-
PDF
- Split View
-
Views
-
Cite
Cite
Seria Masole Shonyela, Chunwei Shi, Wentao Yang, Xin Cao, Guilian Yang, Chunfeng Wang, Recombinant Lactobacillus plantarum NC8 strain expressing porcine rotavirus VP7 induces specific antibodies in BALB/c mice, Acta Biochimica et Biophysica Sinica, Volume 53, Issue 6, June 2021, Pages 707–718, https://doi.org/10.1093/abbs/gmab050
- Share Icon Share
Abstract
The major etiologic agent that causes acute gastroenteritis worldwide in young animals and children is Group A rotavirus. Currently, commercially available vaccines do not often prevent porcine rotavirus (PRV) infection. In this study, we evaluated the efficacy of oral recombinant Lactobacillus vaccine against PRV in a mouse model. Lactobacillus plantarum NC8 was used as the host strain, and bacterial vectors were constructed, because the NC8 isolated has shown the capability to survive gastric transit and to colonize the intestinal tract of humans and other mammals. To explore the immunological mechanisms, lactic acid bacterial vectors were used to express VP7 antigen from PRV. We constructed an L. plantarum strain with surface-displayed VP7, named NC8-pSIP409-pgsA-VP7-DCpep. The expressed recombinant protein had a molecular weight of ∼37 kDa. The strain was used to immunize BALB/c mice to evaluate their immunomodulatory characteristics. Mice were orally immunized with recombinant L. plantarum NC8-pSIP409-pgsA-VP7-DCpep at a dose of 2 × 109 colony forming units/200 µl. The results showed that NC8-pSIP409-pgsA-VP7-DCpep significantly stimulated the differentiation of dendritic cells (DCs) in Peyer’s patches (PPs) and increased the serum levels of IL-4 and IFN-γ, as measured by enzyme-linked immunosorbent assay in mice treated with NC8-pSIP409-pgsA-VP7-DCpep. Compared to the empty vector group, NC8-pSIP409-pgsA-VP7-DCpep significantly increased the production of B220+ B cells in mesenteric lymph nodes (MLNs) and PPs and also increased the titer levels of the VP7-specific antibodies, including IgG and sIgA. The administration of NC8-pSIP409-pgsA-VP7-DCpep mediated relatively broad cellular responses. This study reveals that clear alternatives exist for PRV control strategies and provides information on PRV infection.
Introduction
There are two kinds of genetically engineered live vaccines: one is a gene deletion vaccine and the other is a vector vaccine. The gene-deficient vaccine uses genetic engineering methods to delete virulence-related genes. The virus forms pseudoviral particles and has an effective antigenic structure, but it cannot regain virulence and further replicate and multiply. The vector vaccine connects the protective antigen to the corresponding vector for expression. For example, the VP6 and VP7 genes can be inserted into a suitable vector, such as a viral vector, bacteria proteins, poxvirus, and adenovirus, using insect cells, Nicotiana benthamiana and transgenic potatoes [1–4] or bacteria, such as Salmonella, Escherichia coli, and lactic acid bacteria. The vector, as well as the antigen of interest which is expressed along with the replication of the vector, stimulates the body to produce protective antibodies [4–6]. Because most vaccines are injected subcutaneously into the muscle, this type of immunization can induce strong systemic B-cell and T-cell immune responses, but adaptive immune responses are relatively weak in the mucosal sites, such as the intestinal epithelium. In contrast, rotavirus enters the host through the oral mucosal pathway. Thus, entering the host is more likely to produce an adaptive immune response [4–6]. At present, the rotavirus protein vector vaccine is mainly an oral vaccine that uses lactic acid bacteria and E. coli as delivery vehicles. This newly constructed oral live vaccine has specificity, and the production of IgG and IgA antibodies provides complete protection against porcine rotavirus (PRV) [1–4].
PRV is recognized as a key source of acute gastroenteritis in young piglets as well as nursing and weaned piglets mainly if piglets are colostrum deficient. Of the seven serogroups (A–G), four (A, B, C, and E) rotavirus serogroups have been identified in swine, and Group A rotavirus is considered as the main rotavirus. The external capsid layer of PRV contains two viral proteins, VP4 and VP7, which contain 780 copies of the VP7 protein and are grouped as 260 trimers. The VP7 glycoprotein has 14 serotypes and is the main protein that induces the production of neutralizing antibodies, which has become the focus in vaccine research. The VP7 protein has immunogenicity and immunoreactivity, and it can induce the production of protective neutralizing antibodies [7–10]. Anti-VP7 antibodies are necessary for the protection against RV shedding. Thus, a global public health goal is the development of an effective and safe vaccine.
