-
PDF
- Split View
-
Views
-
Cite
Cite
Ian Graham, Chuck Shear, Pieter De Graeff, Caroline Boulton, Alberico L Catapano, Wendy Gattis Stough, Stefan C Carlsson, Guy De Backer, Joseph Emmerich, Scott Greenfeder, Albert M Kim, Dominik Lautsch, Tu Nguyen, Steven E Nissen, Krishna Prasad, Kausik K Ray, Jennifer G Robinson, William J Sasiela, Karsten Bruins Slot, Erik Stroes, Tom Thuren, Bart Van der Schueren, Maja Velkovski-Rouyer, Scott M Wasserman, Olov Wiklund, Emmanouil Zouridakis, the European Society of Cardiology Cardiovascular Roundtable , New strategies for the development of lipid-lowering therapies to reduce cardiovascular risk, European Heart Journal - Cardiovascular Pharmacotherapy, Volume 4, Issue 2, April 2018, Pages 119–127, https://doi.org/10.1093/ehjcvp/pvx031
- Share Icon Share
Abstract
The very high occurrence of cardiovascular events presents a major public health issue, because treatment remains suboptimal. Lowering LDL cholesterol (LDL-C) with statins or ezetimibe in combination with a statin reduces major adverse cardiovascular events. The cardiovascular risk reduction in relation to the absolute LDL-C reduction is linear for most interventions without evidence of attenuation or increase in risk at low LDL-C levels. Opportunities for innovation in dyslipidaemia treatment should address the substantial risk of lipid-associated cardiovascular events among patients optimally treated per guidelines but who cannot achieve LDL-C goals and who could benefit from additional LDL-C-lowering therapy or experience side effects of statins. Fresh approaches are needed to identify promising drug targets early and develop them efficiently. The Cardiovascular Round Table of the European Society of Cardiology (ESC) convened a workshop to discuss new lipid-lowering strategies for cardiovascular risk reduction. Opportunities to improve treatment approaches and the efficient study of new therapies were explored. Circulating biomarkers may not be fully reliable proxy indicators of the relationship between treatment effect and clinical outcome. Mendelian randomization studies may better inform development strategies and refine treatment targets before Phase 3. Trials should match the drug to appropriate lipid and patient profile, and guidelines may move towards a precision-based approach to individual patient management. Stakeholder collaboration is needed to ensure continued innovation and better international coordination of both regulatory aspects and guidelines. It should be noted that risk may also be addressed through increased attention to other risk factors such as smoking, hypertension, overweight, and inactivity.
Introduction
Cholesterol levels in Western populations have declined over the last few decades.1 However, in many patients, they remain above the guideline recommended levels.2 For those at high risk of cardiovascular events, randomized clinical trial evidence has convincingly shown that lowering LDL cholesterol (LDL-C) to <70 mg/dL (1.8 mmol/L) with high-intensity statins [3-hydroxy-3-methylglutaryl-coenzyme (HMG-CoA) reductase inhibitors] or ezetimibe in combination with statins reduces the risk of major adverse cardiovascular events.1,3 Meta-analyses4,5 and clinical trial6,7 data suggest that this benefit may extend to ∼50 mg/dL (∼1.3 mmol/L) or lower. The reduction in cardiovascular risk appears to be linear in relation to the absolute reduction in LDL-C without any apparent evidence for a levelling off or of a J-curve at lower levels.4,7 However, only a minority of patients in major statin trials achieved low LDL-C levels (<50 mg/dL, 1.3 mmol/L),8,9 and while a greater proportion can achieve these levels with PCSK9 inhibitors, many patients remain at high risk despite low LDL-C levels. It is therefore not surprising that LDL-C-related cardiovascular events are still a major public health issue, even among patients receiving high-intensity statin therapy with and without additional therapy.
Innovations in the field of dyslipidaemia should address several areas of unmet need. First, there is substantial risk of cardiovascular events that remains even among patients receiving statin therapy whose LDL-C is <70 mg/dL.10 Second, effective strategies are still needed to lower LDL-C in patients who experience adverse effects on maximally tolerated statin therapy. New therapies might address both needs, but given the time and cost of developing, registering, and marketing these treatments, novel approaches are needed to reliably identify promising drug targets early and efficiently develop candidate drugs. Third, access to evidence-based therapies with proven benefit is problematic for many patients. Accordingly, better alignment between drugs with regulatory approval, payer decisions on reimbursement, and internationally recognized clinical guidelines are desirable goals.
