-
PDF
- Split View
-
Views
-
Cite
Cite
Vincent P. Willard, Holly A. Leddy, Daniel Palmer, Chia-Lung Wu, Wolfgang Liedtke, Farshid Guilak, Transient Receptor Potential Vanilloid 4 as a Regulator of Induced Pluripotent Stem Cell Chondrogenesis, Stem Cells, Volume 39, Issue 11, November 2021, Pages 1447–1456, https://doi.org/10.1002/stem.3440
- Share Icon Share
Abstract
Transient receptor potential vanilloid 4 (TRPV4) is a polymodal calcium-permeable cation channel that is highly expressed in cartilage and is sensitive to a variety of extracellular stimuli. The expression of this channel has been associated with the process of chondrogenesis in adult stem cells as well as several cell lines. Here, we used a chondrogenic reporter (Col2a1-GFP) in murine induced pluripotent stem cells (iPSCs) to examine the hypothesis that TRPV4 serves as both a marker and a regulator of chondrogenesis. Over 21 days of chondrogenesis, iPSCs showed significant increases in Trpv4 expression along with the standard chondrogenic gene markers Sox9, Acan, and Col2a1, particularly in the green fluorescent protein positive (GFP+) chondroprogenitor subpopulation. Increased gene expression for Trpv4 was also reflected by the presence of TRPV4 protein and functional Ca2+ signaling. Daily activation of TRPV4 using the specific agonist GSK1016790A resulted in significant increases in cartilaginous matrix production. An improved understanding of the role of TRPV4 in chondrogenesis may provide new insights into the development of new therapeutic approaches for diseases of cartilage, such as osteoarthritis, or channelopathies and hereditary disorders that affect cartilage during development. Harnessing the role of TRPV4 in chondrogenesis may also provide a novel approach for accelerating stem cell differentiation in functional tissue engineering of cartilage replacements for joint repair.
Transient receptor potential vanilloid 4 (TRPV4) is an osmo- and mechanosensitive cation channel that is highly expressed in chondrocytes. This study examined the hypothesis that TRPV4 serves as a marker as well as a regulator of chondrogenesis in induced pluripotent stem cells. During the process of chondrogenesis, Trpv4 expression mirrored the expression of classic chondrogenic markers (e.g., Sox9, Acan, and Col2a1). Furthermore, intermittent daily activation of TRPV4 significantly increased cartilaginous matrix production. An improved understanding of the role of TRPV4 in chondrogenesis may provide new insights into the development of new therapeutic approaches for diseases that affect cartilage.
INTRODUCTION
Transient receptor potential vanilloid 4 (TRPV4) is a polymodal calcium-permeable cation channel that is sensitive to a variety of extracellular stimuli and can serve as an integrator of physical and chemical signals in the cellular microenvironment. First discovered in 2000,1,2 TRPV4 was initially found to serve as a transducer of osmotic signals.3-5 It has since been demonstrated to function in signaling related to pain and inflammation.6-8 Subsequently, TRPV4 has been found to be expressed and functionally relevant in several musculoskeletal tissues, including cartilage,9-11 bone,12,13 intervertebral disc,14 and synovium,15,16 where it plays highly complex and diverse roles in different contexts.
In particular, TRPV4 is highly expressed in cartilaginous tissues and is essential in skeletal development as well as joint homeostasis. For example, gain-of-function mutations in TRPV4, termed “channelopathies,” can result in skeletal dysplasias that range from mild deformities to neonatal lethality.17-21 In chondrocytes, TRPV4 helps to maintain cartilage homeostasis by serving as a mechanosensor, secondary to osmotic changes in the cartilage matrix caused by mechanical loading.11 In this respect, TRPV4 has been shown to regulate the anabolic response of chondrocytes to compressive loading.22 The global loss-of-function of TRPV4 (eg, Trpv4−/− mice) results in premature cartilage loss secondary to bone remodeling,9 whereas cartilage-specific deletion of Trpv4 appears to protect against age-related osteoarthritis,23 revealing the diverse function of this ion channel in different tissues.24
The effects of TRPV4 on skeletal development appear to involve the regulation of stem and progenitor cell differentiation. In ATDC5 and C3H10T1/2 cell lines undergoing chondrogenesis, the expression pattern of TRPV4 was found to be similar to those of chondrogenic marker genes, such as type II collagen (Col2a1) and aggrecan (Acan).10 A similar pattern of TRPV4 expression has also been observed in human induced pluripotent stem cells (iPSCs), mesenchymal stem cells (MSCs), and adipose stem cells (ASCs) undergoing chondrogenesis.25-28 Furthermore, ASCs isolated from Trpv4−/− mice exhibit reduced chondrogenic differentiation potential and enhanced osteogenic potential compared to ASCs from Trpv4+/+ mice.29 Interestingly, the chemical activation of TRPV4 induced Sox9-dependent reporter activity,10 and iPSCs derived from patients with a lethal skeletal dysplasia caused by TRPV4 mutation show significantly decreased chondrogenesis and expression of COL2A1, SOX9, ACAN, type X collagen (COL10A1), and runt-related protein 2 (RUNX2).30 Taken together, these findings suggest that TRPV4 is not only a marker of differentiation, but could serve as a functional role in chondrogenesis, upstream of other chondrogenic genes such as SOX9.
