-
PDF
- Split View
-
Views
-
Cite
Cite
Xingyu Zhou, Yisi Liu, Yuebo Jin, Yifan Wang, Miao Miao, Jiali Chen, Yaobin Cheng, Yudong Liu, Jing He, Zhanguo Li, Immune responses after influenza vaccination in patients of primary Sjögren’s syndrome, Rheumatology, Volume 60, Issue 1, January 2021, Pages 224–230, https://doi.org/10.1093/rheumatology/keaa243
- Share Icon Share
Abstract
Influenza vaccination is effective in preventing infections in most people. This study aimed to assess the changes of immune responses in primary Sjögren’s Syndrome (pSS) patients after influenza vaccination and determine the safety of influenza vaccination.
A total of 17 patients with pSS and 16 healthy controls (HCs) were included. Peripheral mononuclear cells were analysed by flow cytometry. Vaccine-specific antibodies were determined by ELISA. Clinical features and serological responses were monitored.
The percentages of T follicular helper cell (Tfh) were significantly elevated in HCs after vaccination (P=0.0005), while no significant differences in the levels of Tfh in pSS patients were identified (P=0.1748). The proportions of Th2 cells were significantly decreased after vaccination in both pSS patients and HCs (P<0.05). In contrast, the percentages of Th1 cells and Th17 cells were significantly increased after vaccination in pSS patients (P<0.05), while no significant differences in the percentages of Th1 and Th17 cells were identified in HCs (P>0.05), although a trend towards higher levels of Th1 cells was observed (P=0.0830). No significant changes in the proportions of memory B cells and plasmablasts were observed after vaccination. Patients with pSS developed higher levels of vaccine-specific IgGs compared with HCs (P=0.001). No significant changes in disease manifestations and laboratory parameters were observed after vaccination. No increased vaccination related adverse effect was observed in pSS.
Our findings suggest the feasibility of applying influenza vaccines to patients with pSS, raising awareness for vaccination among the rheumatology community and involved healthcare professionals.
Influenza vaccination does not aggravate abnormal immune responses in patients with pSS.
Patients with pSS developed higher levels of vaccine-specific IgG titers.
It is well tolerated for pSS patients to receive influenza vaccination.
Introduction
Primary Sjögren’s syndrome (pSS) is a progressive systemic autoimmune disease primarily affecting females, which is characterized by immunological destruction of exocrine glands, primarily the salivary and lacrimal glands [1]. Besides the characteristic glandular symptoms, extra-glandular manifestations, such as non-erosive polyarthritis, vasculitis or myositis may also occur during the disease course. Autoreactive B and T cells contribute to the disease pathogenesis. In pSS patients, the incidence of lower respiratory infections is higher than the general population [2, 3]. Specifically, compared with the general population, patients with autoimmune diseases, such as pSS, display significantly increased risk of influenza infection [4], which may be due to their impaired host defences, as well as the use of immunosuppressive therapy, including biological agents, Disease modifying antirheumatic drugs (DMARDs) and glucocorticoids [5–7].
Influenza vaccination has been shown to protect high-risk patients against infections and greatly reduce hospital admission rates and mortality rates. In autoimmune disorders, such as SLE and myasthenia gravis, the influenza vaccine is safe and effective for patients receiving standard administration [8, 9]. As such, influenza vaccination is recommended for people with autoimmune diseases [4]. Interestingly, a previous study demonstrated that immunosuppressive therapies attenuated vaccine response; thus, vaccines should be administrated before the initiation of immunosuppression [10]. In contrast, another study has shown increased autoantibodies levels in untreated pSS patients upon the influenza vaccine; thus, influenza vaccination is not recommended for untreated patients [11]. Since the efficacy, immunogenicity, and safety of vaccination for patients with autoimmune diseases remain unclear, these populations usually suffer from a suboptimal uptake of vaccinations, in part due to a low rate of recommendation by rheumatologists in China. Given the beneficial effect of influenza vaccination in the general population, it is of paramount importance to address the efficacy and safety, as well as the changes of immune responses upon vaccinations in an autoimmune-biased immune system, such as pSS.
