-
PDF
- Split View
-
Views
-
Cite
Cite
Soyoung Jung, Hiroshi A Maeda, Debottlenecking the L-DOPA 4,5-dioxygenase step with enhanced tyrosine supply boosts betalain production in Nicotiana benthamiana, Plant Physiology, Volume 195, Issue 3, July 2024, Pages 2456–2471, https://doi.org/10.1093/plphys/kiae166
- Share Icon Share
Abstract
Synthetic biology provides emerging tools to produce valuable compounds in plant hosts as sustainable chemical production platforms. However, little is known about how supply and utilization of precursors is coordinated at the interface of plant primary and specialized metabolism, limiting our ability to efficiently produce high levels of target specialized metabolites in plants. L-Tyrosine is an aromatic amino acid precursor of diverse plant natural products including betalain pigments, which are used as the major natural food red colorants and more recently a visual marker for plant transformation. Here, we studied the impact of enhanced L-tyrosine supply on the production of betalain pigments by expressing arogenate dehydrogenase (TyrA) from table beet (Beta vulgaris, BvTyrAα), which has relaxed feedback inhibition by L-tyrosine. Unexpectedly, betalain levels were reduced when BvTyrAα was coexpressed with the betalain pathway genes in Nicotiana benthamiana leaves; L-tyrosine and 3,4-dihydroxy-L-phenylalanine (L-DOPA) levels were drastically elevated but not efficiently converted to betalains. An additional expression of L-DOPA 4,5-dioxygenase (DODA), but not CYP76AD1 or cyclo-DOPA 5-O-glucosyltransferase, together with BvTyrAα and the betalain pathway, drastically enhanced betalain production, indicating that DODA is a major rate-limiting step of betalain biosynthesis in this system. Learning from this initial test and further debottlenecking the DODA step maximized betalain yield to an equivalent or higher level than that in table beet. Our data suggest that balancing between enhanced supply (“push”) and effective utilization (“pull”) of precursor by alleviating a bottleneck step is critical in successful plant synthetic biology to produce high levels of target compounds.
Introduction
Plants produce over one million plant natural products (Afendi et al. 2012; Shi et al. 2024), which play important roles in plant growth, development, and adaptation to surrounding environments (Fang et al. 2019; Erb and Kliebenstein 2020; Zhao and Rhee 2022). Additionally, plant natural products are critical in human health as many of them have pharmaceutical and nutritional properties. Roughly 65% of small molecule drugs approved for cancer treatments are derived from natural products, mainly from plants (Newman and Cragg 2020). Many of these plant-derived drugs are chemically synthesized using fossil fuels since those compounds are produced in small quantities in native plants, some of which are not easy to access geographically. However, plant natural products often have complex structures with multiple chiral centers, not suitable for being chemically synthesized. Recent advances in synthetic biology, an approach to reengineer biological systems through iterative “design-build-test-learn (DBTL)” cycles (Nielsen and Keasling 2016; Pouvreau et al. 2018), have accelerated metabolic engineering processes, providing alternative bio-based production methods for plant natural products. Thus far, microbial systems, such as Escherichia coli and yeast (Saccharomyces cerevisiae), have been used as a favored host for production of plant natural products (Ro et al. 2006; Pyne et al. 2019; Jamil et al. 2022) due to their rapid generation cycle, ease of genetic modification, and well-established large-scale fermentation systems (Cravens et al. 2019).
Microbial hosts, however, are not always suitable to produce plant natural products, as many of these chemicals have evolved to exert antimicrobial activities (Simões et al. 2009; Monte et al. 2014). Also, phytochemicals are often synthesized through a complex biosynthetic pathway spanning across different subcellular compartments, and some plant enzymes (e.g. cytochrome P450 enzymes) are difficult to express in unicellular microbial systems (Zurbriggen et al. 2012; Barnum et al. 2021). While efforts and progress have been made to overcome some of these issues in producing plant natural products in microbial hosts (Montaño López et al. 2022; Zhang et al. 2022), plants are naturally tailored for those enzymes and have larger storage capacity. Additionally, plants can produce these complex compounds directly from CO2 using sunlight energy and do not require expensive fermentation methods which require sugar and energy input. Therefore, plants could be an attractive alternative chassis for a sustainable large-scale production of various beneficial plant natural products.
Recent advances in multiomics approaches accelerated the identification of enzymes leading to the complete elucidation of many complex plant natural product pathways (Itkin et al. 2013; Lou et al. 2021; Bryson et al. 2023; Li et al. 2023). Many of these studies also reconstructed entire biosynthetic pathways through plant synthetic biology as a proof-of-concept (Nett et al. 2020; Hong et al. 2022; De La Peña et al. 2023; Sonawane et al. 2023). Nicotiana benthamiana has been mostly used as a heterologous plant chassis, where multiple target genes can be transiently coexpressed using the Agrobacterium-mediated infiltration method (Sainsbury et al. 2009; Bally et al. 2018). Thus far, the majority of studies mainly focused on the design-build-test components of the DBTL cycle (Nett et al. 2020; Hong et al. 2022; Sonawane et al. 2023). However, as the throughput of “build” and “test” stages is much more limited in plants than in microbes (Shih 2018), carefully evaluating and incorporating the “learning” component of the DBTL cycle to the next “design” phase (Jullesson et al. 2015; Choi et al. 2019) will be crucial for effectively optimizing a target biosynthetic pathway for large-scale production of target compounds in planta.
L-Tyrosine is one of the three aromatic amino acids and is a precursor for various beneficial plant natural products including tocopherols (vitamin E), benzylisoquinoline alkaloids (e.g. morphine), phenethylisoquinoline alkaloids (e.g. colchicine), and catecholamines (e.g. dopamine) (Schenck and Maeda 2018). TyrA arogenate dehydrogenase enzyme catalyzes the last step of L-tyrosine biosynthetic pathway and is feedback inhibited by L-tyrosine in most plant species (Fig. 1) (Rippert and Matringe 2002a, 2002b; Maeda and Dudareva 2012). BvTyrAα from table beet (Beta vulgaris) has been reported to have a relaxed feedback inhibition (Lopez-Nieves et al. 2018), and overexpression of BvTyrAα results in hyperaccumulation of L-tyrosine in planta (Lopez-Nieves et al. 2018, 2022; de Oliveira et al. 2019).
