-
PDF
- Split View
-
Views
-
Cite
Cite
Vincenzo Tufaro, Patrick Washington Serruys, Lorenz Räber, Martin Richard Bennett, Ryo Torii, Sophie Zhaotao Gu, Yoshinobu Onuma, Anthony Mathur, Andreas Baumbach, Christos Vasileios Bourantas, Intravascular imaging assessment of pharmacotherapies targeting atherosclerosis: advantages and limitations in predicting their prognostic implications, Cardiovascular Research, Volume 119, Issue 1, January 2023, Pages 121–135, https://doi.org/10.1093/cvr/cvac051
- Share Icon Share
Abstract
Intravascular imaging has been often used over the recent years to examine the efficacy of emerging therapies targeting plaque evolution. Serial intravascular ultrasound, optical coherence tomography, or near-infrared spectroscopy-intravascular ultrasound studies have allowed us to evaluate the effects of different therapies on plaque burden and morphology, providing unique mechanistic insights about the mode of action of these treatments. Plaque burden reduction, a decrease in necrotic core component or macrophage accumulation—which has been associated with inflammation—and an increase in fibrous cap thickness over fibroatheromas have been used as surrogate endpoints to assess the value of several drugs in inhibiting plaque evolution and improving clinical outcomes. However, some reports have demonstrated weak associations between the effects of novel treatments on coronary atheroma and composition and their prognostic implications. This review examines the value of invasive imaging in assessing pharmacotherapies targeting atherosclerosis. It summarizes the findings of serial intravascular imaging studies assessing the effects of different drugs on atheroma burden and morphology and compares them with the results of large-scale trials evaluating their impact on clinical outcome. Furthermore, it highlights the limited efficacy of established intravascular imaging surrogate endpoints in predicting the prognostic value of these pharmacotherapies and introduces alternative imaging endpoints based on multimodality/hybrid intravascular imaging that may enable more accurate assessment of the athero-protective and prognostic effects of emerging therapies.
1. Introduction
Coronary artery disease (CAD) is the most common cause of death in the developed world, is associated with increased morbidity, and has devastating economic consequences in Europe and in the USA. Therefore, an effort has been made to understand the pathophysiological mechanisms that regulate plaque progression and to develop effective therapies that will inhibit atherosclerosis evolution, improve quality of life, and prolong life expectancy in patients who suffer from CAD.1
Intravascular imaging, which enables detailed assessment of plaque pathology albeit with some limitations (Figure 1), has been used to examine the effect of these therapies on plaque burden (PB) and it has provided unique insights into the effects of these drugs on plaque morphology.2–4 In contrast to outcome trials that require large numbers of patients to prove prognostic benefit, intravascular imaging studies use imaging-based surrogate endpoints such as changes in per cent atheroma volume (PAV) or composition to evaluate their efficacy on plaque progression using a smaller number of patients and at a lower cost.5 Imaging-based studies have been performed to investigate the mechanisms of action of emerging therapies and provide proof of their athero-protective effect that would justify the conduction of large outcome studies, or to complement ongoing outcome studies with mechanistical insights on in vivo modes of action. Nevertheless, some reports demonstrated only a weak association between changes in PB or its characteristics and clinical outcomes, questioning the value of imaging-based endpoints in assessing the efficacy of novel treatments (see Supplementary material online, Figure S1).
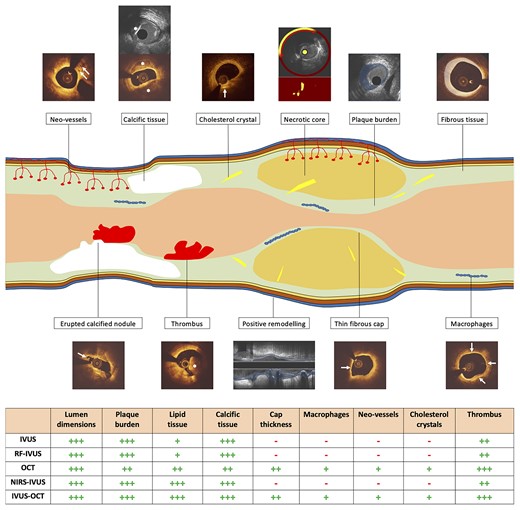
Advantages and limitations of the clinically available intravascular imaging modalities for assessing vessel wall pathology. The table at the bottom of the figure provides a quantification of the efficacy of each modality in detecting different tissue types using histology as the reference standard. The symbol (−) indicates that the modality is unable to detect the specific characteristic. Conversely, the symbols (+), (++), and (+++) indicate weak, moderate, and excellent ability of the modality to detect a plaque characteristic, respectively. White arrows indicate neo-vessels, cholesterol crystal, erupted calcified nodule, thin fibrous cap and macrophages infiltration in cross-sectional OCT images. Asterisks indicate calcific tissue on IVUS (upper panel) and OCT (lower panel) and evidence of intraluminal thrombus on OCT. A red arc defines the lipid necrotic core in NIRS-IVUS image. The superimposed light blue colour indicates plaque burden and positive remodelling on IVUS, while the ivory colour defines fibrous tissue on OCT. IVUS, intravascular ultrasound; NIRS, near-infrared spectroscopy; OCT, optical coherence tomography; RF, radiofrequency.
The aim of this review is to present the findings of intravascular imaging studies evaluating the efficacy of different drugs, summarize the results of clinical trials that tested their prognostic value, and discuss the advantages and limitations of invasive imaging endpoints in predicting the potency of these therapies in reducing cardiovascular event rates.
2. The rationale behind intravascular imaging for assessing the efficacy of novel therapies targeting atherosclerosis
The use of intravascular imaging-based surrogate endpoints to examine the value of novel pharmacotherapies in improving outcome relies on the premise that specific plaque characteristics, which can be assessed by intravascular imaging, are associated with a risk of subsequent major adverse cardiovascular events (MACE).
Cumulative data have shown that PB and its change provide useful prognostic information and identification of patients at risk of future events. A metanalysis including 4137 patients recruited in 6 clinical trials showed that baseline PAV and its change at 18–24 months follow-up were independent predictors of MACE.6
The above findings were also confirmed in prospective studies reporting patient-level results. In the European Collaborative Project on Inflammation and Vascular Wall Remodeling in Atherosclerosis-Intravascular Ultrasound (ATHEROREMO-IVUS) study that included 581 patients undergoing single vessel virtual histology (VH)-intravascular ultrasound (IVUS), patients with PB ≥ 70% and a thin-cap fibroatheroma (TCFA) phenotype were at high-risk of developing MACE.7 The prognostic value of plaque morphology was also shown in the ATHEROREMO-near-infrared spectroscopy (NIRS),8 the lipid-rich plaque (LRP)9 and the Providing Regional Observations to Study Predictors of Events in the Coronary Tree (PROSPECT) II10 studies which demonstrated that increased lipid component detected by NIRS was associated with worse prognosis on a patient-level analysis (Figure 2).
