-
PDF
- Split View
-
Views
-
Cite
Cite
Nicolas Argy, Solène Le Gal, Romain Coppée, Zehua Song, William Vindrios, Laurent Massias, Wei-Chun Kao, Carola Hunte, Yazdan Yazdanpanah, Jean-Christophe Lucet, Sandrine Houzé, Jérôme Clain, Gilles Nevez, Pneumocystis Cytochrome b Mutants Associated With Atovaquone Prophylaxis Failure as the Cause of Pneumocystis Infection Outbreak Among Heart Transplant Recipients, Clinical Infectious Diseases, Volume 67, Issue 6, 15 September 2018, Pages 913–919, https://doi.org/10.1093/cid/ciy154
- Share Icon Share
Abstract
Although trimethoprim-sulfamethoxazole is the more efficient drug for prophylactic and curative treatment of pneumocystosis, atovaquone is considered a second-line prophylactic treatment in immunocompromised patients. Variations in atovaquone absorption and mutant fungi selection after atovaquone exposure have been associated with atovaquone prophylactic failure. We report here a Pneumocystis jirovecii cytochrome b (cyt b) mutation (A144V) associated with such prophylactic failure during a pneumocystosis outbreak among heart transplant recipients.
Analyses of clinical data, serum drug dosage, and molecular modeling of the P. jirovecii Rieske–cyt b complex were performed to investigate these prophylactic failures.
The cyt b A144V mutation was detected in all infected, heart transplant recipient patients exposed to atovaquone prophylaxis but in none of 11 other immunocompromised, infected control patients not treated with atovaquone. Serum atovaquone concentrations associated with these prophylactic failures were similar than those found in noninfected exposed control patients under a similar prophylactic regimen. Computational modeling of the P. jirovecii Rieske–cyt b complex and in silico mutagenesis indicated that the cyt b A144V mutation might alter the volume of the atovaquone-binding pocket, which could decrease atovaquone binding.
These data suggest that the cyt b A144V mutation confers diminished sensitivity to atovaquone, resulting in spread of Pneumocystis pneumonia among heart transplant recipients submitted to atovaquone prophylaxis. Potential selection and interhuman transmission of resistant P. jirovecii strain during atovaquone prophylactic treatment has to be considered and could limit its extended large-scale use in immucompromised patients.
Pneumocystis pneumonia (PCP) is a life-threatening infection in immunocompromised patients undergoing solid organ transplantation, with an incidence varying from 5% to 15% according to the type of transplant [1–3]. Posttransplantation chemoprophylaxis is recommended for the prevention of PCP after solid organ transplantation for a period of 6–12 months [1, 2, 4], but the duration of chemoprophylaxis may vary according to the organ transplanted [2, 5]. The use of available markers to evaluate the immunosuppression status of transplant recipients and help define chemoprophylaxis guidelines has not yet been established [3–7].
Trimethoprim-sulfamethoxazole is the antimicrobial agent of choice for the prevention of PCP in immunocompromised patients [4, 8], but alternative drugs may be used [1, 2]. Among them, atovaquone, an analogue of ubiquinone that binds in the quinol oxidation (Qo) site of the mitochondrial cytochrome bc1 (cyt bc1) complex has shown effectiveness against Pneumocystis jirovecii [2, 4, 9, 10]. However, atovaquone absorption after oral administration varies among patients, and the drug must be taken with fatty meals to increase absorption [2, 4, 11]. In addition, atovaquone chemoprophylaxis failure has been associated with mutations in the mitochondrial cytochrome b (cyt b) gene (CYB) of P. jirovecii [4, 12, 13].
Vindrios et al [14] previously reported a series of 10 heart transplant recipients (HTRs) with contemporaneous development of P. jirovecii infections (7 patients with PCP and 3 with pulmonary colonization) at the heart transplant unit of Bichat–Claude Bernard hospital in Paris, France, from March through September 2015. Seven of these HTRs received atovaquone prophylaxis during this period (6 patients with PCP and 1 colonized patient). This putative outbreak was investigated by identifying P. jirovecii genotypes at 3 loci, the large subunit ribosomal RNA (mtLSU rRNA), the superoxide dismutase, and the CYB genes, and by analyzing patient contact. A transmission map combined with P. jirovecii genotype identification was drawn up, and this suggested person-to-person fungi transmission among 9 of the 10 patients [14]. It was noteworthy that these patients were infected with allele CYB2 [15], which also harbors a previously undescribed nonsynonymous mutation at nucleotide 350 (C350T) in CYB (GenBank accession No. KU521999) [14].
Because of the history of chemoprophylaxis with atovaquone in these patients, selection of a putative atovaquone-resistant strain of P. jirovecii was suspected. In this context, we characterized at the DNA and protein levels the molecular basis underlying atovaquone prophylaxis failure in P. jirovecii.
MATERIALS AND METHODS
Patients and P. jirovecii Isolates
Among the 10 aforementioned HTRs, 9 were clearly involved in the outbreak considering the results of patient encounter analysis combined with the positive P. jirovecii results of genotyping analysis. Clinical and biological data for each patient are shown in Table 1. The median age was 50 years (range, 35–69), and the male-female ratio was 6:3. PCP developed in 7 patients, and 2 patients were colonized by the fungus. Seven patients (6 patients with PCP and 1 colonized patient) were receiving PCP prophylaxis with atovaquone as an oral suspension (750 mg twice daily) when P. jirovecii infection was diagnosed. P. jirovecii was detected in respiratory specimens using a real-time polymerase chain reaction assay targeting the mitochondrial large subunit rRNA gene (Bio-Evolution).
