-
PDF
- Split View
-
Views
-
Cite
Cite
Maite Saura-Sánchez, Gabriel Gomez-Ocampo, Matías Ezequiel Pereyra, Carla Eliana Barraza, Andrés H Rossi, Juan P Córdoba, Javier Francisco Botto, B-Box transcription factor BBX28 requires CONSTITUTIVE PHOTOMORPHOGENESIS1 to induce shade-avoidance response in Arabidopsis thaliana, Plant Physiology, Volume 195, Issue 3, July 2024, Pages 2443–2455, https://doi.org/10.1093/plphys/kiae216
- Share Icon Share
Abstract
Shade avoidance syndrome is an important adaptive strategy. Under shade, major transcriptional rearrangements underlie the reallocation of resources to elongate vegetative structures and redefine the plant architecture to compete for photosynthesis. BBX28 is a B-box transcription factor involved in seedling de-etiolation and flowering in Arabidopsis (Arabidopsis thaliana), but its function in shade-avoidance response is completely unknown. Here, we studied the function of BBX28 using two mutant and two transgenic lines of Arabidopsis exposed to white light and simulated shade conditions. We found that BBX28 promotes hypocotyl growth under shade through the phytochrome system by perceiving the reduction of red photons but not the reduction of photosynthetically active radiation or blue photons. We demonstrated that hypocotyl growth under shade is sustained by the protein accumulation of BBX28 in the nuclei in a CONSTITUTIVE PHOTOMORPHOGENESIS1 (COP1)-dependent manner at the end of the photoperiod. BBX28 up-regulates the expression of transcription factor- and auxin-related genes, thereby promoting hypocotyl growth under prolonged shade. Overall, our results suggest the role of BBX28 in COP1 signaling to sustain the shade-avoidance response and extend the well-known participation of other members of BBX transcription factors for fine-tuning plant growth under shade.
Introduction
The shade-avoidance syndrome is a plastic adaptative strategy for plants to anticipate the presence of neighbors by responding with the elongation of stems and petioles, well before shading occurs, to compete for photosynthesis (Ballaré and Pierik 2017). It is well-known that a reduction in Red:Far-Red (R:FR) ratios is an early light signal sensed by the photoreceptor system, principally phytochrome B (phyB). Then, when the actual shading occurs among plants, the transmitted light is especially depleted in red and blue wavelengths, due to the absorption by chlorophylls, reducing the photosynthetically active radiation (PAR) (Fraser et al. 2016). The reduction of the R:FR ratios in the light environment inactivates the phyB, which promotes PHYTOCHROME INTERACTING FACTORS (PIFs) (Lorrain et al. 2008; Huang et al. 2018; Park et al. 2018) and CONSTITUTIVE PHOTOMORPHOGENESIS1 (COP1)/SUPPRESSOR OF PHYTOCHROME A-105 (SPA1) complex (Pacín et al. 2013; Sheerin et al. 2015) signaling pathways. Both signaling networks converge to promote the expression of growth-regulated genes increasing auxin levels and optimizing resource allocation required for the plant reconfiguration in response to shade (Pacín et al. 2016).
PIF proteins regulate the expression of their target genes by binding directly to G-box and E-box motifs in their promoters (Leivar et al. 2012; Zhang et al. 2013). PIFs act as main positive regulators of growth increasing auxin content and sensitivity of plant tissues under shade (Hornitschek et al. 2012; Li et al. 2012; Procko et al. 2014; de Wit et al. 2015; Müller-Moulé et al. 2016; Pucciariello et al. 2018). Some members of the bHLH family, such as PHYTOCHROME RAPIDLY REGULATED 1 (PAR1), PAR2, PIF3-LIKE 1 (PIL1), and LONG HYPOCOTYL IN FAR-RED 1 (HFR1) contribute to inhibit hypocotyl growth by the formation of non-DNA binding heterodimers with PIF proteins (Hornitschek et al. 2009; Buti et al. 2020). In turn, the expression of genes encoding PAR1, PIL1, and HFR1 is rapidly and reversibly induced in response to low R:FR ratios in a PIF-dependent manner (Sessa et al. 2005; Roig-Villanova et al. 2007; Hornitschek et al. 2012). This feedback regulatory mechanism avoids exaggerated growth responses depending on the duration and intensity of the shade light signals (Ciolfi et al. 2013; Buti et al. 2020).