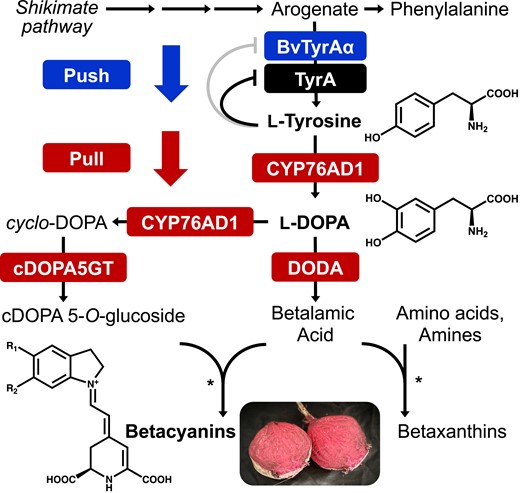
“Push-and-pull” strategy to efficiently produce betalains from tyrosine. Arogenate dehydrogenase (TyrA) catalyzes the last step of L-tyrosine biosynthetic pathway and is feedback inhibited by L-tyrosine in most plant species. BvTyrAα from beet shows relaxed feedback inhibition by L-tyrosine and was used to “push” the supply of tyrosine in this study. Betalain biosynthetic pathway was used to “pull” the enhanced L-tyrosine supply for producing target L-tyrosine-derived compounds, betalains. Cytochrome P450 CYP76AD1 catalyzes the first step to convert L-tyrosine to L-DODA, which is then converted to betalamic acid by DODA or to cDOPA by CYP76AD1. cDOPA5GT glucosylates cDOPA to generate cDOPA 5-O-glucoside. Stars denote the spontaneous condensation of betalamic acid with amino acids or amines to form yellow betaxanthins or with cDOPA 5-O-glucoside to form purple betacyanins. Three compounds in bold with the chemical structures are the ones mainly focused on this study.
Betalain pigment are tyrosine-derived compounds that are widely used for natural food dye, with a growing interest due to their potential health benefits and replacement to synthetic dyes (Azeredo 2009; Calva-Estrada et al. 2022). The first step of the betalain biosynthetic pathway is catalyzed by cytochrome P450 76AD1/5/6 (CYP76AD1/5/6) which hydroxylates L-tyrosine to form L-DOPA, from which the pathway diverges (Fig. 1) (Hatlestad et al. 2012; Polturak et al. 2016; Sunnadeniya et al. 2016). L-DOPA can be cleaved by L-DOPA 4,5-dioxygenase (DODA) to form a 4,5-seco-DOPA intermediate which is then spontaneously cyclized to betalamic acid (Christinet et al. 2004). Alternatively, L-DOPA can be oxidized and cyclized to cyclo-DOPA (cDOPA) by the second activity of the bifunctional CYP76AD1 enzyme, which is then converted to cDOPA 5-O-glucoside by cDOPA 5-O-glucosyltransferase (cDOPA5GT) (Vogt et al. 1999). Then, betalamic acid can be spontaneously condensed either with cDOPA 5-O-glucoside to form red betacyanins or with amino acids or amines to form yellow betaxanthins (Fig. 1) (Schliemann et al. 1999; Sasaki et al. 2004, 2005). Identification of enzymes in the betalain biosynthetic pathway enabled engineering of different plant species to heterologously produce betalains (Harris et al. 2012; Sunnadeniya et al. 2016; Polturak et al. 2017; Grützner et al. 2021; Deng et al. 2023; Ge et al. 2023). Due to their coloration and the fact that their biosynthetic pathway requires relatively few enzymes, betalains have recently been widely used as visual markers, for example, in plant transformation or protein–protein interaction studies (He et al. 2020; Chen et al. 2023; Wang et al. 2023). Despite these wide uses of betalains, it remains to be investigated how different pathway enzymes are coordinated with each other for efficient production of betalain pigments.
Here, we aimed to increase the production of target downstream compounds by supplying enhanced levels of precursor using a “push-and-pull” strategy (Fig. 1, Kacser and Acerenza 1993) that has been implemented successfully in different systems (Zhang et al. 2015; Bröker et al. 2018). The deregulated BvTyrAα was expressed for “pushing” the metabolic flux toward the L-tyrosine biosynthetic pathway, and simultaneously, the biosynthetic pathway of betalain pigments was used as a visual readout to “pull” the increased levels of L-tyrosine in N. benthamiana. Unexpectedly, however, betalain production was reduced, rather than increased, when the betalain pathway genes were coexpressed with BvTyrAα. To decipher this puzzling result, we further conducted detailed metabolite and transcript analyses, which suggested that DODA is a major rate-limiting step of the betalain biosynthetic pathway in this system. Learning from the first DBTL cycle, we then alleviated the rate-limiting step by expressing additional BvDODA together with expression of BvTyrAα to supply additional precursor, which drastically increased betalain levels up to 2.87 mg/gFW (5.21 μmol/gFW). Our findings demonstrate that optimizing a target biosynthetic pathway by alleviating a bottleneck step, coupled with enhanced precursor supply, can substantially increase the production of target compounds in planta. The gained knowledge will be useful for successful implementation of plant synthetic biology to increase chemical production.
Results
Expression of BvTyrAα with betalain pathway genes unexpectedly reduced betalain production in N. benthamiana
To test if the “push-and-pull” strategy (Fig. 1) works for efficient betalain production in N. benthamiana, we generated four DNA constructs: (i) a BvTyrAα-expressing “push” construct, (ii) a “pull” construct overexpressing three betalain biosynthetic genes from either B. vulgaris or Mirabilis jalapa—BvCYP76AD1, BvDODAα1 (hereafter simply termed BvDODA), and MjcDOPA5GT (Polturak et al. 2016, 2017; Timoneda et al. 2018)—(iii) a “push/pull” construct expressing both BvTyrAα and three betalain biosynthetic genes, and (iv) an empty vector (EV) as a negative control (Fig. 2A). The modular Golden Gate cloning method (Engler et al. 2014) was used to assemble level 1 and 2 constructs by using different promoters, terminators, and tags in each transcriptional unit to avoid possible recombination between identical sequences (Fig. 2A).
