-
PDF
- Split View
-
Views
-
Cite
Cite
Mie Mie Aung Naw, Makoto Moriya, Synthesis and ionic conductivity of organic ionic crystals consisting of quaternary phosphonium cations and cyclic perfluorosulfonylamide anion, Chemistry Letters, Volume 54, Issue 4, April 2025, upaf079, https://doi.org/10.1093/chemle/upaf079
- Share Icon Share
Abstract
Organic ionic species are promising candidate for innovative solid electrolyte matrices. In our previous work, we successfully synthesized quaternary ammonium salts exhibiting plastic crystalline behavior over a wide temperature range, specifically focusing on cyclic perfluorosulfonylamide (CPFSA) anion. However, reports on CPFSA-based organic ionic species remain scarce. Herein, novel phosphonium salts with CPFSA anion were synthesized to evaluate the structure–property relationship. Analogous ammonium salts were also synthesized to investigate how cation center elements affect the crystal structures, phase transitions, and ionic conductivities.

Solid-state organic ionic species, represented as organic ionic plastic crystals (OIPCs)1 and organic ionic crystals (OICs),2 are of growing attention for the development of solid electrolyte matrices. A distinction between OICs and OIPCs, which are both composed of organic cations and anions, is the crystal lattice orders; OICs have positional and orientational orders, whereas OIPCs maintain positional order, they forfeit orientational order and demonstrate dynamic behavior within the crystal lattice.
Recently, cyclic perfluorosulfonylamide anions have attracted considerable attention as a component of OICs and OIPCs because of their characteristic properties.3,4 These anions possess restricted conformational degrees of freedom due to its cyclic structure, while its electron-withdrawing groups contribute to its high dissociative properties. Previously, we reported novel OIPCs and OICs consisting of the cyclic perfluorosulfonylamide anion, [N(SO2CF2)2CF2]− (CPFSA) with 6-membered ring structure and quaternary ammonium cation.3 We found that the CPFSA anion contributes to the high melting point and moderate mechanical flexibility of CPFSA-based OIPC, compared with analogous compounds with bis(trifluoromethanesulfonyl)amide anion ([N(SO2CF3)2]−, TFSA) with linear structure.3 For instance, comparing [N(CH3)(CH2CH3)3][CPFSA] ([N1222][CPFSA]; Tm = 268 °C) and [N(CH3)(CH2CH3)3][TFSA] ([N1222][TFSA]; Tm = 96 °C) having a common tetraalkylammonium cation, the melting point of [N1222][CPFSA] is more than 150 °C higher than [N1222][TFSA].3a
Despite the appealing attributes of CPFSA anions as component moieties, their utilization in the synthesis of organic ionic compounds is limited. CPFSA-based OICs and OIPCs are reported mainly for ammonium, pyrrolidinium, and imidazolium salts.3,4 Although the phosphonium cations are structurally comparable to ammonium cations,5,6 the crystal structures, phase transition behaviors, and ionic conductive properties of CPFSA-based phosphonium salts are quite limited.7
From these perspectives, the present study explores the synthesis of novel OICs consisting of CPFSA anion and phosphonium cations. We have also synthesized CPFSA-based OICs with analogous ammonium cations for the comparison of the structural and physical characteristics. We herein report the structure–property relationship for novel CPFSA-based OICs with phosphorous cations, [P(CH2CH2CH2CH3)3R][N(SO2CF2)2CF2], (1; R = CH2CH2CH2CH3 ([P4444][CPFSA]), 2; R = CH3 ([P4441][CPFSA])) and analogous ammonium cations, [N(CH2CH2CH2CH3)3R][N(SO2CF2)2CF2], (3; R = CH2CH2CH2CH3 ([N4444][CPFSA]), 4; R = CH3 ([N4441][CPFSA])).
The OICs 1-4 were obtained following the methodology in previous paper.3 Initially, the appropriate quaternary phosphonium halides, or quaternary ammonium halides, and LiCPFSA were weighed in equimolar ratio, under Ar atmosphere. The resulting solid was dissolved in deionized water and mixed at ambient temperature to afford a white precipitate. The precipitate was washed 3 times with deionized water to remove any water-soluble contaminants, such as lithium halides, and then filtered to extract the crude product. The precipitate was vacuum-dried for 1 day. Subsequently, the dried precipitate was recrystallized from 2-propanol to yield a colorless crystalline solid. The crystals were purified 3 times by washing with n-pentane and then vacuum-dried for 1 day. The outlined procedure successfully yielded OICs 1-4 in approximately 70% yields.