Here, we examined the hypothesis that TRPV4 serves both as a marker and a regulator of chondrogenesis in iPSCs. Due to their virtually unlimited expansion and differentiation potentials, iPSCs can provide an excellent model system for studying cartilage development or disease modeling in vitro using genetically defined populations of cells.31 In this regard, iPSCs may provide an adaptable system to study the functional role of this channel under physiologic conditions as well as with TRPV4 channelopathies. However, even using clonal cell lines, iPSCs tend to exhibit heterogeneous differentiation.28 In this study, we used a Col2a1-GFP reporter in murine iPSCs32 to determine if TRPV4 is specifically expressed as a marker of the chondrogenic subpopulation sorted from differentiated iPSCs, and to determine if TRPV4 serves not only as a marker but also plays a functional role in regulating chondrogenesis in iPSC-derived chondrocytes. Understanding the role of TRPV4 in chondrogenesis will improve our understanding of cartilage development and hopefully lead to new methods to accelerate stem cell differentiation for applications in cartilage tissue engineering.
MATERIALS AND METHODS
iPSCs culture and differentiation
iPSCs were derived, cultured, and differentiated toward the chondrogenic lineage as previously described.31-33 Briefly, tail fibroblasts from adult C57BL/6 mice were reprogrammed to pluripotency with a single doxycycline-inducible lentiviral vector that expressed mouse cDNAs for Oct4 (Pou5f1), Sox2, Klf4, and c-Myc.34 Undifferentiated iPSCs were maintained on mitomycin C-treated mouse embryonic feeder (MEFs, MilliporeSigma, Burlington, Massachusetts) in iPSC media containing Dulbecco's modified Eagle medium-high glucose (DMEM-HG, Gibco, Waltham, Massachusetts), 20% lot-selected fetal bovine serum (FBS) (Atlanta Biologicals, Minneapolis, Massachusetts), 100 nM Modified Eagle Medium (MEM, Gibco), nonessential amino acids (NEAA, Gibco), 55 μm β-mercaptoethanol (2-me, Gibco), 25 ng/mL gentamicin (Gibco), and 1000 U/mL mouse leukemia inhibitory factor (LIF, MilliporeSigma). Nucleofection (Amaxa, Lonza, Basel, Switzerland) of a construct with the Col2a1 promoter driving green fluorescent protein (GFP)35 was used to generate iPSC clones with stable integration of the reporter. Chondrogenic differentiation was carried out in high density micromass culture for 15 days while cultured in serum-free chondrogenic medium containing DMEM-HG, NEAA, 2-me, insulin-transferrin-selenous acid (ITS+ premix, BD, Franklin Lakes, New Jersey), penicillin-streptomycin, 50 μg/mL L-ascorbic acid 2-phosphate, and 40 μg/mL L-proline. For days 3 to 5 only, 50 ng/mL murine BMP-4 (R&D Systems, Minneapolis, Minnesota) and 100 nM dexamethasone (Sigma, St. Louis, Missouri) were added to the medium. At 0, 7, 14, and 21 days, cells within the micromasses were lysed, total RNA was isolated (Norgen Biotek), and cDNA was generated using superscript VILO (Life Technologies, Carlsbad, California). Quantitative reverse transcription polymerase chain reaction (qRT-PCR) was performed using SYBR Green (Applied Biosystems, Waltham, Massachusetts) and gene-specific primers for Nanog, Sox2, Vim, Sox9, Acan, Col2a1, and Trpv4, provided in Table 1. Fold changes in gene expression were calculated with the 2−∆∆Ct method using 18S rRNA as a house-keeping gene. At each time point, additional micromasses were lysed with RIPA buffer containing protease and phosphatase inhibitors (Sigma). Thirty μg of total protein per sample was used for Western blots against SOX2 (#14962), TRPV4 (#65893), and β-actin (#8457) (all antibodies from Cell Signaling Technology, Danvers, Massachusetts). Finally, micromasses at each time point were fixed with 4% paraformaldehyde and stained for sulfated glycosaminoglycan (sGAG) with Alcian blue as an indicator of chondrogenesis. To ensure reproducibility of the results, the full differentiation process from pluripotent cells to micromasses, GFP sorting, and subsequent culture (as described below) was repeated three times. The total sample sizes for each assay (indicated in the figure legends) include biological replicates collected over the course of three separate differentiation experiments.