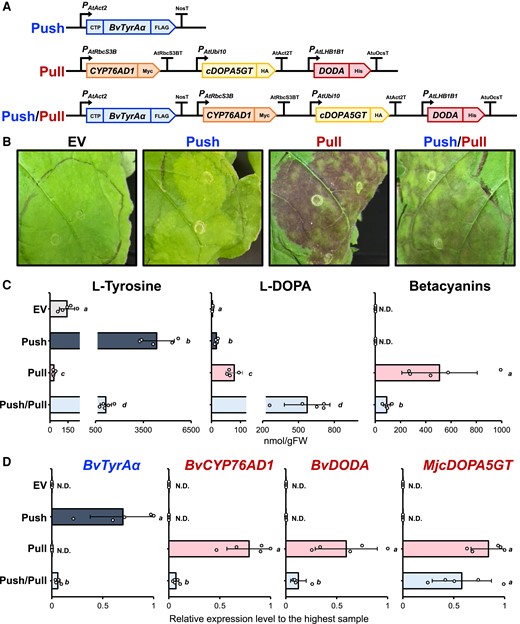
BvTyrAα expression reduces betalain production in N. benthamiana. A) Schematic diagram of recombinant DNA constructs generated using Golden Gate cloning method for testing the “push-and-pull” strategy. Arabidopsis Actin2 promoter (PAtAct2) and nopaline synthase terminator (NosT) were used for expressing BvTyrAα coding sequence flanked with N-terminal petunia chloroplast transit peptide (CTP) and C-terminal FLAG tag; Arabidopsis Rubisco small subunit 3B promoter (PAtRbcS3B) and terminator (AtRbcS3BT) were used for expressing BvCYP76AD1 coding sequence flanked with C-terminal Myc tag; Arabidopsis Ubiquitin10 promoter (PAtUbi10) and Arabidopsis Actin2 terminator (AtAct2T) were used for expressing MjcDOPA5GT coding sequence flanked with C-terminal HA tag; Arabidopsis light-harvesting chlorophyll–protein complex II subunit B1 promoter (PAtLHB1B1) and A. tumefaciens octopine synthase terminator (AtuOcsT) were used for expressing BvDODA flanked with C-terminal His tag. B) Infiltrated N. benthamiana phenotype at 3 dpi expressing the generated constructs from A). Betalain pigmentation was induced in both “pull” and “push/pull”-expressing leaves but not in EV or “push”-expressing leaves. EV, empty vector. C) Metabolite analysis using the infiltrated N. benthamiana samples. L-Tyrosine and L-DOPA levels were analyzed using LC-MS, and total betacyanins was quantified using a spectrophotometer. D) Transcript levels of each transgene in infiltrated N. benthamiana samples detected by RT-qPCR. Letters denote significant differences in a pairwise comparison (P < 0.05, Student's two-tailed t test). Bars represent expression level relative to the sample with the highest expression. Data are means of 5 biological replicates ± Sd. EV, empty vector; N.D, not detectable.
To quickly test their functionality, we transiently expressed these four constructs in N. benthamiana using the agroinfiltration method (Sparkes et al. 2006). Betalain pigments were observed in the leaves expressing either the “pull” or “push/pull” construct but not in those expressing the “push” or EV construct (Fig. 2B), confirming that the assembled betalain constructs are functional in N. benthamiana leaves. Unexpectedly, weaker pigmentation was observed in the leaves infiltrated with the “push/pull” construct than in those infiltrated with the “pull” construct (Fig. 2B). Betalain pigmentation became stronger over time in the leaves expressing either “push/pull” or “pull” construct; however, the clear differences between two constructs persisted throughout the time points after 2 days postinfiltration (dpi) (Supplementary Fig. S1).
To quantitatively analyze metabolic impacts, infiltrated leaf spots were sampled directly into liquid nitrogen at 3 dpi to quickly stop the metabolic reactions in the leaves. To quantify total levels of betacyanins, the dominant class of betalains in plants (Delgado-Vargas et al. 2000), metabolites were extracted from the frozen samples, and their absorbance at 538 nm was measured using spectrophotometer (Calva-Estrada et al. 2022). Corresponding to the visual phenotype (Fig. 2B), the total betacyanin level was significantly lower in the leaves expressing the “push/pull” construct than in those expressing the “pull” construct (P < 0.05), whereas no betalains were detectable in the leaves expressing either the “push” or EV construct (Fig. 2C).
Since we did not see an expected increase in total betacyanins with the “push/pull” construct, we sought to examine the levels of L-tyrosine and L-DOPA, an intermediate of betalain biosynthesis (Fig. 1). When infiltrated leaves were analyzed using LC-MS, L-tyrosine levels were the highest in the leaves expressing the “push” construct, followed by those expressing “push/pull,” EV, and “pull.” The significantly increased L-tyrosine accumulation by either the “push” or “push/pull” construct compared with the EV construct (P < 0.01; Fig. 2C) confirmed that the expression of deregulated BvTyrAα increased L-tyrosine levels in planta (Lopez-Nieves et al. 2018; de Oliveira et al. 2019). The “push/pull” or “pull” construct expression reduced L-tyrosine levels significantly compared with the “push” or EV construct, respectively (P < 0.05; Fig. 2C), suggesting that the expression of the betalain pathway genes led to the consumption of L-tyrosine for betalain production in N. benthamiana.
L-DOPA, which is derived from L-tyrosine via a ring hydroxylation (Fig. 1), was the highest in the leaves expressing “push/pull,” followed by “pull,” “push,” and then EV constructs (Fig. 2C). The higher L-DOPA levels in the “push/pull” and “pull” constructs than in the other constructs confirmed that the expression of BvCYP76AD1 can convert L-tyrosine to L-DOPA in planta (Hatlestad et al. 2012; Polturak et al. 2016, 2017). The “push/pull” or “push” construct expression significantly increased L-DOPA levels compared with the “pull” or EV construct, respectively (P < 0.01; Fig. 2C), suggesting that the additional L-tyrosine precursor supply by the BvTyrAα expression increased L-DOPA levels in N. benthamiana. This result suggests that the elevated levels of L-DOPA were not efficiently directed to downstream betalain biosynthesis, since the expression of the “push/pull” construct led to lower betacyanin accumulation than that of the “pull” only construct (Fig. 2, B and C).
Using the same leaf samples, RT-qPCR was conducted to investigate the transcript levels of different transgenes expressed in N. benthamiana leaves. The BvTyrAα expression was detected only in the leaves infiltrated with either the “push” or “push/pull” construct, as expected (Fig. 2D). Likewise, the expression levels of three betalain genes were only detected in the leaves expressing either “pull” or “push/pull” construct (Fig. 2D). Notably, the expression levels of all transgenes in the leaves expressing the “push/pull” construct were significantly or somewhat lower than those expressing either the “push” or “pull” construct (P < 0.05, BvTyrAα, BvDODA; P < 0.01, BvCYP76AD1; Fig. 2D). Therefore, the reduced transgene expression levels might have contributed to the lower accumulation of L-tyrosine and betacyanins in the “push/pull” compared with the “push” or “pull” only construct, respectively.
Coinfiltration of the “push” and “pull” independent constructs rescued low transgene expression but still showed low betalains
To test if the low transgene expression in the “push/pull” construct is due to posttranscriptional gene silencing, we coexpressed the p19 silencing suppressor (Sainsbury et al. 2009; Brophy et al. 2022; Dudley et al. 2022), together with the generated constructs in N. benthamiana. Even with the p19 expression, the lower pigmentation (Supplementary Fig. S2A) and betacyanin accumulation (Supplementary Fig. S2B) were still observed in the “push/pull” expressing leaf samples than in the “pull” expressing leaves. The RT-qPCR analyses showed that the p19 expression somewhat enhanced the transgene expression in the leaves expressing “push” or “pull” construct but not in the “push/pull”-expressing leaves (Supplementary Fig. S2C). Importantly, the transgene levels were still significantly lower in the leaves expressing the “push/pull” construct than in those expressing the “push” or “pull” construct, even with the p19 coexpression (P < 0.05; Supplementary Fig. S2C).