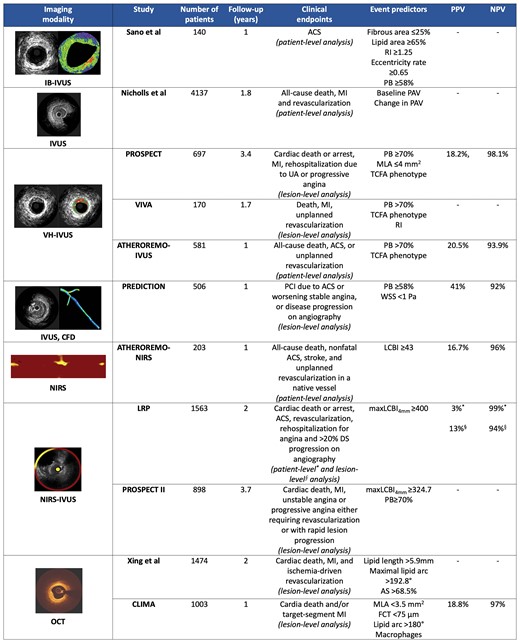
Design and outcome of studies assessing the efficacy of intravascular imaging in predicting events. ACS, acute coronary syndrome; AS, area stenosis; CFD, computational fluid dynamics; DS, diameter stenosis; FCT, fibrous cap thickness; IB, integrated backscatter; IVUS, intravascular ultrasound; LCBI, lipid core burden index; maxLCBI4mm, maximum LCBI in 4 mm segment; MI, myocardial infarction; MLA, minimum lumen area; NIRS, near-infrared spectroscopy; NPV, negative predictive value; OCT, optical coherence tomography; PAV, per cent atheroma volume; PB, plaque burden; PCI, percutaneous coronary intervention; PPV, positive predictive value; RI, remodelling index; TCFA, thin-cap fibroatheroma; UA, unstable angina; VH, virtual histology; WSS, wall shear stress.
Prospective large-scale studies which investigated clinical endpoints on a lesion-level analysis also provided relevant data on the value of intravascular imaging in identifying vulnerable plaques. The PROSPECT,11 the VH-IVUS in vulnerable atherosclerosis (VIVA),12 the Prediction of Progression of Coronary Artery Disease and Clinical Outcome Using Vascular Profiling of Shear Stress and Wall Morphology (PREDICTION),13 and the PROSPECT II10 have underscored the prognostic implications of PB and composition and highlighted the efficacy of intravascular imaging in detecting plaques that were prone to progress and cause events. In addition, the relationship between optical coherence tomography (OCT) coronary plaque morphology and clinical outcome (CLIMA)14 was the first study demonstrating that the thickness of fibrous cap over LRP and vascular inflammation indicated by the presence of macrophages on OCT imaging were independent predictors of MACE (Figure 2).
Based on the findings of the above studies and the evidence from histology reports showing that culprit lesions have a specific phenotype,15–17 it has been hypothesized that a decrease in PB, necrotic core and vascular inflammation as well as an increase in fibrous cap thickness (FCT) over LRP indicate plaque passivation and thus these variables can be used as surrogate endpoints to predict the efficacy of emerging therapies targeting atherosclerosis in reducing cardiovascular events.5
3. Efficacy of drug therapies in modifying plaque size and composition and improving outcomes
In this section, we focused our attention on pharmacotherapies, introduced to inhibit plaque evolution, that have been tested in intravascular imaging-based studies (see Supplementary material online, Tables S1 and S2) and/or outcome trials (see Supplementary material online, Table S3) in secondary prevention of CAD.
3.1 Statins
Statins target hepatocytes and are selective, competitive inhibitors of hydroxymethylglutaryl-CoA (HMG-CoA) reductase, a key regulator of cholesterol biosynthesis (see Supplementary material online, Figure S2). The reduction in intracellular cholesterol production causes upregulation of hepatic LDL receptors which decreases levels of circulating LDL as well as oxidized LDL within the arterial intima thwarting the inflammatory cascade that promotes monocyte recruitment and foam cell formation, the initial and key step in atherogenesis. Furthermore, statins have cholesterol-independent cardiovascular protective effects that include reduction of oxidative stress and platelet aggregation, vascular tone improvement (by increasing nitric oxide synthesis and reducing smooth muscle cell activation and proliferation), plaque stabilization (promoting macrocalcification and increase in FCT), as well as systemic and local anti-inflammatory effects [i.e. they reduce C-reactive protein (CRP), tumour necrosis factor (TNF) alpha, interleukin (IL)-1beta, and leucocyte-endothelial adhesion].18
The prognostic implications of statin therapy are well established and seem to be associated with the reduction in LDL-C induced by these drugs (see Supplementary material online, Figure S3). A meta-analysis including 21 statin trials and more than 129 000 patients showed that at every 1.0 mmol/L reduction in LDL-C there is a 22% reduction in cardiovascular events and a 10% reduction in all-cause mortality.19 In addition, numerous intravascular imaging studies have attempted to provide mechanistic insights and examine the effects of statin therapy on plaque morphology and burden.
3.1.1 Rosuvastatin
The ASTEROID (A Study to Evaluate the Effect of Rosuvastatin on Intravascular Ultrasound-Derived Coronary Atheroma Burden),20 a single-arm observational study, and the SATURN (Study of Coronary Atheroma by Intravascular Ultrasound: Effect of Rosuvastatin Versus Atorvastatin),21 an appropriately powered randomized control trial, showed that treatment with rosuvastatin 40 mg daily led to a marginal but statistical significant reduction in PAV (−0.98%, P < 0.001 and −1.22%, P < 0.001, respectively) on IVUS imaging at 2-year follow-up. An exploratory sub-analysis of the SATURN—including 71 patients undergoing serial VH-IVUS—demonstrated no changes in the lipid and fibrotic tissue components but an increase in the calcific and a reduction in the fibrofatty tissue burden at follow-up.22
The IBIS-4 (The fourth Integrated Biomarker and Imaging Study)4 confirmed that rosuvastatin 40 mg reduces PAV by −0.9% (P = 0.007) in patients with ST-elevation myocardial infarction (STEMI), while VH-IVUS analysis showed an increase in the calcific burden and a reduction of the fibrotic tissue component but no change in the plaque phenotype at follow-up. The OCT-sub-study of IBIS-4 demonstrated that this drug regimen may promote plaque stabilization increasing FCT over LRP by +24.4 μm (P = 0.008) and reducing macrophages accumulation at 13-month follow-up (see Supplementary material online, Table S2).3 An advantage of the IBIS-4 study is the fact that it studied the entire coronary tree including the culprit and non-culprit vessels with both VH-IVUS and OCT at two-time points to identify changes in plaque morphology; however, its main limitation is the lack of a control group that would allow us to test the superiority of this regimen over low-dose statin therapy.