Also enrolled in the study were 11 patients who had Pneumocystis infections contemporaneously diagnosed at Bichat–Claude Bernard hospital but were not hospitalized in the heart transplant unit. They represented a control group for P. jirovecii genotyping analysis (hereafter the “genotyping control group”). In addition, none of these non–HTR infected patients had a history of previous atovaquone prophylaxis. For these patients, the median age was 62 years (range, 44–72 years), and the male-female ratio was 7:4. Risk factors for P. jirovecii infection included human immunodeficiency virus infection (2 patients), liver transplantation (2 patients), cancer (3 patients), and corticosteroid treatment (4 patients). PCP developed in 9 patients, and 2 patients were colonized by the fungus. Final diagnoses in these 2 P. jirovecii colonized patients were idiopathic lung fibrosis and diffuse interstitial pneumonitis. DNA samples of pulmonary specimens were stored at −20°C for further analysis.
CYB Gene Sequencing
A 638–base pair fragment of the CYB gene was amplified using primers described by Esteves et al [15], and the 2 strands were sequenced with the dideoxy chain termination method on the 3130XL Genetic Analyzer (BigDye terminator v1.1 cycle sequencing kit; Applied Biosystems). Chromatograms were analyzed using Pregap and Gap software (Staden package, version 2003.0-beta; Staden Group). Consensus sequences were aligned with a reference sequence (GenBank accession No. AF074871) deposited by Walker et al [12] using BioEdit software version 7.2.5 [17] with the ClustalW program [18]. CYB alleles were named using the nomenclature described elsewhere [15, 16].
Serum Atovaquone Concentration in HTRs
Serum atovaquone concentration was determined as described elsewhere [19] in the 7 HTRs involved in the outbreak on the day of P. jirovecii infection diagnosis and in 4 noninfected HTRs. The 7 infected and 4 noninfected HTRs were given atovaquone prophylaxis at a dose of 750 mg twice daily [4, 14]. The 4 noninfected HTRs represented a control group for the atovaquone concentration determination (hereafter “prophylaxis control group”).
Atovaquone concentrations under the limit of detection (<0.5 µg/mL) were considered as negative for statistical analysis. An efficient steady-state concentration of atovaquone for PCP prophylaxis was defined as ≥15 µg/mL as described elsewhere [20, 21].
P. jirovecii Rieske–cyt b Complex Modeling
The amino acid cyt b sequences of Saccharomyces cerevisiae (strain S288C) and P. jirovecii (GenBank accession Nos. P00163 and AFV57373.1, respectively) were aligned using the program ESPriprt 3.0 [22–25]. The consensus sequence of cyt b refers to a comprehensive multiple sequence alignment of all available cyt b protein sequences (3595 sequences [22]). The degree of conservation for individual amino acid residues was derived from a nonredundant database [22]. The x-ray structure of cyt bc1 from S. cerevisiae at 3.0-Å resolution, with atovaquone bound in the catalytic Qo site, was downloaded from the Protein Data Bank (identifier 4pd4) [22]. Before modeling, protonation states of the ligand matching those at pH 6.9 were assigned using the Avogadro software [26].
Because the Qo site implies only the Rieske–cyt b complex, other cyt bc1 subunits were removed. The S. cerevisiae Rieske–cyt b complex was used as a template to reconstruct the P. jirovecii Rieske–cyt b complex, using Modeller 9v17 software [27], with ionized atovaquone bound in the Qo site and with the iron-sulfur cluster included in the homology model. One hundred models were created and then ranked with the discrete optimized protein energy method. Finally, mutation cyt b A144V was introduced in silico with the swapaa function in Chimera [25], which uses information from a rotamer library.
Statistical Analysis
Nonparametric variables were represented as medians with interquartile ranges. P. jirovecii genotyping in HTRs involved in the outbreak and non-HTR controls was compared using Fisher exact test, and serum atovaquone concentrations were compared done using Wilcoxon signed-rank test. Differences were considered significant at P < .05. Statistical analyses were performed using Stata software, version 12 (StataCorp).
RESULTS
CYB Genotyping
Positive results of P. jirovecii CYB genotyping were obtained in specimens from 9 of 10 HTRs involved in the outbreak (7 patients with PCP and 2 with pulmonary colonization) and 11 patients in the non-HTR genotyping control group. CYB2 allele was identified in the 9 HTRs and in 2 of the 11 genotyping control patients (P < .001; Table 1). Another previously unreported mutation on the CYB gene was found associated with the CYB2 allele in P. jirovecii isolates from HTRs. This mutation corresponds to a transition from C to T at nucleotide 431 (C431T) according to the whole gene sequence (corresponding to nucleotide position 350 in GenBank accession No. AF074871) and to an amino acid substitution from alanine to valine at codon 144 (A144V), located at the Qo site of the cyt bc1 complex. This mutation was not identified in any of the P. jirovecii isolates from the genotyping control group, which carried either CYB2 or non-CYB2 alleles (A144V mutation frequency: 9 of 9 HTRs vs 0 of 11 in the genotyping control group; P < .001).