Lactobacillus is a facultative anaerobic, Gram-positive, microaerophilic, rod-shaped bacterium [11], and it has been confirmed to promote health and improve several health problems [12]. Lactobacillus is a food-grade bacterium. Lactobacillus plantarum is a common lactic acid bacterial strain and has a wide range of applications in industrial lactic acid fermentation and health care. Expression of a foreign antigen using a lactic acid bacterial strain induces mucosal (sIgA) and systemic (IgG) immune responses [13]. Lactic acid bacteria have the following advantages: (i) lactic acid bacteria are easy to culture, and some strains can construct a new expression vector system; (ii) the genetic manipulation method is simple, reproducible, and efficient; (iii) the genetic engineering products guarantee the relative safety of the food-grade expression system for direct oral administration; (iv) the highly regulatable promoter system is capable of expressing toxin genes; (v) the exogenous proteins obtain good expression inside and outside the cell; (vi) lactic acid bacteria effectively cause the body’s immune response that lasts for a long time; and (vii) lactic acid bacteria are safe, and the expressed foreign protein can be taken directly together with the bacteria without purification. Some studies have revealed that L. plantarum strains have probiotic effects on human health [14]. Some patients have been treated with recombinant lactobacilli that express exogenous antigens [15] with the dosages depending on the period of existence in the intestine and the expressed antigen. Many reports have suggested that the L. plantarum NC8 strain can be extensively used for vector-based expression [16]. Therefore, this strain can be used as an ideal antigen presentation vector. The expression of the VP7 gene of PRV as a recombinant fusion protein represents a relatively safe expression system for developing vaccines against PRV infection. To generate a suitable, safe, and efficient vaccine to prevent infection, a recombinant lactic acid bacterial strain that can express an antigen and thus activate the systemic and mucosa immune responses, for example L. plantarum NC8 strain, is needed [17–19]. Several experimental studies using L. plantarum NC8 strain have revealed that the strain induces an efficient immune response to a specific antigen because diverse antigens have been expressed in L. plantarum NC8 strains as probiotics [20–24].
Peptides targeting dendritic cells (DCpeps) play a central role in targeting peptides by directing innate immunity and regulating adaptive/acquired immunity. Phage display allows detection of the three DC-binding peptides containing 12 residues. Recent studies have demonstrated that DCpep binds with DCs from a wide range of species, including avian, chicken, canine, feline, and equine DCs [21,22]. In vitro, oral administration of NC8-pSIP409-NP-M1-DCpep in DCs from chicken is superior in the activation of autologous CD3+CD4+ and CD3+CD8+ T cells in the spleens of vaccinated chickens compared to control groups for avian influenza virus [21,22]. Oral administration of NC8-pSIP409-NP-M1-DCpep enhances T-cell-mediated immune responses as well as mucosal sIgA and IgG levels, providing protection against H9N2 AIV challenge. In addition, DCs pulsed with DCpep in vitro bind to hepatitis virus nonstructural protein 3 (NS3), resulting in better activation of autologous CD4+ and CD8+ T cells from hepatitis C virus-infected patients in comparison with NS3 alone. Thus, DCpep has been shown to be an effective mucosal adjuvant [22–24].
Poly-γ-glutamic acid synthetase A (pgsA) is a protein in the polyglutamic acid synthetase system of Bacillus subtilis, which attaches to some enzymatic systems on cell walls. Recently, pgsA was demonstrated to be a useful exogenous protein on the surface of L. plantarum, resulting in a surface presented with bacteria [23]. Due to its high level of surface display, the system may be functional using Gram-positive bacteria, but studies have been restricted to Lactococcus lactis and Lactobacillus casei to date. Enzymes from different sources and the pgsA bacterial surface display systems have been developed, and this anchor motif has been displayed effectively on the surface of E. coli [25]. However, the genetically engineered vaccines composed of a single recombinant antigen are inadequately immunogenic, so it is essential to increase their immunogenicity by combining with suitable adjuvants, such as cytokines and immunoregulatory agents.
In this study, we constructed a recombinant L. plantarum strain NC8-pSIP409-pgsA-VP7-DCpep that can express the heterologous VP7-DCpep protein and found that the recombinant protein substantially improved the in vivo antibody levels in BALB/c mice.
Materials and Methods
Reagents, bacterial strain, virus, and plasmid vector
The DN30209 PRV isolate VP7 mRNA (complete cds, GenBank: JN388691.1) was purchased from Genewiz (Beijing, China). The pSIP409-pgsA expression vector and the L. plantarum NC8 strain were kindly provided by Professor A. Kolandaswamy (Madurai Kamaraj University, India) and stored in the Laboratory of Jilin Provincial Engineering Research Center of Animal Probiotics [26]. Horseradish peroxidase-conjugated goat anti-rabbit IgG was purchased from Cell Signaling Technology (Boston, USA).
Construction of recombinant plasmid
The VP7-DCpep fusion gene was cleaved from the T4-VP7-DCpep plasmid (Genewiz) by incubating 10 µl of template with 30 µl of ddH2O, 5 µl of 10× buffer solution, 2.5 µl of XbaI, and 2.5 µl of HindIII at 37°C for 4 h, followed by 0.8% agarose gel electrophoresis. The expected amplicons of VP7 (1029 bp) were excised from the gel and purified with a Genomic DNA Kit (Tiangen, Beijing, China) according to the manufacturer’s instructions.
The VP7-DCpep gene fragment was ligated into the pSIP409-pgsA vector overnight at 16°C and transformed into E. coli BL21 competent cells the next day. In brief, the vector was incubated with BL21 cells in an ice bath for 30 min, followed by incubation in a 42°C water bath for 90 s and in an ice bath for another 6 min. Then, 600 µl of Luria-Bertani (LB) medium was added, and the cells were shaken at 220 rpm for 1 h at 37°C. Then, 100 µl of bacterial suspension was taken out and applied to solid culture medium containing 50 μg/ml kanamycin. After overnight incubation at 37°C, colonies were cultured on a shaker at 180 rpm for 12 h at 37°C. Monoclones were selected and cultured in 5 ml of LB liquid medium containing 50 μg/ml kanamycin. A plasmid extraction kit (Tiangen) was used to extract the plasmid according to the manufacturer’s instructions. The pSIP409-pgsA-VP7 recombinant plasmid was then verified by XbaI/HindIII digestion at 37°C in a water bath. The enzyme products were detected by 0.8% agarose gel electrophoresis and then sent to Jilin Province Comate Bioscience Co., Ltd (Changchun, China) for sequencing.