Target . | Forward primer . | Reverse primer . |
---|---|---|
r18s | 5′-CGGCTACCACATCCAAGGAA-3′ | 5′-GGGCCTCGAAAGAGTCCTGT-3′ |
Nanog | 5′-GCACTCAAGGACAGGTTTCA-3′ | 5′-CGCTTGCACTTCATCCTTTG-3′ |
Sox2 | 5′-AACGCCTTCATGGTATGGTC-3′ | 5′-CTCGGTCTCGGACAAAAGTT-3′ |
Vim | 5′-CAAACGAGTACCGGAGACAG-3′ | 5′-TAGCAGCTTCAAGGGCAAAA-3′ |
Sox9 | 5′-GAAGTCGGTGAAGAACGGAC-3′ | 5′-CAGCGCCTTGAAGATAGCAT-3′ |
Acan | 5′-GCATGAGAGAGGCGAATGGA-3′ | 5′-CTGATCTCGTAGCGATCTTTCTTCT-3′ |
Col2a1 | 5′-TCCAGATGACTTTCCTCCGTCTA-3′ | 5′-AGGTAGGCGATGCTGTTCTTACA-3′ |
Trpv4 | 5′-TCTTCACCCTCACCGCCTACT-3′ | 5′-TCCACTGTGGTCCGGTAAG-3′ |
Target . | Forward primer . | Reverse primer . |
---|---|---|
r18s | 5′-CGGCTACCACATCCAAGGAA-3′ | 5′-GGGCCTCGAAAGAGTCCTGT-3′ |
Nanog | 5′-GCACTCAAGGACAGGTTTCA-3′ | 5′-CGCTTGCACTTCATCCTTTG-3′ |
Sox2 | 5′-AACGCCTTCATGGTATGGTC-3′ | 5′-CTCGGTCTCGGACAAAAGTT-3′ |
Vim | 5′-CAAACGAGTACCGGAGACAG-3′ | 5′-TAGCAGCTTCAAGGGCAAAA-3′ |
Sox9 | 5′-GAAGTCGGTGAAGAACGGAC-3′ | 5′-CAGCGCCTTGAAGATAGCAT-3′ |
Acan | 5′-GCATGAGAGAGGCGAATGGA-3′ | 5′-CTGATCTCGTAGCGATCTTTCTTCT-3′ |
Col2a1 | 5′-TCCAGATGACTTTCCTCCGTCTA-3′ | 5′-AGGTAGGCGATGCTGTTCTTACA-3′ |
Trpv4 | 5′-TCTTCACCCTCACCGCCTACT-3′ | 5′-TCCACTGTGGTCCGGTAAG-3′ |
Target . | Forward primer . | Reverse primer . |
---|---|---|
r18s | 5′-CGGCTACCACATCCAAGGAA-3′ | 5′-GGGCCTCGAAAGAGTCCTGT-3′ |
Nanog | 5′-GCACTCAAGGACAGGTTTCA-3′ | 5′-CGCTTGCACTTCATCCTTTG-3′ |
Sox2 | 5′-AACGCCTTCATGGTATGGTC-3′ | 5′-CTCGGTCTCGGACAAAAGTT-3′ |
Vim | 5′-CAAACGAGTACCGGAGACAG-3′ | 5′-TAGCAGCTTCAAGGGCAAAA-3′ |
Sox9 | 5′-GAAGTCGGTGAAGAACGGAC-3′ | 5′-CAGCGCCTTGAAGATAGCAT-3′ |
Acan | 5′-GCATGAGAGAGGCGAATGGA-3′ | 5′-CTGATCTCGTAGCGATCTTTCTTCT-3′ |
Col2a1 | 5′-TCCAGATGACTTTCCTCCGTCTA-3′ | 5′-AGGTAGGCGATGCTGTTCTTACA-3′ |
Trpv4 | 5′-TCTTCACCCTCACCGCCTACT-3′ | 5′-TCCACTGTGGTCCGGTAAG-3′ |
Target . | Forward primer . | Reverse primer . |
---|---|---|
r18s | 5′-CGGCTACCACATCCAAGGAA-3′ | 5′-GGGCCTCGAAAGAGTCCTGT-3′ |
Nanog | 5′-GCACTCAAGGACAGGTTTCA-3′ | 5′-CGCTTGCACTTCATCCTTTG-3′ |
Sox2 | 5′-AACGCCTTCATGGTATGGTC-3′ | 5′-CTCGGTCTCGGACAAAAGTT-3′ |
Vim | 5′-CAAACGAGTACCGGAGACAG-3′ | 5′-TAGCAGCTTCAAGGGCAAAA-3′ |
Sox9 | 5′-GAAGTCGGTGAAGAACGGAC-3′ | 5′-CAGCGCCTTGAAGATAGCAT-3′ |
Acan | 5′-GCATGAGAGAGGCGAATGGA-3′ | 5′-CTGATCTCGTAGCGATCTTTCTTCT-3′ |
Col2a1 | 5′-TCCAGATGACTTTCCTCCGTCTA-3′ | 5′-AGGTAGGCGATGCTGTTCTTACA-3′ |
Trpv4 | 5′-TCTTCACCCTCACCGCCTACT-3′ | 5′-TCCACTGTGGTCCGGTAAG-3′ |
Trpv4 expression in sorted chondroprogenitor subpopulations of iPSCs
To determine the specificity of Trpv4 expression to the chondrogenic subpopulation of iPSCs, a Col2a1-GFP reporter integrated in the cells was used to sort the chondrogenic cell population (GFP+) from the nonchondrogenic cells (GFP−) as described previously.32 At the end of the 15-day culture period, micromasses were digested and single cells were sorted on the basis of positive GFP expression using flow cytometry. GFP+ cells were expanded for two passages in chondrogenic medium with the addition of 10% FBS and 4 ng/mL basic fibroblast growth factor (Roche). After sorting, cells were lysed for RNA isolation and subsequent qRT-PCR to measure expression of Nanog, Sox9, Acan, Col2a1, and Trpv4 as described above, or seeded on gelatin-coated coverslips for 48 hours for TRPV4 immunocytochemistry. Cells were fixed with 4% paraformaldehyde, permeabilized, and labeled with Hoechst 33342 and an anti-TRPV4 antibody (#ACC-034, Alomone Labs, Jerusalem, Israel). Undifferentiated iPSCs and knee articular chondrocytes (enzymatically isolated from the femoral condyles of 12-day old C57BL/6 mice) served as controls.