Characteristics of 9 Heart Transplant Recipients and 11 Patients in the Genotyping Control Group Infected with Pneumocystis jirovecii a
Patient Code . | Age y . | Sex . | Type of Pulmonary Specimen . | Date of Pulmonary Specimen Retrieval (in 2015) . | Underlying Condition . | Clinical Presentation of Pneumocystis Infection . | Prophylaxis . | CYB Alleleb . |
---|---|---|---|---|---|---|---|---|
HTRs | ||||||||
HTR1 | 44 | M | BAL | 17 March | Heart and renal transplantation; HIV infection | PCP | Atovaquone | CYB2 (C350T)c |
HTR2 | 35 | F | Induced sputum | 12 May | Heart transplantation | PCP | Atovaquone | CYB2 (C350T)c |
HTR3 | 68 | M | BAL | 15 July | Heart transplantation | PCP | None | CYB2 (C350T)c |
HTR4 | 64 | M | BAL | 28 July | Heart transplantation | PCP | Atovaquone | CYB2 (C350T)c |
HTR5 | 50 | M | BAL | 6 August | Heart transplantation | PCP | Atovaquone | CYB2 (C350T)c |
HTR6 | 69 | F | BAL | 24 August | Heart transplantation | PCP | Atovaquone | CYB2 (C350T)c |
HTR7 | 68 | F | Nasopharyngeal swab | 31 August | Heart transplantation | Colonization | None | CYB2 (C350T)c |
HTR8 | 38 | M | BAL | 2 September | Heart transplantation | PCP | Atovaquone | CYB2 (C350T)c |
HTR9 | 45 | M | Nasopharyngeal swab | 2 September | Heart transplantation | Colonization | Atovaquone | CYB2 (C350T)c |
Genotyping control patients | ||||||||
C1 | 69 | F | Induced sputum | 8 January | Corticosteroid treatment | Colonization | None | CYB1 |
C2 | 53 | M | BAL | 22 January | Liver transplantation | PCP | None | CYB2 |
C3 | 53 | M | BAL | 26 January | HIV infection | PCP | None | CYB1 |
C4 | 65 | M | BAL | 5 February | Corticosteroid treatment | PCP | None | CYB1 |
C5 | 70 | F | BAL | 24 February | Corticosteroid treatment | PCP | None | Mixed infectiond |
C6 | 44 | F | Induced sputum | 9 March | HIV infection | PCP | None | CYB1 |
C7 | 68 | M | BAL | 21 March | Liver transplantation | PCP | None | CYB2 |
C8 | 66 | M | BAL | 31 March | Cancer | PCP | None | Mixed infectione |
C9 | 64 | M | BAL | 13 April | Cancer | PCP | None | Mixed infectionf |
C10 | 72 | F | Sputum | 5 May | Cancer | PCP | Cotrimoxazole | CYB6 |
C11 | 56 | M | BAL | 7 May | Corticosteroid treatment | Colonization | None | Mixed infectione |
Patient Code . | Age y . | Sex . | Type of Pulmonary Specimen . | Date of Pulmonary Specimen Retrieval (in 2015) . | Underlying Condition . | Clinical Presentation of Pneumocystis Infection . | Prophylaxis . | CYB Alleleb . |
---|---|---|---|---|---|---|---|---|
HTRs | ||||||||
HTR1 | 44 | M | BAL | 17 March | Heart and renal transplantation; HIV infection | PCP | Atovaquone | CYB2 (C350T)c |
HTR2 | 35 | F | Induced sputum | 12 May | Heart transplantation | PCP | Atovaquone | CYB2 (C350T)c |
HTR3 | 68 | M | BAL | 15 July | Heart transplantation | PCP | None | CYB2 (C350T)c |
HTR4 | 64 | M | BAL | 28 July | Heart transplantation | PCP | Atovaquone | CYB2 (C350T)c |
HTR5 | 50 | M | BAL | 6 August | Heart transplantation | PCP | Atovaquone | CYB2 (C350T)c |
HTR6 | 69 | F | BAL | 24 August | Heart transplantation | PCP | Atovaquone | CYB2 (C350T)c |
HTR7 | 68 | F | Nasopharyngeal swab | 31 August | Heart transplantation | Colonization | None | CYB2 (C350T)c |
HTR8 | 38 | M | BAL | 2 September | Heart transplantation | PCP | Atovaquone | CYB2 (C350T)c |
HTR9 | 45 | M | Nasopharyngeal swab | 2 September | Heart transplantation | Colonization | Atovaquone | CYB2 (C350T)c |
Genotyping control patients | ||||||||
C1 | 69 | F | Induced sputum | 8 January | Corticosteroid treatment | Colonization | None | CYB1 |
C2 | 53 | M | BAL | 22 January | Liver transplantation | PCP | None | CYB2 |
C3 | 53 | M | BAL | 26 January | HIV infection | PCP | None | CYB1 |
C4 | 65 | M | BAL | 5 February | Corticosteroid treatment | PCP | None | CYB1 |
C5 | 70 | F | BAL | 24 February | Corticosteroid treatment | PCP | None | Mixed infectiond |
C6 | 44 | F | Induced sputum | 9 March | HIV infection | PCP | None | CYB1 |
C7 | 68 | M | BAL | 21 March | Liver transplantation | PCP | None | CYB2 |
C8 | 66 | M | BAL | 31 March | Cancer | PCP | None | Mixed infectione |
C9 | 64 | M | BAL | 13 April | Cancer | PCP | None | Mixed infectionf |
C10 | 72 | F | Sputum | 5 May | Cancer | PCP | Cotrimoxazole | CYB6 |
C11 | 56 | M | BAL | 7 May | Corticosteroid treatment | Colonization | None | Mixed infectione |
Abbreviations: BAL, bronchoalveolar lavage; CYB, cytochrome b (cyt b) gene; HIV, human immunodeficiency virus; HTRs, heart transplant recipients; PCP, Pneumocystis pneumonia.
aPatients were monitored at Bichat–Claude Bernard hospital, Paris, France, and P. jirovecii isolates were examined at the CYB gene.
bCytochrome b alleles were named using nomenclature described elsewhere by Esteves et al [15] and Maitte et al [16].
cCYB2 (C350T) allele corresponds to allele CYB2, as described elsewhere by Esteves et al [15], but it harbors a previously undescribed mutation on position 350 (C350T).
dPatient C5 presented with a mixed infection related to association of the alleles CYB1 and CYB7.
ePatients C8 and C11 presented with mixed infections. The alleles were not identified because polymorphisms were observed at 2 nucleotide positions; that is, 2 peaks were observed on chromatograms at 2 different positions.
fPatient C9 presented with a mixed infection related to association of the alleles CYB1 and CYB2.