The crystal structures of OICs 1-4 were elucidated by single-crystal X-ray diffraction analysis. Figure 1 and Supplementary Fig. S1 illustrates a pair of cations and anions consisting of phosphonium or ammonium cations and CPFSA anions. The detailed single crystal data for OICs 1-4 are described in Supplementary Tables S1 and S2. Focusing on cationic moieties, the average P-C bond lengths for 1 and 2 were calculated to be 1.79 Å, and the average N-C bond lengths of 3 and 4 were estimated to be 1.52 Å (Supplementary Tables S3–6). The average bond angles of C–P–C in cation moieties of 1 and 2 were estimated to be between 107.5 and 110.6 Å (Supplementary Tables S7 and S8). The average C–N–C bond angles in 3 and 4 were calculated to be between 106.81 and 112.08 Å (Supplementary Tables S9 and S10). The CPFSA anion of 1-4 adopted chair-conformation as corroborated by the CPFSA-based ammonium salt in the previous study (Fig. 1).3b,4b,7,8 These bond lengths and angles for CPFSA-based OICs 1-4 were also similar to those of the previous results for OICs containing [P4444],9 [P4441],10 [N4444],11 and [N4441]12 cations.
![Crystal structures of the obtained compounds: a) [P4444][CPFSA] (1), b) [P4441][CPFSA] (2), c) [N4444][CPFSA] (3), d) [N4441][CPFSA] (4). (C: gray, N: blue, O: red, F: green, S: dark yellow, P: Orange. Hydrogen atoms were omitted for clarity. Ellipsoid corresponds to 30% probability.).](https://oup.silverchair-cdn.com/oup/backfile/Content_public/Journal/chemlett/54/4/10.1093_chemle_upaf079/1/m_upaf079f1.jpeg?Expires=1748600727&Signature=VAwxyeEM~1xxfzRQnBIy6pqsyJ0--qkTPW5Mqr7i48RkFzRX2FquSPE3wJjExeILzEyPlv2nhQzjOjaxA~0jnLMxV2BJz7Ro5LuRWvZ~v-2Py6Iq6essTaTJAZ8fOKA5PktgiRx4gnHf7GLYLYL2HKsvuaIgQbTxkLF0nIpzOrPdx4oCTkI3ZBoMNhBV-gWTOJ4-CoKix0q~LhY7v29ZW3AXGpOvUVsCkYdmipbfH~8D1shaliP1ue9tnXpM2epNQRggorfCydacwr~zLa15fS7lGYQjm-PqETjhas6Cfo~FMi9iLsjSzCrygB59IPvuyCdTlgrCypjpERNz0MVV9w__&Key-Pair-Id=APKAIE5G5CRDK6RD3PGA)
Crystal structures of the obtained compounds: a) [P4444][CPFSA] (1), b) [P4441][CPFSA] (2), c) [N4444][CPFSA] (3), d) [N4441][CPFSA] (4). (C: gray, N: blue, O: red, F: green, S: dark yellow, P: Orange. Hydrogen atoms were omitted for clarity. Ellipsoid corresponds to 30% probability.).
The powder X-ray diffraction (PXRD) analysis results are depicted in Fig. 2. These results demonstrate that all crystals exhibit strong and sharp diffraction peaks. It indicates the high crystallinity of the obtained compounds 1-4. We also confirmed the PXRD patterns of 1-4 are almost identical to those of the simulated patterns obtained from single-crystal X-ray diffraction study (Supplementary Fig. S2).
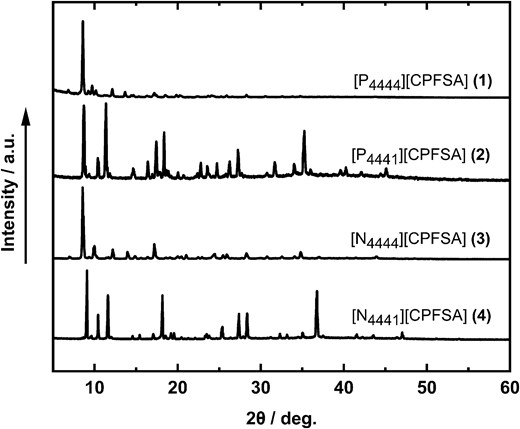
The phase transition behavior of 1-4 was evaluated by differential scanning calorimetry (DSC) from −50 to 300 °C. The detailed results are presented in Fig. 3. In the obtained OICs, 1 and 3 exhibited a single small endothermic peak at −6.7 and 31.4 °C, respectively, which corresponds to the solid-solid phase transition. The melting points of 1-4 were observed at 120.6, 105.8, 160.4, and 128.6 °C, respectively. Notably, CPFSA-based phosphonium salts 1 and 2 showed lower melting points than the corresponding ammonium salts 3 and 4, respectively. This behavior is attributable to the influence of central elements in the cation moieties.5,6 Furthermore, the entropy of fusion for 1-4 was calculated to be 66.0, 44.2, 66.3, and 61.6 J mol−1 K−1, respectively. Timmermans reported an empirical criterion of ΔSf < 20 J mol−1 K−1 as a definition of plasticity in molecular crystals.13 The values of ΔSf for 1-4 do not meet Timmerman's criteria. This result shows that compounds 1-4 preferred to exist in OICs.