The Cardiovascular Round Table (CRT) of the European Society of Cardiology (ESC) convened a 2-day workshop (27–28 September 2016) to discuss new lipid-lowering strategies for the reduction of cardiovascular risk beyond those currently available. This article summarizes the key discussions from the workshop and provides an overview of the evidence base for lipid lowering to reduce cardiovascular risk, identifies new opportunities to improve treatment approaches, considers strategies for the development of new therapies for novel targets based on accumulating knowledge, and calls for engagement of all stakeholders to promote continued innovation in the field and ensure access to safe and effective therapies that improve patient outcomes.
New strategies to extend the evidence base for LDL-C lowering
Although the international guidelines are complex and contain significant differences in tools used to assess risk, thresholds for treatment initiation, and application of LDL-C treatment goals (see Supplementary material online, Table S1),11 the extent to which these differences are clinically important remains uncertain. Different guidelines and treatment qualification standards can, however, present challenges for pharmaceutical companies planning global drug development programmes.
Notwithstanding some variation in the details, the guidelines consistently recommend intensive LDL-C lowering among patients at high or very high cardiovascular risk (see Supplementary material online, Table S1). Despite these recommendations, data suggest that LDL-C goals are achieved in <25% of very high risk and <40% of high-risk patients.2,12 Intolerance, non-adherence, and under-prescribing are all factors that contribute to the low proportion of patients reaching treatment goals. Thus, opportunities remain to improve the attainment of LDL-C goals in patients at risk of cardiovascular events.10
LDL-C lowering has dominated the dyslipidaemia field, primarily because it is the only parameter that has been well studied as a surrogate that explains the predominant effects of agents that up-regulate the LDL receptor and shown to reliably reflect cardiovascular outcomes in major randomized controlled clinical trials. However, in some patient subsets, the cardiovascular risk associated with LDL-C alone might be less than that associated with other lipid parameters (e.g. non-HDL-C or apolipoprotein B),13 although it is important to separate risk estimation from treatment response. Guideline recommendations necessarily focus on LDL-C as the target of therapy, because drugs that primarily lower LDL-C have been found to reduce cardiovascular events in major randomized controlled clinical trials,1,3 whereas drugs focused on raising HDL-C or lowering triglycerides have not. Investigators should aim to further the study of non-HDL-C, so future guideline iterations can comment on its importance.
Relative and absolute cardiovascular risk
Relative cardiovascular risk declines as LDL-C is decreased, and, while theoretically a ‘floor’ exists, the level at which LDL-C reduction does not further reduce risk has not been defined.4,5 Reduced progression and regression of atherosclerosis has been demonstrated with high-intensity statin therapy, and it is proportional to the degree of LDL-C lowering.14,15 Recent evidence has also shown that treatment with the PCSK9 inhibitor evolocumab compared with placebo, on the background of statin therapy, resulted in lower LDL-C levels (36.6 vs. 93 mg/dL, P < 0.001), a greater decrease in percent atheroma volume (PAV) over 78 weeks of treatment and a larger proportion of patients with PAV regression.16 The recent Further Cardiovascular Outcomes Research with PCSK9 Inhibition in Subjects with Elevated Risk (FOURIER) trial showed that evolocumab improved cardiovascular outcomes (hazard ratio 0.85, 95% confidence interval 0.79–0.92; P < 0.001, for the composite of cardiovascular death, myocardial infarction, stroke, hospitalization for unstable angina, or coronary revascularization) compared with placebo in patients with clinically evident atherosclerotic disease on optimal lipid-lowering therapy (69% on high-intensity statin and 30% moderate intensity statin).7 An absolute difference in LDL-C of 50 mg/dL was achieved at 168 weeks between the evolocumab (median 30 mg/dL) and placebo group, demonstrating that cardiovascular risk reduction can still be achieved at lower LDL-C levels, without evidence of a benefit threshold or increased risk at the lowest quartile of baseline LDL-C.