Characteristics of 9 Heart Transplant Recipients and 11 Patients in the Genotyping Control Group Infected with Pneumocystis jirovecii a
Patient Code . | Age y . | Sex . | Type of Pulmonary Specimen . | Date of Pulmonary Specimen Retrieval (in 2015) . | Underlying Condition . | Clinical Presentation of Pneumocystis Infection . | Prophylaxis . | CYB Alleleb . |
---|---|---|---|---|---|---|---|---|
HTRs | ||||||||
HTR1 | 44 | M | BAL | 17 March | Heart and renal transplantation; HIV infection | PCP | Atovaquone | CYB2 (C350T)c |
HTR2 | 35 | F | Induced sputum | 12 May | Heart transplantation | PCP | Atovaquone | CYB2 (C350T)c |
HTR3 | 68 | M | BAL | 15 July | Heart transplantation | PCP | None | CYB2 (C350T)c |
HTR4 | 64 | M | BAL | 28 July | Heart transplantation | PCP | Atovaquone | CYB2 (C350T)c |
HTR5 | 50 | M | BAL | 6 August | Heart transplantation | PCP | Atovaquone | CYB2 (C350T)c |
HTR6 | 69 | F | BAL | 24 August | Heart transplantation | PCP | Atovaquone | CYB2 (C350T)c |
HTR7 | 68 | F | Nasopharyngeal swab | 31 August | Heart transplantation | Colonization | None | CYB2 (C350T)c |
HTR8 | 38 | M | BAL | 2 September | Heart transplantation | PCP | Atovaquone | CYB2 (C350T)c |
HTR9 | 45 | M | Nasopharyngeal swab | 2 September | Heart transplantation | Colonization | Atovaquone | CYB2 (C350T)c |
Genotyping control patients | ||||||||
C1 | 69 | F | Induced sputum | 8 January | Corticosteroid treatment | Colonization | None | CYB1 |
C2 | 53 | M | BAL | 22 January | Liver transplantation | PCP | None | CYB2 |
C3 | 53 | M | BAL | 26 January | HIV infection | PCP | None | CYB1 |
C4 | 65 | M | BAL | 5 February | Corticosteroid treatment | PCP | None | CYB1 |
C5 | 70 | F | BAL | 24 February | Corticosteroid treatment | PCP | None | Mixed infectiond |
C6 | 44 | F | Induced sputum | 9 March | HIV infection | PCP | None | CYB1 |
C7 | 68 | M | BAL | 21 March | Liver transplantation | PCP | None | CYB2 |
C8 | 66 | M | BAL | 31 March | Cancer | PCP | None | Mixed infectione |
C9 | 64 | M | BAL | 13 April | Cancer | PCP | None | Mixed infectionf |
C10 | 72 | F | Sputum | 5 May | Cancer | PCP | Cotrimoxazole | CYB6 |
C11 | 56 | M | BAL | 7 May | Corticosteroid treatment | Colonization | None | Mixed infectione |
Patient Code . | Age y . | Sex . | Type of Pulmonary Specimen . | Date of Pulmonary Specimen Retrieval (in 2015) . | Underlying Condition . | Clinical Presentation of Pneumocystis Infection . | Prophylaxis . | CYB Alleleb . |
---|---|---|---|---|---|---|---|---|
HTRs | ||||||||
HTR1 | 44 | M | BAL | 17 March | Heart and renal transplantation; HIV infection | PCP | Atovaquone | CYB2 (C350T)c |
HTR2 | 35 | F | Induced sputum | 12 May | Heart transplantation | PCP | Atovaquone | CYB2 (C350T)c |
HTR3 | 68 | M | BAL | 15 July | Heart transplantation | PCP | None | CYB2 (C350T)c |
HTR4 | 64 | M | BAL | 28 July | Heart transplantation | PCP | Atovaquone | CYB2 (C350T)c |
HTR5 | 50 | M | BAL | 6 August | Heart transplantation | PCP | Atovaquone | CYB2 (C350T)c |
HTR6 | 69 | F | BAL | 24 August | Heart transplantation | PCP | Atovaquone | CYB2 (C350T)c |
HTR7 | 68 | F | Nasopharyngeal swab | 31 August | Heart transplantation | Colonization | None | CYB2 (C350T)c |
HTR8 | 38 | M | BAL | 2 September | Heart transplantation | PCP | Atovaquone | CYB2 (C350T)c |
HTR9 | 45 | M | Nasopharyngeal swab | 2 September | Heart transplantation | Colonization | Atovaquone | CYB2 (C350T)c |
Genotyping control patients | ||||||||
C1 | 69 | F | Induced sputum | 8 January | Corticosteroid treatment | Colonization | None | CYB1 |
C2 | 53 | M | BAL | 22 January | Liver transplantation | PCP | None | CYB2 |
C3 | 53 | M | BAL | 26 January | HIV infection | PCP | None | CYB1 |
C4 | 65 | M | BAL | 5 February | Corticosteroid treatment | PCP | None | CYB1 |
C5 | 70 | F | BAL | 24 February | Corticosteroid treatment | PCP | None | Mixed infectiond |
C6 | 44 | F | Induced sputum | 9 March | HIV infection | PCP | None | CYB1 |
C7 | 68 | M | BAL | 21 March | Liver transplantation | PCP | None | CYB2 |
C8 | 66 | M | BAL | 31 March | Cancer | PCP | None | Mixed infectione |
C9 | 64 | M | BAL | 13 April | Cancer | PCP | None | Mixed infectionf |
C10 | 72 | F | Sputum | 5 May | Cancer | PCP | Cotrimoxazole | CYB6 |
C11 | 56 | M | BAL | 7 May | Corticosteroid treatment | Colonization | None | Mixed infectione |
Abbreviations: BAL, bronchoalveolar lavage; CYB, cytochrome b (cyt b) gene; HIV, human immunodeficiency virus; HTRs, heart transplant recipients; PCP, Pneumocystis pneumonia.