Conversely, the IBIS-3 (The third Integrated Biomarker and Imaging Study)23 showed that rosuvastatin 40 mg did not change the necrotic core volume at 6 or 12-month follow-up (see Supplementary material online, Table S2). This was an observational single-arm study that aimed to recruit 300 patients but managed to enrol 241 patients. From these patients, 164 had evaluable serial VH-IVUS imaging, while 103 matched baseline and follow-up NIRS. The study was underpowered for the primary endpoint that was the change in the necrotic core volume at follow-up assessed by VH-IVUS. The secondary endpoint of the study was the change in the lipid core burden index (LCBI) of the studied segment assessed by NIRS, and the authors found no difference in the LCBI at follow-up; however, for this endpoint there was no power calculation. For the above reasons, the IBIS-3 findings should be interpreted with caution.
Multimodality IVUS and NIRS imaging was also used in the YELLOW (Reduction in Yellow Plaque by Aggressive Lipid LOWering Therapy) study24 to evaluate the short-term implications (6–8 week follow-up) of rosuvastatin 40 mg in flow-limiting lesions. Patients treated with this regimen exhibited a higher reduction in maxLCBI4 mm compared to the control group, but there were no changes in PB at follow-up (see Supplementary material online, Table S1). The small number of recruited patients, differences in plaque composition between groups and the need for NIRS and IVUS co-registration at two-time points raised concerns about the validity of the reported results. Furthermore, these findings were not confirmed in the YELLOW II study25 which had a similar design and demonstrated no difference in the maxLCBI4mm between baseline and follow-up. However, in the YELLOW II also serial OCT was used showing a significant increase in minimum FCT and decrease in the incidence of TCFA (see Supplementary material online, Table S2), but it was an exploratory analysis because the study was not powered for these endpoints.
Finally, the STABLE (Statin and Atheroma Vulnerability Evaluation) study26 which randomized patients with non-flow-limiting disease to high or moderate-dose of rosuvastatin showed a similar reduction in both groups in the per cent necrotic core volume and in the incidence of VH-IVUS-defined TCFA at 1-year follow-up (see Supplementary material online, Table S1). However, the study included only 225 patients with serial intravascular imaging instead of 276 and, thus, it was underpowered for the primary endpoint—that was the change in VH-defined per cent compositional volume within the target segment from baseline to follow-up—and for the secondary endpoint—defined as the change in per cent compositional volume at follow-up between the two treatment groups.
Studies investigating the prognostic implications of rosuvastatin in secondary prevention of CAD are limited and were performed only in patients with chronic heart failure. Two randomized studies27,28 including patients suffering from ischaemic or non-ischaemic heart failure failed to demonstrate a prognostic benefit of rosuvastatin in this cohort (see Supplementary material online, Table S3); however, a patient-level metanalysis29 of these two studies demonstrated that treatment with rosuvastatin is associated with a lower incidence of myocardial infarction (MI) in patients suffering from ischaemic heart disease (HR 0.81, 95% CI 0.66–0.99, P < 0.05); therefore, it is expected that rosuvastatin will be equally effective as the other statins in preventing MACE in patients with CAD.
3.1.2 Atorvastatin
Several studies have underscored the beneficial effects of intensive or moderate-dose atorvastatin therapy on PB. In the REVERSAL (Reversal of Atherosclerosis with Aggressive Lipid Lowering) study30 atorvastatin 80 mg daily inhibited disease progression, whereas there was plaque progression on IVUS in the pravastatin group at 18-month follow-up (total atheroma volume, TAV: −0.4 vs. +2.7%, P = 0.02). Conversely, there was no difference in PAV changes between the rosuvastatin and atorvastatin group in the SATURN 21 where atorvastatin 80 mg daily reduced PAV by −0.99% (P < 0.001) at follow-up.
Small randomized studies demonstrated that even low-dose atorvastatin may have beneficial effects on atheroma burden assessed by IVUS in patients with acute coronary syndrome (ACS)31 and in those with mild coronary atherosclerotic lesions (see Supplementary material online, Table S1).32
The Effect of Atorvastatin Therapy on Fibrous Cap Thickness in Coronary Atherosclerotic Plaque as Assessed by Optical Coherence Tomography (EASY-FIT) was the only prospective and properly powered study that compared the implications of two atorvastatin doses (20 mg vs. 5 mg daily) on FCT. This study showed that the higher atorvastatin dose significantly increased FCT at 12-month follow-up leading to plaque stabilization (69% vs. 17%, P < 0.001).33
The prognostic value of atorvastatin therapy in patients with CAD was first tested in the PROVE IT-TIMI22 (Pravastatin or Atorvastatin Evaluation and Infection Therapy-Thrombolysis in Myocardial Infarction 22) trial34 that randomized 4162 patients who suffered an ACS to atorvastatin 80 mg or pravastatin 40 mg and described a lower MACE rate in the atorvastatin arm (22.4% vs. 26.3%, P = 0.005) at 2-year follow-up. In patients with stable CAD, the TNT (Treating to New Targets) trial35 demonstrated that 80 mg/day of atorvastatin was more effective than 10 mg/day in reducing the risk of death from CAD, non-fatal MI, resuscitation after cardiac arrest or stroke over a median follow-up of 4.9 years (see Supplementary material online, Table S3).
3.1.3 Pravastatin and pitavastatin
The effects of pravastatin and pitavastatin on PB and composition have also been studied.
In the REVERSAL trial,30 treatment with pravastatin 40 mg was associated with an increase in TAV at follow-up as previously documented. The JAPAN-ACS (Japan Assessment of Pitavastatin and Atorvastatin in Acute Coronary Syndrome) study demonstrated that treatment with pitavastatin 4 mg/day was associated with a significant reduction in PAV in the treated culprit vessel at 8–12 month follow-up with no significant differences compared to a low-dose atorvastatin regimen.31 The TRUTH36 (treatment with statin on atheroma regression evaluated by IVUS with VH) was a small prospective study that randomized Japanese patients to pitavastatin or pravastatin and showed that both statin regimens modified plaque composition by reducing the fibrofatty and increasing the calcified plaque component assessed by VH-IVUS at 8-month follow-up.