Dysregulated adaptive immune responses are implicated in the pathogenesis of pSS. The characteristic immunological features of pSS, including antibody production and germinal centre formation, are modulated by hyperactive B cells and T follicular helper (Tfh) cells [12–18]. In addition, the imbalance between Th1 and Th2 cells, and the increased levels of Th17 cells are also involved in the pathogenesis of pSS [19, 20]. In this study, we evaluated the safety and efficacy of influenza vaccination in pSS patients and assessed the immune responses of these patients upon vaccination by analysing the changes of lymphocyte subsets.
Methods
Patients and healthy controls
A total of 17 pSS patients (16 female, 1 male) were recruited in the Department of Rheumatology and Immunology, Peking University People’s Hospital. All patients fulfilled the 2002 American-European Consensus Group Criteria for pSS [21]. pSS patients with other rheumatic diseases were excluded. Disease activity was evaluated by European League Against Rheumatism Sjögren’s Syndrome Patient Reported Index (ESSPRI) and European League Against Rheumatism Sjögren’s Syndrome Disease Activity Index (ESSDAI) [22]. Sixteen age and sex-matched healthy controls (15 female, 1 male) were included. Study participants were followed after receiving influenza vaccination. Blood samples were collected immediately before vaccination and one week after vaccination and tested within 8 h after isolation. The study was approved by the ethical committee of Peking University People’s Hospital (approval number 2017PHB167-01) and all participants provided written informed consent before study inclusion.
Vaccine and antibody detection
The vaccine used in this study was China’s domestic quadrivalent influenza vaccine, which was an attenuated live vaccine containing A/Michigan/45/2015 (H1N1) pdm09-like virus, A/Singapore/INFIMH-16–0019/2016 (H3N2)-like virus, B/Colorado/06/2017–like virus (Victoria lineage) and B/Phuket/3073/2013–like virus (Yamagata lineage). All the patients and healthy controls (HCs) received a single dose of the influenza vaccine intramuscularly. ELISA was used to determine the vaccine-specific immunoglobulin G antibodies.
Flow cytometry analysis
Peripheral blood mononuclear cells (PBMCs) were isolated by Ficoll-Hypaque density gradient centrifugation. PBMCs were incubated with fluorophore-conjugated monoclonal antibodies listed in Supplementary Table S1, available at Rheumatology online. Relative proportions of Treg, Tfh, Th1, Th2 and Th17 cell subsets were analysed by flow cytometry using FACSAria III (BD) and FlowJo software (Tree Star). The phenotype of T and B-cell subsets were listed in Supplementary Table S2 available at Rheumatology online. Gating strategies and phenotypic characterization of lymphocyte subpopulations are shown in Supplementary Fig. S1, available at Rheumatology online and Supplementary Table S2, available at Rheumatology online, respectively, as previously described [23].
Clinical indicators
Serological tests, including tests of ANA, anti-Ro/SSA antibody (anti-SSA), anti-La/SSB antibody (anti-SSB), anti-α-fodrin antibody, RF, complement component 3 (C3), C4, IgA, IgG, IgM and γ-globulin were performed in all patients. All clinical indicators were performed at the Clinical laboratory at Peking University People’s Hospital.
Statistical analysis
SPSS 20.0 (SPSS Inc., Chicago, IL, USA) and Prism 5 (GraphPad Software, San Diego, CA, USA) were used to analyse the data. Numeric data were described as mean (s.d.), categorical variables were presented as percentage frequencies. Mann–Whitney U test or Wilcoxon matched-pairs signed-rank test were used to compared continuous variables. χ2 analysis was used to compared categorical variables. P-values of <0.05 were considered statistically significant.
Result
Patients’ baseline characteristics
Among the 17 pSS patients, the female gender was predominant (94.12%), with a mean age of 49.23 ± 14.37 years. The prevalence of ANA, anti-SSA, anti-SSB and Ro-52 were 94.12%, 94.12%, 70.59% and 94.21%, respectively. No statistical differences were observed in the mean age and sex ratio between patients and healthy controls (Table 1).