In plants, the E3 ligase activity of COP1 depends on its interaction with SPA proteins. Under low R:FR ratios typical of dense canopies, cop1 and spa1 spa2 spa4 mutants fail to enhance the hypocotyl growth in response to the reduced phyB activity (McNells et al. 1994; Rolauffs et al. 2012). Indeed, the shade signals induce COP1 accumulation in the nucleus (Pacín et al. 2013), and together with SPA1 form an ubiquitin E3 ligase complex, to target growth repressors such as HFR1 (Pacín et al. 2016) or DELLA proteins (Blanco-Touriñán et al. 2020) for their degradation through the 26S proteasome. COP1 can also trigger the stabilization of PIF5 and BES1 proteins to induce growth-induced responses under low R:FR ratios (Sharma et al. 2019, Costigliolo Rojas et al. 2022). Furthermore, the convergence at the transcriptional level between cop1 and pifq mutants, together with the lack of additive effects between cop1 and pif3, pif4, or pif5 mutants reinforces the dependence between both signaling pathways under shade (Pacín et al. 2016).
In Arabidopsis, B-Box transcription factors are encoded by 32 genes, and their proteins are classified into five structural groups according to the number of B-Box domains and the presence of the CCT domain. BBX28 contains one B-Box domain and belongs to the structure group V (BBX26-BBX32) (Gangappa and Botto 2014; Yadav et al. 2020; Song et al. 2020a). In the context of the shade-avoidance responses (SAR), BBX19, BBX21, and BBX22 repress, while COL7/BBX16, BBX18, BBX24, and BBX25 promote the hypocotyl growth under low R:FR ratios (Crocco et al. 2010; Wang et al. 2013). BBX21 and BBX22 are involved in the COP1 signaling because bbx21 cop1, bbx22 cop1, and bbx21 bbx22 cop1 mutants partially restore the SAR (Crocco et al. 2010). Moreover, BBX21 can inhibit the hypocotyl growth by reducing the expression of brassinosteroid (BR) biosynthetic genes including DWF4, BR6OX1, and BR6OX2 in white light (WL), and promoting BAS1, a BR catabolism gene in WL supplemented with FR light (Gómez-Ocampo et al. 2023). Furthermore, the short hypocotyls of bbx24 bbx25 double mutant seedlings under shade are also completely COP1-dependent because the bbx24 bbx25 cop1 triple mutant resembles the cop1 phenotype (Gangappa et al. 2013). BBX24 is involved in the SAR through the sequestration of DELLA proteins, which alleviates the repression of PIF4 activity giving plant plasticity in transient exposures to shade (Crocco et al. 2015). Recently, Saura-Sanchez et al. (2023) found that BBX24 can also interact with JAZ3 to promote the SAR by reducing the DELLA activity. These results suggest complex and overlapping mechanisms for the fine-tuning of the SAR mediated by the BBX transcription factors.
BBX28 is a transcription factor involved that inhibits seedling photomorphogenesis (Lin et al. 2018) and can affect flowering on long days (Liu et al. 2020; Wang et al. 2021), but its function in the SAR is completely unknown. BBX28 is a repressor of seedling photomorphogenesis involved in the COP1-HY5 signaling hub, which activity is tightly regulated by COP1 in darkness (Lin et al. 2018). In the light, BBX28 interacts physically with HY5 and interferes with HY5 binding to the promoters of BBX30 and BBX31 to repress their expression (Song et al. 2020b). In addition, BBX28 is a positive regulator of BR signaling, and physically interacts with BR-ENHANCED EXPRESSION 1, 2, and 3 (BEE1-BEE3), enhancing the transcriptional activity to promote skotomorphogenesis (Cao et al. 2022). Here, we studied the function of BBX28 in the SAR. We found that the growth of hypocotyls under shade is sustained by the protein accumulation of BBX28 in a COP1-dependent manner at the end of the photoperiod to promote the expression of transcription factors and auxin-related genes under prolonged shade.