Despite advances in therapy, many patients remain at increased cardiovascular risk. Developing new therapies that further reduce risk when added to optimal statin and other preventive therapies remains a challenge. Although the reduction in relative risk is proportional to the degree of LDL-C lowering, the reduction in absolute risk over a given period of time will be less at lower LDL-C levels (Figures 1 and 2).17 As absolute risk reductions decline, the number needed to treat (NNT) for a given time rises, as will the sample size for cardiovascular outcome trials with a fixed power to detect a constant relative risk reduction.18 This paradigm increases the time and cost of development and also has a secondary impact on cost-effectiveness, which becomes less with lowering absolute risk and higher NNTs for a constant relative risk reduction.
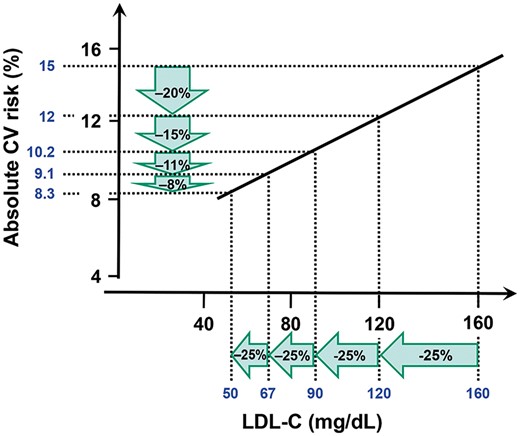
Diminishing risk reduction for the same relative LDL-C lowering with lower baseline LDL-C. Reprinted with permission from Laufs et al.17
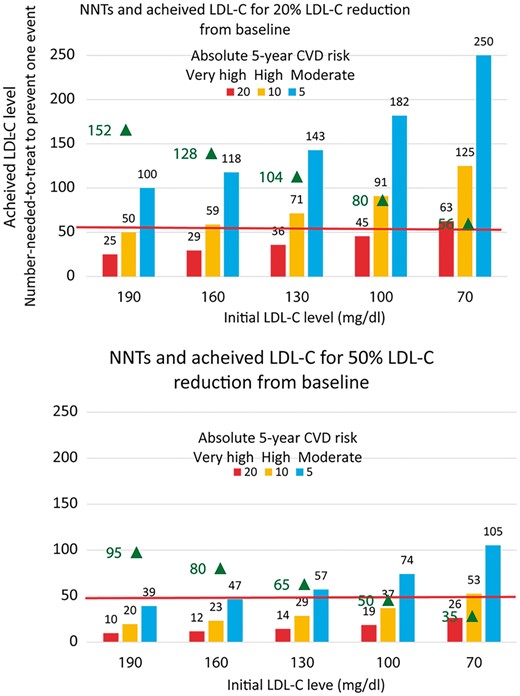
Achieved LDL-cholesterol levels (black triangle) when initial LDL-cholesterol levels of 190 mg/dL (4.9 mmol/L), 160 mg/dL (4.2 mmol/L), 130 mg/dL (3.4 mmol/L), 100 mg/dL (2.6 mmol/L), or 70 mg/dL (1.8 mmol/L) are reduced by 20% or 50%, and the expected number needed to be treated over a period of 5 years to prevent one cardiovascular event (NNT) for the anticipated relative reduction in the risk of cardiovascular events for a given level of absolute risk and magnitude of LDL-C lowering based on the Cholesterol Treatment Trialists meta-analysis (adapted from Cholesterol Treatment Trialists’ (CTT) Collaborators. Efficacy and safety of cholesterol lowering treatment: prospective meta-analysis of data from 90 056 participants in 14 randomized trials of statins. Reprinted with permission from Baigent et al.5 and Robinson et al. Eur Heart J 2016; 37: 1380–1383.
Net benefit considers baseline cardiovascular risk, baseline LDL-C, the expected LDL-C reduction and corresponding expected relative risk reduction.10 Larger reductions in LDL-C are needed to realize clinically meaningful reductions in cardiovascular risk at lower levels of baseline LDL-C. Thus, therapies that have modest effects on LDL-C may have insufficient impact on cardiovascular risk in this context.
