-
PDF
- Split View
-
Views
-
Cite
Cite
Pal Boto, Timea Beatrix Gerzsenyi, Adel Lengyel, Balint Szunyog, Istvan Szatmari, Zbtb46-Dependent Altered Developmental Program in Embryonic Stem Cell-Derived Blood Cell Progenitors, Stem Cells, Volume 39, Issue 10, October 2021, Pages 1322–1334, https://doi.org/10.1002/stem.3424
- Share Icon Share
Abstract
Zbtb46 is a recently identified dendritic cell (DC)-specific transcription factor with poorly defined biology. Although Zbtb46 is highly expressed in conventional DCs, evidence also points to its presence in erythroid progenitors and endothelial cells suggesting that this factor might influence the early hematopoietic development. Here, we probe the effect of this transcription factor in embryonic stem cell (ESC)-derived blood cell progenitors using chemically inducible mouse cell lines. Unexpectedly, forced expression of this protein elicited a broad repressive effect at the early stage of ESC differentiation. Ectopic expression of Zbtb46 interfered with the mesoderm formation and cell proliferation was also negatively impacted. More importantly, reduced number of CD11b+ myeloid blood cells were generated from ESC-derived Flk1+ mesoderm cells in the presence of Zbtb46. Consistent with this finding, our gene expression profiling revealed that numerous myeloid and immune response related genes, including Irf8, exhibited lower expression in the Zbtb46-primed cells. Despite these repressive effects, however, Zbtb46 overexpression was associated with enhanced formation of erythroid blood cell colonies and increased adult hemoglobin (Hbb-b1) expression at the early phase of ESC differentiation. Moreover, elevated percent of CD105 (Endoglin) positive cells were detected in the Zbtb46-primed samples. In summary, our results support that Zbtb46 suppresses the ESC-derived myeloid development and diverts mesoderm cells toward erythroid developmental pathway. Moreover, our transcriptomic data provide a resource for exploration of the Zbtb46 regulatory network in ESC-derived progenitors.
Significance statement
The transcription factor Zbtb46 is hallmark of dendritic cells; however, the exact function of this protein is still poorly characterized. In this study, Zbtb46 was probed in embryonic stem cell-derived blood cell progenitors using chemically inducible transgenic cell lines. The authors found that forced expression of Zbtb46 profoundly suppressed the myeloid blood cell development; on the other hand, ectopic expression of this factor enhanced the erythroid colony formation and the adult hemoglobin expression. These findings suggest that Zbtb46 can act as a molecular regulator to redirect the early blood cell differentiation program in pluripotent stem cell-derived progenitors.
INTRODUCTION
Conventional dendritic cells (cDCs) represent a unique population of professional antigen presenting cells; however, it is still challenging to distinguish these immune cells from monocytes or macrophages. To demarcate DCs from other mononuclear phagocytic cells, DC-specific transcription factors have been identified.1 The transcription factor Zbtb46 (also knowns as zDC or Btbd4) is widely considered as a hallmark of this immune cell type because it is strongly expressed in cDCs but lacking in macrophages and monocytes.1-3 Importantly, in situ analysis with a Zbtb46-driven reporter demonstrated that the activity of this gene was correlated with DC phenotype in intact tissue environments.2 However, further investigations uncovered that the expression of Zbtb46 is not entirely restricted to cDCs. For example, Langerhans cells also express Zbtb46 and murine monocytes can give rise to Zbtb46+ DC-like cells especially in the presence of IL-4.2,4,5 In addition, Zbtb46 was also detected in erythroid progenitors, nonproliferating endothelial cells, and even hematopoietic stem cells.2,6 Despite the elevated expression on the DC compartment, Zbtb46 null animals exhibited a normal DC development and similar number of cDCs was detected in the gene deficient spleen or lymph nodes.2 On the other hand, a separate study found that huge number of monocyte-specific and maturation-related transcripts exhibited higher expression in Zbtb46 null cDCs, suggesting that without this transcription factor these immune cells are partially activated.7 Interestingly, Zbtb46 can also modulate the endothelial cell function, especially this gene interferes with the endothelial cell proliferation.8 In summary, multiple immunological pathways are regulated by Zbtb46 including DC maturation, but more studies are needed to define the function of this transcription factor especially outside the DC compartment.
In this study, the Zbtb46 transgene was induced during the embryonic stem cell (ESC)-derived DC (ES-DC) differentiation and analyzed the impact of this regulatory protein on blood cell progenitors. Strikingly, at the early stage of ESC differentiation, forced expression of Zbtb46 interfered with the myeloid blood cell development. On the other hand, ectopic expression of Zbtb46 was associated with enhanced CD105+ cell production and superior erythroid colony formation. Consistent with these findings, our global gene expression analysis revealed that the myeloid lineage determining transcription factor Irf8 was barely detected in the Zbtb46-programmed progenitors. On the other hand, Zbtb46-primed cells exhibited an elevated expression of adult type hemoglobin (Hbb-b1).
MATERIALS AND METHODS
ES cell culture and differentiation
Mouse ESCs were cultured as described previously with minor modifications.9 In brief, ESCs were maintained on mitomycin C (Merck Millipore, Burlington, Massachusetts) treated primary mouse embryonic fibroblast (MEF) feeder layer in knockout DMEM (Thermo Fisher Scientific) supplemented with 15% FBS (Thermo Fisher Scientific, Waltham, Massachusetts), 1000 U/mL LIF (Merck), 50 U/mL penicillin, and 50 mg/mL streptomycin (Merck). After 3 days, cells were harvested with 0.25% trypsin-EDTA (Thermo Fisher Scientific). Before differentiation, ESCs were expanded without MEF feeder cells on 0.1% gelatin (Merck) coated six-well plate in knockout DMEM in the presence of 200 to 300 μg/mL G418 (Thermo Fisher Scientific) to reselect the transgenic cells.10
For mesoderm and myeloid cell differentiation, an OP9 coculture-based protocol was applied.9,11 In short, ESCs were seeded onto previously plated OP9 feeder layers (typically, 40 000 OP9 cells plus 40 000 ESCs/well in six-well plate) and cultured in α-MEM (Thermo Fisher Scientific), supplemented with 20% FBS, 50 U/mL penicillin and 50 mg/mL streptomycin for 5 days; half the volume of media was replaced at day 3. At day 5, cells were harvested with 0.25% trypsin-EDTA and further cultured on OP9 feeder cells (40 000 OP9 cells plus 200 000 differentiated cells/well in six-well plates) in alpha-MEM medium containing 20% FBS, 50 ng/mL GM-CSF (PeproTech, London, UK), and 50 μM 2-ME (β-mercaptoethanol; Merck) for additional 3 to 6 days. To generate 14-day differentiated cells, myeloid progenitors were harvested at day 11 and further cultured for additional 3 days on new OP9 feeder cells (40 000 OP9 cells plus 200 000 differentiated cells/well in six-well plates) using the same conditions as for the 11-day differentiated cells. For some experiments, at day 5, Flk1+ cells were enriched with magnetic purification and the separated cells were further cultivated (50 000 Flk1+ cells/well in 24-well plates) without OP9 feeders for 1 or 3 days in alpha-MEM containing 20% FBS, 50 ng/mL GM-CSF, and 50 μM 2-ME.