Ca2+ signaling and functional activity of TRPV4
To determine the expression and functional activity of TRPV4, samples were collected and sorted into GFP+ and GFP− groups. Cells were expanded for 48 hours, and TRPV4-mediated Ca2+ signaling was measured in the sorted cell populations using confocal calcium ratio imaging or a fluorometric imaging plate reader. For confocal imaging, cells were seeded onto gelatin-coated coverslips at 10 k cells/cm2. Cells were loaded with Ca2+ sensitive dyes, Fluo4-AM and Fura Red (ThermoFisher Scientific, Waltham, Massachusetts),31 and imaged using a laser scanning confocal microscope (LSM 510, Zeiss). After measuring dose-dependent response to 0, 0.1, 1, 10, or 100 nM GSK1016790A (GSK101), a TRPV4-specific agonist, cells were stimulated with 0, 1, or 10 nM GSK101 to induce Ca2+ signaling. Undifferentiated iPSCs and primary articular chondrocytes from 12-day-old mice served as controls. To determine the mechanisms of Ca2+, cells were seeded into gelatin-coated 96-well plates at 10 k cells/cm2 and imaged using a fluorometric imaging plate reader (FLIPR, Molecular Devices). To determine the source of Ca2+ flux, cells were treated with 10 nM GSK101 with or without 10 μM GSK205 (TRPV4-specific inhibitor), 3 μM thapsigargin (inhibitor of intracellular Ca2+ release; MilliporeSigma), or in Ca2+-free medium containing 10 mM EGTA (MilliporeSigma).11,36
Effects of dynamic TRPV4 activation on chondrogenesis and matrix formation
To determine if dynamic TRPV4 activation could stimulate chondrogenesis and cartilage matrix accumulation, GFP− and GFP+ populations were sorted based on the Col2a1-GFP reporter. The cells were subsequently passaged twice and then reformed in micromass culture. For 7 days, micromasses were cultured in chondrogenic medium containing 10 ng/mL transforming growth factor beta 3 (TGF-β3) and 10 ng/mL bone morphogenetic protein 6 (BMP-6) (R&D Systems) to induce chondrogenesis. These growth factors were then removed, and the micromasses were cultured for 14 more days with daily treatment of either 10 nM GSK101, or 10 nM GSK101 + 10 μM GSK20536 for 1 hour per day. After the 1-hour treatment, micromasses were washed with warm phosphate buffered saline and fed fresh culture medium. On day 21, micromasses were digested in papain (MilliporeSigma) overnight and then analyzed using the PicoGreen assay (Life Technologies) to measure double-stranded DNA, the hydroxyproline assay for measuring total collagen content, and the dimethylmethylene blue assay for measuring the total sulfated glycosaminoglycan content of tissues.31,32 To determine the chondrogenic response to GSK101 treatment at the transcriptional level, cells within micromasses were lysed for RNA isolation and subsequent qRT-PCR as described previously, using the gene-specific primers for Sox9, Acan, and Col2a1 from Table 1. Additional micromasses were fixed with 4% paraformaldehyde, paraffin embedded, and cross-sections were cut at 8-μm thickness. The resulting slides were stained with Safranin-O/fast green/hematoxylin. Immunohistochemistry for collagens type I (#72026, Cell Signaling Technology) and II (#II-II6B3, Developmental Studies Hybridoma Bank, Iowa City, Iowa) was performed as described previously.32 Tibial plateaus from adult C57BL/6 mice served as positive controls for osteochondral staining.