. | pSS (n = 17) . | HC(n = 16) . | P-value . |
---|---|---|---|
Age, mean (s.d.) | 49.23 (14.37) | 44.38 (8.27) | 0.531 |
Female, n (%) | 16 (94.12) | 15 (93.75) | 0.965 |
Autoantibodies, n (%) | |||
ANA positive | 16 (94.12) | – | – |
Anti-SSA positive | 16 (94.12) | – | – |
Anti-SSB positive | 12 (70.59) | – | – |
Anti-Ro-52 positive | 16 (94.12) | – | – |
. | pSS (n = 17) . | HC(n = 16) . | P-value . |
---|---|---|---|
Age, mean (s.d.) | 49.23 (14.37) | 44.38 (8.27) | 0.531 |
Female, n (%) | 16 (94.12) | 15 (93.75) | 0.965 |
Autoantibodies, n (%) | |||
ANA positive | 16 (94.12) | – | – |
Anti-SSA positive | 16 (94.12) | – | – |
Anti-SSB positive | 12 (70.59) | – | – |
Anti-Ro-52 positive | 16 (94.12) | – | – |
Data are expressed as n (%) or mean (s.d.). ANA: anti-nuclear antibodies; anti-SSA: anti-Ro/SSA antibodies; anti-SSB: anti- La/SSB antibodies; anti-Ro-52: anti-Ro-52 antibodies.
. | pSS (n = 17) . | HC(n = 16) . | P-value . |
---|---|---|---|
Age, mean (s.d.) | 49.23 (14.37) | 44.38 (8.27) | 0.531 |
Female, n (%) | 16 (94.12) | 15 (93.75) | 0.965 |
Autoantibodies, n (%) | |||
ANA positive | 16 (94.12) | – | – |
Anti-SSA positive | 16 (94.12) | – | – |
Anti-SSB positive | 12 (70.59) | – | – |
Anti-Ro-52 positive | 16 (94.12) | – | – |
. | pSS (n = 17) . | HC(n = 16) . | P-value . |
---|---|---|---|
Age, mean (s.d.) | 49.23 (14.37) | 44.38 (8.27) | 0.531 |
Female, n (%) | 16 (94.12) | 15 (93.75) | 0.965 |
Autoantibodies, n (%) | |||
ANA positive | 16 (94.12) | – | – |
Anti-SSA positive | 16 (94.12) | – | – |
Anti-SSB positive | 12 (70.59) | – | – |
Anti-Ro-52 positive | 16 (94.12) | – | – |
Data are expressed as n (%) or mean (s.d.). ANA: anti-nuclear antibodies; anti-SSA: anti-Ro/SSA antibodies; anti-SSB: anti- La/SSB antibodies; anti-Ro-52: anti-Ro-52 antibodies.
Comparison of the proportion of CD4+ T-cell subsets in the peripheral blood before and after influenza vaccination
The proportions of Tfh, Th1, Th2 and Th17 cells, as well as subsets of Tfh cells, were assessed by flow cytometry (Fig. 1, Supplementary Fig. S1A, available at Rheumatology online). Before vaccination, the proportions of Tfh were significantly higher in patients with pSS than in HCs (P=0.0219) (Supplementary Fig. S2, available at Rheumatology online). After vaccination, the proportions of Tfh cells were significantly elevated in HCs (P=0.0005) (Fig. 1D). A trend towards higher percentages of circulating Tfh cells was also observed in patients with pSS, although it did not reach statistical significance (P=0.1748). In patients with pSS, the proportions and absolute numbers of CXCR3+CCR6-Tfh1 cells did not change significantly, whereas the proportions of CXCR3-CCR6-Tfh2 cells were significantly decreased (P=0.0024), although no significant changes in absolute numbers were observed (P=0.1677) (Fig. 1E, Supplementary Fig. S3, available at Rheumatology online). In contrast, both absolute numbers (P=0.0034) and percentages (P=0.0005) of CXCR3-CCR6+Tfh17 cells were significantly increased after vaccination (Fig. 1E, Supplementary Fig. S3, available at Rheumatology online, Supplementary Table S3, available at Rheumatology online).