Construction of recombinant L. plantarum NC8 strain
L. plantarum NC8 strain was transformed into competent cells as previously reported [27]. Then, the competent NC8 bacterial cells were electrotransformed with recombinant plasmid following a previously described protocol [28]. In brief, pSIP409-pgsA-VP7-DCpep was mixed with the L. plantarum NC8 competent cells in an ice bath. After 5 min, the mixture was transferred into a pre-cooled 0.2 cm electric shock cuvette, subject to electrotransformation by using an electric transformer (Gene Pulser XcellTM System; Bio-Rad, Hercules, USA), producing NC8-pSIP409-pgsA-VP7-DCpep recombinant strain. Then, 800 µl of prewarmed MRS liquid medium (Solarbio, Beijing, China) containing 0.5 M sucrose was added and then transferred into a 1.5 ml centrifuge tube for anaerobic culture at 30°C for 3 h. Then, 200 μl bacterial suspension was sprayed on MRS solid medium containing 10 μg/ml erythromycin (Em; Solarbio) and cultured under anaerobic conditions at 30°C until single colonies formed. A single colony was inoculated into 5 ml MRS liquid medium (Em+) and incubated overnight at 30°C under anaerobic conditions. Then, 800 µl of the NC8-pSIP409-pgsA-VP7-DCpep bacterial suspension was mixed with 80% glycerol (200 µl) to preserve the strain and frozen at −80°C.
Sodium dodecyl sulfate-polyacrylamide gel electrophoresis (SDS-PAGE)
The NC8-pSIP409-pgsA-DCpep strain was subcultured, and the second generation of bacteria was added to MRS liquid medium (Em+) at 1:100 inoculum. Anaerobic culture was performed in a 30°C incubator until the optical density (OD) value reached 0.3. Then, 20 μg/ml SppIP (Sangon Biotech, Shanghai, China) was added at 1:400 (v/v) to induce protein expression overnight. The overnight culture was centrifuged at 5000 g for 10 min at 4°C. The pellet was washed three times with cold phosphate buffered saline (PBS), digested in 500 µl of pyrolysis buffer (Beyotime, Shanghai, China) for 30 min on a shaker (120 rpm) at 37°C and then centrifuged at 10,000 g for 30 min. Then, 160 µl of the supernatant containing VP7 was added to 40 µl of 5× SDS buffer, denatured by boiling at 100°C for 5 min, and centrifuged at 8000 g for 2 min. Then, 15 µl of the protein sample was subject to SDS-PAGE (5% stacking gel and 12% separation gel). The gel was then incubated with Coomassie brilliant blue staining (Beyotime) and analyzed on an Amersham Imager (General Electric Company, Shanghai, China).
Western blot analysis
After SDS-PAGE, the proteins were electro-transferred to PVDF membrane (Millipore, Darmstadt, Germany). After the transfer was completed, the PVDF membrane was blocked using blocking solution (Beyotime) for 3 h at room temperature. Then, the polyvinylidene fluoride (PVDF) membrane was incubated with the 6 × His Epitope Tag monoclonal antibody (ThermoFisher Scientific, Waltham, USA) and then horseradish peroxidase-conjugated rabbit anti-mouse secondary antibody (ThermoFisher Scientific). After washing, the blot was visualized by enhanced chemiluminescence (ECL; ThermoFisher Scientific) and analyzed on the Amersham Imager.
Flow cytometry analysis
The expression of VP7-DCpep fusion protein by NC8-pSIP409-pgsA-VP7-DCpep recombinant strain was confirmed by analyzing the fluorescence intensity on the bacterial surface. Briefly, NC8-pSIP409-pgsA-VP7-DCpep was induced as described above, and NC8-pSIP409-pgsA was used as a negative control. The bacterial suspension was centrifuged for 1 min at 14,000 g, and the bacterial cells was washed three times with PBS containing 0.5% bovine serum albumin. The cells were collected and incubated with 6 × His Epitope Tag monoclonal antibody (1:100 dilution), followed by incubation with fluorescein isothiocyanate-labeled rabbit anti-mouse IgG secondary antibody (1:100 dilution; ThermoFisher Scientific), and then detected by a BD LSR Fortessa flow cytometer (BD Bioscience, San Jose, USA). The data were analyzed using FlowJo 7.6.1 software [29].
Inoculation of mice with recombinant NC8 strain
To study the immune responses to recombinant NC8-pSIP409-pgsA-VP7-DCpep, rotavirus antibody-free SPF adult female BALB/c mice (7 weeks of age, weighing 25–30 g) were obtained from Huafukang Biotechnology Co., Ltd (Beijing, China). Briefly, mice were randomly divided into three groups (15 mice in each group): NC8-pSIP409-pgsA-VP7-DCpep (Group A), NC8-pSIP409-pgsA (Group B), and PBS (Group C). All mice received an oral dose of 2 × 109 colony forming units in 200 µl PBS for three consecutive days (days 0, 1, and 2). On days 14, 15, and 16, a booster immunization was administered, and a second booster was administered at days 28, 29, and 30. In Group C, only PBS was administered. All experiment protocols were approved by the Institutional Animal Care and Use Committee of Jilin Agricultural University (No. 20190520003).