Statistical analysis
Statistical analysis was performed using a one-way analysis of variance with a Tukey's HSD post hoc test where applicable (α = .05). Chi-square analysis was used for confocal calcium ratio imaging to determine differences in the percentage of cells responding to GSK101 (α = .05).
RESULTS
Trpv4 expression increases during chondrogenesis of iPSCs
Over 21 days of micromass culture, iPSCs underwent chondrogenic differentiation as exhibited by increased Alcian blue staining (Figure 1A). Quantitative RT-PCR showed significant decreases in expression for the pluripotency genes Nanog and Sox2 during this process, while the mesenchymal marker Vim and chondrogenic markers Sox9, Acan, and Col2a1 were increased by approximately 20-, 100-, 90-, and 1600-fold, respectively (Figure 1B). Concomitantly, Trpv4 expression increased by over 50-fold during chondrogenesis. Western blot analysis of SOX2 and TRPV4 confirmed that these changes in gene expression resulted in accompanying changes in protein production during micromass differentiation (Figure 1C).
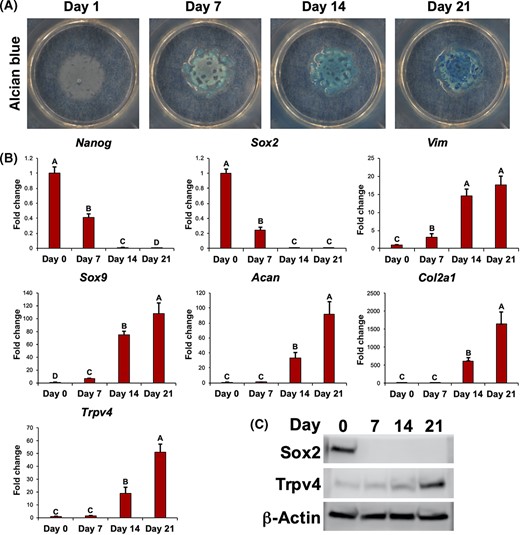
Trpv4 expression during chondrogenesis of induced pluripotent stem cells (iPSCs). A, Alcian blue staining of iPSCs undergoing chondrogenic differentiation over 21 days. B, Gene expression for pluripotency genes Nanog and Sox2, mesenchymal marker Vim, and chondrogenic markers Sox9, Acan, and Col2a1, and Trpv4. Mean ± SEM, n = 5 per group, groups not sharing the same letter are statistically different from one another (analysis of variance with Tukey's post hoc, P < .05). C, Western blots for SOX2 and TRPV4, as well as loading control β-actin
Trpv4 expression is specific to the chondrogenic cell population identified by a Col2a1-GFP reporter
Following 15 days of chondrogenic culture in micromass, cells were enzymatically isolated and sorted into GFP+ and GFP− populations based on a Col2a1-GFP reporter. Expression for the pluripotency gene Nanog decreased significantly for both sorted populations and primary chondrocytes, as compared to undifferentiated iPSCs (Figure 2). GFP+ cells showed significantly increased Sox9, Acan, Col2a1, and Trpv4 expression as compared to GFP− cells or undifferentiated iPSCs. GFP+ cells also showed higher Sox9 and Trpv4 expression, but lower Acan and Col2a1 expression relative to primary chondrocytes. Immunocytochemistry labeling for TRPV4 protein confirmed that expression was specific to the GFP+ population (Figure 2).