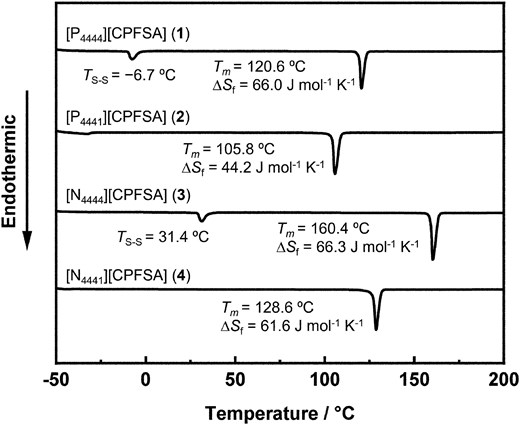
The ionic conductivities of 1-4 were measured using AC impedance measurements (Fig. 4). Compounds 1-4 exhibited the ionic conductivities in the crystalline phase. Similar activation energy (Ea) values were observed for the 4 compounds. The values of Ea for compounds 2-4 were calculated to be 129.4, 128.5, and 147.5 kJ mol−1, respectively. The CPFSA-based phosphonium salts (1 and 2) exhibited higher ionic conductivities than the corresponding CPFSA-based ammonium salts (3 and 4). It may be a reason that the bulkiness of the phosphorous ion compared to the ammonium ion contributes to reducing its cationic charge density and, as a result, lowering the electrostatic interactions between the counter-ions.5,6
![Ionic conductivities of 1-4. (1: [P4444][CPFSA], 2: [P4441][CPFSA], 3: [N4444][CPFSA], 4: [N4441][CPFSA]).](https://oup.silverchair-cdn.com/oup/backfile/Content_public/Journal/chemlett/54/4/10.1093_chemle_upaf079/1/m_upaf079f4.jpeg?Expires=1748600727&Signature=D53uBdZavK0RtkHVHad45nAHsA9J068w2HvxeZIWIu3e~JR~FWZ~ymjCHjZHln8u44ZrcwmeacOLtabw9SI4Q8aLI0ySM5~ZpdwawBcQOPSy9xSXQhvwCqnU-lAza~g6IFkqcdun5DwqZ1DmMcU1SAX-CwpilaCGu~S2JnAyY4N8FA45yEG3MBDRYiKVJPoZfqQfjTPoUfUCqbhdW~vs8mLEzKXJ5yRANwqwpoDcRr7t0Ri0tLb1iMRYnbpXncwsmGVRbOG3f-TnwgZapTStGMhb-cY9~9I56c0kND~7KYvc2GU6O7YB2vb0H57rDtUqDtBib6UdIIVb~jC7Snf-8g__&Key-Pair-Id=APKAIE5G5CRDK6RD3PGA)
Ionic conductivities of 1-4. (1: [P4444][CPFSA], 2: [P4441][CPFSA], 3: [N4444][CPFSA], 4: [N4441][CPFSA]).
The ionic conductivities of the OICs obtained in this study were observed at temperatures relatively close to their melting points. A similar behavior was reported for CPFSA-based OIPCs with cyano-substituted quaternary ammonium cations, [N(CH3)3(CH2CN)][CPFSA].3b This compound shows solid-state ion conductivity at temperatures slightly below the phase transition point from crystal to plastic crystal. Based on these results, it is suggested that the behavior of the OICs near the melting point appears to resemble that of plastic crystals, or they may undergo partial melting. It is suggested that the ion conductivity observed in the OICs within the crystalline phase at temperatures below the melting point may be due to the phenomena discussed above.
In conclusion, novel CPFSA-based OICs 1-4 were successfully synthesized using CPFSA anion and quaternary phosphonium or ammonium cations. The crystal structures of 1-4 were determined by single-crystal X-ray diffraction. We found that CPFSA-based phosphonium salts have lower melting points and exhibited higher solid-state ionic conductivities than the CPFSA-based ammonium salts.
Acknowledgments
We thank Editage for editing and reviewing the manuscript for English language.
Supplementary data
Supplementary material is available at Chemistry Letters online.
Details of preparations, characterizations, electrochemical measurements, and additional XRD, and NMR data of the CPFSA-based OICs are provided in the Supplementary Information. Crystallographic data reported in this manuscript have been deposited with Cambridge Crystallographic Data Centre as supplementary publication no. CCDC 2424184-2424187. Copies of the data can be obtained free of charge via CCDC Website. Instruction for depositing the crystallographic data is available on the Web at https://www.ccdc.cam.ac.uk/community/access-deposit-structures/deposit-a-structure/.
Funding
This work was supported by Japan Science and Technology Agency A-STEP (Adaptable and Seamless Technology Transfer Program through Target-driven R&D) (JPMJTR20TH) and CREST (Core Research for Evolutional Science and Technology) (JPMJCR22O4), and Research Institute of Green Science and Technology Fund for Research Project Support (2023-RIGST-23206) by National University Corporation, Shizuoka University.
References
Author notes
Conflict of interest statement. None declared.