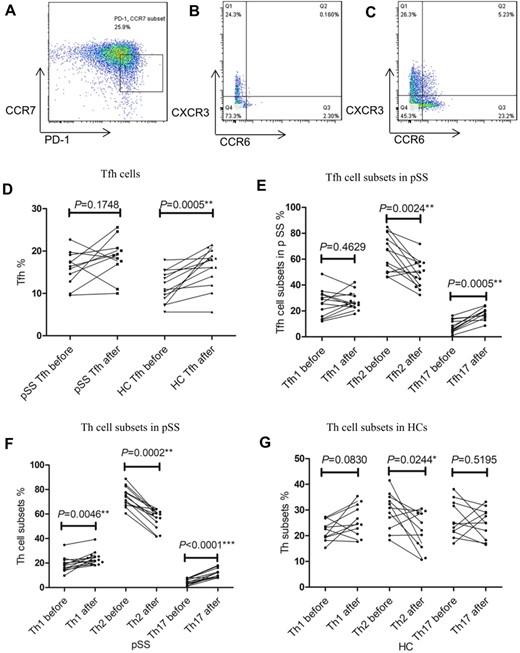
Changes in the proportion of CD4+ T-cell subsets before and after influenza vaccination
PBMCs were collected from pSS patients before and 1 week after influenza vaccination. The proportion of CD4+ T-cell subsets were calculated by flow cytometry. Tfh cells were determined as CD3+CD4+CD127+CD25-CD45RA-CXCR5+CCR7lowPD-1+ cells. Tfh1, Tfh2 and Tfh17 cells were identified as CXCR6-CXCR3+ (Tfh1), CXCR6-CXCR3- (Tfh2) and CXCR6+CXCR3- (Tfh17) within CD3+CD4+CD127+CD25-CD45RA-CXCR5+ group. Th1, Th2 and Th17 cells were identified as CXCR6-CXCR3+ (Th1), CXCR6-CXCR3- (Th2) and CXCR6+CXCR3- (Th17) within CD3+CD4+CD127+CD25- group. Representative flow cytometry analysis of CCR7lowPD-1+ Tfh cells (A), Tfh cells subsets (Tfh1, Tfh2, and Tfh17 cells) (B) and Th cells subsets (Th1, Th2, and Th17 cells) (C) respectively. (D) Changes of Tfh cell proportions in pSS patients and HCs before and after influenza vaccination. (E) Changes in proportions of Tfh subsets in pSS patients and HCs before and after influenza vaccination. (F) Changes in proportions of Th cell subsets in pSS patients before and after influenza vaccination. (G) Changes of proportions of Th cell subsets in HCs before and after influenza vaccination. The statistical analysis was determined by Wilcoxon matched-pairs signed-rank test. *P<0.05; **P<0.005; ***P<0.0001. Differences with P<0.05 were considered significant. Tfh: follicular helper T cell; Th: helper T cell; before: before influenza vaccination; after: 1 week after influenza vaccination; PD-1: programmed cell death 1; CCR7: C-C chemokine receptor type 7; CCR6: C-C chemokine receptor type 6; CXCR3: C-X-C chemokine receptor type 3.
Regarding the CD4+ T-cell subsets, proportions of Th2 cells were significantly reduced (P<0.05), while proportions of Th1 cells were elevated in both pSS patients and HCs after vaccination (Fig. 1F and G). Interestingly, the percentages of Th17 cells remained unchanged in HCs (P=0.5195), but were significantly increased in pSS patients (P<0.0001) (Fig. 1F and G).
The changes in the proportions of CD27highCD38high plasmablasts and CD24highCD27high memory B cells after vaccination and levels of vaccine-specific antibodies
The proportions of B-cell subsets were also assessed by flow cytometry (Fig. 2, Supplementary Fig. S1B, available at Rheumatology online). No significant differences in the proportions or absolute number of CD27highCD38high plasmablasts and CD24highCD27high memory B cells were observed in both pSS patients and HCs after vaccination (Fig. 2C and D, Supplementary Fig. S4, available at Rheumatology online).