It is acknowledged that clinical trials of new therapies in patients at high cardiovascular risk must be conducted against a background of usage of a moderate or high-intensity statin. Although there are clear ethical reasons for studying new therapies on top of statins, an add-on approach may not fully characterize potential efficacy; a new therapy could have advantages over existing therapy, or in specific patient subpopulations, that are difficult to detect in add-on trials. Further, while it is theoretically possible that some high-risk populations may not need a statin but could benefit from drugs that target other lipid pathways, this approach has not been studied. Add-on therapies also pose problems related to patient adherence with taking multiple drugs or doses and cost; less expensive or better tolerated LDL-C-lowering alternatives may be desirable. Thus, there is concern that studying new agents on top of moderate-/high-intensity statins creates challenges that may stifle innovations in the lipid field, such as the need for large trials, small absolute risk reductions, consequently higher NNT, uncertain acceptance in clinical practice, and second- or third-line placement in guideline recommendations.
Identifying niche indications could be another strategy. Placebo-controlled trials of a new agent could be performed in statin-intolerant patients, although statin intolerance in clinical practice is often subjective and many can tolerate some dose of a statin.19 Statin intolerance is not an easily recognizable entity from a regulatory standpoint, and clinically reported rates differ substantially from those reported in randomized controlled trials.20 Regulators acknowledge there is no consensus definition for statin intolerance, but the European Medicines Agency (EMA) guideline indicates ‘there should be documented evidence of intolerance due to emerging adverse events to 2 different statins administered in doses required to achieve the target LDL-C level’.21 A statement to provide guidance for the diagnosis and management of statin-associated muscle symptoms has also been issued by professional societies,22 but the absence of a standard definition may be used as justification to deny reimbursement post-approval. Conducting trials in patients with documented statin-intolerance is operationally difficult, in part due to the challenge of developing a rigorous definition and the lack of any objective tests. For example, only 43% of the patients randomized to the rigorous placebo-controlled crossover atorvastatin rechallenge phase of the Goal Achievement After Utilizing an anti-PCSK9 Antibody in Statin-Intolerant Subjects-3 (GAUSS-3) trial had recurrence of symptoms on atorvastatin but not placebo and were eligible to proceed further in the trial.23 This challenge to patient recruitment cannot be disregarded, especially for cardiovascular outcome trials where large sample sizes are generally needed.
Multiple risk factors contribute to cardiovascular disease; therefore, targeting other risk factors such as smoking cessation and improved management of hypertension in conjunction with LDL-C lowering is another strategy to achieve greater cardiovascular risk reduction in high- or very high-risk patients who are already treated with high-intensity statin.24 Such trials may be useful to inform guidelines but are less useful to support drug approval for a specific agent if it is difficult to ascertain which component of the strategy had the greatest influence on cardiovascular risk.
Duration and early initiation of therapy
As an alternative to add-on therapy, a strategy for earlier initiation of LDL-C-lowering therapy in lower risk individuals may be another approach to reduce cardiovascular events by reversing or preventing atherosclerosis.25,26 Ideally, a randomized controlled trial would be convened to answer the question of whether longer exposure to lower levels of LDL-C influences the development or progression of atherosclerosis. Such an approach would unfortunately likely be infeasible due to the requisite time and costs involved. Observational epidemiological studies are more feasible, but they are plagued by problems of confounding, reverse causation, and other biases. Thus, observational studies can only provide information on associations, not causality.27 As an alternative, the field has turned towards a method termed ‘Mendelian randomization’. Mendelian randomization studies use genetic variants that reflect the modifiable exposure of interest (e.g. genetic variants associated with LDL-C levels in this case) to make inferences about causality.28 Mendelian randomization studies offer advantages over observational epidemiological studies, because they group individuals according to the genetic variant of interest. This approach mimics randomization, because alleles are allocated randomly at conception, independently of other alleles. The random, independent, and non-modifiable nature of this genetic process makes Mendelian randomization studies generally free from the factors that would typically confound an observational study.27 Furthermore, although observational studies can only detect associations, the attributes of Mendelian randomization studies make them reasonably similar to prospective randomized trials, enabling assessment of causality.28