aPatients were monitored at Bichat–Claude Bernard hospital, Paris, France, and P. jirovecii isolates were examined at the CYB gene.
bCytochrome b alleles were named using nomenclature described elsewhere by Esteves et al [15] and Maitte et al [16].
cCYB2 (C350T) allele corresponds to allele CYB2, as described elsewhere by Esteves et al [15], but it harbors a previously undescribed mutation on position 350 (C350T).
dPatient C5 presented with a mixed infection related to association of the alleles CYB1 and CYB7.
ePatients C8 and C11 presented with mixed infections. The alleles were not identified because polymorphisms were observed at 2 nucleotide positions; that is, 2 peaks were observed on chromatograms at 2 different positions.
fPatient C9 presented with a mixed infection related to association of the alleles CYB1 and CYB2.
Atovaquone Concentration in HTRs Receiving Atovaquone Prophylaxis
The serum concentration of atovaquone was obtained for 7 HTRs involved in the outbreak and 4 noninfected HTRs who served as an atovaquone control group. Median atovaquone concentrations did not differ significantly between HTRs on the day of PCP diagnosis (8.8 µg/mL; range, 0–13.1 µg/mL) and noninfected HTRs from the atovaquone control group (10.9 µg/mL; 5.9–18.8 µg/mL; P = .70). Only 1 infected HTR and 2 noninfected HTR controls had atovaquone concentration above the alleged effective steady-state concentration of 15 µg/mL (30.5, 15.8, and 21.8 µg/mL).
Rieske–cyt b Complex Modeling
The cyt b A144V mutation is located in the helix cd1 lining the Qo site of the cyt bc1 complex where atovaquone binds (Figure 1). A putative critical, functional role of position cyt b 144 was further supported by the high degree of consensus sequence conservation found for position 144 of cyt b (91%) across a large array of species, using a nonredundant database of 120 cyt b sequences. The consensus residue at position 144 was a highly conserved Ala, also harbored by P. jirovecii. Because in vitro culture is not possible for P. jirovecii, further assessment of cyt b mutations requires alternative functional and computational models [28–30].
![Partial alignment of Saccharomyces cerevisiae and Pneumocystis cytochrome (cyt) b amino acid sequences covering all residues that have side-chain interactions with atovaquone in the structure. Residues involved directly [20] and maybe indirectly (side chain distance to atovaquone, ≤6 Å) in atovaquone binding in S. cerevisiae cyt bc1 are shown below the sequences with asterisks and plus signs, respectively. Positions associated with atovaquone resistance in Pneumocystis jirovecii and in Plasmodium falciparum clinical isolates are indicated below the sequences with letters j and f, respectively. The new cyt b A144V mutation in P. jirocevii is shaded in black. Secondary structural elements are shown at the bottom, with helices as gray boxes and loops as dotted lines. Abbreviations: P. carinii, Pneumocystis carinii; P. murina, Pneumocystis murina.](https://oup.silverchair-cdn.com/oup/backfile/Content_public/Journal/cid/67/6/10.1093_cid_ciy154/1/m_ciy15401.jpeg?Expires=1749854237&Signature=OuC8Rn7ci9xZpCiITH5aQZ9UoySmqLDxrheS4zB~SoTK6ktoxlCGUlwiDy4q-u77Xtbfaf55IojHaLxFOrmVo-DjOEFmH3fVPCKcqJIBupyEvWjKdkYD~8~6C37x8WpsL3R96MFHJUFMx0Sr5cjyi2ynwLh67aZG9vF7yf~CVmu94HuekawfOCkt-ab2hH1pOdrZRxvYk9cEaSE8DJPm95mZKb5dttGR6nZ1fafNRIsDk1Fl87i2ul0OXhoIHrIinGQ7oecvb7V29c04yXjCZfpNlkQF-EBmUzi0VkA2em8FDbNTE0xeQR5VObLCVtmG~IKj0BFIzRq-7iYGiNjxUA__&Key-Pair-Id=APKAIE5G5CRDK6RD3PGA)
Partial alignment of Saccharomyces cerevisiae and Pneumocystis cytochrome (cyt) b amino acid sequences covering all residues that have side-chain interactions with atovaquone in the structure. Residues involved directly [20] and maybe indirectly (side chain distance to atovaquone, ≤6 Å) in atovaquone binding in S. cerevisiae cyt bc1 are shown below the sequences with asterisks and plus signs, respectively. Positions associated with atovaquone resistance in Pneumocystis jirovecii and in Plasmodium falciparum clinical isolates are indicated below the sequences with letters j and f, respectively. The new cyt b A144V mutation in P. jirocevii is shaded in black. Secondary structural elements are shown at the bottom, with helices as gray boxes and loops as dotted lines. Abbreviations: P. carinii, Pneumocystis carinii; P. murina, Pneumocystis murina.
We used computational modeling to evaluate the alteration of atovaquone-binding pocket resulting of the cyt b A144V mutation. The x-ray structure of the Rieske–cyt b complex from S. cerevisiae at 3.0-Å resolution, with ionized atovaquone bound in the catalytic Qo site [22], was used as a template to model the P. jirovecii Rieske–cyt b complex. We inspected alterations in the wild-type P. jirovecii modeled atovaquone-binding pocket, as compared with the S. cerevisiae template. We focused on 16 positions that interact directly with the drug in the structure of the yeast complex [22] and on 12 extra positions that might interact with the drug (corresponding to atom residues within ≤6Å of atovaquone; Figure 1 and Table 2).