Previous studies have reported that high levels of aromatic compounds, including L-DOPA, can cause toxicity in microbes (Gosset 2009; Eisenman et al. 2011). Therefore, we speculated that potential accumulation of aromatic intermediates (e.g. L-tyrosine, L-DOPA) might have negatively affected the viability of Agrobacterium containing the “push/pull” construct, leading to the reduced transgene expression upon infiltration. To this end, we tested if coinfiltration of separate Agrobacterium strains harboring each “push” and “pull” construct would alleviate this potential issue (Supplementary Fig. S3A). Consistent with the earlier result of expressing the single “push/pull” construct (Fig. 2B), betalain pigmentation was again weaker when the “pull” construct was coexpressed with the “push” construct than when the “pull” construct was coexpressed with the EV construct (Supplementary Fig. S3B). LC-MS metabolite quantification showed that the coinfiltration of the “push” and “pull” constructs led to significantly higher L-tyrosine and L-DOPA levels (P < 0.01) but lower betacyanin levels (P < 0.05) than that with the coinfiltration of EV and “pull” constructs (Supplementary Fig. S3C). Importantly, RT-qPCR analysis of BvCYP76AD1 and BvDODA transcript levels showed that these genes were similarly expressed in the leaves coexpressing the “push” and “pull” construct as compared with those in the leaves coexpressing the EV and “pull” constructs (Supplementary Fig. S3D). Still, the highly accumulated L-DOPA in the “push” and “pull” coexpressing leaves were not efficiently converted to betalains (Supplementary Fig. S3B). These results demonstrate that although the coinfiltration of the “push” and “pull” constructs alleviates the reduced expression of BvCYP76AD1 and BvDODA, betalain production remains lower compared with that in the leaves coexpressing EV and “pull” constructs. These findings together suggest that the expression of BvTyrAα and BvCYP76AD1 produced high amounts of L-tyrosine and L-DOPA, respectively; however, this high level of L-DOPA was not converted to betalains efficiently, likely due to the presence of a limiting enzymatic step(s) within betalain biosynthesis.
The conversion from L-DOPA to betalains is still limited in the RUBY construct
The RUBY construct, expressing three betalain biosynthetic genes in a single polycistronic expression unit, has recently been used to produce betalains in different plant species, such as Arabidopsis (Arabidopsis thaliana), rice (Oryza sativa) calli, carrot (Daucus carota ssp. Sativus), and cotton (Gossypium hirsutum) (He et al. 2020; Deng et al. 2023; Ge et al. 2023). To test if this widely used betalain biosynthetic construct can overcome the limited conversion of L-DOPA into betalains, the RUBY construct was used as a new “pull” construct and was coexpressed with either EV or the “push” construct in N. benthamiana leaves (Fig. 3A). The expression of the RUBY “pull” construct resulted in expected pigment production; however, the additional coexpression of the “push” with the RUBY “pull” constructs did not significantly increase the betalain production (Fig. 3, B and C). Again, the coexpression of the “push” and the RUBY “pull” constructs led to hyperaccumulation of both L-tyrosine and L-DOPA (Fig. 3C), similarly to the earlier results (Fig. 2C and Supplementary Fig. S3C). Therefore, these accumulated precursors were not efficiently converted to betalain pigments, suggesting that the conversion of L-DOPA to betacyanin is also limited in the RUBY construct.
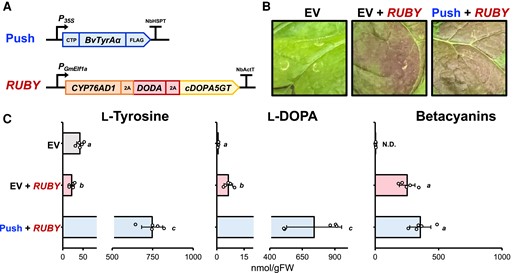
The conversion from L-DOPA to betalains is still limited in the RUBY “pull” construct. A) Schematic diagram of recombinant DNA used in this coinfiltration assay. P35S and N. benthamiana heat shock protein terminator (NbHSPT) were used for expressing the push construct; the PGmElf1a and NbActT were used for expressing the RUBY construct. B) N. benthamiana phenotype coinfiltrated with the generated constructs from A) at 3 dpi. EV, empty vector. C) Metabolite analysis using infiltrated N. benthamiana samples. L-Tyrosine and L-DOPA levels were analyzed using LC-MS, and total betacyanins was quantified using a spectrophotometer. Letters denote significant differences in a pairwise comparison (P < 0.05, Student's two-tailed t test). Data are means of 5 biological replicates ± Sd. EV, empty vector; N.D, not detectable.
Additional DODA expression boosts betalain production when coinfiltrated with the “push” and the “pull” constructs
Incorporating the learned knowledge from the initial DBTL cycle above, we designed the next experiment to test if an additional expression of BvDODA could rescue this reduced betalain production phenotype observed with the “push” and “pull” coexpression. To this end, we generated an additional construct that overexpresses each one of the three betalain pathway genes—BvCYP76AD1, BvDODA, or MjcDOPA5GT (Fig. 4A). An Agrobacterium strain containing each one of these constructs, or the EV construct as a negative control, was mixed with those containing the “pull” construct together with the “push” or EV construct and infiltrated into N. benthamiana.
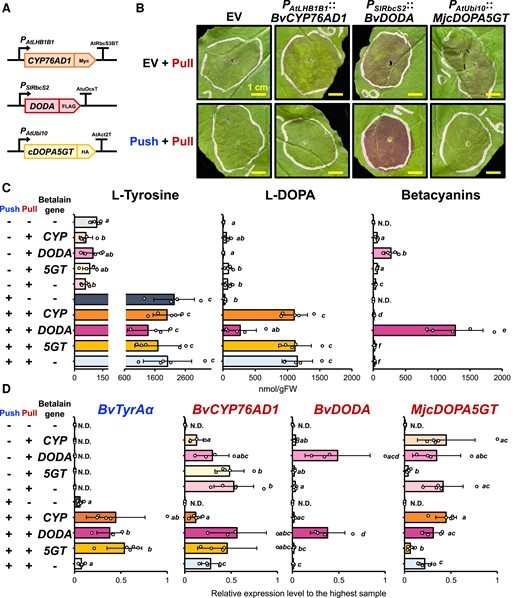
Additional BvDODA expression together with the “push” and “pull” constructs boosts betalain production. A) Schematic diagram of additional betalain gene constructs used in this coinfiltration assay. PAtLHB1B1 and Arabidopsis Rubisco small subunit 3B terminator (AtRbcS3BT) were used for expressing BvCYP76AD1 coding sequence flanked with C-terminal Myc tag; tomato Rubisco promoter (PSlRbcS2) and AtuOcsT were used for expressing BvDODA flanked with C-terminal His tag; PAtUbi10 and AtAct2T were used for expressing MjcDOPA5GT coding sequence flanked with C-terminal HA tag. B) Infiltrated N. benthamiana phenotype at 3 dpi expressing the generated constructs from A). EV, empty vector. C) Metabolite analysis using infiltrated N. benthamiana samples. L-Tyrosine and L-DOPA levels were analyzed using LC-MS, and total betacyanins was quantified using a spectrophotometer. D) Transcript levels of each transgene in the infiltrated N. benthamiana samples detected by RT-qPCR. Letters denote significant differences in a pairwise comparison (P < 0.05, Student's two-tailed t test). Bars represent expression level relative to the sample with the highest expression. Data are means of 4 or 5 biological replicates ± Sd. N.D, not detectable.