The CARE (Cholesterol and Recurrent Events) trial examined the prognostic value of pravastatin in secondary prevention and showed that pravastatin 40 mg/day was more effective than placebo in reducing fatal and non-fatal coronary events (24% relative risk reduction, 95% CI: 9–36%; P = 0.003) in patients with ACS who had normal cholesterol.37 Similarly, the LIPID study (long-term intervention with pravastatin in ischaemic disease) showed that pravastatin reduced cardiovascular events and all-cause mortality compared to placebo in patients who had a previous ACS (see Supplementary material online, Table S3).38 Pitavastatin does not have an indication in secondary prevention, but it is approved for the treatment of primary hyperlipidaemia.
3.2 Ezetimibe plus statin
Ezetimibe is a lipid-lowering drug that targets the Niemann–Pick C1-like 1 protein and localizes in the brush border of the small intestinal enterocytes reducing the uptake of cholesterol into the enterocytes (see Supplementary material online, Figure S2) and its overall delivery to the liver, thereby promoting the synthesis of LDL receptors with a subsequent reduction of serum LDL-C. Apart from this systemic effect, recent studies suggested that ezetimibe also inhibits macrophages migration by decreasing VCAM-1, MCP-1, and TNF-α levels and reduces ROS levels that are instigators of plaque progression.39
When added to statins, ezetimibe reduces LDL-C levels by −22.3% compared to placebo40; therefore, ezetimibe is recommended as an add-on therapy in patients who do not reach the LDL-C goal with the maximum tolerated dose of statin.41
The PRECISE-IVUS (Plaque Regression With Cholesterol Absorption Inhibitor or Synthesis Inhibitor Evaluated by Intravascular Ultrasound) is the largest study investigating the effect of ezetimibe on PB; it randomized 246 patients to atorvastatin 10 mg plus ezetimibe 10 mg daily or atorvastatin alone and demonstrated a greater PAV regression (−1.4% vs. −0.3%, P = 0.001) in the dual-therapy group.42 A recently published meta-analysis pooling data from the PRECISE-IVUS study and five smaller studies comprising 583 patients in total confirmed that the combination of ezetimibe and statin therapy was more effective than statin monotherapy in reducing atheroma volume.43 Small scale studies examining the role of combined ezetimibe and statin therapy on plaque composition have consistently demonstrated that the addition of ezetimibe has no significant effect on the changes of plaque characteristics at follow-up (see Supplementary material online, Table S1).
The clinical benefit of combining ezetimibe and a statin was shown for the first time in the IMPROVE-IT (Improved Reduction of Outcomes: Vytorin Efficacy International Trial)44 which randomized 18 144 patients admitted with ACS to simvastatin 40 mg plus ezetimibe 10 mg once a day or simvastatin 40 mg monotherapy. A 6.4% relative risk reduction in the dual-therapy group was noted for the composite endpoint of cardiovascular death, non-fatal MI, unstable angina requiring rehospitalization, coronary revascularization or non-fatal stroke at 7-year follow-up (32.7% vs. 34.7%, P = 0.016). A similar trend was also reported in the HIJ-PROPER trial (Heart Institute of Japan Proper level of lipid lOwering with Pitavastatin and Ezetimibe in acute coRonary syndrome) that included 1734 patients with ACS and dyslipidaemia who were randomized to dual therapy with ezetimibe 10 mg and pitavastatin 2 mg daily or pitavastatin monotherapy; however, the difference in the event rate between groups was not statically significant as the study was underpowered for the primary endpoint (see Supplementary material online, Table S3).45
3.3 Proprotein convertase subtilisin-kexin type 9 inhibitors
Proprotein convertase subtilisin-kexin type 9 (PCSK9) inhibitors prevent the degradation of LDL receptors which in turn increase their number on the surface of hepatocytes and promote LDL clearance (see Supplementary material online, Figure S2); these drugs can reduce LDL-C by 45–60% if used alone or in combination with a statin.46,47 PCSK9 inhibitors may also have pleiotropic effects as it appears that PCSK9 is expressed by various cell types involved in atherosclerosis and it affects endothelial function, promotes smooth muscle cell migration, and exerts paracrine function on macrophages in the intima increasing the expression of pro-inflammatory cytokines and modifying the uptake of oxidized LDL.48
The GLAGOV (Global Assessment of Plaque reGression with a PCSK9 antibOdy as Measured by intraVascular Ultrasound)2 was the first trial that examined the effect of PCSK9 inhibitors on PB. The included patients were randomized to evolocumab plus high or moderate-intensity statin or statin monotherapy and had serial IVUS imaging in a single vessel with non-significant stenosis at baseline and at 76-week follow-up. In this study, the PAV decreased by −0.95% (P < 0.001) in the combined therapy group, while it remained unchanged in the statin monotherapy arm. A pre-specified sub-study of GLAGOV49 which included patients who had concomitant VH-IVUS imaging showed no differences in changes in plaque composition between groups (see Supplementary material online, Table S1).
Conversely, the ODYSSEY-J IVUS (Evaluation of Effect of Alirocumab on Coronary Atheroma Volume in Japanese Patients Hospitalized for Acute Coronary Syndrome With Hypercholesterolemia) trial failed to demonstrate any difference in the changes in PAV or TAV between patients treated with alirocumab and a statin (rosuvastatin ≥5 mg/day or atorvastatin ≥10 mg/day) and those receiving statin monotherapy at 36-week follow-up.50 However, this study had significant limitations: power calculation assumed a large % change difference in the normalized TAV between the two groups that led to the recruitment of a small number of patients; IVUS imaging was performed in both culprit and non-culprit vessels where it is likely the TAV to be different and thus introduce bias; ezetimibe was added in the control group in 40% of the patients during the follow-up period.
A recent randomized study51 including only 48 patients showed a reduction in the lipid index and macrophages grade and an increase in the FCT assessed by OCT in patients treated with alirocumab compared to those receiving statin, whereas an observational report52 of 53 patients showed a reduction in the maxLCBI4mm on NIRS-IVUS imaging in patients treated with PCSK9 inhibitors compared to those being on statin monotherapy. However, in the former study no power calculation was performed, while the latter included two different PCSK9 inhibitors and was not a randomized study. Therefore, both reports should be regarded as exploratory analyses and their findings require confirmation in the two large appropriately powered randomized control studies that are currently ongoing. The Imaging of Coronary Plaques in Subjects Treated With Evolocumab (HUYGENS; NCT03570697)53 trial aims to assess the effect of treatment with evolocumab on FCT in 164 patients admitted with an non-STEMI, while in the Vascular Effects of Alirocumab in Acute MI-Patients (PACMAN-AMI; NCT03067844)54 study serial NIRS-IVUS and OCT imaging has been performed in 300 patients with acute MI to assess the effect of alirocumab on plaque volume, lipid burden, and FCT.