Embryoid bodies (EBs) were created as described with minor modifications.12 EB differentiation (EBD) medium consisted of IMDM (Thermo Fisher Scientific) with 15% FBS, 200 ng/mL iron-saturated transferrin (Sigma, St. Louis, Missouri), 4.5 mM monothioglycerol (Sigma), and 50 ng/mL ascorbic acid (Sigma). ESCs were collected and plated in hanging drops at 100 cells per 10 μL drop in an inverted bacterial dish in EBD medium. EBs were harvested from the hanging drops at day 2 and transferred into 6 mL EBD medium in slowly rotating 6 cm dishes. At day 4, EBs were fed by exchanging half of their medium and harvested at day 4 or 6 with 0.25% trypsin-EDTA. For some experiments, the collected cells were further cultured on OP9 feeder cells (40 000 OP9 cells plus 200 000 cells/well in six-well plates) in alpha-MEM medium containing 20% FBS, 50 ng/mL GM-CSF, and 50 μM 2-ME for additional 3 days.
For neural progenitor cell (NPC) generation, mouse, ESCs were differentiated on 0.1% gelatin (Merck) coated six-well plates in a chemically defined synthetic medium (N2B27). Cells were cultured for 4, 6, or 8 days and the medium was replaced every 48 hours. N2B27 was prepared from 25 mL N2 medium combined with 25 mL B27 medium plus; in addition, the final solution contains 100 μM 2-ME. The N2 component was prepared from 25 mL DMEM/F12 with Glutamax + 0.5 mL N2 supplement; B27 was prepared from 25 mL Neurobasal Plus medium + 1 mL B27 Plus supplement (all ingredients from Thermo Fischer Scientific).
Generation of doxycycline inducible ESC clones
Targeting vectors were engineered that carry the murine Irf8, Zbtb46, EGFP, or a bicistronic Zbtb46-T2A-EGFP open reading frames. The coding sequences were amplified with FastStart High Fidelity PCR System (Roche, Basel, Switzerland) using Gateway-specific primers from mouse bone marrow-derived cDNAs or EGFP containing plasmids. For the bicistronic fragment generation, the coding sequences of the EGFP-T2A plus T2A-EGFP were amplified; thereafter, the two polymerase chain reaction (PCR) fragments were reamplified with Gateway compatible attB-attached primers. Primer sequences are shown in Table S1. The PCR products were subcloned into pDONR221 plasmid (Thermo Fischer Scientific). The entry clone was then recombined with a p2lox Gateway compatible vector.10,13
For generation of doxycycline-inducible ESC clones, the ZX1 mouse ESC line was used as previously described.9,10 In brief, p2lox targeting vectors were delivered into the ZX1 cells by electroporation by Neon Transfection System (Thermo Fischer Scientific). Thereafter, cells were seeded onto neomycin resistant MEF feeder layer and chemical selection was started for 8 days in knockout DMEM containing 300 μg/mL G418.
mRNA sequencing
RNA sequencing analysis was performed with the Illumina sequencing platform. TRI reagent (Molecular Research Center, Cincinnati, Ohio) was used for total RNA isolation. RNA sample quality was checked on Agilent BioAnalyzer. RNA-Seq libraries were prepared from total RNA using Ultra II RNA Sample Prep kit (New England BioLabs, Ipswich, Massachusetts) according to the manufacturer's protocol. Sequencing runs were executed on NextSeq 500 (Illumina, San Diego, California) using single-end 75 cycles sequencing. Library preparation, sequencing, and basic bioinformatics were performed by the Medical Genomics and Bioinformatics Core Facility of the University of Debrecen. Sequenced reads were aligned to the mm10 genome assembly (GRCm38) and bam files were generated. Raw sequencing data were deposited into the NCBI SRA database (PRJNA647717). Further transcriptomic analyses were executed with Strand NGS 3.4 software (Agilent Technologies, Santa Clara, California). To select changing genes, mRNA transcripts were filtered on gene expression setting 1 read density (normalized reads per kilobase of transcript per million mapped reads) cutoff level, and selected those entities for which at least 2 out of 15 samples had higher read density than the cutoff. Next, genes were filtered with Oneway ANOVA (corrected P-value cutoff: .05; fold change cutoff: 2; multiple testing correction: Benjamini-Hochberg) including all conditions. The final gene list was hierarchically clustered; similarity was measure by Squared Euclidean. To generate Zbtb46-regulated gene lists, first transcripts were filtered on gene expression using 1 read density cutoff level including only the 6 or 8-day differentiated samples. Those transcript entities were selected for which at least 2 out of 12 samples had higher read density than the cutoff. These data were further filtered by comparing the Zbtb46-induced and noninduced samples at day 6 or 8 using the following settings: twofold change or greater, P-value cutoff was .05 using paired T test. Finally, the 6- and 8-day filtered gene lists were combined and hierarchically clustered; similarity was measure by Squared Euclidean.