A meta-analysis of Mendelian randomization studies suggested that a very small lifelong increase in LDL-C may be associated with substantially increased risk of coronary heart disease.29 In this meta-analysis, there was no heterogeneity (I2 0.0%, P = 0.998) in the finding that exposure to single nucleotide polymorphisms (adjusted per 0.25 mmol/L lower LDL-C) were associated with a lower risk of coronary heart disease.29 This strategy reflects a ‘lower for longer’ concept29,30 that could mimic the low rates of coronary events for instance observed in people with low lifelong levels of LDL-C due to non-sense mutations in PCSK9.31
The legacy effect32 observed with statin therapy also provides support for the concept that achieving low LDL-C levels can have lasting beneficial effects, most specifically in primary prevention. In the 20 years of follow-up of the West of Scotland Coronary Prevention Study (WOSCOPS), reductions in all-cause mortality, cardiovascular mortality, and coronary mortality were observed for patients originally randomized to pravastatin vs. placebo.32 The number and rate of any cardiovascular hospitalization were lower for the pravastatin group compared with the placebo, as were cause-specific hospitalizations including myocardial infarction, heart failure, and coronary heart disease.32 The investigators did not observe an increased risk of overall or cause-specific cancers.32 This documentation of a long-term legacy effect following a relatively short-term randomized treatment could support more salutary cost–benefit relationships for expensive new preventive agents.25,26,33
The ‘lower for longer’ paradigm differs from the traditional approach to drug development. Drug outcome trials generally have a duration of about 5 years. The concept of a low-dose statin starting at an early age and continued for many years, although theoretically attractive and plausible, has not been tested and appropriate trials might be clinically and ethically challenging. From a regulatory standpoint, labelled indications would initially not likely reflect the legacy effect, which is related to the duration of follow-up rather than the treatment effect per se, but amendments could be made later. The results of clinical trials designed to evaluate the efficacy of achieving low LDL-C for long durations would also be reflected in clinical guidelines and could potentially impact reimbursement decisions.
Safety of very low LDL-C levels
Concerns have been raised about lowering LDL-C levels to very low levels,20,34,35 emphasizing the importance of assessing the safety of this approach. To address this concern, the biology of cholesterol should be considered. Intracellular cholesterol homeostasis is a finely regulated process that involves cholesterol synthesis and uptake. Most tissues derive their cholesterol needs by endogenous synthesis and can function independently of circulating cholesterol levels.36 Some tissues such as the brain remain independent of circulating cholesterol levels.37 Other tissues with a high demand of cholesterol such as the adrenal apparently preferentially utilize serum HDC cholesterol (HDL-C).38,39 In tissues that do utilize serum LDL-C, the threshold concentration required to support proliferation is low (<0.5 mg/dL), and other cholesterol sources such as HDL-C can be utilized if needed.40,41 In contrast to intracellular cholesterol, extracellular LDL-C is found in the circulation, where it plays a major role in atherosclerosis and its complications at excess or elevated levels.
The physiological level of LDL-C at 25 mg/dL was suggested in 1977 based on the work of Goldstein and Brown.42 Given the open questions regarding the relationship of low LDL-C levels to adverse effects, non-uniformity in lipid measurements across studies is a limitation to interpreting the findings of associations between low LDL-C and off-target adverse effects. The currently available evidence does not indicate that low circulating (i.e. extracellular) LDL-C portends excess risk, 7,20,43 but future studies will further clarify this important issue.
Expanding targets beyond LDL-C: strategies for further drug development
The successes with drugs that predominantly lower LDL-C by up-regulating the LDL receptor have made LDL-C reduction an accepted primary efficacy surrogate endpoint to explain the predominant effect of these drugs and support registration and a claim of lipid lowering in patients with hypercholesterolaemia, although a harmful effect on morbidity and mortality should be excluded before registration for new, non-statin therapies that lower LDL-C.21 These data can be generated either from a dedicated cardiovascular outcomes trial or using a meta-analytic/pooled approach.21,44
Changes in other parameters, for example triglyceride levels (and their associated remnant lipoproteins), HDL-C, apolipoproteins, lipoprotein(a), qualitative lipid abnormalities, or inflammation, have either not been tested or not consistently correlated with beneficial effects on cardiovascular outcomes. As a result, these measures are only acceptable as secondary endpoints for clinical trials from a regulatory point of view (see Limitations of biomarkers section).21 For drugs modifying lipid parameters other than LDL-C, demonstration of a positive clinical outcome in terms of morbidity and mortality is required for both market approval in Europe and for clinicians, as randomized, controlled, outcome trials form the basis for evidence-based guidelines.21 Importantly, region-specific requirements may vary regarding the size of the safety database or the need for local pharmacokinetic or pharmacodynamic data, which adds complexity for global trials.
