class B type 1; and TG, triglycerides.
Role of CSL112 in lecithin-cholesterol acyltransferase activation and high-density lipoprotein maturation
ApoA-I has a critical role in the second step of reverse cholesterol transport since it increases the activity of lecithin-cholesterol acyltransferase (LCAT), which catalyses the esterification of free cholesterol.45 The docking of LCAT onto HDL particles brings it into close proximity of its substrates, free cholesterol and phospholipid. LCAT then catalyses the esterification of free cholesterol with a phospholipid-derived fatty acid to yield cholesteryl ester. The transformation of free cholesterol to the more hydrophobic cholesteryl ester ‘pushes’ the esterified cholesterol from the surface to the hydrophobic core of HDL particles, and particles must recruit further apolipoproteins to accommodate the increase in cholesterol esters.46 The end result is the generation of enlarged spherical alpha-HDL particles (HDL-2 and HDL-3) that transport cholesterol to the liver and steroidogenic tissues.47
LCAT activity and cholesterol esterification rate are both depressed following a myocardial infarction,48–50 but infusion of CSL112 leads to a robust increase in LCAT-driven esterification of cholesterol.21 Both the phospholipid and apoA-I components of CSL112 participate in this action since binding of LCAT to apoA-I is necessary for enzymatic activity,51 and the phospholipid component of CSL112 supplies substrate for the LCAT enzyme. Completing the process of reverse cholesterol transport, apoA-I plays a key role in the delivery of cholesterol to the liver. ApoA-I is a ligand for SR-B1 on hepatocytes,52 a bidirectional cholesterol transporter that is key to the selective uptake of esterified cholesterol from HDL into the liver. Therefore, apoA-I is a crucial player at three points during reverse cholesterol transport, supporting the utility of lipid-poor apoA-I infusions as a pharmacological approach in high-risk coronary artery disease.
Rapid cholesterol efflux and implications for plaque stability
A substantial portion of an atherosclerotic plaque consists of lipid-laden macrophages, as well as smooth muscle cells and monocytes.53,54 The characteristic rupture-prone plaque is a thin cap fibroatheroma (fibrous cap thickness of <65 μm) with a large necrotic core, irregular borders, and lipid-laden macrophage infiltration in the plaque shoulder.54,55 These lipid-laden macrophages forming the plaque lipid core sometimes referred to as foam cells (although non-monocytic origins are also possible) contribute to the low density observed on intravascular ultrasound and computed tomography of highly rupture-prone plaque.56–59 Biologically, rupture-prone plaque exhibits an increased activity of foam cell-produced matrix metalloproteinases, which cleave reinforcing collagen strands, degrade extracellular matrix, and increase macrophage infiltration, thus promoting inflammation and cap-shoulder thinning.60–62 In addition, accumulation of cholesterol in the smooth muscle cells, the major collagen-producing cell in an atheroma, may lead to apoptosis, thereby further decreasing the production of stabilizing collagen.63,64 Therefore, at the cellular level, promotion of mechanisms that increase cholesterol efflux, including CSL112 intervention, may serve to increase plaque stability and subsequently prevent plaque rupture and AMI. This is supported by animal studies demonstrating that infusion of human apoA-I increased plaque collagen content65 and decreased plaque metalloprotease activity.66
Impact of CSL112 on inflammation
Animal models have revealed that raising levels of functional, lipid-poor HDL, by genetic methods or infusion of reconstituted HDL, inhibits vascular inflammation and improves endothelial function.67–75 An early study with CSL111 showed anti-inflammatory effects in terms of reducing expression of vascular cell adhesion molecule-1, as well as monocyte CD11b, in 15 patients with diabetes.76 Later, in vitro studies mimicking the inflammatory milieu of human blood showed that CSL112 reduces expression of a leucocyte adhesion molecule known as intercellular adhesion molecule 1.21Ex vivo, a stronger inhibitory effect has been observed on interleukin-1β and tumour necrosis factor-α, thus pointing towards a possible downstream modulation of the NF-kB pathway and inflammasome, which are both implicated in the pathogenesis of atherosclerosis.43,77,78