Sample collection
Blood sample was collected from the tail on days 14 and 28 and from the eye on day 42. Serum was obtained by centrifugation and stored at −80°C until use. On day 42 after immunization, mice were weighed. Three mice from each group were sacrificed by cervical dislocation. The heart, liver, spleen, lung, and kidney (two) were weighed, and the organ index was calculated using the following equation: organ index = (organ weight/mouse body weight) × 100%.
Single-cell suspension preparation
On a super-clean platform, Peyer’s patches (PPs), mesenteric lymph nodes (MLNs), and spleens were collected and ground in a 35 mm sterile dish containing a 200-mesh steel mesh with 1 ml of RPMI 1640 medium (ThermoFisher Scientific). The cell mixture was washed twice with RPMI 1640 medium, and the supernatant was discarded. Erythrocyte lysate buffer (Beyotime) was added to lyse red blood cells. After washing, the cell suspension was passed through a nylon membrane (Solarbio) and diluted with PBS, and the number of leukocytes was then counted. A segment of duodenum was collected from the mice and washed with PBS containing phenylmethanesulfonyl fluoride (PMSF). The intestinal lavage fluid was collected and frozen at −80°C.
Detection of NC8-pSIP409-pgsA-VP7-DCpep on B cells by flow cytometry
For the surface staining of B cells, the PP and MLN cell suspensions were adjusted to 1×104 cells/μl, incubated with anti-B220-APC antibody (BD Biosciences, San Jose, USA) for 20 min to detect B cells, and then washed twice with PBS at 4°C with shaking at 2000 rpm for 5 min. Then, 300 μl of the cell suspension was placed into a fluorescence-activated cell sorting tube and subject to flow cytometric analysis on the BD LSR Fortessa flow cytometer to detect B cells.
Enzyme-linked immunosorbent assay analysis
IL-4 and IFN-γ in serum and sIgA in intestinal lavages fluid were detected by using the enzyme-linked immunosorbent assay (ELISA) kits for mouse IL-4, IFN-γ, and sIgA, respectively (R&D Systems, Minneapolis, USA). The specific anti-His tag IgG in serum was detected by a commercial ELISA kit (GenScript, Nanjing, China). The protocols were according to the manufacturer’s instructions. The OD value of each well was detected with a microplate reader (BioTek, Winooski, USA) at 450 nm.
Challenge of mice with the DN30209 Chinese PRV strain
Four weeks after the last oral immunization, three mice from each group were challenged with 4 × 104 FFU(100) 50% infective dose (ID50) of the DN30209 Chinese PRV (a kind gift from Prof. Xiaofeng Ren, Northeast Agricultural University, Harbin, China) by oral gavage to evaluate vaccine-induced protection. The survival and body weight changes of mice after PRV challenge were monitored.
Histopathological examination
Because VP7 can invade intestinal epithelial cells, we selected the small intestine of mice to detect histopathological changes. Small intestine, liver, spleen, and lung were also harvested and fixed with 4% formaldehyde at room temperature for at least 48 h. Paraffin sections were made and stained with hematoxylin and eosin (H&E; Beyotime), and histological examination was performed under a fluorescence microscope (DMI8; Leica, Germany), and representative images of small intestine, liver, lung, and spleen were taken.
Statistical analysis
FlowJo 7.6.1 software was used for flow cytometric analysis, and GraphPad Prism 5 software was used for data analysis. One-way ANOVA was used for statistical analysis of the differences between groups. P<0.05 was considered of significant difference.
Results
Cloning, expression, and purification of the rotaviral VP7 gene in the prokaryotic expression system
VP7 protein is a suitable target for the development of vaccines against rotavirus. In this study, we cloned the VP7 gene from the DN30209 Chinese PRV isolate, and sequencing and BLAST results showed that the cloned VP7 gene represents the DN30209 Chinese PRV isolate (GenBank accession No. JN388691.1) [26]. The schematic representations of the rotaviral full length VP7 are shown in Supplementary Fig. S1.
The VP7-DCpep fusion gene with His tag was successfully released by the XbaI/HindIII double enzyme digestion, resulting in a 1029 bp fragment visualized via 0.8% agarose gel electrophoresis (Supplementary Fig. S2). The pSIP409-pgsA-VP7-DCpep recombinant plasmid was verified by restriction enzyme digestion using the XbaI/HindIII, followed by detection via 0.8% agarose gel electrophoresis. Two bands were observed with the expected size (Supplementary Fig. S2).
SDS-PAGE result showed that there was no obvious specific band at 37 kDa representing VP7-DCpep fusion protein in the group of NC8-pSIP409-pgsA-VP7-DCpep (Fig. 1A, lanes 1–4). This may be attributed to the low expression level of VP7-DCpep and low sensitivity of Coomassie brilliant blue staining method. However, Western blot analysis showed that there was specific band for NC8-pSIP409-pgsA-VP7-DCpep and no band for NC8-pSIP409-pgsA, which was a negative control (Fig. 1B). These results indicated that VP7 protein was expressed on the cell wall surface of recombinant L. plantarum NC8.
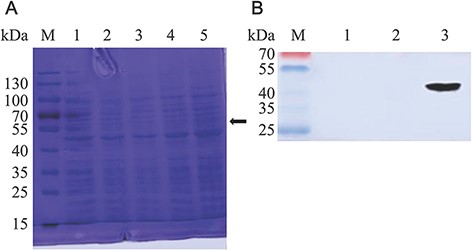
Detection of VP7 protein expression (A) Expression analysis of NC8-pSIP409-pgsA-VP7-DCpep by Coomassie brilliant blue-stained SDS-PAGE of L. plantarum NC8. For the analysis, 10 µl was loaded on 10% SDS-PAGE gel, which was run at 150 V. Lane M: marker; lane 1: induced NC8-pSIP409-pgsA; lanes 2, 3, and 4: 4, 6, and 8 h after induction of NC8-pSIP409-pgsA-VP7-DCpep, respectively. (B) Western blot analysis of the expression of recombinant NC8-pSIP409-pgsA-VP7-DCpep. Lane M, low molecular weight protein marker; lanes 1 and 2, induced NC8-pSIP409-pgsA; lane 3, induced NC8-pSIP409-pgsA-VP7-DCpep.