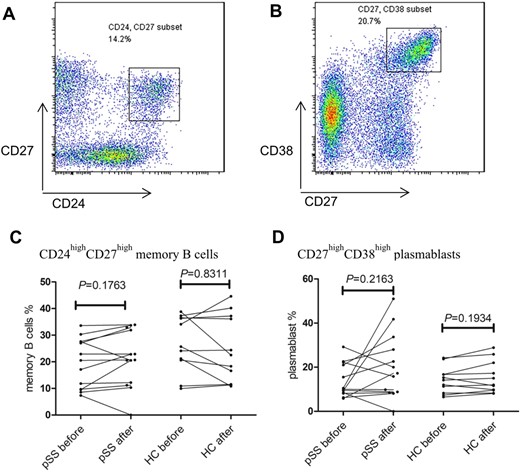
Changes of the CD24highCD27high memory B cells and CD27 high CD38high plasmablasts in pSS patients and HCs
PBMCs were collected from pSS patients before and 1 week after influenza vaccination. The proportion of CD24highCD27high memory B cells and CD27high CD38high plasmablasts subsets were calculated by flow cytometry. Representative flow cytometry analysis of CD24highCD27high memory B cells (A) and CD27highCD38high plasmablasts (B) subsets in CD19+CD3- B cells. (C) Change of proportion of CD19+CD3-CD24highCD27high memory B cells before and after influenza vaccination. (D) Change of proportion of CD19+CD3-CD27highCD38high plasmablasts before and after influenza vaccination. The statistical analysis was determined by Wilcoxon matched-pairs signed-rank test. *P<0.05; **P<0.005; ***P<0.0001. Differences with P<0.05 were considered significant.
The levels of circulating vaccine-specific IgG antibodies before and after vaccination were tested by ELISA. Importantly, the OD values of anti-H1N1 IgG antibodies were significantly enhanced in pSS patients 1 week after vaccination (P=0.001), while the OD values of IgG antibodies in serum of HCs did not change significantly (Table 2).
Groups . | OD before . | OD after 1 week . | t . | P . |
---|---|---|---|---|
pSS (n = 17) | 0.042 (0.015) | 0.082 (0.036) | −3.765 | 0.001* |
HC (n = 16) | 0.067 (0.020) | 0.058 (0.011) | 1.163 | 0.260 |
Groups . | OD before . | OD after 1 week . | t . | P . |
---|---|---|---|---|
pSS (n = 17) | 0.042 (0.015) | 0.082 (0.036) | −3.765 | 0.001* |
HC (n = 16) | 0.067 (0.020) | 0.058 (0.011) | 1.163 | 0.260 |
Data were described as mean (s.d.), The statistical difference was determined by paired sample t test. Difference with *P<0.05 was considered significant. OD: optical density.
Groups . | OD before . | OD after 1 week . | t . | P . |
---|---|---|---|---|
pSS (n = 17) | 0.042 (0.015) | 0.082 (0.036) | −3.765 | 0.001* |
HC (n = 16) | 0.067 (0.020) | 0.058 (0.011) | 1.163 | 0.260 |
Groups . | OD before . | OD after 1 week . | t . | P . |
---|---|---|---|---|
pSS (n = 17) | 0.042 (0.015) | 0.082 (0.036) | −3.765 | 0.001* |
HC (n = 16) | 0.067 (0.020) | 0.058 (0.011) | 1.163 | 0.260 |
Data were described as mean (s.d.), The statistical difference was determined by paired sample t test. Difference with *P<0.05 was considered significant. OD: optical density.
Clinical symptoms and laboratory parameters after vaccination
No severe adverse effects related to vaccination were observed in both pSS patients and HCs. Only one pSS patient displayed mild adverse effects (fever) after vaccination. We also monitored clinical signs of disease activity and laboratory parameters after vaccination. No significant changes in disease activity scores, including ESSDAI and ESSPRI, were observed 3 months after vaccination in pSS patients (Supplementary Tables S4 and S5 available at Rheumatology online). In addition, no significant differences in laboratory parameters were observed one month after vaccination (Table 3, Supplementary Table S6 available at Rheumatology online).