The additional expression of BvDODA, but not of BvCYP76AD1 or MjcDOPA5GT, showed significantly higher betalain accumulation, and the most drastic increase in betalain accumulation was observed when both the “push” and the “pull” constructs were coinfiltrated with the additional BvDODA (1,272 ± 430 nmol/gFW) (Fig. 4, B and C). Again, L-tyrosine and L-DOPA were highly accumulated only when the “push” construct was coinfiltrated with the “pull” construct (Fig. 4C). Notably, L-DOPA levels were significantly decreased (P < 0.05) when the “push” and the “pull” constructs were coexpressed with the additional BvDODA but not with the additional BvCYP76AD1 or MjcDOPA5GT constructs (Fig. 4C). These results suggest that the high levels of L-DOPA, resulting from coexpression of the “push” and the “pull” constructs, were efficiently converted to betalains upon additional BvDODA expression.
RT-qPCR analyses showed that the transcript levels of BvDODA were substantially elevated in the leaves expressing additional BvDODA (Fig. 4D), whereas those of BvCYP76AD1 or MjcDOPA5GT were significantly lower when additional BvCYP76AD1 or MjcDOPA5GT was expressed (P < 0.05), potentially due to the transgene silencing (Fig. 4D). As BvCYP76AD1 and MjcDOPA5GT were driven by different promoters (AtLHB1B1 and AtUbi10, respectively) than BvDODA (SlRbcS2) in this experiment, we next generated additional constructs of BvCYP76AD1 and MjcDOPA5GT driven under the same SlRbcS2 promoter (Supplementary Fig. S4A) and conducted a coinfiltration assay. However, the resulting BvCYP76AD1 and MjcDOPA5GT transcript levels were still decreased when either of those was additionally expressed (Supplementary Fig. S4D). Also, the strongest pigmentation and the highest betacyanin levels were again observed only when additional BvDODA was expressed with the “push” and “pull” constructs (Supplementary Fig. S4, B and C).
We also conducted a similar experiment using the RUBY “pull” construct, which is codon optimized and thus could escape homology-mediated gene suppression (Grzech et al. 2023). The additional expression of BvCYP76AD1, BvDODA, or MjcDOPA5GT genes led to expected increases in expression (Fig. 5A). This time, the additional betalain gene expression did not cause silencing of the RUBY-derived betalain pathway gene expression (Fig. 5A), suggesting that we successfully avoided the homology-mediated gene suppression. Still, the strongest pigmentation and betacyanin levels were observed only when the additional BvDODA was expressed with the “push” and RUBY “pull” constructs (Fig. 5, B and C). Additional BvCYP76AD1 or MjcDOPA5GT expression did not alter betacyanin production when the RUBY “pull” was expressed either with or without “push” expression (Fig. 5C). Consistent with other experiments, L-tyrosine and L-DOPA levels were highly accumulated only when the “push” construct was coexpressed. This time, the average accumulation of betacyanins with the expression of the “push,” RUBY “pull,” and additional BvDODA constructs reached 3,339 ± 1,058 nmol/gFW (Fig. 5C) with the highest sample producing at 5,211 nmol/gFW, which is equivalent to 2.87 mg/gFW of betacyanin pigments. These data together indicate that DODA was the rate-limiting enzyme of the reconstructed betalain biosynthetic pathway in N. benthamiana. Once the betalain pathway is optimized by alleviating this rate-limiting step, additional L-tyrosine precursor supply can drastically increase the production of betalain pigments.
DODA is a rate-limiting step in the betalain biosynthetic pathway. A) Schematic diagram of additional betalain gene constructs used in this coinfiltration assay. PSlRbcS2 was used in all three additional betalain gene expressing constructs. AtRbcS3BT, AtuOcsT, and AtAct2T were used for BvCYP76AD1, BvDODA, and MjcDOPA5GT expression constructs, respectively. Transcript levels of each transgene in infiltrated N. benthamiana samples detected by RT-qPCR. Bars represent expression level relative to the sample with the highest expression. B) Infiltrated N. benthamiana phenotype at 3 dpi expressing the generated constructs from A). EV, empty vector. C) Metabolite analysis using infiltrated N. benthamiana samples. L-Tyrosine and L-DOPA levels were analyzed using LC-MS, and total betacyanins was quantified using a spectrophotometer. Letters denote significant differences in a pairwise comparison (P < 0.05, Student's two-tailed t test). Data are means of 5 biological replicates ± Sd. N.D, not detectable.
Discussion
Combined strategies to increase in planta betalain production by “learning” from a previous DBTL cycle
Betalains are tyrosine-derived pigments with yellow or red coloration, uniquely found in plants within the Caryophyllales order. Not only do betalains have potential health benefits including anticancer, anti-inflammatory, or antidiabetic activities (Gandía-Herrero et al. 2016; Khan 2016), they are also widely used as natural food colorants due to their stability in a wide pH range (Azeredo 2009; Calva-Estrada et al. 2022). Core enzymatic steps of betalain biosynthetic pathway have been elucidated throughout various studies (Vogt et al. 1999; Christinet et al. 2004; Hatlestad et al. 2012; Polturak et al. 2016; Sunnadeniya et al. 2016), which enabled engineering various plant species, including Arabidopsis, tomato (Solanum lycopersicum), potato (Solanum tuberosum), cotton, and carrot (Harris et al. 2012; Sunnadeniya et al. 2016; Polturak et al. 2017; Grützner et al. 2021; Deng et al. 2023; Ge et al. 2023), to heterologously produce betalains. Due to its visual property, the RUBY betalain biosynthetic construct has recently been widely used as a reporter for selecting early transformation events, haploid identification, protein–protein interaction, or DNA–protein interaction (He et al. 2020; Chen et al. 2023; Sun et al. 2023; Wang et al. 2023). However, prior studies primarily focused on the formation of the final product, betalains, without monitoring the intermediates (Polturak et al. 2017; Timoneda et al. 2018; Grützner et al. 2021). In our work, by carefully analyzing changes of upstream intermediate metabolites upon expression of various betalain constructs, we identified a key bottleneck step of the betalain biosynthetic pathway.