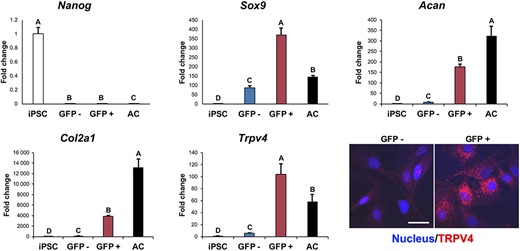
Trpv4 expression within chondrogenic cell population identified by Col2a1-GFP reporter. Induced pluripotent stem cells (iPSCs) underwent chondrogenesis for 15 days and were sorted into GFP+ and GFP− populations based on a Col2a1-GFP reporter before quantitative reverse transcription polymerase chain reaction (qRT-PCR) gene expression analysis for pluripotency gene Nanog, chondrogenic markers Sox9, Acan, and Col2a1, and Trpv4. Controls: undifferentiated iPSCs and isolated primary murine articular chondrocytes (AC). Mean ± SEM, n = 5, groups not sharing the same letter are statistically different from one another (analysis of variance with Tukey's post hoc, P < .05). Bottom right: GFP+ cells also showed positive immunolabeling for TRPV4 protein, while GFP− cells had little to no staining (nucleus stained with Hoechst 33342)
GFP+ chondroprogenitor cells exhibit TRPV4-mediated Ca2+ signaling
Ca2+ measurements indicated that GFP+ cells had a higher level of basal signaling compared to the GFP− cells or undifferentiated iPSCs (Figure 3A). GFP+ chondroprogenitor cells and primary chondrocytes showed significantly higher TRPV4-mediated Ca2+ signaling with GSK101 treatment as compared to iPSCs or GFP− cells using either confocal fluorescence or FLIPR assays (Figure 3). The GFP+ population also increased Ca2+ signaling in response to a low dose of GSK101, while the GFP− and iPSC groups only increased signaling in response to a high dose of GSK101 (Figure 3B,C). Calcium signaling showed a clearly dose-dependent response to GSK101, statistically significant at 10 nM GSK101 (Figure 3D). TRPV4-mediated Ca2+ signaling was inhibited by the TRPV4-specific inhibitor GSK205 and by removal of Ca2+ from the extracellular medium, indicating TRPV4-mediated Ca2+ influx was necessary for the observed signaling response (Figure 3E). Thapsigargin treatment did not result in a statistically significant inhibition of Ca2+ signaling.
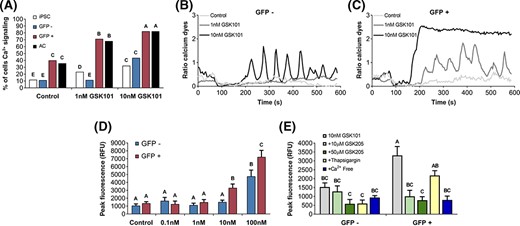
TRPV4-mediated Ca2+ signaling. A, GFP-sorted induced pluripotent stem cell (iPSC)-derived chondroprogenitor cell response to TRPV4-mediated Ca2+ signaling with GSK1016790A (GSK101) treatment measured by confocal cell traces, as compared to undifferentiated iPSCs or primary murine articular chondrocytes (AC) (N = 3 experiments, n = 78-161 cell traces per group; groups not sharing the same letter are statistically different from one another by Chi-square test, P < .05). Representative Ca2+ traces in response to increasing concentrations of GSK101 in (B) GFP− and (C) GFP+ chondroprogenitor cells. D, Fluorometric imaging plate reader (FLIPR)-based assay dose-response of GFP− and GFP+ populations in response to GSK101 (n = 8). E, TRPV4-mediated Ca2+ signaling response to TRPV4-specific inhibitor GSK205, thapsigargin, and removal of Ca2+ from the extracellular medium (Ca2+ Free) as measured by FLIPR (n = 5). Mean ± SEM, groups not sharing the same letter are statistically different from one another by analysis of variance with Tukey's post hoc, P < .05
Transient activation of TRPV4 enhances cell proliferation and chondrogenesis in iPSC-derived chondroprogenitors
GFP+ and GFP− cells were formed into micromasses and were treated with GSK101 or GSK101+GSK205 for 1 hour per day, in the absence of chondrogenic growth factors. In control conditions, micromasses generated from GFP+ cells contained higher sGAG/DNA ratio than micromasses generated from GFP− cells, consistent with previous findings.32 Activation of TRPV4 with GSK101 significantly increased DNA content as well as sGAG/DNA ratio compared to control conditions or treatment with GSK101 and GSK205, notably only in micromasses containing GFP+ cells (Figure 4B). Total collagen/DNA was not affected, underscoring the specificity of the observed response as well as the general metabolic health of the GFP− cells. qRT-PCR revealed similar results at the transcriptional level, with GFP+ cells displaying significantly greater Sox9 and Acan expression in response to GSK101 treatment compared to controls conditions, consistent with the observed increase in sGAG production (Figure 4C). These changes were not observed in the GFP− cells. Expression of Col2a1 was not significantly altered in either cell population in response to TRPV4 activation, consistent with no observed changes in total collagen production. Histologically, significant increases in tissue accumulation and Safranin-O staining were observed only in GFP+ micromasses treated with GSK101 (Figure 5). The stimulatory effects of GSK101 on the GFP+ cells were inhibited by the TRPV4 antagonist, GSK205. Across the treatment groups, micromasses formed from GFP+ cells stained strongly for collagen II with minimal collagen I signal, indicative of a chondrogenic phenotype (Figure 5). In contrast, GFP− micromasses stained strongly for collagen I with minimal collagen II staining.