Quantitative real-time reverse transcription (RT)-PCR
TRI reagent was used for total RNA isolation. RT was performed with high capacity cDNA RT Kit (Thermo Fisher Scientific). Quantitative PCR was performed with LC480 or LC96 (Roche) PCR instruments. PCR conditions: 1 cycle for denaturation at 95°C for 60 seconds; 40 cycles at 95°C for 10 seconds, and 60°C for 30 seconds using Taqman (Thermo Fisher Scientific) hydrolysis probes. Assay IDs and specifications are listed in Table S2. Gene expression levels were determined and quantified by comparative threshold cycle method and normalized to ActB. RT-PCR reactions were carried out in triplicates plus one control reaction which was generated without reverse transcriptase. Relative expression was represented as mean ± SD.
Western blot analysis
Protein extracts derived from 40 000 cells were separated by electrophoresis on a 10% polyacrylamide gel and transferred to PVDF membrane (Pall Corporation, New York, New York). Membranes were probed with an anti-IRF8 polyclonal antibody (ab245607, Abcam, Cambridge, UK) and then reprobed with an anti-GAPDH monoclonal antibody (AM4300; Thermo Fisher Scientific).
Flow cytometry
Flow cytometric analyses and cell sorting were carried out with FACS Aria III (BD Biosciences, San Jose, California). For cell surface staining, 100 000 cells were incubated in 10 μL blocking buffer (CD16/32 Fc block solution diluted in PBS 1:100) for 15 minutes at 4°C. Thereafter, cells were incubated with antibodies for 30 minutes at 4°C. The following anti-mouse antibodies were used: Flk-1 (CD309)-BV421 (Avas 12α1), CD11b-BV711 (M1/70), CD105-BV711 (MJ7/18), and CD45-FITC (30-F11). Intracellular Zbtb46 staining was performed after surface staining with an anti-mouse Zbtb46-PE (U4-1374) antibody using the Transcription Factor Buffer Set (BD Biosciences) according to the manufacturer's instructions.
Magnetic cell separation
Flk-1 (CD309) positive cells were purified using CD309 MicroBead Kit (Miltenyi Biotec, Bergisch Gladbach, Germany) according to the manufacturer's instructions using MS separation column (Miltenyi Biotec) and OctoMACS separator.
Apoptosis and cell cycle assays
Apoptosis assay was performed using FITC Annexin V Apoptosis Detection Kit with propidium iodide (Biolegend, San Diego, California) according to the manufacturer's recommendations.
Cell distribution of cell cycle compartments was determined using DNA staining with propidium iodide. Briefly, samples were fixed with 70% ethanol then cells were washed and followed by the treatment of 50 μL RNase (100 μg/mL) and 200 μL propidium iodide (20 μg/mL) solutions. Samples were acquired on FACS Aria III.
Hematopoietic colony forming assay
Colony Forming Unit (CFU) assays were employed with Methocult GF M3434 semisolid medium (STEMCELL Technologies, Vancouver, Canada). Differentiated cells were harvested and resuspended in PBS, and then cells were mixed with M3434 medium and plated into 3 cm diameter cell culture dishes (volume 1.5 mL/well) at a final concentration of 5 × 104 cells/well. Colonies were scored after 8 days of culture.
Statistical analysis
Significant differences were calculated using two-tailed, unpaired Student's t test (*P < .05, **P < .01, ***P < .001).
RESULTS
Zbtb46-dependent reduced myeloid cell formation
We have recently quantified the mRNA level of 17 transcription factors in ex vivo differentiated murine ES-DCs.9 Some of these DC-affiliated transcription factors were highly expressed, but a few of them were barely detected. Interestingly, Zbtb46, a cDC marker, was also weakly expressed in ES-DCs and their progenitors.9 This finding inspired us to analyze the impact of this transcription factor during ES-DC differentiation using a gain of function approach. Zbtb46 inducible murine ESCs were engineered and several stable ESC clones were generated. After initial testing, two ESC clones (C2 and C4) were expanded and characterized. We confirmed that the transcript level of Zbtb46 was strongly upregulated after 2 days of doxycycline treatment in these cell clones (Figure 1A). In addition, the Zbtb46 protein was detected in 95% of the chemically induced cells (Figure 1B). Together, these data suggest that Zbtb46 can be robustly and uniformly induced in drug-controlled transgenic cells. Next, we evaluated the effects of this transgene during ex vivo ESC differentiation.
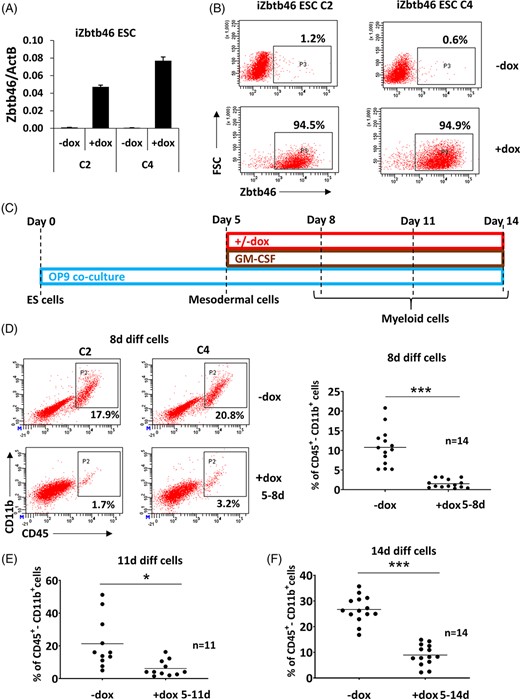
Zbtb46-inhibited myeloid cell production. Two Zbtb46-inducible embryonic stem cell (ESC) clones were tested (C2 and C4). A, Quantitative RT-PCR assay to measure the mRNA level Zbtb46. To induce the Zbtb46 transgene, ESCs were treated with doxycycline (+dox) for 48 hours. B, Plots show quantified protein expression of Zbtb46 in ESC clones (C2 and C4) at single cell level using intracellular flow cytometry (FSC, forward scatter). ESCs were treated with doxycycline for 48 hours. C, Experimental scheme of the Zbtb46-inducible ESC differentiation. Cells were harvested for flow cytometry at day 8, 11, or 14. D, Representative flow plots and statistics show the percentage of the CD45/CD11b double positive cell populations of 8-day differentiated cells. E,F, Statistics show the percentage of the CD45/CD11b double positive cell populations of 11- (E) and 14-day (F) differentiated cells. Cells were treated with doxycycline for the indicated time period
Zbtb46-inducible ESC clones were differentiated to ES-DC progenitors with an OP9-based coculture method.9 The induction of the transgene was started after mesodermal priming at day 5 and the differentiated cells were harvested at day 8, 11, or 14 (Figure 1C). Strikingly, minimal number of CD45+/CD11b+ cells were detected in the presence of Zbtb46 after 8 days of differentiation (Figure 1D), suggesting that ectopic expression of this transcription factor strongly represses the myeloid cell specification. At day 11, we still observed an impaired formation of myeloid cells, although the inhibition was less prominent (Figure 1E). Moreover, we also obtained an impaired myeloid cell production at day 14 (Figure 1F). Finally, myeloid cells were also generated with a distinct protocol: EBs were developed for 6 days, and the EB-derived cells were cultured on OP9 layers for 3 additional days (Figure S1A). In line with the previous findings, Zbtb46 negatively influenced the frequency of the CD45/CD11b+ cell fraction in EB-derived samples (Figure S1B). Collectively, these results suggest that the myeloid cell development is profoundly suppressed by Zbtb46.