Prior studies reported that L-tyrosine availability is a limiting factor to produce betacyanins in Arabidopsis (Sunnadeniya et al. 2016), and the expression of BvTyrAα increased the betalain production in N. benthamiana and tomato fruit by around 7- and 10-time, respectively (Timoneda et al. 2018; Grützner et al. 2021). However, during our first iteration to introduce the betalain pathway into N. benthamiana, we unexpectedly observed that BvTyrAα expression negatively impacts betalain production. As the betalain pigments were mostly detected in the vacuoles of mesophylls (Supplementary Fig. S5, A and B), endogenous transport systems appear to be able to shuttle the nonnative betalain pigments into vacuoles in N. benthamiana leaves. Further detailed analyses of the pathway intermediates showed that both the “push/pull” expression and coexpression of the “push” with “pull” constructs led to high accumulation of L-tyrosine and L-DOPA in N. benthamiana; however, the further conversion from L-DOPA to betalains was limited, resulting in lower betalain production (Fig. 2C and Supplementary Fig. S3C). The decarboxylated products of L-tyrosine and L-DOPA, tyramine and dopamine, respectively, were increased but only slightly (around 3-fold; Supplementary Fig. S6) and at much lower quantities than tyrosine and L-DOPA (around 28- or 69-fold increase), suggesting that the formation of these side products by endogenous N. benthamiana enzymes is likely minimal.
These results together revealed that, at least in our tested system, the conversion of the accumulated L-DOPA is a major rate-limiting step of the betalain biosynthetic pathway. Furthermore, the additional expression of the BvDODA gene together with the “push” and “pull” constructs drastically increased betalain production (by over 35-fold; Fig. 4C), which was also the case when the BvDODA gene was expressed with the “push” and RUBY “pull” constructs (Fig. 5C). Given that L-DOPA is a branch point of the betalain biosynthetic pathway (Fig. 1), enhancing the L-DOPA oxidation to form cDOPA can also accelerate L-DOPA conversion toward betalain production. However, increased expression of the bifunctional CYP76AD1 enzyme will not only enhance L-DOPA oxidase activity but also tyrosine hydroxylase activity. A prior study has identified mutations that alter L-tyrosine hydroxylation and L-DOPA oxidation activity of CYP76AD1, but none of them increased only L-DOPA oxidase activity (DeLoache et al. 2015). Therefore, searching for such mutation could further alleviate L-DOPA accumulation and boost betalain production, especially with the DODA overexpression.
Our finding illuminated that even in the betalain pathway that requires expression of a few enzymes, it is critical to optimize the relative expression of the target biosynthetic genes to achieve optimal “pull” strategy. Only then, additional engineering to “push” the upstream precursor supply can enable efficient and high-level production of the final product. As a result, this study reached a maximum betacyanin production of 2.87 mg/gFW, which is higher than those in earlier studies and the native plant host, red beet, in which it can accumulate in the range of 1.57 to 2.70 mg/gFW (Sawicki et al. 2016). Therefore, careful “testing” of the engineering outcome provides valuable knowledge to “learn” and then “redesign” to further increase the in planta production of target products.
Regulatory mechanisms of DODA that potentially limit betalain production
DODA enzymes catalyze the ring cleavage of L-DOPA to form unstable 4,5-seco-DOPA, which is then spontaneously cyclized to betalamic acid (Brockington et al. 2015; Bean et al. 2018; Sheehan et al. 2020). A very recent study showed that the in vitro activity of BvDODAα1 enzyme from B. vulgaris, which was used in our study, is subjected to a strong substrate inhibition as L-DOPA concentration increases beyond 0.28 mM (Guerrero-Rubio et al. 2023). In our study, the expression of the “push/pull” construct, as compared with the “pull” only construct, led to higher levels of L-DOPA but not betalains. Therefore, this unexpected result could be due to the inhibition of the DODA enzyme activity when L-DOPA is highly accumulated upon coexpressing BvTyrAα and betalain biosynthetic genes.
Previous studies, however, did not observe the reduced betalain production when BvTyrAα was coexpressed with the betalain pathway genes in tomato fruit and N. benthamiana leaves (Timoneda et al. 2018; Grützner et al. 2021), which somewhat contradicts our current findings. In these prior studies, the same constitutive promoters (e.g. 35S and Ubi10) were used multiple times to express all three betalain biosynthetic genes, whereas our study used different promoters for each betalain biosynthetic gene to avoid a potential unwanted recombination event during the transformation process. Unintentionally, we used a relatively weaker promoter (i.e. AtLHB1B1) to drive expression of BvDODA than of the other two genes in the “pull” construct (Fig. 2A) (Klepikova et al. 2016). Therefore, it is possible that the prior studies likely had a strong DODA expression, which might have prevented the accumulation of L-DOPA to a level high enough to inhibit DODA activity.
To overcome this issue in the future, we can use either a strong promoter to drive DODA and/or a different DODA enzyme with a weak to no substrate inhibition for better betalain production. Although only a few DODA enzymes have been characterized so far (Girod and Zryd 1991; Gandía-Herrero and García-Carmona 2012; Contreras-Llano et al. 2019; Guerrero-Rubio et al. 2023), the DODA enzyme from Anabaena cylindrica showed substrate inhibition by L-DOPA but at a much higher concentration than BvDODAα1 (beyond 1.5 mM) (Guerrero-Rubio et al. 2020). The other characterized DODA enzyme from Gluconacetobacter diazotrophicus did not show substrate inhibition by L-DOPA up to 4 mM, although it was inhibited by alternative substrates, such as catechol and dihydrocaffeic acid (Contreras-Llano et al. 2019). Exploring biochemical diversity of DODA enzymes from various betalain-producing species may further reveal different DODA regulation and identify useful DODA enzymes that can be used for high production of betalains.
A potential intermediate toxicity in Agrobacterium and heterologous plant hosts
In this study, transgene expression was reduced when the single “push/pull” construct, rather than either the individual “push” or “pull” construct, was expressed in N. benthamiana (Fig. 2D). Even the coexpression of p19 silencing suppressor (Sainsbury et al. 2009; Brophy et al. 2022; Dudley et al. 2022) could not rescue this reduced expression (Supplementary Fig. S2C); however, coexpressing both “push” and “pull” constructs by mixing and coinfiltrating separate Agrobacterium cultures, rather than expressing a single “push/pull” construct, resolved this issue (Supplementary Fig. S3D). A recent study also observed reduced transgene expression when multitranscriptional units required for biosynthesis of precondylocarpine acetate, a late stage precursor of vinblastine, were expressed in a single construct, as compared with when separate constructs of individual transcriptional units were mixed (Grzech et al. 2023). The use of different regulatory elements (i.e. promoters and terminators) for each of four transcriptional units in the single construct improved the transgene expression and the final product yield, suggesting that homology-mediated epigenetic silencing may be an underlying issue in the prior study (Grzech et al. 2023). However, this may not be the case in our study since we used different promoters and terminators for each transcriptional unit.