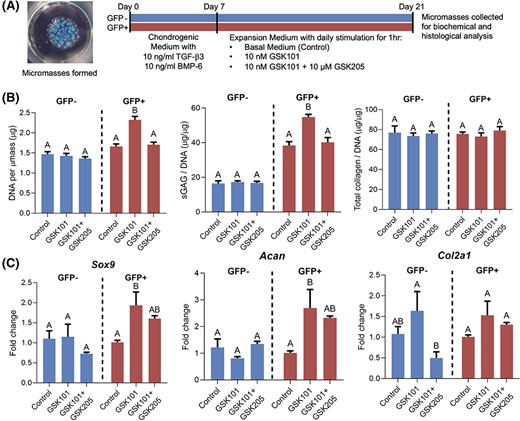
Cell proliferation, sulfated glycosaminoglycan (sGAG) production, and chondrogenic gene expression in induced pluripotent stem cell (iPSC)-derived chondroprogenitors after transient activation of TRPV4. A, GFP+ and GFP− cells were formed into micromasses and underwent chondrogenesis for 7 days before being treated with GSK1016790A (GSK101) or GSK101+GSK205 for 1 hour per day, in the absence of chondrogenic growth factors. B, Day 21 biochemical analysis for DNA, sGAG, and collagen content showed that activation of TRPV4 with GSK101 increased DNA content, sGAG/DNA ratio in GFP+ groups only. Total collagen/DNA was not affected. C, Quantitative reverse transcription polymerase chain reaction (qRT-PCR) at day 21 showed significantly increased Sox9 and Acan expression in GFP+ cells in response to GSK101 treatment compared to controls conditions. Col2a1 expression was not significantly altered in either cell population in response to TRPV4 activation. Mean ± SEM, n = 5-6 for qPCR, n = 5 for biochemical measurements, groups not sharing the same letter are statistically different from one another (analysis of variance with Tukey's post hoc, P < .05)
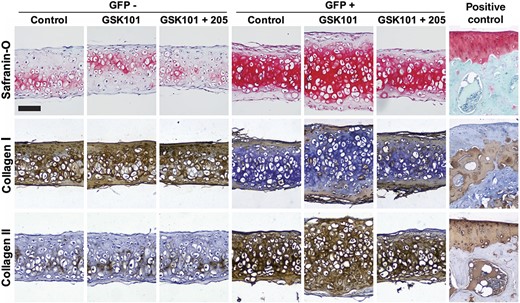
Tissue accumulation, Safranin-O staining, and collagen types I and II immunohistochemistry (IHC) in induced pluripotent stem cell (iPSC)-derived chondroprogenitors after transient activation of TRPV4. Increases in tissue thickness and Safranin-O labeling were observed in GSK101-treated GFP+ cells at day 21, and this effect was inhibited by the TRPV4 antagonist, GSK205. IHC revealed increased collagen type II staining in GFP+ micromasses, while GFP− micromasses displayed increased collagen type I staining. Positive controls were performed on the mouse tibial plateau. Scale bar = 100 μm
DISCUSSION
Our findings indicate that TRPV4 serves as critical regulator of chondrogenesis in iPSCs. TRPV4 expression increased over time in micromass-differentiated iPSCs, paralleling the expression of chondrogenic markers such as Sox9, Acan, and Col2a1. Importantly, this expression was specific to the chondrogenic cell population (GFP+) and absent in the nonchondrogenic population (GFP−). Furthermore, gene expression of Trpv4 led to functional expression of TRPV4 protein as well as the formation of functional ion channels, as verified by fluorescence imaging of Ca2+ signaling. Importantly, we found that TRPV4 serves not just as a marker of chondrogenesis, but also as a regulator of chondrogenesis and cartilage extracellular matrix synthesis. In the purified GFP+ chondrogenic cell population, daily activation of TRPV4 increased cell number and matrix production, mirroring the response of primary chondrocytes.22
TRPV4 gene and protein expression increased significantly and monotonically in iPSC micromasses as they underwent chondrogenesis. This finding is consistent with our previous observations in human stem cells, such as bone marrow-derived MSCs and ASCs, which exhibit increases of several orders of magnitude in Trpv4 expression during chondrogenesis.25-28 During development, Trpv4 is one of the earliest expressed genes in cartilage, appearing during mesenchymal condensations at 12.5 days post coitum.37 These findings suggest that in a wide range of stem/progenitor cells, TRPV4 serves as a marker of chondrogenesis, with expression patterns similar to classical chondrogenic marker genes such as Sox9, Col2a1, and Acan, providing an additional indicator of chondrogenic differentiation.