Zbtb46 expressing cells inefficiently give rise to myeloid cells
In spite of the consistent inhibition, detectable number of CD45+ cells were obtained in the doxycycline treated cell culture especially at day 11 and 14, suggesting that a subpopulation can still give rise to myeloid cells. This phenomenon can be explained by a Zbtb46-dependent delayed myeloid development or a subset of the differentiated cells might fail to express the transgene. In case of undifferentiated ESCs, a more or less uniform Zbtb46 induction was detected (Figure 1B); however, the transgene might be silenced in differentiated cells. To test this possibility, we have assessed the intracellular level of the Zbtb46 protein together with staining the cell surface expression of CD11b in 11- and 14-day differentiated cells (Figure 2A,C). Indeed, Zbtb46 protein was only detected in 40% to 50% of the cells at day 11 and only 10% to 15% Zbtb46 expressing cells were obtained at day 14. More importantly, the great majority of the CD11b+ cells were Zbtb46 negative suggesting that Zbtb46-expressing progenitors are poorly converted to myeloid cells. To further substantiate this observation, inducible ESCs were constructed using a bi-cistronic transgene configuration in which Zbtb46 and EGFP can be coinduced with doxycycline treatment. As a further control, we also created purely EGFP-inducible ESC clones. Consistent with the intracellular Zbtb46 staining, the great majority of the emerged CD11b+ myeloid cells were GFP negative in the bi-cistronic transgenic cells (Figure 2). In contrast, GFP+/GFP− ratio of the CD11b+ cell fraction was much higher in the absence of the Zbtb46 transgene (Figure 2B,D). Together, these results revealed that Zbtb46 strongly inhibits the myeloid development and the CD11b+ cells are inefficiently emerged from Zbtb46-programmed cells.
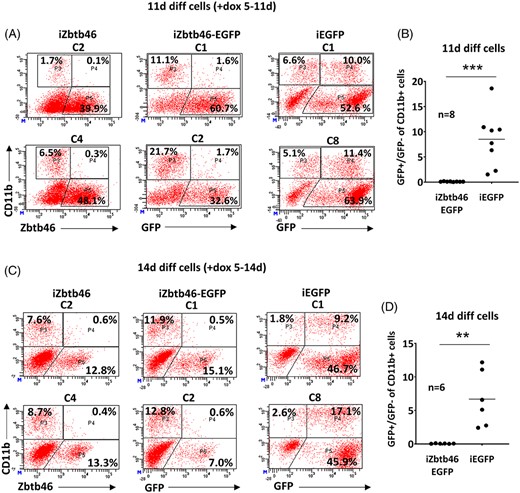
Assessment of Zbtb46/EGFP induction during ex vivo differentiation. A, Representative flow plots show quantified protein expression of Zbtb46 in 11-day differentiated Zbtb46 inducible cell clones (C2 and C4) with intracellular flow cytometry. These cells were also pre-stained with a CD11b-specific antibody. In addition, representative flow plots show the detection of CD11b and GFP in 11-day differentiated Zbtb46-EGFP coexpressing (C1 and C2) or EGFP expressing cell clones (C1 and C8) with flow cytometry. To induce the transgenes, cells were treated with doxycycline for 6 days (day 5-11). B, Statistics show the percentage of the GFP+/GFP− cell ratio of the CD11b+ cell fraction in 11-day differentiated and doxycycline treated (day 5-11) cells. C, Representative flow plots show protein expression of Zbtb46 or GFP as in part “A” in 14-day differentiated cells. To induce the transgenes, cells were treated with doxycycline for 9 days (day 5-14). D, Statistics show the percentage of the GFP+/GFP− cell ratios of the CD11b+ cell fraction in 14-day differentiated and doxycycline treated (day 5-14) cells derived from Zbtb46-EGFP or EGFP ESC clones
These results also imply that this transgenic system is limited for monitoring the impact of Zbtb46 at the late phase of the ESC-derived myeloid DC development, because the vast majority of the developed myeloid cells are Zbtb46 negative, especially at day 14. The other option would be to turn on the Zbtb46 transgene in committed myeloid cells at the late stage of cell differentiation. However, we failed to efficiently induce the Zbtb46 transgene with doxycycline treatment in 11-day differentiated cells (data not shown). This is not a Zbtb46-specific effect because an EGFP reporter was also poorly expressed when differentiated cells were treated with doxycycline at day 11 (data not shown). We conclude that this inducible system is not suitable to analyze the effects of Zbtb46 overexpression in committed myeloid cells; instead, these transgenic cells can be applied to monitor the early impact of Zbtb46 in mesoderm and pre-hematopoietic stage. Therefore, in this study we examined the effects of the ectopically expressed Zbtb46 during the early stage of ESC differentiation.