Interatomic Distances for Binding Interactions Between Atovaquone and Rieske–Cytochrome b Complex for the Saccharomyces cerevisiae Template and the Pneumocystis jirovecii Model
Residue . | Interatomic Distance, Å . | |
---|---|---|
Saccharomyces cerevisiae Template . | Pneumocystis jirovecii Model . | |
Head group of atovaquone | ||
Met139 | 3.4 | 3.4 |
Gly143 | 3.7/3.7 | 3.7/3.7 |
Pro271 | 3.8/3.5/3.2 | 3.8/3.5/3.2 |
Ile269 | 3.4 | 3.2 |
Trp142 | 4.2 | 4.1 |
Phe129 | 3.3/3.7 | 3.8/4.6 |
Val146 | 3.5/3.9/3.9/3.6 | 3.6/4.1/4.3/4.1 |
Tyr279 | 3.7/3.6 | 3.9/3.9 |
Leu282 | 4.0 | 4.1 |
Arg283 [Trp]a | 7.0 | 2.7 (multiple contacts) |
His181 (Rieske) | 2.8 | 3.7 |
Tail of atovaquone | ||
Ile147 | 3.6/3.3 | 4.9/4.7 |
Leu275 | 3.5/3.8/3.8/3.6 | 4.3/4.7/4.7/4.4 |
Met295 | 3.9 | 3.7 |
Phe278 | 3.8 | 3.7 |
Ile299 | 3.2 | 5.3 |
Phe296 [Leu]a | 4.0 | 5.1 |
Ile122 [Leu]a | 5.3 | 3.7 |
Residue . | Interatomic Distance, Å . | |
---|---|---|
Saccharomyces cerevisiae Template . | Pneumocystis jirovecii Model . | |
Head group of atovaquone | ||
Met139 | 3.4 | 3.4 |
Gly143 | 3.7/3.7 | 3.7/3.7 |
Pro271 | 3.8/3.5/3.2 | 3.8/3.5/3.2 |
Ile269 | 3.4 | 3.2 |
Trp142 | 4.2 | 4.1 |
Phe129 | 3.3/3.7 | 3.8/4.6 |
Val146 | 3.5/3.9/3.9/3.6 | 3.6/4.1/4.3/4.1 |
Tyr279 | 3.7/3.6 | 3.9/3.9 |
Leu282 | 4.0 | 4.1 |
Arg283 [Trp]a | 7.0 | 2.7 (multiple contacts) |
His181 (Rieske) | 2.8 | 3.7 |
Tail of atovaquone | ||
Ile147 | 3.6/3.3 | 4.9/4.7 |
Leu275 | 3.5/3.8/3.8/3.6 | 4.3/4.7/4.7/4.4 |
Met295 | 3.9 | 3.7 |
Phe278 | 3.8 | 3.7 |
Ile299 | 3.2 | 5.3 |
Phe296 [Leu]a | 4.0 | 5.1 |
Ile122 [Leu]a | 5.3 | 3.7 |
aResidues in brackets are those in Pneumocystis jirovecii that differ from those in Saccharomyces cerevisiae.
Interatomic Distances for Binding Interactions Between Atovaquone and Rieske–Cytochrome b Complex for the Saccharomyces cerevisiae Template and the Pneumocystis jirovecii Model
Residue . | Interatomic Distance, Å . | |
---|---|---|
Saccharomyces cerevisiae Template . | Pneumocystis jirovecii Model . | |
Head group of atovaquone | ||
Met139 | 3.4 | 3.4 |
Gly143 | 3.7/3.7 | 3.7/3.7 |
Pro271 | 3.8/3.5/3.2 | 3.8/3.5/3.2 |
Ile269 | 3.4 | 3.2 |
Trp142 | 4.2 | 4.1 |
Phe129 | 3.3/3.7 | 3.8/4.6 |
Val146 | 3.5/3.9/3.9/3.6 | 3.6/4.1/4.3/4.1 |
Tyr279 | 3.7/3.6 | 3.9/3.9 |
Leu282 | 4.0 | 4.1 |
Arg283 [Trp]a | 7.0 | 2.7 (multiple contacts) |
His181 (Rieske) | 2.8 | 3.7 |
Tail of atovaquone | ||
Ile147 | 3.6/3.3 | 4.9/4.7 |
Leu275 | 3.5/3.8/3.8/3.6 | 4.3/4.7/4.7/4.4 |
Met295 | 3.9 | 3.7 |
Phe278 | 3.8 | 3.7 |
Ile299 | 3.2 | 5.3 |
Phe296 [Leu]a | 4.0 | 5.1 |
Ile122 [Leu]a | 5.3 | 3.7 |
Residue . | Interatomic Distance, Å . | |
---|---|---|
Saccharomyces cerevisiae Template . | Pneumocystis jirovecii Model . | |
Head group of atovaquone | ||
Met139 | 3.4 | 3.4 |
Gly143 | 3.7/3.7 | 3.7/3.7 |
Pro271 | 3.8/3.5/3.2 | 3.8/3.5/3.2 |
Ile269 | 3.4 | 3.2 |
Trp142 | 4.2 | 4.1 |
Phe129 | 3.3/3.7 | 3.8/4.6 |
Val146 | 3.5/3.9/3.9/3.6 | 3.6/4.1/4.3/4.1 |
Tyr279 | 3.7/3.6 | 3.9/3.9 |
Leu282 | 4.0 | 4.1 |
Arg283 [Trp]a | 7.0 | 2.7 (multiple contacts) |
His181 (Rieske) | 2.8 | 3.7 |
Tail of atovaquone | ||
Ile147 | 3.6/3.3 | 4.9/4.7 |
Leu275 | 3.5/3.8/3.8/3.6 | 4.3/4.7/4.7/4.4 |
Met295 | 3.9 | 3.7 |
Phe278 | 3.8 | 3.7 |
Ile299 | 3.2 | 5.3 |
Phe296 [Leu]a | 4.0 | 5.1 |
Ile122 [Leu]a | 5.3 | 3.7 |
aResidues in brackets are those in Pneumocystis jirovecii that differ from those in Saccharomyces cerevisiae.