The purification of chondrogenic progenitors using a Col2a1-GFP reporter revealed that in the heterogeneous population of cells within a differentiating micromass, the gene and protein expression of TRPV4 as well as functional activity of TRPV4 was selectively localized to the GFP+ population. Furthermore, the GFP+ cells showed significantly higher sensitivity to the TRPV4 agonist, GSK101, as verified by single-cell and FLIPR-based imaging of Ca2+ signaling. This TRPV4-mediated Ca2+ signaling required influx of extracellular Ca2+, as the response was inhibited by GSK205 or removal of Ca2+ from the medium, but not by inhibition of intracellular Ca2+ release by thapsigargin. While using the Col2a1-GFP reporter provided a robust method for purifying the chondrogenic cell population, it also prevented us from validating these results using iPSC lines of different genetic backgrounds. We have recently published work describing the purification of highly chondrogenic cells from differentiated hiPSC lines with or without the use of a genetic reporter,28 allowing future studies to further validate these findings in human cells.
Not only was Trpv4 expression and function associated with chondrogenesis, we also observed that activation of TRPV4 signaling in GFP+ cells resulted in increased expression of chondrogenic marker genes as well as enhanced chondrogenic matrix production as observed by increased cell number and sGAG/DNA ratio. The increase in biosynthetic activity induced by the GSK101 TRPV4-activator was specific to TRPV4, as it was inhibited by GSK205, a TRPV4-selective antagonist. Increases in chondrogenic marker expression, matrix production, and cell proliferation were not observed in the GFP− (nonchondrogenic) cells after GSK101 stimulus, possibly due to the distinct Ca2+ signaling response of these cells, or due to this heterogeneous cell population having already differentiated into other cell lineages. The enhanced biosynthesis of GFP+ cells is consistent with previous studies showing that stimulation of TRPV4 with mechanical, osmotic, or chemical activators results in a strong anabolic response in primary chondrocytes, characterized by significant increases in extracellular matrix synthesis and accumulation.22,38,39 It is important to note that while mechanical factors can enhance chondrogenesis,40 the ability to accelerate cartilage tissue formation using a small molecule chemical agonist may have significant advantages over the use of growth factors or direct mechanical stimulation (eg, in a bioreactor), particularly for large, inhomogeneous, or anatomically shaped tissue grafts.41,42
The specific mechanisms involved in TRPV4 enhancement of chondrogenesis remain to be determined. Previous studies suggest that activation of TRPV4 drives expression of Sox9 via Ca2+/calmodulin signaling,10 which is known to play a role in cartilage homeostasis and interact with the TGF-β pathway.43,44 TGF-β and TRPV4 signaling pathways demonstrate synergistic transcriptomic profiles, including targets of SMAD3, JUN, and SP1, while also displaying Ca2+/calmodulin-dependence.44 Together with our results, these findings depict TRPV4 as an active member in chondrogenic development, where it directly drives chondrogenic gene expression, including Sox9, by Ca2+/calmodulin-transduced interaction with the TGF-β pathway.10,44 It is also of interest to note that activation of TRPV4 by GSK101 appears to require the presence of extracellular matrix molecules,22 particularly hyaluronic acid,45 which may be associated with the increased matrix production observed in GFP+ cells.
CONCLUSION
In summary, our findings indicate that TRPV4 serves both as a marker and a regulator of iPSC chondrogenesis. Within a heterogeneous population of cells in a micromass, the enhancement of chondrogenesis appears to occur specifically in the GFP+, chondroprogenitor cell population. An improved understanding of the role of TRPV4 in chondrogenesis promises to provide new insights into the development of new therapeutic approaches for diseases of cartilage such as osteoarthritis or hereditary disorders that affect cartilage during development.46,47 The role of TRPV4 signaling in iPSC-derived chondrocytes mimics that seen in native articular chondrocytes; thus, iPSC chondrogenesis could provide a model system for studying the role of TRPV4 and TRPV4 channelopathies in cartilage development and pathogenesis. Controlled modulation of activity of TRPV4 evolves as a promising novel approach for optimizing stem cell differentiation in functional tissue engineering of cartilage replacements for joint repair.
ACKNOWLEDGMENTS
The authors would like to thank Sara Oswald for outstanding editorial assistance. Supported in part by the Shriners Hospitals for Children and NIH grants AG15768, AG46927, AR072999, AR075899, AR074992, and AR073752.
AUTHOR CONTRIBUTIONS
V.P.W., D.P., C.L.W.: conception and design of project, data collection, editing and final approval of manuscript; H.A.L., data collection, editing and final approval of manuscript; W.L.: conception and design of project, financial support, editing and final approval of manuscript; F.G.: conception and design of project, financial support, manuscript writing, editing and final approval of manuscript.
DATA AVAILABILITY STATEMENT
The data that support the findings of this study are available from the corresponding author upon reasonable request.
REFERENCES
How to cite this article:
Author notes
CONFLICT OF INTEREST
V.P. Willard and F. Guilak are employees and shareholders of Cytex Therapeutics, Inc. The other authors declared no potential conflicts of interest.
Wolfgang Liedtke and Farshid Guilak contributed equally to this study.