Despite the association of some lipid parameters such as low HDL-C with cardiovascular risk in epidemiological population-based observational studies, Mendelian randomization studies have remained inconclusive,45 and many apparently promising pharmacological approaches did not prove effective or were confounded by off-target effects when tested in randomized controlled trials.
Limitations of biomarkers
As extensively reviewed elsewhere, a circulating biomarker may be associated with cardiovascular risk, but association does not necessarily imply causality and a biomarker’s association with outcome is insufficient to conclude that interventions that influence biomarker levels will also modify outcome.46,47 Lipoprotein-association phospholipase A2 (LP-PLA2) was a promising therapeutic target,48 but its inhibition did not improve outcomes despite a reduction in LP-PLA2 activity.49–51 Another well-known example is HDL-C, a marker that reflects reverse cholesterol transport and was associated with cardiovascular events.52 In the dal-OUTCOMES trial, dalcetrapib increased HDL-C to an extent that would be expected to exert favourable effects on clinical outcomes, but it did not reduce the risk of cardiovascular events.53
In addition to circulating biomarkers, functional (e.g. cholesterol efflux, antioxidant activity, anti-inflammatory activity, and proteomics) and imaging biomarkers are often employed later in Phase 2 to provide more evidence around the expected mechanism of drug effect. Functional biomarkers aim to reflect the factors leading to atherosclerosis progression or instability. Imaging biomarkers such as intravascular ultrasound provide information on atherosclerotic disease burden, atheroma volume, or plaque regression.54–56 Biomarkers other than LDL-C are not yet acceptable to obtain drug approval, but they can provide information relevant to the biological plausibility of a drug’s effect and partially inform drug development decisions, provided the data are interpreted with appreciation for its limitations.57
Selecting the correct target population
The risk of coronary heart disease increases with increasing triglyceride levels, but treatment of triglycerides with fenofibrate did not reduce the incidence of major cardiovascular events [non-fatal myocardial infarction (MI), non-fatal stroke, or cardiovascular death] in the Action to Control Cardiovascular Risk in Diabetes (ACCORD) trial.58 Subgroup analysis suggested a possible signal for benefit in patients with triglyceride levels ≥204 mg/dL and HDL-C ≤ 34 mg/dL. Although subgroup analyses are inherently limited and inconclusive,59 the subgroup finding was consistent with other studies.60,61 Thus, the lack of benefit observed in this trial may have been more closely related to the population studied, which overall did not have severely elevated triglyceride levels (median triglyceride 162 mg/dL, median HDL-C 38 mg/dL).58 A prospective, randomized trial conducted in a population similar to this subgroup would be necessary to confirm the findings observed in the subgroup analysis. Similarly, in the Outcome Reduction with an Initial Glargine Intervention (ORIGIN) trial and the Heart Protection Study 2–Treatment of HDL to Reduce the Incidence of Vascular Events (HPS2-THRIVE), the study populations did not reflect a population that would typically be treated with or expected to benefit from omega-3 polyunsaturated fatty acids (ORIGIN) or niacin (HPS2-THRIVE).62,63 These examples underscore the importance of carefully considering the target population when designing clinical trials of future lipid therapies.