Among those 28 positions, 3 exhibited different wild-type residues in P. jirovecii compared with S. cerevisiae sequences (positions 122, 283, and 296) (Figure 1), with varying impact on the P. jirovecii Qo site (Table 2). The P. jirovecii Leu122 residue induced a small constriction at the entry of the cavity (data not shown). The most striking change in the overall P. jirovecii model was the Pneumocystis-specific Trp283 residue (helix ef, lining the cavity) whose side chain unexpectedly pointed toward the cavity and faced the Rieske docking site (Figure 2A), at short distances of His181 from Rieske (3.0 Å) and of atovaquone (2.7 Å; Table 2). Trp283 also induced a small twist of both Tyr279 (helix ef) and His181 (Rieske) residues without altering the position of the iron-sulfur cluster (Figure 2B).
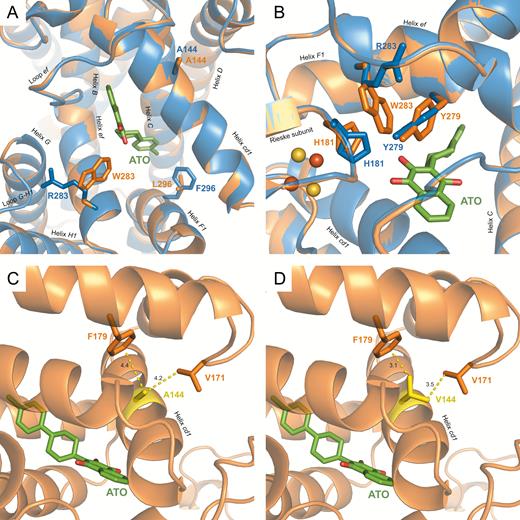
View of the modeled Pneumocystis jirovecii and reference Saccharomyces cerevisiae quinol oxidation sites and of the environment of residue 144 in P. jirovecii. The x-ray structure of S. cerevisiae cytochrome (cyt) bc1 with atovaquone bound (Protein Data Bank identifier 4pd4) in a deprotonated state was used as a template, and a model of the P. jirovecii Rieske–cyt b complex was obtained using the Modeller program. The model was checked for quality using the RAMPAGE server (http://mordred.bioc.cam.ac.uk/~rapper/rampage.php) and showed 95.7% and 3.2% for favored and allowed regions, respectively, and 1.1% for outliers. The P. jirovecii model overlapped to the template with excellent accuracy, as evidenced by the very low root-mean-square deviation of atomic positions of 0.25 Å. The P. jirovecii protein is shown in orange and the S. cerevisiae one in blue. Atovaquone (ATO) is shown in green. A, Detailed view of the binding site with side chains of cyt b residues 283 and 296 differing between S. cerevisiae and P. jirovecii. The Rieske subunit and cyt b I122L were hidden for clarity. B, View of the atovaquone-binding site showing R283W-induced local structural alterations; orange and yellow spheres correspond, respectively, to iron and sulfur atoms of the iron-sulfur cluster in the Rieske subunit. C, Wild-type cyt b Ala144 residue in the modeled P. jirovecii. D, Mutant cyt b Val144 residue associated with clinical atovaquone resistance in P. jirovecii. The mutant cyt b Val144 residue in the modeled P. jirovecii was generated with the function swapaa (in silico mutagenesis) in Chimera. Residue 144 is shown in yellow, residues involved in steric clashes with residue 144 are shown in orange, and interatomic distances are shown as yellow dashed lines.
Overall this resulted in a locally elongated surface of the P. jirovecii modeled terminal-binding cavity, with the ionized hydroxyl group of the naphtoquinone head group being closer to the surface cavity. However, slightly altered distances between atovaquone atoms and side chains of several other cyt b residues might compensate for Trp283-induced changes (Table 2). Concerning the P. jirovecii cyt b A144V mutation associated with atovaquone prophylaxis failures, the change for a bulkier Val144 residue in helix cd1 resulted in steric clash distances between the side chain of Val144 and those of Val171 (loop between cd2 and D helices) and Phe179 (helix D; distances of 3.5 and 3.1 Å, respectively; Table 2 and Figure 2C and 2D). As a consequence, we propose that helix cd1 may be pushed toward the atovaquone-binding pocket, decreasing the pocket volume and altering some of the stabilizing contacts contributed by cd1 helix residues that are important for atovaquone binding [22].
DISCUSSION
We have identified a novel nonsynonymous mutation (C350T) in the P. jirovecii CYB gene from a series of infected HTRs who were involved in a heart transplant unit–based outbreak and who received atovaquone prophylaxis. This mutation corresponds to an amino acid substitution from alanine to valine at position 144 (A144V) of cyt b, located at the Qo site of the cyt bc1.
The cyt b A144V mutation has been identified in all P. jirovecii isolates from HTRs involved in the outbreak, but it was not identified in the genotyping control isolates from patients who had contemporaneously diagnosed Pneumocystis infections in the same hospital but were not hospitalized in the heart transplant unit. Interestingly, 7 of the 9 HTRs involved in the outbreak were receiving atovaquone prophylaxis at the time of P. jirovecii infection diagnosis, whereas none of the patients in the genotyping control group had a history of previous atovaquone prophylaxis. Several other nonsynonymous mutations in the P. jirovecii CYB gene have been reported elsewhere as associated with atovaquone exposure, although these associations were not documented by serum drug dosages [12, 13].