Large outcomes trials reported that PCSK9 inhibitors combined with a statin therapy decrease the risk of MACE. In the FOURIER (Further Cardiovascular Outcomes Research with PCSK9 Inhibition in Subjects with Elevated Risk) trial, evolocumab reduced the rate of MI (3.4% vs. 4.6%, P < 0.001) and coronary revascularization (5.5% vs. 7%, P < 0.001) compared to placebo in statin-treated patients at a median follow-up of 2.2 years, without reducing cardiovascular mortality.55 Similarly, the ODYSSEY OUTCOMES (Evaluation of Cardiovascular Outcomes After an Acute Coronary Syndrome During Treatment With Alirocumab) trial that included patients with an ACS showed that the addition of alicorumab to high-dose statin therapy reduces the incidence of recurrent cardiovascular events at 2.8-year follow-up (see Supplementary material online, Table S3).56
3.4 Drugs increasing HDL cholesterol
3.4.1 HDL mimetics
HDL cholesterols have been a target for drug development because of their anti-atherogenic properties. Several HDL mimetic drugs have been developed by combining peptides and proteins with varying structures, all of which bind lipids found in HDL. Infusion of reconstituted HDL directly increases the number of functional HDL particles in the circulation and thus cholesterol efflux capacity,57 in other words it promotes the extraction of cholesterol from donor cells located in peripheral tissues—such as macrophages—and its transportation to the liver (see Supplementary material online, Figure S2).
HDL mimetic agents have attracted attention after animal studies highlighting their athero-protective effects.58,59 Over the last 20 years three different HDL mimetics (ETC-126, now called MDCO-216, CER-001, and CSL-111) have been evaluated in randomized control trials which enrolled patients with history of ACS and used invasive imaging surrogate endpoints to assess their value in inhibiting plaque progression. Apart from the first study that showed that 5-week ETC-216 infusion reduces PB on IVUS imaging,60 all the other trials demonstrated a neutral effect of HDL mimetics on PB (see Supplementary material online, Table S1).61–64 A possible explanation of this paradox is the small number of patients included in the first report that did not allow to draw safe conclusion; in addition, patients recruited in recent studies received contemporary treatment for atherosclerotic disease and had normal HDL-cholesterol levels which may limit the effect of HDL mimetics on plaque pathobiology. Whether infusion of HDL mimetics is effective in the context of strongly reduced HDL-cholesterol levels and impaired cholesterol efflux capacity remains unknown.
There is no current evidence on the role of HDL mimetics in preventing cardiovascular events. The efficacy and safety of CSL-112 in patients after an ACS is currently under investigation in the ApoA-I Event Reducing in Ischaemic Syndromes II (AEGIS II, NCT03473223) trial.65
3.4.2 Cholesterylester transfer protein inhibitors
Cholesterylester transfer protein (CETP) is a hydrophobic glycoprotein that is synthesized mainly in the liver and regulates the exchange of lipids between different lipoprotein particles. This process leads to a net mass transfer of cholesterol esters and triglycerides from non-atherogenic HDLs to ApoB100-containing lipoproteins such as very low-density lipoproteins (VLDLs) and LDLs that are pro-atherogenic (see Supplementary material online, Figure S2). Inhibition of this pathway eventually increases the content of cholesterol in HDL particles and the formation of larger HDL particles that are catabolized slower than the normal HDL.66
The role of these agents in modifying plaque size has been evaluated in the Investigation of Lipid Level Management Using Coronary Ultrasound to Assess Reduction of Atherosclerosis by CETP Inhibition and HDL Elevation (ILLUSTRATE) trial67 which randomized patients with evidence of mild to moderate CAD to atorvastatin alone and atorvastatin in addition to torcetrapib 60 mg daily and showed no differences in PAV changes between the two groups after 24 months (see Supplementary material online, Table S1).
Interestingly, the Investigation of Lipid Level Management to Understand its Impact in Atherosclerotic Events (ILLUMINATE) trial68 demonstrated that torcetrapib in association with atorvastatin significantly increased the risk of MACE and all-cause mortality compared to atorvastatin in patients with history of type 2 diabetes mellitus or previous cardiovascular disease. Conversely, anacetrapib—another CETP inhibitor—demonstrated promising results in reducing MACE,69 while evacetrapib had a neutral effect on MACE70 when compared to placebo in patients with cardiovascular disease (see Supplementary material online, Table S3). A possible explanation of the prognostic benefit of anacetrapib is the fact that, in contrast to the other CETP inhibitors, this medication not only enhances reverse LDL transport, but also reduces apolipoprotein b levels. On the other hand, evacetrapib and torcetrapib increase apoA1 in HDL subspecies containing apoC3 and other HDL subspecies associated with increased risk of CAD; these mechanisms may explain their lack of clinical benefits although they raise HDL.71
4. Anti-inflammatory drugs
Apart from lipid-lowering drugs that have an established role in reducing MACE in patients with CAD, recent evidence indicates that aggressive inhibition of inflammation may also improve prognosis in this population.72 Several anti-inflammatory drugs have been introduced to inhibit vascular inflammation; the lipoprotein-associated phospholipase A2 (Lp-PLA2) inhibitor darapladib was the first drug that has been evaluated in both intravascular imaging and large-scale outcome trials.