Purified Flk1+ mesoderm cells fail to give rise myeloid cells in the presence of Zbtb46
Next, we monitored the effects of Zbtb46 at the very early stage of ESC differentiation (days 3-5). Interestingly, lower percent of Flk1+ cells was produced at day 5 in the presence of Zbtb46 (Figure 3A) suggesting that this protein suppresses the myeloid development as well as mesoderm cell formation. This finding raised the possibility that the impaired myeloid cell development is just the consequence of the reduced mesoderm differentiation. Premesodermal cells can also be present in the culture at day 5 and those cells might be unable to convert into Flk1+ mesoderm cells, which are the precursors of the myeloid cells. To distinguish these two inhibitory mechanisms, Flk1+ cells were magnetically purified at day 5 and these committed cells were differentiated for 3 days in the presence of the transgenic Zbtb46 without OP9 feeder layer. Importantly, similar to the nonseparated cells, the purified Flk1+ cells also showed an impaired CD45+ cell formation in the presence of the transgene suggesting that myeloid differentiation of the already committed blood cell precursors is suppressed by Zbtb46 (Figure 3B). Moreover, these results also revealed that the Zbtb46-dependent suppressive effect can be detected without OP9 feeder cells.
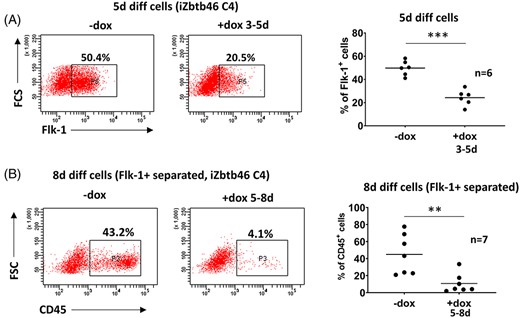
Zbtb46 inhibited mesodermal and myeloid cell production. A, Representative flow plots and statistics show the percentage of the Flk1+ cell populations of 5-day differentiated Zbtb46-inducible cell clones (FSC, forward scatter). B, Diminished myeloid development upon Zbtb46 introduction in Flk1+ sorted cells. Representative flow plots and statistics show the percentage of the CD45 expression in 8-day differentiated mesoderm cells derived from Zbtb46-inducible ESC clone. Flk1+ mesoderm cells were separated on day 5 with MACS, transgene was induced between days 5 and 8
Zbtb46-modulated neurogenesis and endoderm differentiation
To further investigate the effects of this transcription factor during ESC differentiation, NPCs were generated. In this ex vivo differentiation system, the transcript levels of Neurog2 were strongly correlated with the neuronal development (Figure S2). In contrast, Pax6 expression was only slightly increased during NPC formation. Interestingly, these neuronal markers exhibited similar expression in differentiated ESC-derived neuronal progenitors with ectopic Zbtb46 (Figure S2). Together, the results presented above indicate that Zbtb46 only exerts a moderate effect on early neurogenesis.
Next, we assessed the gene expression signature of three prominent endoderm markers in ESC-derived EBs. Cell aggregates were generated from ESCs and the gene expression profiles of Sox7, Sox17, and Foxa1 were analyzed with RT-PCR (Figure S3). Sox7 showed a lower expression in the Zbtb46-primed 4- or 6-day EBs; in contrast, Foxa1 exhibited an elevated expression in the presence of Zbtb46. Sox17 was selectively upregulated by Zbtb46 in 4-days EBs. Overall, ectopic expression of Zbtb46 elicited a complex effect on the endoderm-specific gene expression profiles in ESC-derived EBs. Moreover, these findings also indicated that the outcome of Zbtb46 induction is not always inhibitory.
Zbtb46-instructed cells have an altered cell cycle profile
It is worth mentioning that, beside modulation of the ESC differentiation, cell number was also affected by this transcription factor. Decreased number of cells was harvested from the Zbtb46-primed 8- or 11-day differentiated cultures. Furthermore, reduced number of cells was observed during the early stage of differentiation (day 0-5) or even in nondifferentiated ESC cultures in case of forced expression of Zbtb46 (Figure 4A). These findings suggest that Zbtb46 negatively modulates the viability and/or the proliferation capacity of the ESCs and their differentiated forms. Unexpectedly, lower percent of propidium-iodide and Annexin V (AV) positive cells were detected from the Zbtb46-primed cells compared with control ESCs (Figure 4B). These data suggested that the viability or rate of the apoptotic cell formation is not the reason for the reduced cell number. Instead, an altered cell cycle profile was detected in the presence of Zbtb46: lower percent of G0/G1 cells was obtained, whereas the G2/M cell fraction was higher in the Zbtb46 instructed ESCs. This modified cell cycle profile can contribute for the impaired cell proliferation.