Confocal microscopy results showed that there was green fluorescence on the bacterial cell surface representing SppIP-induced NC8-pSIP409-pgsA-VP7-DCpep (Fig. 2B). Correspondingly, confocal microscopy showed that the SppIP-induced NC8-pSIP409-pgsA-VP7-DCpep strain expressing VP7 resulted in green fluorescence on the cells compared to the NC8-pSIP409-pgsA empty vector group that showed no green fluorescence (Fig. 2A). These results indicated that VP7 protein was expressed on the outer side of the cell wall.

Immunofluorescence analysis of VP7 protein expression in recombinant L. plantarum VP7 protein expression was analyzed by confocal fluorescence microscopy in (A) NC8-pSIP409-pgsA group and (B) recombinant NC8-pSIP409-pgsA-VP7-DCpep group. Magnification, 630 ×.
Flow cytometry results showed that the peak value of the NC8-pSIP409-pgsA-VP7-DCpep group was 28.4, while that of the NC8-pSIP409-pgsA group was 16 (Fig. 3A,B), and there was an obvious shift to right due to the expression of VP7 protein on the surface of L. plantarum (Fig. 3A). These data further indicated the expression of VP7 on the surface of recombinant L. plantarum NC8 strain.
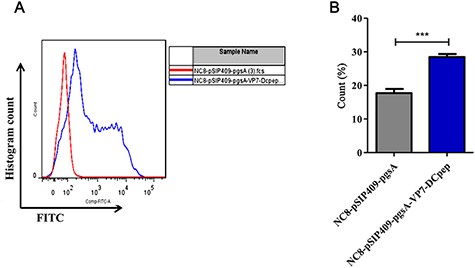
VP7 protein expression at the surface of L. plantarum (A) VP7 protein expression at the surface of L. plantarum was detected by flow cytometry. (B) Statistical analysis of the VP7 protein expression. ***P<0.001.
NC8-pSIP409-pgsA-VP7-DCpep promoted immune cells in mice
After 42 days of immunization, the MLN and PP junctions of three mice in each group were collected. The number of B220+ B cells in MLNs and PPs was detected by flow cytometry, with gating strategy shown in Fig. 4A,B. The results showed that the percentage of B220+ B cells in both MLNs (Fig. 4C; P<0.001) and PPs (Fig. 4D; P<0.01) in the NC8-pSIP409-pgsA-VP7-DCpep group was significantly higher than that in the NC8-pSIP409-pgsA group. These data indicated that NC8-pSIP409-pgsA-VP7-DCpep promoted the population of B cells.
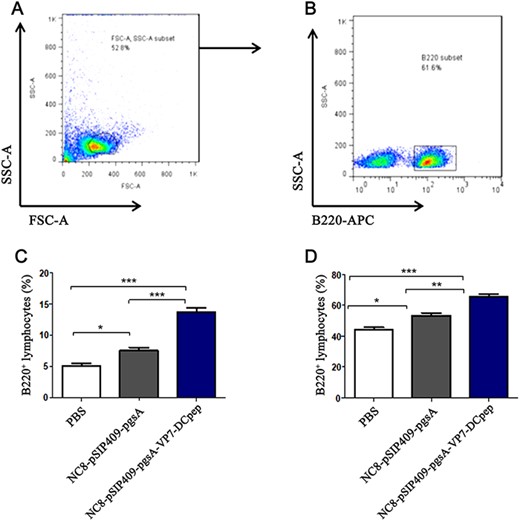
The number of B220+ B cells in MLNs and PPs was detected by flow cytometry (A) FSC-A SSC-A subset. (B) B220+ subset. (C) MLN B220+ percentage of lymphocytes. (D) PPs B220+ percentage of lymphocytes. *P<0.05, **P<0.01, ***P<0.001.
NC8-pSIP409-pgsA-VP7-DCpep induced specific antibodies in mice
To further evaluate the role of the NC8-pSIP409-pgsA-VP7-DCpep recombinant strain in humoral immunity, ELISA was used to detect the levels of the IFN-γ and IL-4 cytokines in mouse serum. The results showed that compared to the NC8-pSIP409-pgsA group, the levels of IL-4 (Fig. 5A) and IFN-γ (Fig. 5B) in the NC8-pSIP409-pgsA-VP7-DCpep group were increased significantly (P<0.001).

Serum cytokine levels detected by ELISA (A) IL-4 cytokine levels in mouse serum. (B) IFN-γ cytokine levels in mouse serum.***P<0.001. ns, not significant.
As shown in Fig. 6A,B, the expression levels of specific IgG (anti-His tag) and mucosal antibody sIgA in the NC8-pSIP409-pgsA-VP7-DCpep group were also significantly higher than those in the NC8-pSIP409-pgsA group (P<0.001). These data indicated that oral administration of NC8-pSIP409-pgsA-VP7-DCpep induced mucosal immune response.