4.1 Darapladib
Darapladib is a direct inhibitor of the Lp-PLA2 which is a calcium-independent enzyme secreted by the inflammatory cells (including monocyte-derived macrophages, T cells, and mast cells), circulates in plasma in its active form and it is primarily bound to LDL-cholesterol. Lp-PLA2 is involved in the metabolism of oxidized LDL (see Supplementary material online, Figure S2) generating potent pro-inflammatory mediators that contribute to plaque development, progression, and destabilization by promoting foam cell formation, endothelial dysfunction, and apoptosis.73–75 Histological data76 showed that the concentration of Lp-PLA2 protein is increased in TCFAs compared to smaller and more stable plaques, and there is evidence that raised Lp-PLA2 plasma levels are associated with coronary events.77
In the Integrated Biomarker and Imaging Study-2 (IBIS-2), 12-month therapy with darapladib had no effect on TAV but it appeared to inhibit necrotic core progression, resulting in a significant difference in the changes of the necrotic core volume between patients treated with darapladip and the control group at follow-up (see Supplementary material online, Table S1).78 Of note, the IBIS-2 study was not powered to assess changes in TAV and composition which were secondary endpoints of the study but to examine the effect of darapladib on plaque deformability estimated by palpography, a modality that later was proven unreliable in assessing plaque vulnerability.79
The effects of darapladip on clinical outcomes were examined in the STABILITY (Stabilization of Atherosclerotic Plaque by Initiation of Darapladib Therapy) trial which included 15 828 patients with stable CAD randomized to darapladib or placebo who were followed up for 3.7 years.80 Darapladib did not reduce the primary composite endpoint of cardiovascular death, MI, or stroke, but decreased the rate of major and total coronary events. Similarly, the SOLID-TIMI 52 (Stabilization of Plaque Using Darapladib-Thrombolysis in Myocardial Infarction 52) trial that included 13 026 patients admitted with ACS showed no prognostic benefit of darapladib at 3-year follow-up (see Supplementary material online, Table S3).81
4.1.1 Methotrexate
Methotrexate is a chemotherapy agent and immune-system suppressant that inhibits the enzyme dihydrofolate reductase essential for nucleotide synthesis. There is evidence that patients with chronic inflammatory diseases such as rheumatoid or psoriatic arthritis treated with low-dose methotrexate had fewer cardiovascular events than patients who received other therapies or placebo.82,83 Methotrexate appears to suppress YAP (yes-associated protein 1) activation that leads to a reduction in the levels of inflammatory factors (e.g. IL-6, connective tissue growth factor) and adhesion molecules; and through this mechanism, it may inhibit atherosclerotic disease progression.84
The National Institutes of Health-sponsored CIRT (Cardiovascular Inflammation Reduction Trial) that included 4786 patients who had stable atherosclerosis with diabetes mellitus or metabolic syndrome reported that low-dose methotrexate did not reduce MACE (see Supplementary material online, Table S3).85 A possible explanation of this finding is the fact that low-dose methotrexate also failed to reduce the plasma levels of IL-1β, IL-6, or CRP.
4.1.2 Canakinumab
Canakinumab is a recombinant human monoclonal antibody that has anti-inflammatory effects by selectively inhibiting IL-1β receptor binding. IL-1β is released following activation of the NLRP3 inflammasome and plays a central role in the systemic inflammatory response by increasing the production of IL-6 by various cell types and driving its signalling pathway.86 IL-6 mediates the acute phase response by stimulating the liver to produce proteins for host defences, but also promotes thrombosis and inhibits fibrinolysis. IL-1β has also direct pro-atherogenic effects as it increases the expression of leucocyte adhesion molecules and thrombogenic mediators and promotes smooth muscle cells proliferation and endothelial cells activation.87,88
The CANTOS (Canakinumab Anti-Inflammatory Thrombosis Outcome Study)89 demonstrated that in patients with previous MI and evidence of ongoing sub-clinical inflammation—defined by a high-sensitivity CRP ≥2 mg/L—inhibition of IL-1β with canakinumab at a dose of 150 mg every 3 months was associated with a 15% reduction in MACE regardless of lipid-level lowering (see Supplementary material online, Table S3). A subsequent analysis of the CANTOS trial found that random allocation to canakinumab compared to placebo reduced the total number of serious cardiovascular events during a median follow-up of 3.7 years irrespective of the administrated dose.90 However, this drug did not have any effect on the all-cause mortality compared to placebo due to a higher rate of fatal infections.89
4.1.3 Colchicine
Colchicine is a unique anti-inflammatory agent with broad cellular effects. It is accumulated in leucocytes, and by binding to microtubules and interfering with their function this drug modulates the expression of cytokines and interleukins, and the ability of neutrophils to marginate, ingress, aggregate, express superoxide, release neutrophil extracellular traps, and interact with platelets.91
In a serial angiography and IVUS study, Deftereos et al.92 demonstrated that colchicine was associated with a lower incidence of binary restenosis due to a reduction in the normalized neointima volume in diabetic patients treated with bare metal stents. However, there is lack of evidence on the effect of colchicine in preventing coronary plaque evolution in native segments.
So far, four independent randomized controlled trials have evaluated the effect of colchicine in patients with acute and chronic coronary syndromes (see Supplementary material online, Table S3). The Low-Dose Colchicine (LoDoCo) study,93 which included patients with stable CAD, demonstrated a reduction in the incidence of cardiovascular events in patients treated with colchicine; however, this was an open-label trial involving only 532 patients. The Low-Dose Colchicine 2 (LoDoCo2)94—a randomized, controlled, double-blind, event-driven trial—enrolled 5522 patients who were randomized to placebo or colchicine 0.5 mg once daily and demonstrated that colchicine prevents cardiovascular events. Similarly, the Colchicine Cardiovascular Outcomes Trial (COLCOT)95 showed a reduction in the incidence of MACE by −23% in patients with ACS at 2-year follow-up. Nevertheless, in contrast to the LoDoCo and COLCOT trials, the Australian Colchicine in Patients with Acute Coronary Syndrome (COPS)96 trial demonstrated that colchicine did not reduce cardiovascular events at 1-year follow-up and there was a trend toward a higher rate of all-cause mortality in the colchicine group compared to placebo; however, this study was underpowered to assess the effect of colchicine on clinical outcome. A recent metanalysis97 including 11 816 patients with CAD showed that colchicine reduced MACE rate as well as the risk of MI, stroke, and coronary revascularization compared to placebo, with no significant differences in all-cause mortality or cardiovascular death between the two groups.
The above studies were important to highlight the value of colchicine in secondary prevention but also had significant limitations. None of them used clinical or biological markers of inflammation for the selection of participants, cholesterol levels or blood pressure at enrolment were not reported and they recruited predominantly male patients. In the coming years, the CLEAR SYNERGY and the COLCARDIO trial (ACTRN12616000400460) will provide additional evidence on the efficacy and long-term safety of colchicine in patients with ACS.