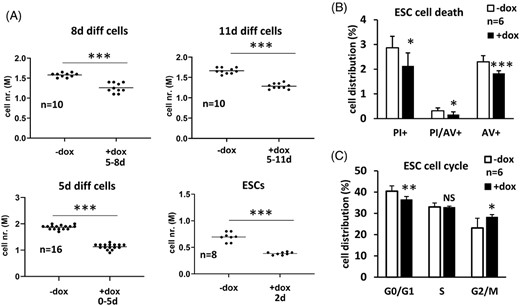
Impact of Zbtb46 on cell number, cell death, and cell cycle progression. A, Scatter plots show reduced cell numbers in different developmental stages upon introduction of Zbtb46. Numbers represent absolute cell counts per well in a six-well culture plate. Zbtb46 was induced for the indicated time period. B, Effect of Zbtb46 on cell death was assessed using costaining of AV and propidium iodide (PI) and quantified with flow cytometry. Transgene was induced for 72 hours using the Zbtb46-expressing C4 ESCs. Average and SD values were calculated from six independent experiments. C, Distribution of cell cycle compartments (G1/G0, S, and G2/M) was quantified with PI staining with permeabilized cells and measured on flow cytometer. Zbtb46 was induced for 72 hours testing the Zbtb46-inducible C4 ESCs. Average and SD values were calculated from six independent experiments
Reduced myeloid gene expression signature in Zbtb46-programmed cells
To define the transcriptional changes after Zbtb46 induction, we applied an ESC differentiation protocol to get relatively pure and homogeneous cell populations. Five-day differentiated, magnetically separated Flk1+ cells were cultivated without OP9 feeder cells with or without transgenic Zbtb46. To uncover the early impact of the transgene, cells were harvested after 24 hours; in addition, the transcriptomic profile was also determined after 3 days of differentiation (Figure S4A). We employed genome-scale gene expression analyses with RNA sequencing testing three biological replicates (together 15 samples were analyzed). We initially investigated Zbtb46 gene expression profile; as expected, highly elevated transcript levels were detected in the doxycycline treated triplicated samples (Figure S4B). Next, we evaluated the global gene expression signature with principle component analysis including all samples and RNA transcripts. This analysis revealed that the Flk1+ starting cell population exhibited a rather distinct gene expression signature compared with the 6- or 8-day differentiated cells (Figure S4C). Next, we preselected the expressing gene and ANOVA test was employed to detect those transcripts which were differently expressed amid the five conditions. Strikingly, 3030 changing entities were identified out of the 15 371 expressing genes, suggesting that the profile is very dynamic at this stage of the cell differentiation. Hierarchical cluster analysis of this gene set revealed a distinct gene population with elevated expression levels at the 8-day samples; importantly, most of these transcripts exhibited blunted expression in the presence of the transgenic Zbtb46. (Figure S4D). This finding is consistent with the broad repressive effect of this transcription factor which is readily visible after 3 days of doxycycline exposure. To further characterize those transcripts which were negatively regulated by Zbtb46, we implemented an additional transcript selection pipeline assessing only the effect of Zbtb46 induction in the 6- or 8-day differentiated cells. Altogether, 726 transcripts with reduced expression at day 6 and/or 8 in the Zbtb46-primed cells were identified. Our cluster analysis indicated that many of these entities showed a diminished expression at both time points (Figure 5A). Next, gene set enrichment analysis was carried out for identifying overrepresented gene ontology (GO) categories in this gene list. Remarkably, several immune cell-related GO categories were overrepresented (Table S3). For example, the “immune system process” category contains 148 transcripts, including Irf8, CD14, Itgal (CD11a), and Itgam (CD11b). All of these genes showed a lower expression, especially at day 8. This finding suggests that many myeloid cell-specific genes exhibit an impaired expression in the Zbtb46-programmed cells. We could validate the Irf8 downregulation at day 8 with quantitative PCR and immunoblot using independent sample sets (Figure 5B,C). In summary, our genome-scale transcript analysis provides evidence for the inhibitory role of Zbtb46 during ESC-derived myeloid blood cell differentiation.
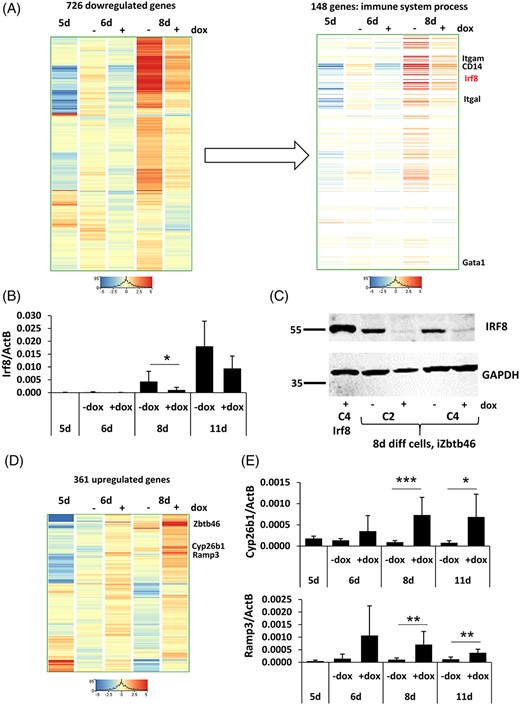
Zbtb46-dependent gene expression regulation. A, Hierarchical clustering of 726 transcripts with reduced expression at day 6 and/or 8 upon Zbtb46 induction. Within this gene list the “immune system process” category was found as a strongly overrepresented gene ontology category; this gene subset contains known regulators of myeloid development. B, Quantitative RT-PCR assay to measure the mRNA level of Irf8. C, IRF8 protein detection with Western blot testing 8-day differentiated, Zbtb46-inducible cell clones. The identity of specific bands was confirmed by comigration with a band seen in the extract of Irf8-induced ESCs (Irf8 C4 +dox). D, Hierarchical clustering of 361 transcripts with elevated expression at day 6 and/or 8 upon Zbtb46 induction. E, Transcript levels of Cyp26b1 and Ramp3 were validated with quantitative RT-PCR. For RT-PCR, 5 day differentiated Flk1+ cells were MACS sorted (5d) and cultured for 1 or 3 additional days (6d or 8d) in the presence of absence of doxycycline. In addition, nonsorted cells were differentiated for 11 days (11d) and the Zbtb46 induction was started at day 5. Average and SD values were calculated at least from six independent experiments
Next, we analyzed the transcripts upregulated by Zbtb46. Lower number of positively regulated genes (361) were identified using the same transcript selection method (Figure 5D). We identified and validated two transcripts (Cyp26b1 and Ramp3) which were consistently upregulated in the Zbtb46-primed cells suggesting that these genes can be directly regulated by this regulatory protein (Figure 5E).
Zbtb46 confers enhanced erythroid developmental potential on ESC-derived progenitors
In this study, we have demonstrated that Zbtb46 inhibits the ESC-derived myeloid blood cell differentiation. Of note, erythroid progenitors can also be emerged from ESCs and our transcriptomic analysis indicated that embryonic as well as adult hemoglobin genes showed an elevated expression in the 6- or 8-day differentiated cells compared with freshly isolated Flk1+ cells (Figure 6A). Moreover, increased expression of Hbb1 and Hbb2 was detected from 8-day differentiated Zbtb46-primed cells, suggesting that this factor might also modulate the red blood cell production. We extended this transcript analysis with RT-PCR and found that the major beta globin gene (Hbb-b1) was upregulated in the 8- and 11-day differentiated Zbtb46-primed cells. Similarly, this adult-type of hemoglobin exhibited a higher transcript level in the Zbtb46-instructed EBs and EB-derived progenitors (Figure 6B). In contrast, the expression of the fetal hemoglobin (Hbb-y) was unaltered in 8- and 11-day differentiated cells or in EBs. Although after EB formation and 3 days of differentiation, the Hbb-y was also upregulated in the presence of Zbtb46 (Figure S5). These results suggest that the adult beta globin gene showed an elevated expression in the Zbtb46-programmmed ESC-derived progenitors.