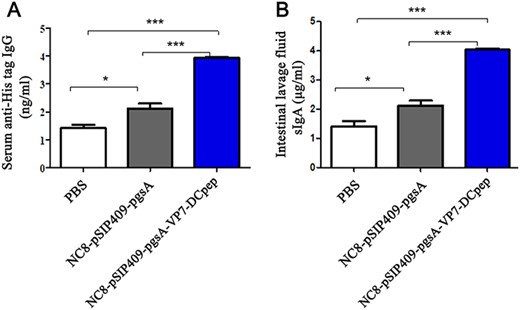
Specific antibodies detected by ELISA The expression of specific anti-His tag (A) IgG content in serum and (B) sIgA in intestinal lavage fluid. *P<0.05, ***P<0.001.
NC8-pSIP409-pgsA-VP7-DCpep was safe for immunization in mice
In the evaluation of the safety of the recombinant NC8-pSIP409-pgsA-VP7-DCpep, we found that the mice in the NC8-pSIP409-pgsA-VP7-DCpep group had normal hair, normal food intake, normal urine output, normal fecal output, and the same body weight as those in the PBS group within a week after immunization. The weight of the mice in the NC8-pSIP409-pgsA-VP7-DCpep recombinant strain group was increased as shown in Fig. 7A,B. On day 42, there was no significant difference in the index of heart, liver, spleen, lung, and kidney (two viscera) among the NC8-pSIP409-pgsA-VP7-DCpep, NC8-pSIP409-pgsA, and PBS groups.
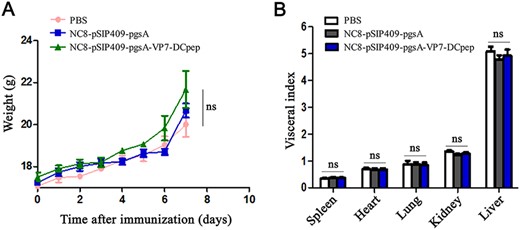
Safety evaluation of recombinant NC8-pSIP409-pgsA-VP7-DCpep in mice Effects on (A) body weight and (B) organ indexes after immunization. ns, not significant.
Because PRV mainly invades small intestinal epithelial cells, we selected mouse duodenum, liver, lung, and spleen from five viscera for pathological analysis (Fig. 8A–L). The intestinal villi in the NC8-pSIP409-pgsA-VP7-DCpep, NC8-pSIP409-pgsA, and PBS groups were intact, and the liver structure was clear. Moreover, the alveolar boundary in the lung was clear, and the red and white pulp boundary in spleen was clear. No pathological changes were found in these three groups, indicating that the immunization of mice with NC8-pSIP409-pgsA-VP7-DCpep was safe.
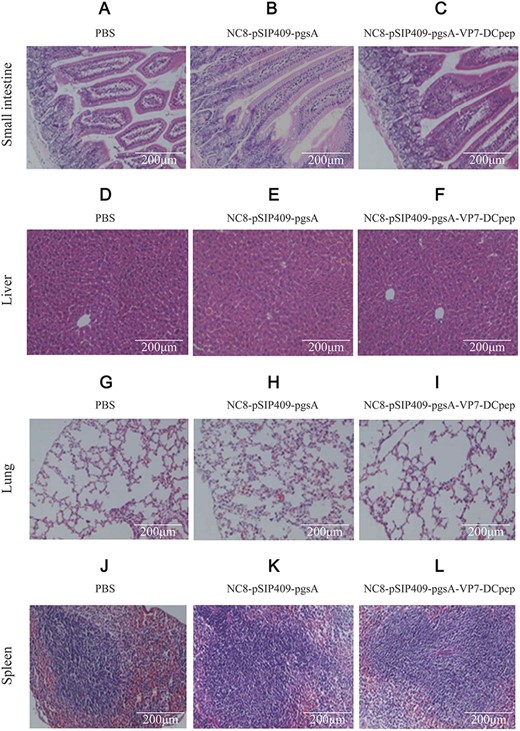
Pathological changes in mouse induced by recombinant NC8-pSIP409-pgsA-VP7-DCpep revealed by HE staining (A–C) Small intestine. (D–F) Liver. (G–I) Lung. (J–L) Spleen. A, D, G, and J represent mice treated with PBS; B, E, H, and K represent mice immunized with empty vector; and C, F, I, and L represent mice immunized with recombinant NC8-pSIP409-pgsA-VP7-DCpep. Scale bar: 200 μm.
NC8-pSIP409-pgsA-VP7-DCpep immunization protected mice against PRV challenge
To assess the protection activity of the NC8-pSIP409-pgsA-VP7-DCpep for mice against PRV infection post vaccination, a virus challenge experiment was conducted. By oral gavage, the immunized mice were challenged with 100 ID50 of the DN30209 Chinese PRV isolate strain per mouse for 3 weeks following the last vaccination. All mice survived after PRV challenge. However, mice treated with the NC8-pSIP409-pgsA-VP7-DCpep vaccine demonstrated improved body weight compared to those in the vaccine control groups receiving NC8-pSIP409-pgsA or PBS (Fig. 9A,B). These results demonstrated that oral immunization with NC8-pSIP409-pgsA-VP7-DCpep has promising protection against the PRV.
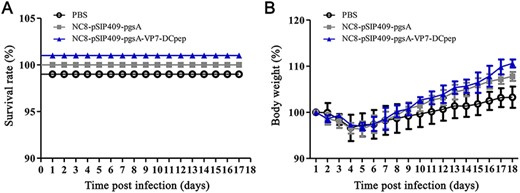
Oral immunization with NC8-pSIP409-pgsA-VP7-DCpep has promising protection against PRV challenge (A) Percent survival and (B) body weight changes of mice after infection.