Applying lessons learned to strategies for future drug development
Pursuing clinical trials that are aimed at targets not clearly causally associated with increased risk diverts limited resources away from research that might ultimately benefit patients. Reflecting on lessons learned may facilitate better decision-making and reduce the risk of late-phase failures in drug development. Less reliance on circulating biomarkers to identify treatment targets and predict efficacy is one example. Mendelian randomization studies64 or genome-wide association studies65,66 can be more informative than circulating biomarkers in identifying therapeutic targets and should be more widely integrated in drug development programmes for this purpose. Indeed, the finding from a Mendelian randomization analysis that secretory phospholipase A2 (sPLA2-IIA) was unlikely to be a suitable target to prevent cardiovascular outcomes64 was concordant with the results from a randomized controlled trial showing no benefit and potential harm with an inhibitor of sPLA2-IIA activity.51,64 More recent analyses showing a relationship between cardiovascular disease and ANGPTL367 and ATP citric lyase68 have yet to be validated with pharmaceutical-based randomized clinical outcome trials. Other markers, such as atherosclerosis imaging, while not acceptable as stand-alone endpoints for approval,21 can link a change in lipid parameters with an objective response (plaque regression), provide supportive evidence of efficacy, and reassure sponsors about moving towards Phase 3 clinical trials. As more data accrue that characterize the cardiovascular safety of new lipid-lowering therapies, stakeholders may consider whether Mendelian randomization studies with concordant imaging or other supportive data could provide some indication of safety and potentially replace the need for cardiovascular outcome studies in some circumstances such as for drugs in the same class as other agents shown to reduce cardiovascular risk, provided that safety data are sufficient to rule out a detrimental effect.21 This approach could be of considerable importance in patient populations too small to support a cardiovascular outcomes trial.
Cardiovascular clinical trials have traditionally enrolled broad patient populations in large, simple trials and have used an ‘intention-to-treat’ approach that will detect any overall net benefit. This approach is undoubtedly appropriate at the population level, but it poses challenges because, in any given trial, some patients may not be responsive to the therapy and, for a trial to demonstrate a positive effect overall, the treatment effect must be large enough to overcome the ‘noise’ of non-response. Thus, the size, complexity, cost, and risk of failure for conducting such trials have resulted in conversations about moving towards targeted populations with specific phenotypes (precision medicine).69,70 A more efficient strategy for dyslipidaemia clinical trials may be to focus initially on efficacy evaluation in a narrow target study population, enriched with the target of interest, for example a study population enriched with patients with high lipoprotein (a) levels for a therapy targeting lipoprotein (a) or to target combined familial hyperlipidaemia with raised tryglyceride level for a triglyceride-lowering drug. This may be followed by expansion to clinical trials in broader but still clinically appropriate populations. This will also depend on the indication claimed. The EMA’s pilot adaptive pathway programme was launched to potentially accelerate patients’ access to medicines and may also be applicable to the study of new lipid targets.71 More discussion among regulators, academia, learned societies, and sponsors as well as payers is needed to achieve consensus on whether and when broad or narrow study populations should be targeted in clinical trials.
Engaging payers as stakeholders
Payers are key stakeholders in the health care system, but the process of engaging them in the drug development process can be challenging because of the traditional stepwise paradigm of development, approval, prescribing, and reimbursement. Integrating payers earlier in the process may allow for pivotal clinical trials to be designed with additional endpoints that are meaningful from the payer perspective and drive reimbursement decisions, although payers may not be bound by advice provided during development.72 Reduction in subsequent cardiovascular events, health care utilization, and quality of life may better inform payer considerations of cost–benefit. Proactively addressing evidentiary requirements from payers may speed access of effective therapies to patients after approval, but drivers of reimbursement decisions are not transparent and delays or barriers to access could still occur. This early collaboration might improve consensus building in areas of ongoing debate such as identification of appropriate patients for additional cardiovascular risk reduction therapies, including LDL-C reduction, definition of statin intolerance, and expectations for ‘real-world’ statin use.
Conclusions
LDL-C lowering consistently reduces the risk of cardiovascular events, but events still occur even in patients on maximally tolerated statin therapy. Thus, there remain opportunities to further reduce cardiovascular events in high-risk patients, perhaps through achieving lower LDL-C levels and for a longer duration, or through new non-LDL-C targets. Genetic and subgroup analyses suggest that cholesterol remnants and lipoprotein (a) appear to be promising lipid species for therapeutic intervention.73,74 Careful attention should be placed on the lessons learned from the past major lipid trials to increase the likelihood of identifying effective therapies that can improve patient outcomes.
Current regulatory guidance reflects contemporary thinking regarding drug development, and the requirements are unlikely to be modified based on the present evidence. However, regulatory authorities are willing to consider adaptations in the guidance as new data emerge. In addition to planning clinical trials necessary to obtain marketing authorization, collaboration among researchers, regulators, clinicians, patients, and payers is essential to better align objectives, promote efficiencies, encourage recruitment in clinical trials, and ensure patients have access to effective and safe therapies.