Clinical indexes . | Before . | After 7 days . | After 1 months . | P . |
---|---|---|---|---|
IgG (g/l) | 17.02 (3.87) | 16.62 (3.87) | 17.10 (4.54) | 0.771 |
IgM(g/l) | 1.10 (0.56) | 1.09 (0.55) | 1.11 (0.51) | 0.689 |
IgA(g/l) | 3.11 (1.25) | 3.00 (1.15) | 2.98 (1.00) | 0.981 |
C3 | 0.81 (0.16) | 0.92 (0.44) | 0.89 (0.36) | 0.926 |
C4 | 0.19 (0.60) | 0.20 (0.94) | 0.19 (0.41) | 0.584 |
α1, % | 3.50 (0.52) | 3.80 (0.55) | 3.68 (0.54) | 0.383 |
α2, % | 7.90 (0.89) | 8.20 (0.86) | 8.11 (0.80) | 0.368 |
β1, % | 5.52(0.56) | 5.70 (0.49) | 5.65 (0.57) | 0.135 |
β2, % | 4.65 (0.57) | 4.72 (0.81) | 4.70 (0.77) | 0.832 |
γ-G, % | 22.11 (4.22) | 21.20 (4.23) | 21.70 (4.49) | 0.584 |
WBC (×109/l) | 4.28 (0.78) | 4.93 (0.98)a | 4.22 (0.51)b | 0.012* |
N% (%) | 56.18 (10.89) | 61.31 (12.45) | 58.48 (7.34) | 0.926 |
PLT (×109/l) | 224.62 (58.51) | 234.23 (53.58) | 230.85 (55.85) | 0.092 |
HGB (g/l) | 126.77 (8.80) | 124.23 (9.54) | 125.38 (8.16) | 0.383 |
Clinical indexes . | Before . | After 7 days . | After 1 months . | P . |
---|---|---|---|---|
IgG (g/l) | 17.02 (3.87) | 16.62 (3.87) | 17.10 (4.54) | 0.771 |
IgM(g/l) | 1.10 (0.56) | 1.09 (0.55) | 1.11 (0.51) | 0.689 |
IgA(g/l) | 3.11 (1.25) | 3.00 (1.15) | 2.98 (1.00) | 0.981 |
C3 | 0.81 (0.16) | 0.92 (0.44) | 0.89 (0.36) | 0.926 |
C4 | 0.19 (0.60) | 0.20 (0.94) | 0.19 (0.41) | 0.584 |
α1, % | 3.50 (0.52) | 3.80 (0.55) | 3.68 (0.54) | 0.383 |
α2, % | 7.90 (0.89) | 8.20 (0.86) | 8.11 (0.80) | 0.368 |
β1, % | 5.52(0.56) | 5.70 (0.49) | 5.65 (0.57) | 0.135 |
β2, % | 4.65 (0.57) | 4.72 (0.81) | 4.70 (0.77) | 0.832 |
γ-G, % | 22.11 (4.22) | 21.20 (4.23) | 21.70 (4.49) | 0.584 |
WBC (×109/l) | 4.28 (0.78) | 4.93 (0.98)a | 4.22 (0.51)b | 0.012* |
N% (%) | 56.18 (10.89) | 61.31 (12.45) | 58.48 (7.34) | 0.926 |
PLT (×109/l) | 224.62 (58.51) | 234.23 (53.58) | 230.85 (55.85) | 0.092 |
HGB (g/l) | 126.77 (8.80) | 124.23 (9.54) | 125.38 (8.16) | 0.383 |
Data were described as mean (s.d.). The statistical difference was determined by non-parametric paired t test. *P<0.05. Difference with P<0.05 was considered significant.
Denotes the significant different between Before vs After 7 days and after 1 months.
Denotes the significant different between After 7 days vs After 1 months. IgG, IgM, IgA: serum immunoglobulin G, M and A; C3, C4: serum complement 3 and 4; α1, α2, β1, β2, γ-G: serum macroglobulin α1, α2, β1, β2 and γ-G.
HGB: haemoglobin; N: neutrophilic granulocyte; PLT: platelets; WBC: white blood cells.