Histopathological changes were little in mice after challenge following immunization
Because VP7 can invade intestinal epithelial cells, we selected the small intestine of mice to detect histopathological changes. Small intestine, liver, spleen, and lung were harvested from five viscera and fixed at room temperature with 4% formaldehyde for at least 48 h. Paraffin sections were made and stained with H&E for pathological analysis (Fig. 10A–L). The intestinal villi in the NC8-pSIP409-pgsA-VP7-DCpep recombinant group were intact, and the liver structure was clear. Moreover, the alveolar boundary in lung was clear, and the red and white pulp boundary in spleen was clear. No pathological changes were observed, indicating that the immunization of mice with NC8-pSIP409-pgsA-VP7-DCpep was safe and resulted in an immune response (Fig. 10C). In the NC8-pSIP409-pgsA empty carrier group and PBS group, moderate villous atrophy and fusions as well as moderate crypt hyperplasia were observed throughout the small intestine sections (Fig. 10A). These data indicated that NC8-pSIP409-pgsA-VP7-DCpep was effective for protecting mice from rotavirus challenge.
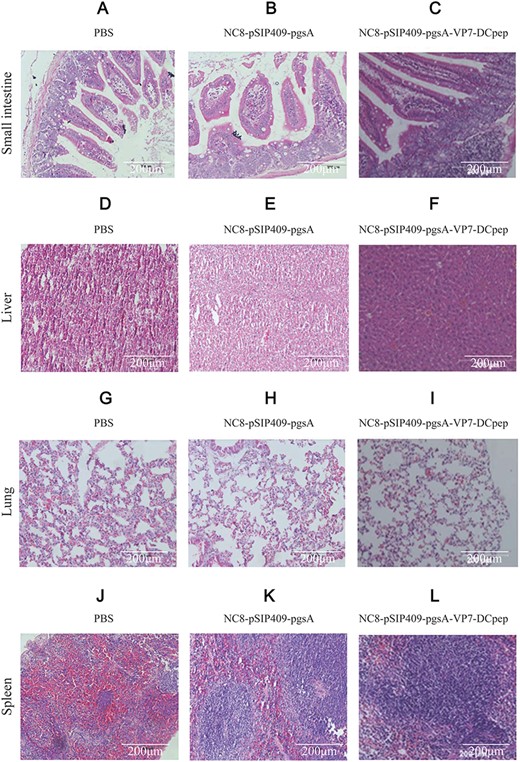
Histopathological changes in mice infected with the DN30209 Chinese PRV isolate strain following immunization (A–C) Small intestine. (D–F) Liver. (G–I) Lung. (J–L) Spleen. A, D, G, and J represent mice treated with PBS; B, E, H, and K represent mice immunized with empty vector; and C, F, I, and L represent mice immunized with recombinant NC8-pSIP409-pgsA-VP7-DCpep.
Discussion
In the prevention of infections, mucosal immunity is the primary barrier present in the mouth, genital tracts and respiratory system. Traditional vaccines are mainly immunized by intramuscular injection. However, oral vaccines are more convenient and time-saving. As delivery vectors for oral vaccines, bacterial vectors have attracted attention due to their advantages of easy cultivation and immunity. Attenuated pathogens, such as Salmonella and Listeria, can be used as vaccine carriers [30]. However, the application of attenuated pathogenic bacteria is limited because of their independent ancestry. However, probiotics, such as lactic acid bacteria, can be used in animals and humans because of their safety characteristics. L. lactis and Lactobacillus lactis are the most studied L. lactis vaccines [13,31]. The induction of mucosal immunity is the major effect stimulated by oral immunization because it induces sIgA production and stimulates immunity systemically. However, pathogens, such as Streptococcus pyogenes and Streptococcus pneumoniae, are also generally recognized as safe [32,33]. Some specific strains of lactic acid bacteria, Lactobacillus, Streptococcus, and Bifidobacteria are probiotics in humans and animals, maintaining gastrointestinal homeostasis, affecting other organs to produce biologically active metabolites and regulating immune parameters and intestinal tract [32,33]. Permeability plays an important role. Lactobacillus can be effectively colonized in the host cavity and plays a vital role in maintaining the balance of the natural flora. Adhesion is an important property for recombinant lactic acid bacterial vaccines because adhesion ensures long-term presence of the vaccines in the host and may extend the time the antigen enters the immune system [34]. The natural adjuvant activity of lactic acid bacteria makes it attractive in the development of oral vaccines. More importantly, lactic acid bacteria can express a variety of foreign antigens and have a certain protective effect. All of the abovementioned antigens expressed in lactic acid bacteria have a certain protective effect, which makes lactic acid bacteria more attractive and a promising host for oral vaccine production and delivery [32,33]. A live expression vector can both correctly carry and survive in the intestinal environment and express a heterogenic antigen [35,36]. We examined the possible binding and stability of orally administered NC8-pSIP409-pgsA-VP7-DCpep in the intestine of mice. NC8-pSIP409-pgsA is an ideal expression system, as it survives in the intestinal mucosa and is safe. Additionally, the use of NC8-pSIP409-pgsA in the food industry represents a beneficial use of these types of organisms. NC8-pSIP409-pgsA is one of the most utilized species in food industry, medicine, and fermentation. NC8-pSIP409-pgsA rapidly grows and is simple to process, making it a perfect expression vector for exogenous gene products [36,37]. Oral vaccination in mice with the NC8-pSIP409-pgsA strain as an expression vector for the rotavirus VP7 surface antigen was effective and safe. Oral immunization with the recombinant strain elicited specific humoral and cellular immunity.