5. Other therapies
5.1 Antihypertensive agents
Several antihypertensive agents, in particular angiotensin-converting enzyme (ACE) inhibitors and calcium channel blockers, have been tested in intravascular imaging-based studies to investigate their role in inhibiting plaque progression. The rationale behind their potential benefits in inhibiting atherosclerosis might be explained by the pleiotropic effects of these drugs in addition to blood pressure reduction. For instance, ACE inhibitors downregulate the pro-atherogenic effects induced by angiotensin-II that increases oxidative stress, expression of inflammatory cytokines, and adhesion molecules modulating endothelial function as well as cellular migration and proliferation.98 Calcium channel blockers may affect smooth muscle cells proliferation and migration, increase lipid resistance to oxidative stress, and improve endothelial function by inhibiting apoptosis and modulating nitric oxide expression.99
The Comparison of Amlodipine vs. Enalapril to Limit Occurrences of Thrombosis (CAMELOT) study showed that neither amlodipine nor enalapril reduced PAV after 2 years of treatment.100 In the PERindopril's Prospective Effect on Coronary aTherosclerosis by Angiography and IntraVascular Ultrasound Evaluation (PERSPECTIVE) study,101 no difference was noted between perindopril and placebo in the changes in plaque area at 3-year follow-up. Similar findings were reported by the Effect of Nifedipine on Coronary Endothelial Function and Plaque Formation in Patients With Coronary Artery Disease (ENCORE II) study where nifedipine did not reduce PAV compared to placebo during a follow-up of 18–24 months (see Supplementary material online, Table S1).102 It has to be stressed, however, that IVUS-based endpoints were not the primary outcomes of these studies and thus none of them was powered to detect differences in PB.
In line with the intravascular imaging studies, calcium channel blockers do not seem to improve outcomes in patients with CAD. In the PREVENT (Prospective Randomized Evaluation of the Vascular Effects of Norvasc Trial) study amlodipine 5–10 mg did not reduce MACE over a 3-year follow-up period, despite reducing hospital admissions for unstable angina and coronary revascularization.103 Similarly, the ACTION (A Coronary disease Trial Investigating Outcome with Nifedipine) study showed no prognostic benefit of treatment with nifedipine in patients with stable CAD.104 Conversely, the HOPE (Heart Outcomes Prevention Evaluation)105 and the EUROPA (EUropean Trial on Reduction of Cardiac Events with Perindopril in stable CAD)106 trials which evaluated the prognostic benefit of treatment with ramipril and perindopril, respectively, showed that both drugs improve outcomes in patients with established CAD. On the other hand, in the Prevention of Events with Angiotensin-Converting Enzyme inhibition (PEACE) trial, trandolapril did not improve prognosis in patients with stable CAD and preserved left ventricular systolic function (see Supplementary material online, Table S3).107 This paradox can be explained by the fact that ACE inhibitors are not equally effective against cardiovascular disease; perindopril may be superior to trandolapril in this setting as it has a beneficial effect on endothelial function.108,109
5.1.1 Antidiabetic drugs
The implications of oral glucose-lowering agents on PB have been investigated in IVUS-based studies. In the Pioglitazone Effect on Regression of Intravascular Sonographic Coronary Obstruction Prospective Evaluation (PERISCOPE) study,110 pioglitazone was superior to glimepiride in inhibiting plaque progression (PAV: −0.16% vs. +0.73%, P = 0.02), whereas the Assessment on the Prevention of Progression by Rosiglitazone on Atherosclerosis in Diabetes Patients With Cardiovascular History (APPROACH) study111 showed no difference between the effect of rosiglitazone and glipizide on PAV (see Supplementary material online, Table S1).
A meta-analysis demonstrated that pioglitazone reduces the risk of MACE, stroke, and MI in patients with previous history of cardiovascular disease, but it also increases the risk of heart failure.112 In contrast, in a sub-analysis of the Bypass Angioplasty Revascularization Investigation 2 Diabetes (BARI 2D) trial rosiglitazone did not affect MACE in patients with type 2 diabetes mellitus and established CAD.113 Similarly, the Trial Evaluating Cardiovascular Outcomes with Sitagliptin (TECOS) study showed that sitaglipitin was not associated with a higher risk of MACE and hospitalization for heart failure when added to usual care in patients with type 2 diabetes mellitus and cardiovascular disease (see Supplementary material online, Table S3).114
6. Discussion
From the above studies, it is apparent that the pharmacotherapies which have been associated with a prognostic benefit in large-scale outcome trials have a minimal but consistent effect on PB among appropriately powered intravascular imaging studies, while there is limited data about their implications on plaque composition. Studies assessing the effects of emerging therapies on necrotic core component, FCT, and macrophage accumulation have included small numbers of patients or have not been powered for these endpoints; therefore, further research is needed towards this direction to fully explore the potential of these intravascular imaging-based surrogate endpoints in predicting the prognostic value of novel therapies.
Although there is a consistency between intravascular imaging and outcome studies—i.e. drugs that reduce PB are associated with prognostic benefit—it seems that the changes in PB are disproportionately lower than the reduction of events reported in clinical studies. This could be due to differences in inclusion criteria between studies, but also to the fact that an increased PB does not necessarily indicate a high-risk lesion. In the PROSPECT study,11 PB ≥70% was the strongest predictor of MACE but it had a low positive predictive value for events (9.5%) in a lesion-level analysis.
Plaque features such as lipid burden, thin fibrous cap over lipid tissue, and macrophage accumulation have been associated with plaque vulnerability and future events. For instance, in the PROSPECT II study10 PB and composition assessed by NIRS-IVUS provided complementary information and these variables were independent predictors of MACE. Similar were the findings of the CLIMA study14 where patients with lesions characterized by small lumen area, increased lipid component, a thin fibrous cap over the lipid tissue and macrophages infiltration were at higher risk of suffering future events.
It is likely that pharmacotherapies targeting coronary plaque evolution may change not only PB but also its composition and reduce vascular inflammation leading to plaque passivation.