Clinical indexes . | Before . | After 7 days . | After 1 months . | P . |
---|---|---|---|---|
IgG (g/l) | 17.02 (3.87) | 16.62 (3.87) | 17.10 (4.54) | 0.771 |
IgM(g/l) | 1.10 (0.56) | 1.09 (0.55) | 1.11 (0.51) | 0.689 |
IgA(g/l) | 3.11 (1.25) | 3.00 (1.15) | 2.98 (1.00) | 0.981 |
C3 | 0.81 (0.16) | 0.92 (0.44) | 0.89 (0.36) | 0.926 |
C4 | 0.19 (0.60) | 0.20 (0.94) | 0.19 (0.41) | 0.584 |
α1, % | 3.50 (0.52) | 3.80 (0.55) | 3.68 (0.54) | 0.383 |
α2, % | 7.90 (0.89) | 8.20 (0.86) | 8.11 (0.80) | 0.368 |
β1, % | 5.52(0.56) | 5.70 (0.49) | 5.65 (0.57) | 0.135 |
β2, % | 4.65 (0.57) | 4.72 (0.81) | 4.70 (0.77) | 0.832 |
γ-G, % | 22.11 (4.22) | 21.20 (4.23) | 21.70 (4.49) | 0.584 |
WBC (×109/l) | 4.28 (0.78) | 4.93 (0.98)a | 4.22 (0.51)b | 0.012* |
N% (%) | 56.18 (10.89) | 61.31 (12.45) | 58.48 (7.34) | 0.926 |
PLT (×109/l) | 224.62 (58.51) | 234.23 (53.58) | 230.85 (55.85) | 0.092 |
HGB (g/l) | 126.77 (8.80) | 124.23 (9.54) | 125.38 (8.16) | 0.383 |
Clinical indexes . | Before . | After 7 days . | After 1 months . | P . |
---|---|---|---|---|
IgG (g/l) | 17.02 (3.87) | 16.62 (3.87) | 17.10 (4.54) | 0.771 |
IgM(g/l) | 1.10 (0.56) | 1.09 (0.55) | 1.11 (0.51) | 0.689 |
IgA(g/l) | 3.11 (1.25) | 3.00 (1.15) | 2.98 (1.00) | 0.981 |
C3 | 0.81 (0.16) | 0.92 (0.44) | 0.89 (0.36) | 0.926 |
C4 | 0.19 (0.60) | 0.20 (0.94) | 0.19 (0.41) | 0.584 |
α1, % | 3.50 (0.52) | 3.80 (0.55) | 3.68 (0.54) | 0.383 |
α2, % | 7.90 (0.89) | 8.20 (0.86) | 8.11 (0.80) | 0.368 |
β1, % | 5.52(0.56) | 5.70 (0.49) | 5.65 (0.57) | 0.135 |
β2, % | 4.65 (0.57) | 4.72 (0.81) | 4.70 (0.77) | 0.832 |
γ-G, % | 22.11 (4.22) | 21.20 (4.23) | 21.70 (4.49) | 0.584 |
WBC (×109/l) | 4.28 (0.78) | 4.93 (0.98)a | 4.22 (0.51)b | 0.012* |
N% (%) | 56.18 (10.89) | 61.31 (12.45) | 58.48 (7.34) | 0.926 |
PLT (×109/l) | 224.62 (58.51) | 234.23 (53.58) | 230.85 (55.85) | 0.092 |
HGB (g/l) | 126.77 (8.80) | 124.23 (9.54) | 125.38 (8.16) | 0.383 |
Data were described as mean (s.d.). The statistical difference was determined by non-parametric paired t test. *P<0.05. Difference with P<0.05 was considered significant.
Denotes the significant different between Before vs After 7 days and after 1 months.
Denotes the significant different between After 7 days vs After 1 months. IgG, IgM, IgA: serum immunoglobulin G, M and A; C3, C4: serum complement 3 and 4; α1, α2, β1, β2, γ-G: serum macroglobulin α1, α2, β1, β2 and γ-G.
HGB: haemoglobin; N: neutrophilic granulocyte; PLT: platelets; WBC: white blood cells.