In this study of patients with PCP, the association of prophylaxis failure with the presence of a CYB protein–altering mutation (A144V) and detectable serum atovaquone concentration is consistent with the hypothesis that this mutation confers diminished sensitivity to atovaquone in P. jirovecii. Nonetheless, 2 HTRs harboring this CYB mutation had no history of earlier atovaquone prophylaxis. This observation could be explained by circulation of the atovaquone-resistant P. jirovecii strain within the HTR population in the context of the outbreak [14], as already observed for the dihydropteroate synthase locus considered a circulation marker of P. jirovecii in humans [31–34].
Several hypotheses, not mutually exclusive, could explain the outbreak documented in 7 HTRs despite atovaquone prophylaxis. Serum atovaquone concentrations were lower than the reported effective steady-state concentration of 15 µg/mL [20, 21] in 6 of the 7 HTRs. These subeffective atovaquone concentrations could result from a low self-reported compliance with prevention treatment and/or poor atovaquone absorption if the drug was not taken with fatty meals, and they may explain P. jirovecii breakthrough despite chemoprophylaxis. However, in 1 HTR involved in the outbreak, P. jirovecii cyt b A144V mutant infection developed despite an effective atovaquone concentration of 30.5 µg/mL, and serum atovaquone concentrations did not differ significantly between infected HTRs and atovaquone-exposed noninfected HTRs. Altogether, these results support the hypothesis of a lower atovaquone sensitivity of P. jirovecii related to the cyt b A144V mutation. Although the origin of the cyt b A144V mutation is unknown, it is possible that subeffective atovaquone concentrations in some HTRs may have favored the nosocomial de novo acquisition of this mutation, which may have then enhanced the transmission of atovaquone-resistant P. jirovecii in the HTR population.
In addition to clinical data, we report here the first model of the P. jirovecii Rieske–cyt b complex, using as a template the x-ray structure at 3.0-Å resolution of S. cerevisiae cyt bc1 [22]. The modeling analysis suggests that the P. jirovecii cyt b A144V mutation in helix cd1 probably induces steric clashes with adjacent residues from loop cd2-D and helix D. Helix cd1, which lines the atovaquone-binding pocket, could then be pushed toward the pocket whose volume would decrease. This would largely alter the binding of the bulky head of atovaquone, which contains 2 aromatic cycles, whereas it would be less detrimental to substrate binding because the head of ubiquinol contains only 1 aromatic cycle.
To sum up, we suggest that the cyt b A144V mutation reduces the volume of the atovaquone-binding pocket in the P. jirovecii Rieske–cyt b complex, which would reduce the pharmacological efficiency of atovaquone against mutant P. jirovecii. A critical, functional role of an Ala at position 144 in the structure of the binding pocket is further supported by its high degree of conservation across species. In addition, it seems that CYB-mediated atovaquone resistance mutations in P. jirovecii tend to cluster mainly in helix cd1, with 4 other reported mutations in helix cd1 of cyt b (I147V, T148I, L150F, and S152A) in addition to A144V [28, 29]. We hypothesize that, in P. jirovecii, mutations in helix cd1 would be less detrimental to substrate binding and/or would destabilize more atovaquone binding than other Qo site mutations. We note that 2 other P. jirovecii atovaquone-resistant cyt b mutant residues in helix cd1 are also facing away from the atovaquone-binding pocket (T148I and S152A) [28, 29], such as A144V. These 3 mutant residues are not directly in contact with atovaquone and may alter the position, structure, or stability of the cd1 helix, and hence alter some of the stabilizing contacts with atovaquone that are contributed by the side of cd1 helix facing the pocket [22].
Importantly, our modeling of the P. jirovecii Rieske–cyt b complex also suggests that the atovaquone-binding cavity is expected to differ slightly between wild-type P. jirovecii and wild type S. cerevisiae, chiefly because the P. jirovecii Trp283 side chain (helix ef) points toward the terminal binding cavity. Other structural alterations were reported along the modeled P. jirovecii atovaquone-binding cavity, and some of these might compensate each other to accommodate native substrate binding, such as Phe129/Val146-mediated enlargement and His181/Trp283/Tyr279 constriction in the terminal binding cavity. However, these changes very likely affect the types and strengths of some contacts between atovaquone and the P. jirovecii Qo site, as compared with S. cerevisiae. Additional studies on the modeled P. jirovecii Qo site (such as ligand docking, molecular dynamics), in silico resistance prediction [30], and site-directed mutagenesis in S. cerevisiae [28, 29] would help in predicting and interpreting the consequences of these putative structural alterations in native substrate binding and atovaquone resistance.
In conclusion, despite the absence of in vitro model culture enabling resistance studies on P. jirovecii, the findings of our study combining clinical data and modeling of the P. jirovecii Rieske–cyt b complex strongly support the implication of the P. jirovecii cyt b A144V mutation in atovaquone prophylaxis failures during a PCP outbreak. The possible selection of atovaquone-resistant CYB mutated P. jirovecii strains after prolonged atovaquone exposition in patients without human immunodeficiency virus infection should be considered when atovaquone is used for PCP prophylaxis.
Notes
Acknowledgments. We thank Michèle Virmaux for her technical contribution.
Disclaimer. The funders had no role in study design, data collection and interpretation, or the decision to submit the work for publication.
Financial support. This work was supported by the European Commission and the ERANet-LAC program (grant CAPRI-PC HID-0254).
Potential conflicts of interest. All authors: No reported conflicts of interest. All authors have submitted the ICMJE Form for Disclosure of Potential Conflicts of Interest. Conflicts that the editors consider relevant to the content of the manuscript have been disclosed.
References
Author notes
Present affiliation: Service d’Immunologie Clinique et de Maladies Infectieuses, Hôpital Henri Mondor, APHP, Créteil, France
J. C. and G. N. contributed equally to this work.