Instead, it is possible that leaky expression of the set of transgenes from the “push/pull” construct in Agrobacterium might have resulted in accumulation of toxic intermediates, such as L-DOPA or its derivatives, negatively affecting the viability of Agrobacterium (Gosset 2009; Eisenman et al. 2011). Mixing several Agrobacterium strains containing different transcriptional units, as conducted here (Supplementary Fig. S3) and in many studies for rapid combinatorial testing in the transient expression system (Nett et al. 2020; Brophy et al. 2022; Sonawane et al. 2023), might have prevented this issue. However, when the mixing strategy is not feasible or ideal, such as for coordinated expression of multiple pathway genes, an alternative solution could be to insert introns in the coding sequences, which prevents the expression of functional transgenes in prokaryotes and hence avoid potential accumulation of toxic intermediates in Agrobacterium (Vancanneyt et al. 1990; Ibrahim et al. 2001; Brophy et al. 2022).
L-DOPA can also negatively affect plant viability, as it is one of the allelochemicals that suppress the growth of neighboring plants (Soares et al. 2014). In a previous study, to avoid possible L-DOPA toxicity, CYP76AD6 was expressed under a tomato fruit-specific promoter, together with MYB12 to enhance L-tyrosine supply, resulting in high accumulation of L-DOPA (Breitel et al. 2021). During the engineering of the cyanogenic glycoside dhurrin biosynthetic pathway, transgenic Arabidopsis expressing first two out of three enzymes, CYP79A1 and CYP71E1, showed stunted and stressed phenotypes due to the accumulation of the toxic cyanohydrine intermediate, which was rescued by the additional expression of UGT85B1, catalyzing the third step (Kristensen et al. 2005). Our study also demonstrated that the additional expression of a later step enzyme, i.e. DODA, to efficiently convert the L-DOPA intermediate drastically improved overall production of betalains (Figs. 4C and 5C). Therefore, optimizing the overall biosynthetic pathway by ensuring strong activity of a rate-limiting step enzyme(s) to avoid accumulation of potentially toxic intermediates will be important to prevent negative physiological impacts that can complicate our engineering effort and/or diminish overall yield of plant biomass and target compounds.
Conclusions and future directions/perspectives
In this study, we successfully optimized the “push-and-pull” strategy for high production of betalains in plants by balancing the enhanced L-tyrosine precursor supply with the efficient precursor utilization. We found that once the betalain biosynthetic pathway is optimized by alleviating a rate-limiting step, the enhanced L-tyrosine precursor can drastically increase the production of betalains. Although blocking side branches from intermediates might see a further improvement, this study reached a maximum betacyanin yield of 2.87 mg/gFW, which is higher than that in previous studies or betalain-producing red beets. Although it remains to be tested if similar strategies work in other plant systems, the gained knowledge and the “push-and-pull” strategy established in this study will be useful for in planta production of other tyrosine-derived compounds as well, especially for those not having an alternative production method established, other than extracting from native plant hosts, such as morphine or colchicine (Glenn et al. 2013; Diamond and Desgagné-Penix 2016). While plant synthetic biology tools are becoming available to efficiently design and build constructs, we still need to work around the limited throughput of testing these constructs due to longer plant life cycles and limited plant transformation capacity. Our findings highlight that the rigorous analysis and learning from the impact of initially designed and built constructs will be crucial especially in plants for effective implementation of synthetic biology.
Materials and methods
Plasmid construction
All recombinant DNAs were generated using the Golden Gate cloning method. The full-length coding sequences of BvCYP76AD1, BvDODAα1, and MjcDOPA5GT were provided by Dr. Alfonso Timoneda and Dr. Samuel Brockington at University of Cambridge, UK. The level 1 RUBY construct under the control of the soybean (Glycine max) elongation factor 1a promoter (PGmElf1a) and N. benthamiana actin terminator (NbActT) and pAGM4673 with the aada1 part for spectinomycin resistant were provided by Dr. Ray Collier from Wisconsin Crop Innovation Center (WCIC). The coding sequences of BvCYP76AD1, BvDODA, and MjcDOPA5GT were amplified using cDNA specific primers flanked with BsaI restriction site and overhangs compatible with the vector pAGM1287 (level 0 vector) from the plant modular cloning (MoClo) toolkit (Engler et al. 2014) (Supplementary Table S1). The coding sequence of BvTyrAα was synthesized into the pUC57 vector with overhangs and BsaI site (El-Azaz et al. 2023). These level 0 parts were assembled into level 1 acceptors using the Golden Gate reaction with promoter, C-terminal tag, and terminator parts as described in Supplementary Table S2 and used for coinfiltration assay. The “push” construct used for coinfiltration assays (Figs. 3 to 5; Supplementary Figs. S3 to S6) was made in our previous study (El-Azaz et al. 2023). The generated level 1 parts were further used for level 2 assembly to generate EV, “push,” “pull,” and “push/pull” constructs as described in Supplementary Table S2. The final level 2 vectors also include the aad1a gene driven by CaMV 35S promoter (P35S) and a C-terminal red fluorescence protein (RFP) fusion protein driven by a seed-specific Oleosin1 (At4g25140) native promoter (Supplementary Table S2).
Transient expression of generated DNA constructs in N. benthamiana
N. benthamiana plants were grown under ∼200 μE of light intensity with a 12 h light and 12 h dark at 24°C and 60% humidity. The generated binary vectors were transformed into Agrobacterium tumefaciens strain GV3101 using electroporation. A. tumefaciens strains harboring each construct was grown in 10 mL of LB media with antibiotics at 28°C in a shaking incubator at 250 rpm for ∼24 h. Overnight saturated cultures were centrifuged at 3,000 × g for 5 min at room temperature. The pellets were washed twice with 3 mL of induction media (10 mM MES pH 5.6, 0.5% [w/v] glucose, 2 mM NaH2PO4, 20 mM NH4Cl, 1 mM MgSO4, 2 mM KCl, 0.1 mM CaCl2, 0.01 mM FeSO4, and 0.2 mM acetosyringone) and incubated in the induction media for 2 to 3 h at room temperature in dark. After the incubation, the cells were pelleted at 3,000 × g for 5 min at room temperature and resuspended into 3 mL of 10 mM MES pH 5.6 with 0.2 mM acetosyringone. All resuspended cultures were diluted to OD600nm of 1.0 and mixed in equal ratios as described by the experimental condition for coinfiltration assay and diluted to OD600nm of 0.5 for level 2 single-construct infiltration. Four-week-old plants were infiltrated on the abaxial side of the leaf using a 1 mL needleless syringe (Becton, Dickinson and Company, 309659) and grown for 3 d under the same condition as described above.