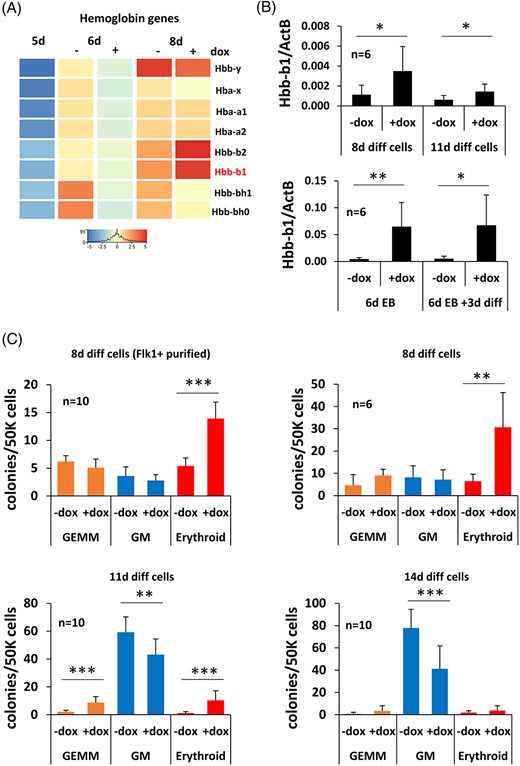
Zbtb46-induced erythropoiesis. A, RNA-seq data indicate elevated expression of the adult hemoglobin beta-genes (Hbb-b1 and b2) in the 8-day differentiated Zbtb46-primed cells. B, Quantitative RT-PCR assay to measure the mRNA level of Hbb-b1. Eight- and 11-day differentiated nonsorted cells were analyzed in the presence or absence of doxycycline, in these cases drug treatment (+dox) was started at day 5. In addition, embryoid bodies were grown for 6 days (6d EB); thereafter, they were disaggregated and further cultured for 3 days on OP9 cells (6d EB + 3d diff). For these experiments, the indicated samples (+dox) were treated with doxycycline from day 4. Average and SD values were calculated from six independent experiments. C, Increasing number of erythroid colonies were detected in the 8- or 11-day differentiated Zbtb46-instructed cells. To determine the hematopoietic colony (GEMM, granulocyte, erythrocyte, macrophage, and megakaryocyte; GM, granulocyte and macrophage) forming potential the 8 to 11- and 14-day differentiated cells were further cultured in MethoCult M3434 medium for 8 days. In case of the Flk-1+ purified, 8-day differentiated cells, the Flk1+ cells were MACS sorted at day 5 and cultured for 3 additional days in the presence of absence of doxycycline
Next, we investigated the hematopoietic colony forming capacity of the ex vivo generated cells: they were further cultured in Methocult M3434 semisolid medium for additional 8 days. Interestingly, a low number of definitive hematopoietic colonies were emerged regardless of the transgene induction of the 8-day differentiated cells (Figure 6C). In contrast, elevated number of erythroid colonies was detected in the presence of Zbtb46. Similarly, enhanced erythroid colony formation was obtained if the 11-day differentiated cells were analyzed. These cells also formed more mixed colonies (GEMM) after the ectopic expression of Zbtb46. On the other hand, the myeloid colony number (GM) was diminished in the Zbtb46-primed samples at day 11 and 14. Together, these results imply that forced expression of Zbtb46 shifts the blood cell specification from the myeloid to the erythroid pathway in murine ESC-derived blood cell progenitors.
Interestingly, the key regulators of erythroid development (Gata1, LDB1, KLF1, and LMO2) (24) were repressed or unaltered in Zbtb46-instructed cells (Figure 5A, and data not shown). Therefore, the elevated erythroid development cannot be explained by the enhanced expression of the erythroid lineage-specific transcriptional regulators. However, we observed that ex vivo differentiated ESCs exhibited an elevated percent of CD105+ population in the presence of Zbtb46 (Figure 7A,B). This subset of cells was distinct from the CD45+ population and oppositely modulated by Zbtb46. CD105 (Endoglin) is a marker of the adult erythroid progenitors14; thus, the enlarged CD105+ cell fraction might be associated with the enhanced erythroid development.
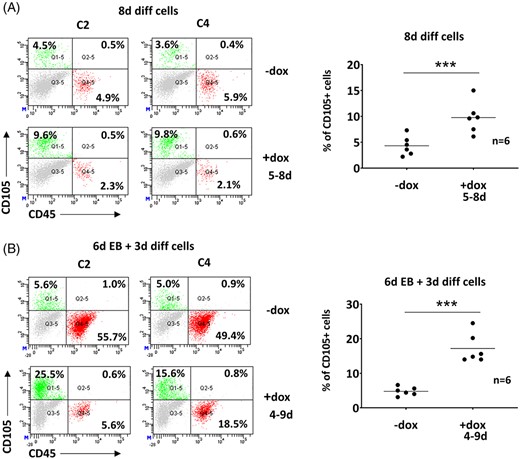
More CD105+ cells were detected in the Zbtb46-primed samples. A, Representative flow plots and statistics show the percentage of the CD105 single positive populations of 8-day differentiated, Zbtb46-inducible cell clones. B, Representative flow plots and statistics show the percentage of the CD105 single positive populations of 9-day cultured embryoid body derived (6d EB + 3d diff), Zbtb46-inducible cell clones. Cells were treated with doxycycline for the indicated time period
DISCUSSION
Herein, we provide support for the regulatory function of Zbtb46 on early blood cell specification. Zbtb46 is a recently described DC-specific transcription factor, which expression is restricted to DCs among the mature blood cell subsets. Despite the lineage-specific expression, Zbtb46 appears dispensable for DC development and its biological and transcriptional function is still incompletely characterized.2,7 Remarkably, this regulatory protein is also expressed in erythroid progenitors and endothelial cells implying a potential role during blood cell specification.2 Consistent with these data, we found that forced expression of Zbtb46 invoked a prominent effect during early hematopoietic development. ESC-derived Zbtb46-instructed mesodermal cells inefficiently converted into CD45+/CD11b+ progenitors suggesting that the myeloid blood cell development was repressed by this transcription factor. On the other hand, ectopic expression of Zbtb46 was associated with enhanced formation of erythroid blood cell colonies. Strikingly, Zbtb46-instructed cells expressed more adult-type beta-hemoglobin (Hbb-b1) suggesting that this factor specifically stimulates the adult-like red blood cell development. It was already documented that Zbtb proteins can regulate erythropoiesis. For example, Lrf deficiency causes lethal anemia in mouse embryo and this protein has a critical role on the repression of the fetal beta-globin gene.15,16
Notably, repressive functions of Zbtb46 have also been documented. For example, Zbtb46-deficient DCs seem to be more mature/activated suggesting that this factor somehow interferes with the maturation of these antigen presenting cells.7 More related to our results that ectopic expression of Zbtb46 in bone marrow-derived blood cell progenitors repressed the myeloid granulocyte commitment and skewed the cell specification toward cDC phenotype.2 In addition, a large number of monocyte affiliated genes were upregulated in Zbtb46 deficient DCs implying that this protein inhibits the myeloid/monocyte-specific gene regulatory networks.7 We observed a reduced myeloid blood cell generation; however, we failed to detect DC-like immune cell formation upon enforced expression of Zbtb46 in ESC-derived precursors. These findings suggest that Zbtb46 elicits a general inhibitory effect on the myeloid cell program but it can divert the developmental pathway to DC formation in adult blood cell progenitors. In contrast, Zbtb46 behaves differently in case of the less committed cells: it promotes the adult-like erythroid lineage specification in ESC-derived cells.