Discussion
Findings on the safety and efficacy of vaccines in the context of autoimmune diseases are critical in providing evidence to the clinical practice. In this prospective study on the influenza vaccination in a cohort of Chinese patients with pSS, we found that pSS patients developed higher levels of vaccine-specific IgG antibodies than HCs. Importantly, these robust immune responses to vaccination are not accompanied by an increase in disease-specific serological immune responses. More importantly, we show that vaccination against influenza does not aggravate disease severity or leads to any significant adverse effects. Our findings suggest the feasibility of applying influenza vaccines to patients with pSS, shedding light on the use of influenza vaccines in pSS patients in China.
The primary goal of this study was to investigate the safety and efficacy of influenza vaccines in Chinese patients with pSS. We did not find any injection-site reactions, such as muscle ache, local inflammation and tissue necrosis in pSS group and HC upon vaccination. In addition, we did not identify systemic adverse reactions, such as fever and pneumonia. We followed the pSS patients up to 3 months and did not find any significant increases in clinical or laboratory parameters of the disease activity. More importantly, we found that pSS patients generated higher levels of vaccine-specific IgG antibodies than matched controls, which was consistent with the results from Brauner et al. [11]
We also delineated the post-vaccination immune responses in pSS patients. Tfh cells, which are essential for the germinal center formation, autoantibody production, and B-cell activation, are implicated in the pathogenesis of pSS [24]. Consistent with the previous study [12, 14, 15, 25, 26], we found that the levels of circulating Tfh cells were significantly higher compared with those in HCs before vaccination. However, after vaccination, significantly elevated levels of Tfh cells were only observed in HCs, but not in pSS patients. Interestingly, although the levels of Tfh cells remained unchanged, we did observe significant changes in Tfh subsets after vaccination. It has been shown that Tfh17 cells can be induced by live attenuated vaccines and mucosal vaccines [27, 28]. Interestingly, Tfh2 and Tfh17 cells, but not Tfh1 cells, have been shown to efficiently induce in vitro naïve B-cell proliferation and differentiation into plasmablasts and production of IgGs [23]. Given that vaccination did not aggravate the disease severity and autoantibodies levels but promoted the production of antibodies to influenza, and vaccination led to elevated levels of Tfh17 cells and decreased levels of Tfh2 cells, we hypothesize that vaccination may skew the Tfh2 dominant autoimmune responses to vaccination-induced Tfh17 responses. Further studies on the roles of vaccine-induced Tfh2 and Tfh17 responses in the context of autoimmune diseases are needed.
In this study, we found that after influenza vaccination, pSS patients and HCs displayed similar patterns of immune responses in terms of Th subsets and B cells. Although we observed a significant increase of Th17 cells in patients with pSS, the overall disease activity was not changed. It has been suggested that vaccines can induce stable, long-term Th17 memory responses [29]. It is likely that the increased levels of Th17 cells are induced by vaccination and are not pathogenic in pSS. Further studies are needed to elucidate the function of these Th17 cells in the context of post-vaccination.
It should be noted that the main limitation of this study is a small number of patients, further investigations should be extended to different cohorts with larger sample size and longer observation period to better understand the immune response of vaccinated pSS patients, as well as the long-term effect on disease severity.
Conclusion
The influenza vaccination is well tolerant for patients with pSS. The influenza vaccination will not lead to aggravation of the primary disease. Therefore, influenza vaccination should be recommended for patients with pSS. In light of the low uptake of vaccination among the autoimmune population in China, our findings present evidence supporting the use of vaccines in patients with pSS.
Acknowledgements
The authors are grateful to professor Jing He, Yisi Liu, Yuebo Jin, Miao Miao, Jiali Chen, Yaobin Cheng and Yudong Liu. All authors were involved in drafting the article or revising it critically for important intellectual content, and all authors approved the final version to be published.
Funding: This work was supported by national natural science Foundation of China (31870879 and 81701607), Beijing sci-Tech grant (Z191100006619114), Clinical Medicine Plus X-Young scholars Project of Peking University (PKU2019lCXQ013) supported by the Fundamental Research Funds for the Central Universities.
Disclosure statement: The authors have declared no conflicts of interest.
Supplementary data
Supplementary data are available at Rheumatology online.
References
Author notes
.
Comments