Supplementary material
Supplementary material is available at European Heart Journal—Cardiovascular Pharmacotherapy online.
Acknowledgements
This article was generated from discussions during a Cardiovascular Round Table (CRT) Workshop organized on 27–28 September 2016 by the European Society of Cardiology (ESC). The CRT is a strategic forum for high-level dialogues between ESC leadership, academia, and industry to identify and discuss key strategic issues for the future of cardiovascular health in Europe. Twenty-two industry organizations provide financial support for the ESC Cardiovascular Round Table.
The opinions expressed in this paper are those of the authors and cannot be interpreted as the opinion of any of the organizations that employ the authors.
Conflict of interest: I.G.: personal fees from MSD, Pfizer, Amgen and co-chair of the 2016 ESC/EAS Guidelines on the management of dyslipiaemias;
C.S.: Employee of Pfizer;
P.D.G.: none declared;
C.B.: employee of Novartis;
A.L.C.: grants, consulting fees, and/or honoraria and delivering lectures from Aegerion, Abbot, Amgen, BMS, Eli Lilly, Genzyme, Kowa, Merck, Novartis, Pfizer, Recordati, Roche, Sanofi, and Sigma-Tau;
W.G.S.: personal fees from consulting to European Society of Cardiology, Heart Failure Association of the European Society of Cardiology, Heart Failure Society of America, Overcome [Cardiovascular Clinical Trialists (CVCT) and Investigation Network Initiative-Cardiovascular and Renal Clinical Trialists (INI-CRCT)], Celyad, and Respicardia;
S.C.C.: employee of AstraZeneca R&D;
G.D.B.: none declared;
J.E.: none declared;
S.G.: full-time employee of Daiichi-Sankyo;
A.M.K.: full-time employee of Pfizer, Inc. (pending patent Treatment with Anti-PCSK9 Antibodies, US Provisional Patent No. 62/093, 885);
D.L.: employee of Merck & Co., Inc., Kenilworth, NJ, USA;
T.N.: employee of Sanofi;
S.E.N.: research grants from Pfizer, AstraZeneca, The Medicines Company, Esperion Therapeutics, Amgen, Eli Lilly;
K.P.: none declared;
K.R.: research grants from Amgen, Sanofi, Regeneron, MSD, Pfizer and consultant for Amgen, Sanofi, Regeneron, MSD, Pfizer, AstraZeneca, Lilly, Medicines Company, Kowa, IONIS, Takeda, Novo Nordisk, Boehringer Ingelheim, Esperion, Cipla, Algorithm, Abbvie, Resverlogix, Cerenis;
J.G.R.: research grants to Institution from Amarin, Amgen, AstraZeneca, Eli Lilly, Esai, Esperion, GlaxoSmithKline, Merck, Pfizer, Regeneron/Sanofi, Takeda and consultant to Akcea/Ionis, Amgen, Dr Reddy, Eli Lilly, Esperion, Merck, Pfizer, Regeneron/Sanofi;
W.J.S.: Employee of Regeneron at the time of writing;
K.B.S.: none declared;
E.S.: lecturing fees paid to institution from Amgen, Sanofi/Regeneron, Chiesi;
T.T.: employee of Novartis Pharma AG;
B.V.S: none declared;
M.V-R.: employee of Merck & Co., Inc., Kenilworth, NJ, USA;
S.M.W: employee of Amgen, Inc. (as an employee, Scott Wasserman appears on multiple patents owned by Amgen, Inc. relating to evolocumab and PCSK9 inhibition);
O.W.: personal fees from Amgen, Sanofi, Merck;
E.Z.: none declared.
Appendix
European Society of Cardiology Cardiovascular round table participants
Ghislaine Clement-Baudena, Servier, Paris, France; Savion Gropper, Boehringer-Ingelheim Pharma GmBH & Co. KG, Ingelheim, Germany; Andrew Hamer, Amgen, Thousand Oaks, CA, USA; Bart Molemans, Amgen Europe, Zug, Switzerland; Timothée Sourdille, Sanofi, Paris, France; Arash Tahbaz, Eli Lilly, Basingstoke, UK; and Cathrine Thorstensen, Pfizer International Operations, Paris, France.
References
Author notes
Co-chairs.
The full list of all participants is given in Appendix.