6.1 Multimodality–hybrid imaging for more accurate assessment of plaque phenotype changes
Over the recent years, there has been a shift toward the use of multimodality imaging to better assess vessel wall response to treatment with novel therapies.3,4,25,115 However, this approach has also significant limitations. Firstly, all the existing intravascular imaging modalities have limited efficacy in assessing some high-risk plaque features, such as its composition, the presence of macrophages, FCT, cholesterol crystals, and neo-vessels (Figure 1). Secondly, the co-registration of the imaging data acquired by two different catheters is a challenging and tedious process that is prone to errors. Advances in hybrid intravascular imaging and the design of catheters with multiple imaging probes for simultaneous data acquisition are expected the overcome this limitation enabling a complete and comprehensive assessment of plaque composition and biology.115
Moreover, without prospective evidence from large-scale multimodality intravascular imaging studies, there are no established scores that combine different plaque features to allow more accurate quantification of plaque stability. Finally, some of the current intravascular imaging studies focus on the changes in PB and composition in the entire studied segment. This approach averages changes in PB in disease-free and atherosclerotic sub-segments, whereas effect of pharmacotherapies may be more intense on vulnerable plaques; therefore, this approach is likely to underestimate their effects on plaque stabilization.4,24 Future studies focusing on plaque changes at lesion-level will allow more representative assessment of therapies on plaque vulnerability and will reduce the cost of studies as they will require recruitment of a smaller number of patients to meet their primary endpoints since high-risk patients have multiple plaques in the coronary tree.11,116
6.2 Implications of pharmacotherapies on local hemodynamic forces
Cumulative data have highlighted the role of endothelial shear stress in vulnerable plaque formation and destabilization showing that low shear stress promotes the formation of high-risk plaques, whereas high shear stress appears to activate mechano-transduction pathways that lead to their destabilization.117,118 The PREDICTION13 and ad hoc analyses of the PROSPECT study119,120 have recently shown that shear stress may be an independent predictor of plaque vulnerability and has a higher accuracy than IVUS or VH-IVUS-derived variables in predicting MACE. Similarly, plaque axial and longitudinal stress also provided useful prognostic information to identify lesions at risk (Figure 3).120,121 Minor changes in PB and composition noted in intravascular imaging studies may have a detrimental effect on shear stress distribution. In the IBIS 4 study,4 high-dose rosuvastatin therapy did not change lumen area at follow-up in ‘segment-level analysis’, but increased lumen area in the 10 mm most diseased segment by +2.5%. This change is expected to affect shear stress distribution and reduce mean shear stress in this segment by approximately -3.6% if there is no change in coronary flow. Likewise, a minor decrease in PB, remodelling index, and a necrotic core burden and especially an increase in the FCT may increase the minimum stress required to cause fibrous cap destabilization and plaque rupture.122 It is essential, therefore, to focus on lesion-level analysis, use multimodality imaging to thoroughly and meticulously assess the effects of novel athero-protective medications on plaque morphology and examine their implications on its physiology that determines plaque evolution and vulnerability.123
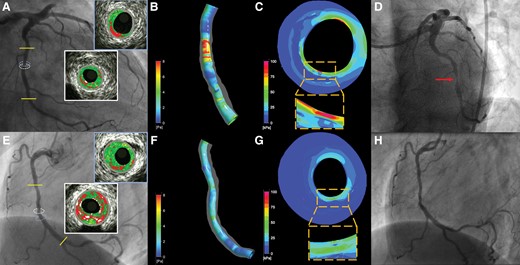
Case examples highlighting the prognostic value of the local hemodynamic forces in predicting events. Panel (A) illustrates the angiographic image of a lesion located in the mid left circumflex that caused an event at 13-month follow-up (D). VH-IVUS imaging showed a moderate lesion with a minimum lumen area of 2.94 mm2 (indicated with a white circle on angiography, the corresponding VH-IVUS cross-section is shown in the white inset), a plaque burden of 70.5% and a TCFA phenotype. Blood flow simulation analysis demonstrated high wall shear stress (WSS) at the throat of the lesion with the maximum predominant WSS estimated at 6.94 Pa (B), while plaque structural stress (PSS) analysis also showed increased plaque stress (C) estimated at 123 kPa (maximum PSS location on coronary angiography is indicated with a light blue circle, the corresponding VH-IVUS cross-section is shown in the light blue inset). Panel (E) portrays the angiographic image of a moderate lesion located in the right coronary artery that remained quiescent at 13-month follow-up (H). VH-IVUS imaging showed a plaque with a TCFA phenotype, minimum lumen area of 3.75 mm2 (its location in coronary angiography is shown with a white circle and the VH-IVUS image in the white inset) and similar plaque burden (78.6%) compared to the previous lesion. However, in this occasion, the maximum predominant WSS was normal (3.73 Pa, F), while PSS (the location in angiography is shown with a light blue circle and the corresponding VH-IVUS frame in the light blue inset) was lower (71 kPa, G) suggesting an athero-protective hemodynamic environment that inhibited atherosclerotic disease progression.
6.3 Pleiotropic effects of drugs targeting atherosclerosis
In addition to plaque anatomy and pathophysiology, atherosclerotic evolution also depends on systemic factors such as blood viscosity, platelet activity, fibrinogen levels, and the interplay between coagulation and fibrinolytic system which regulate thrombus formation.124,125 These pathways determine the clinical consequences of plaque rupture as well as the effects of plaque erosion or the eruption of a calcific nodule that constitute common causes of acute coronary events.16 Some of the tested pharmacotherapies have pleiotropic effects affecting plaque evolution and also endothelial function, platelet reactivity, and vascular inflammation. For instance, statins not only increase the number of intimal smooth muscle cells and the expression of type I procollagen, but also decrease the proliferation and activation of macrophages as well as tissue factor expression in animal models.126,127 Similarly, experimental studies have shown that anti-PCKS9 antibodies reduce macrophages infiltration within aortic plaques and increase the endothelial progenitor and circulating angiogenic cells.128 These changes may play a key role in promoting plaque healing following plaque rupture and thus reducing the risk of ACS.129 Finally, lipid-lowering drugs by reducing cholesterol levels seem also to induce collagen synthesis which is important not only for the passivation of high-risk lesions but also for the healing of ruptured or eroded plaques.3,130,131 It is apparent that intravascular imaging studies cannot assess all these pleiotropic effects of novel therapies on plaque biology and their prognostic implications. Therefore, outcome studies should be always considered as the ultimate test for examining the potency of novel drugs.
7. Conclusions
The changes in PB which has been the traditional intravascular imaging endpoint for assessing the efficacy of pharmacotherapies targeting atherosclerosis have a consistent but limited efficacy in predicting their prognostic benefit. While this may reflect the pleiotropic effects of some of these drugs, it also indicates that PB alone does not accurately reflect plaque vulnerability. Future studies evaluating the effects of novel drugs on plaque characteristics are expected to utilize serial multimodality/hybrid imaging to assess more accurately plaque morphology and incorporate physiological endpoints such as shear stress and plaque stress.
These studies are anticipated to enrich our understanding, provide mechanistic insights about the effect of drugs on atherosclerotic evolution, and fully explore the potential of intravascular imaging in predicting their effect on clinical outcomes.
Supplementary material
Supplementary material is available at Cardiovascular Research online.
Funding
V.T. and C.V.B. are funded by the National Institute of Health Research (NIHR) Barts Biomedical Research Centre. M.R.B. is funded by the British Heart Foundation (BHF) grants RG71070 and RG84554, the NIHR Cambridge Biomedical Research Centre, and the Cambridge BHF Centre for Research Excellence [RE/18/1/34212]. S.Z.G. is funded by the BHF Clinical Research Fellowship FS/19/66/34658.
Data availability
The data underlying this article are available in the article and in its online supplementary material.
References
Author notes
Conflict of interest: L.R. received research grants from Abbott, Boston Scientific, Infraredx and consulting fees from Medtronic. A.B. received payment for lectures and educational events from Medtronic. The remaining authors do not have any conflicts of interest to disclose.