Safety, tolerability, and pharmacokinetics/pharmacodynamics of ApoA-I-based intravenous infusions and CSL112
CSL112 clinical development program
Seven clinical trials of CSL112 have been completed, including four Phase 1 and three Phase 2 studies; a pivotal Phase 3 CV outcomes trial (AEGIS-II) is ongoing.14–16,18,20,79,80 These trials investigated the safety, pharmacokinetics (PK), and pharmacodynamics (PD) of CSL112 in healthy subjects, stable atherosclerotic disease, and AMI patients. Studies showed that CSL112 is well tolerated, with a good renal and hepatic safety profile. In Phase 2a and 2b trials, CSL112 infusion caused immediate dose-dependent elevation in plasma apoA-I levels and CEC.16,17 Little variation in response was seen between healthy subjects, patients with stable atherosclerotic disease, and those post-AMI. A study in AMI patients with moderate renal impairment showed that no dose adjustment was needed.18 Based on data from the Phase 2b (AEGIS-I) study, a 6-g dose was selected for further development due to positive PK/PD as well as safety and is associated with a 4.3-fold elevation in CEC in post-AMI patients. AEGIS-II (NCT03473223) is an ongoing, randomized, placebo-controlled trial to evaluate the efficacy of CSL112 on reducing the risk of MACEs (CV death, MI, or stroke) from randomization through 90 days in post-AMI patients.20
Summary of pharmacokinetics and pharmacodynamics of CSL112
The PK and PD of CSL112 have been consistent across the clinical development program. Infusion of CSL112 causes an immediate rise in plasma apoA-I concentration at all doses tested.14,15,79,81 Peak plasma apoA-I levels are dose-dependent, with maximum concentration reached at the end of the 2-h infusion, followed by a decline. After infusion of CSL112, apoA-I concentrations remain above baseline for ≥3 days. The mean steady‐state volume of distribution ranges from 5.6 to 9.7 L and is slightly greater than the plasma volume, indicating movement of CSL112 out of the plasma into the tissues and other fluid compartments.79 In addition, substantial increases in very small HDL particle number, total CEC and ABCA1-dependent CEC have been observed, respectively; increases in these parameters are also dose-proportional.14 There is no significant difference in the PK profile with respect to the dose-dependent elevations in apoA-I and CEC among patients with moderate renal impairment.18,19,80 Based on these findings and the renal and hepatic safety profile in the clinical development program, a schedule of four weekly, 2-h infusions of 6 g of CSL112 was chosen for investigation in the Phase 3 AEGIS-II trial.
Conclusion
The focus of novel HDL therapeutics has shifted from increasing circulating HDL concentration to improving HDL function, particularly cholesterol efflux, which is impaired among patients with CVD. CSL112, a novel formulation of apoA-I (human), enriches the intravascular compartment with lipid-poor cholesterol acceptors, which rapidly elevate efflux of unesterified cholesterol from macrophages abundant in atherosclerotic plaque to plasma HDL particles for transport to the liver and excretion. In post-MI patients, this process is hypothesized to lead to a rapid reduction in plaque lipid content, as well as a reduction in inflammation and oxidative stress, thereby promoting plaque stabilization. Early phase clinical trials have demonstrated the safety/tolerability and the consistent PK/PD profile of CSL112 and have set the stage for the assessment of the efficacy of the agent in an adequately powered, multicentre, global, Phase 3 randomized clinical trial in ∼17 400 patients with ACS (AEGIS-II, NCT03473223). The rationale and design of the AEGIS-II study have previously been published.20 The data from this Phase 3 study could represent a breakthrough in the treatment paradigm for AMI, particularly in the immediate weeks and months following the event when patients remain at high risk for recurrent ischaemic events.
Conflict of interest: S.K., S.H.A.K., G.C., A.K., J.J.L., A.T.P., and U.T. have no conflicts of interest to disclose. C.M.G., R.M., and P.M.R. have received research support from CSL Behring. B.K., S.D.W., and D.D. are employees of CSL Behring.
Data availability
No new data were generated or analyzed in support of this research.
References
Paramel Varghese G,