Our results argue that forced expression of Zbtb46 elicits an altered developmental program in ESC-derived progenitors. We proposed that multiple genes and pathways are likely to be affected by this transcription factor because of the complex phenotype. To examine the genomic impact of this transcription factor, we analyzed the Zbtb46-dependent upregulated and downregulated mRNA transcript profiles. More than 700 genes were repressed upon enforced expression of Zbtb46 in the 6- or 8-day differentiated cells. Among these repressed entities, several myeloid-specific transcripts were detected, including Irf8, CD14, and Itgam (CD11b). It is possible that some of these myeloid-specific genes are directly silenced by Zbtb46. Interestingly, it was already described that several myeloid/monocyte-specific gene regulatory regions can be occupied with Zbtb46.7 Moreover, it is well documented that multiple members of the Zbtb transcription factor family exert inhibitory effects during blood cell differentiation.17 Zbtb proteins contain one or more C-terminal zinc finger domains and an N-terminal BTB domain.18,19 The BTB domain can directly interact with various corepressor complexes, for example, with SMRT, NCOR, or BCOR, and thus mediates chromatin repression and gene silencing.20-23 It was proposed that Zbtb46 can also inhibit gene expression in cooperation with corepressor complexes.7 It is worth mentioning that besides myeloid suppression, cell proliferation was also negatively influenced by Zbtb46. This repressive effect was not restricted to myeloid progenitors but even undifferentiated ESCs exhibited lower cell number after Zbtb46 induction. It has been already described that ectopic expression of Zbtb46 negatively influences the endothelial cell proliferation via accumulating more cells in the G0/G1 phase of the cell division cycle.8 Interestingly, in case of Zbtb46-instructed ESCs, we observed an elevated percent of G2/M phase cells.
Despite the broad repressive effect, several transcripts were positively modulated by Zbtb46. For example, elevated expression of Cyp26b1 was detected with RNA-seq and this pattern was confirmed with RT-PCR. In addition, more CD105+ positive cells were noticed in the Zbtb46-programmed cells. CD105 (Endoglin) is important for endothelial development and vascularization24; moreover, erythroid progenitors also express CD105.14 The function of CD105 on erythropoiesis is complex,24 but it was described that the ESC-derived erythroid development is positively influenced by ectopic expression of CD105.25 Therefore, the Zbtb46-dependent enlarged CD105+ cell compartment can be connected with the accelerated erythropoiesis. However, further studies will be necessary to define the molecular mechanisms of the Zbtb46-regulated erythroid and myeloid development.
CONCLUSION
Together, our results illustrate how a single transcription factor can overwrite the blood cell development program in ex vivo differentiated ESCs. We found that ectopic expression of Zbtb46 in ESC-derived progenitors is sufficient to suppress the myeloid gene regulatory networks and activate the erythroid pathway. The very robust effect of Zbtb46 in ESC-derived progenitors suggests that it may be useful to probe other members of the Zbtb transcription factor family alone or in combination to facilitate directed differentiation of ESCs or for cell lineage conversion. Moreover, this work provides unique insights and a novel framework for studying the molecular mechanisms of the Zbtb46-dependent cell fate regulation. Finally, our genome-scale transcript analysis provides a catalogue for exploration of the Zbtb46 regulatory network in blood cell progenitors.
ACKNOWLEDGMENTS
The authors would like to acknowledge the excellent technical help of Iren Mezo. We thank Michael Kyba (University of Minnesota, Minnesota, USA) for providing the OP9 cells, the ZX1 mouse ES cell line, and the p2Lox targeting plasmid construct. This study was funded by Hungarian Scientific Research Funds (NKFIH K124890 and GINOP-2.3.2-15-2016-00044 PHARMPROT).
AUTHOR CONTRIBUTIONS
P.B.: conception and design, data collection, analysis and interpretation, manuscript writing; T.B.G., A.L.: data collection and analysis; B.S.: design, data collection and analysis; I.S.: conception and design, data analysis and interpretation, financial support, manuscript writing.
DATA AVAILABILITY STATEMENT
The data that support the findings of this study are available from the corresponding author upon reasonable request.
REFERENCES
SUPPORTING INFORMATION
Additional supporting information may be found online in the Supporting Information section at the end of this article.
How to cite this article:
Author notes
CONFLICT OF INTERESTS
The authors declared no potential conflicts of interest.
Funding information Hungarian Scientific Research Funds, Grant/Award Numbers: GINOP-2.3.2-15-2016-00044 PHARMPROT, NKFIH K124890