Many protective antigens can be expressed in L. lactis [38,39] and Lactobacillus lactis [40–42]. In addition, L. lactis in Lactobacillus can express rotavirus proteins, such as VP6 and VP7, which allows more effective delivery of the plasmid DNA to the host cells, improving the expression of exogenous antigens and enhancing humoral immune response. In our lab, we mainly use L. plantarum NC8 as host bacteria to deliver protective antigens, such as avian influenza, Newcastle disease, transmissible gastroenteritis, and porcine epidemic diarrhea [26,43–46]. The construction of recombinant-expressing heterologous pathogen antigens has been performed in several lactobacilli, such as the cholera toxin B subunit, transmissible gastroenteritis coronavirus spike glycoprotein, and the S. pneumoniae antigens PsaA and PspA [47,48]. For mucosal immunization, organisms have been revealed to be good live vehicles for the delivery of antigens. In the present study, we established that the NC8-pSIP409-pgsA-VP7-DCpep recombinant strain may be used to produce protective anti-VP7 antibodies and stimulate mucosal and systemic immunization following mucosal vaccination in a mouse model.
In addition, induction of antigen-presenting cells and immunostimulatory responses can be regulated by probiotic vaccines, such as DCs and macrophages [49]. Some studies have noted that the immunostimulatory role has tissue-specific differences in DC level and in stimulating the responses of T helper cells [50,51]. Splenic DCs specifically induce Th1 responses, while DCs isolated from mucosal tissues are involved in the promotion of Th2 responses. Our focus was on the variation in the IFN-γ and IL-4 levels in the serum. When activated in vivo, DCs stimulate T cells to differentiate into helper T cells, such as Th1 and Th2, which play an important role in secreting IFN-γ and IL-4 cytokines, respectively. After the activation of DCs, VP7-expressing L. plantarum cells stimulate T cells to secrete more IL-4, which results in T cell differentiating into Th2. This result is consistent with previously reports showing that the production of IFN-γ and IL-4 cytokines was increased [52–54]. Therefore, we speculate that although the VP7-expressing L. plantarum vector may be limited as a DNA vaccine vector, the presence of VP7-DCpep may play an adjuvant role in the co-expression of exogenous antigens.
Antibody-mediated passive immunization can provide protection against infection by invading pathogens [55]. Therefore, it is important to develop novel passive immunization approaches to prevent PRV infection. PRV VP7 is capable of inducing neutralizing antibodies and mediating adaptive immune responses [7]. Our results demonstrated that the use of NC8-pSIP409-pgsA-VP7-DCpep as the PRV VP7 antigen following oral vaccination in mice was efficient and safe. Following immunization with NC8-pSIP409-pgsA-VP7-DCpep, all mice were in a good condition. None of the mice showed any illness, and none of the mice died. Because no studies have been conducted on recombinant NC8-pSIP409-pgsA-VP7-DCpep, we mainly explored the role of VP7-expressing L. plantarum in cellular and humoral immunity, such as differentiation of helper T cells and B cells. According to the ELISA results, the antibody titers of VP7-specific IgG (P=0.0012) and sIgA (P<0.001) in serum were increased in mice treated with NC8-pSIP409-pgsA-VP7-DCpep compared to mice treated with the empty vector. NC8-pSIP409-pgsA-VP7-DCpep increased the number of B220+ B cells in the PP junction, promoted the production of VP7-specific antibodies, and improved the specific humoral immune response. The production of B220+ B cells was also significantly increased in MLNs (P<0.001) and in PPs (P<0.001).
We mainly explored the role of VP7-expressing L. plantarum in cellular and humoral immunity, such as activation of helper T cells and B cells. First, VP7-expressing L. plantarum enhanced the infection protection in vivo, as confirmed by flow cytometry. At the same time, VP7-expressing L. plantarum increased the expression of IL-4 by approximately 3 folds, suggesting that the infection protection may be related to the production of IL-4. It has been reported that IL-4 is related to the efficiency of the vaccine [56,57]. L. plantarum strains can be used as delivery vectors for oral vaccines due to their probiotic properties, and the VP7 protein can change the probiotic characteristics of L. plantarum. Moreover, the safety of this system has also attracted attention. Pathological analysis confirmed that the expression of VP7 on L. plantarum surface was safe and did not cause pathological damage. L. plantarum expressing VP7 activates DCs in vivo and promotes the differentiation of CD4+ T cells into Th1 and Th2, and it further promotes the expression of B220+ B cells in PP junctions, promotes the production of VP7-specific antibodies, and improves the specific humoral immune response. Future studies should concentrate on investigating the NC8-pSIP409-pgsA-VP7-DCpep recombinant strain vaccine in a porcine model of the disease as a means of evaluating the efficiency of the formulation against infections in its natural host.
Supplementary Data
supplementary data is available at Acta Biochimica et Biophysica Sinica online.
Funding
This work was supported by the grants from the National Key Research and Development Program of China (Nos. 2017YFD0501000, 2017YFD0501200, and 2017YFD0500400), the National Natural Science Foundation of China (No. 31672528) and the Science and Technology Development Program of Jilin Province (Nos. 20180201040NY, 20190301042NY, and 20200402041NC).
Conflict of Interest
The authors declare that they have no conflict of interest.
References
Author notes
Seria Masole Shonyela, Chunwei Shi contributed equally to this work.