Metabolite analyses
To measure metabolite levels in infiltrated N. benthamiana leaves, infiltrated spots were collected at 3 dpi, flash-frozen in liquid nitrogen, ground to powder, and kept at −80°C until use. Approximately 20 to 30 mg of frozen powders were mixed in 400 μL of the extraction buffer containing 2:1 (v/v) of methanol and chloroform. After adding 300 μL of H2O and then 125 μL of chloroform, samples were centrifuged at 10,000 × g for 5 min. Four hundred fifty μL of the polar phase was transferred to a fresh tube, dried down overnight in a SpeedVac at room temperature, and resuspended into 100 μL of LC-MS-grade water before LC-MS analysis.
For LC-MS analysis, 1 μL of each resuspended sample was injected onto a HSS T3 C18 reversed phase column (100 × 2.1 mm i.d., 1.8 μm particle size; Waters, Milford, USA) and eluted using a 25 min gradient comprising 0.1% (v/v) formic acid in LC-MS-grade water (solvent A) and 0.1% (v/v) formic acid in 90% (v/v) LC-MS-grade acetonitrile (solvent B) at a flow rate of 0.4 mL/min and column temperature of 40°C. The binary linear gradient with the following ratios of solvent B was used: 0 to 1 min, 1%; 1 to 10 min, 1% to 10%; 10 to 13 min, 10% to 30%; 13 to 14.5 min, 30% to 70%; 14.5 to 15.5 min, 70% to 99%; 15.5 to 20 min, 99%; 20 to 20 min, 99% to 1%; and 20 to 25 min, 1%. The MS spectra were recorded using the full scan in positive mode, covering a mass range from 100 to 1,000 mass/charge ratio (m/z). The resolution was set to 140,000, and the maximum scan time was set to 200 ms. The transfer capillary temperature was set to 150°C, while the heater temperature was adjusted to 300°C. The spray voltage was fixed at 3 kV, with a capillary voltage and a skimmer voltage of 25 and 15 V, respectively. The identity of L-tyrosine and L-DOPA peaks were confirmed by comparing their accurate masses and retention times with those of the corresponding authentic standards. Quantification was based on the standard curves of L-tyrosine and L-DOPA generated by injecting different concentrations of authentic chemical standards.
For betacyanin quantification, 30 μL of the same resuspended samples used for LC-MS were transferred to individual wells of a 96 Greiner transparent plate, flat bottom, half well size (#675101) with 5 times serial dilutions. Absorbance at 538 nm was measured using a plate reader (Infinite 200 PRO, TECAN). Multiple data points in the linear range were used for further analysis. Absorbance values were converted to betacyanin content using the molar extinction coefficient ε = 60,000 M/cm (Stintzing et al. 2003). Statistical significance was determined using a 2-tailed Student's t test with a P-value threshold of 0.05 or 0.01 as indicated in the figure legends.
RT-qPCR expression analysis
To test the expression levels of transgenes in the infiltrated N. benthamiana leaves, transcript levels of transgenes were analyzed by RT-qPCR from the same frozen tissues used for corresponding metabolite analyses. Total RNA was isolated from ∼50 to 100 mg of frozen ground tissue using TRIzol (Invitrogen), treated with deoxyribonuclease I (Thermo Fisher Scientific), and reverse-transcribed to synthesize cDNA with M-MLV reverse transcriptase and random hexamer primers (Promega) according to the manufacturer's protocol. qPCR was conducted in a Stratagene Mx3000P (Agilent Technologies) thermocycler using GoTaq qPCR Master Mix (Promega). Specific primers used for the target genes are listed in Supplementary Table S2. Expression of the NbPP2A gene was used to normalize the sample-to-sample variations between different cDNA preparations (Liu et al. 2012). Relative expression levels among different infiltrated leaves were analyzed for each transgene using the 2−ΔΔCt method (Livak and Schmittgen 2001). Statistical significance was determined using a 2-tailed Student's t test with a P-value threshold of 0.05 or 0.01 as indicated in the figure legends.
Accession numbers
Sequence data from this article can be found in the GenBank data libraries under the following accession numbers: KY207372 (BvTyrAα), MH836617 (BvCYP76AD1), MH836616 (BvDODA), and MH836618 (MjcDOPA5GT).
Acknowledgments
We thank Dr. Samuel Brockington and Dr. Alfonso Timoneda for providing us with DNA parts of BvCYP76AD1, BvDODAα1, and MjcDOPA5GT and Dr. Ray Collier for providing us with the RUBY construct and modified pAGM4673 vector with the aad1a part. We thank Dr. Marcos V. V. de Oliveira for critical discussion on designing recombinant DNA construct and Dr. Jorge El-Azaz for critical reading of the manuscript. We thank Dr. Sarah Swanson for initial assistance with microscopy imaging and Madelyn Schaut for initial assistance with LC-MS methodology.
Author contributions
S.J. and H.A.M. conceived the experiments and wrote the manuscript. S.J. performed experiments and analyzed the data.
Supplementary data
The following materials are available in the online version of this article.
Supplementary Figure S1. Betalain pigmentation became stronger over a period, but the difference between constructs persisted.
Supplementary Figure S2. Reduced betalain production in the push/pull construct expressing N. benthamiana even with the p19 silencing suppressor expression.
Supplementary Figure S3. Coinfiltration of mixed Agrobacterium cultures separately harboring “push” or “pull” construct still reduces betalain production in N. benthamiana.
Supplementary Figure S4. An additional betalain gene expression driven by the same promoter also showed dramatically increased betalain production only when the additional BvDODA was expressed.
Supplementary Figure S5. Betalain accumulation was detected in the vacuole of mesophyll cells.
Supplementary Figure S6. The formation of side products, tyramine and dopamine, was slightly increased with the “push” and/or “pull” expression.
Supplementary Table S1. Primers used in this study.
Supplementary Table S2. Golden Gate reaction to generate recombinant DNAs used in this study.
Funding
This work is supported by the grant from the United States Department of Agriculture (NIFA-AFRI 2020-67013-30898).
Data availability
The data underlying this article are available in the article and in its online supplementary material.
Dive Curated Terms
The following phenotypic, genotypic, and functional terms are of significance to the work described in this paper:
References
Author notes
The author responsible for distribution of materials integral to the findings presented in this article in accordance with the policy described in the Instructions for Authors (https://dbpia.nl.go.kr/plphys/pages/General-Instructions) is Hiroshi A. Maeda ([email protected]).
Conflict of interest statement. None declared.