Results
BBX28 promotes hypocotyl elongation under shade in response to low R:FR ratios
Arabidopsis thaliana seedlings growing under shade exhibit SAR, including the induction of hypocotyl elongation and the inhibition of cotyledon expansion (Morelli and Ruberti 2002). To evaluate the implication of BBX28 in the SAR, we examined the hypocotyl length and the cotyledon size in Col-0 wild-type, two loss-of-function mutants (bbx28-4 and bbx28-5,Supplementary Fig. S1), and two independent overexpressing lines (35S:GFP-BBX28 and 35S:myc-BBX28). Two-day-old de-etiolated seedlings were exposed to WL (Photosynthetic Photon Flux Density, PPFD = 90 µmol m−2 s−1 and Red:Far-Red:Blue, R:FR:B ratio = 1:1:1) or simulated shade, SS (PPFD = 20 µmol m−2 s−1, R:FR:B ratio = 0.14:1:0.23) under short-day photoperiod (10 h of light and 14 h dark, 10L14D) for 4 d. Compared to the wild-type, the bbx28-4 and bbx28-5 loss-of-function mutant lines exhibited a strong and statistically significant reduction in the hypocotyl length under SS in contrast to 35S:GFP-BBX28 and 35S:myc-BBX28 overexpressing lines that displayed exaggerated hypocotyl growth and reduction in the cotyledon size (Fig. 1, A and B and Supplementary Fig. S2). Overall, these results suggest that BBX28 promotes the SAR of Arabidopsis seedlings.
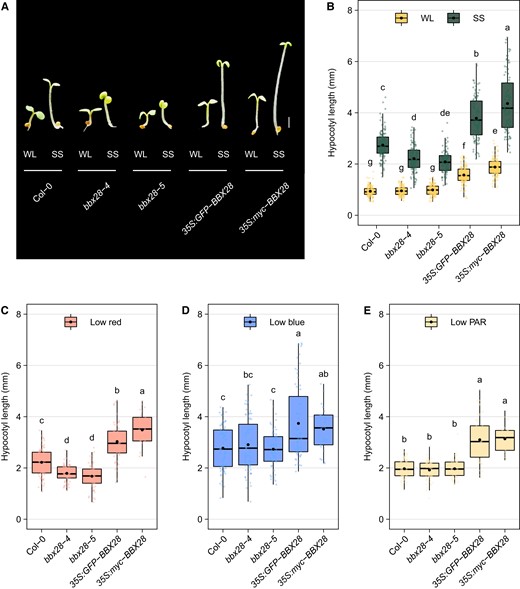
BBX28 promotes hypocotyl elongation under shade. A) Representative images of Col-0, bbx28-4, bbx28-5, 35S:GFP-BBX28, and 35S:myc-BBX28 seedlings grown in a short-day photoperiod (L10:D14) at 22°C for 2 d and maintained in white light (WL) or transferred to simulated shade (SS) treatment for 4 d. Images were digitally extracted for comparison. Scale bar: 1 mm. B) Hypocotyl length of seedlings referred in (A). The boxplots show the median as a solid line and the mean as a black point, the hinges representing the 25th and 75th percentiles, and the whiskers that extend up to a maximum of 1.5 times the interquartile range from the hinges. Individual observations are represented by colored points (n > 90). Different letters indicate significantly different means obtained by two-way ANOVA followed by the post-hoc Tukey's HSD (P < 0.05). C to E) Hypocotyl length of Col-0, bbx28-4, bbx28-5, 35S:GFP-BBX28, and 35S:myc-BBX28 seedlings grown in a short-day photoperiod (L10:D14) at 22°C for 2 d and transferred to (C) low red, (D) low blue or (E) low PAR treatment for 4 d. The boxplots show the median as a solid line and the mean as a black point, the hinges representing the 25th and 75th percentiles, and the whiskers that extend up to a maximum of 1.5 times the interquartile range from the hinges. Individual observations are represented by colored points (n > 50). Different letters indicate significantly different means obtained by one-way-ANOVA followed by the post-hoc Tukey's HSD (P < 0.05).
The SAR can be induced by phytochromes and cryptochromes that perceive the reduction in R:FR ratios; and the reduction of B photons and PAR, respectively (Casal 2013; Ballaré and Pierik 2017). To determine which photoreceptor system is involved in the BBX28-mediated hypocotyl growth under shade, we conducted another experiment. After full de-etiolation, the 2-d-old seedlings were exposed to WL control (PPFD = 90 µmol m−2 s−1 and R:FR:B ratio = 1:1:1) or three different simulated shade treatments for 4 d. We used different light filters to reduce (i) the PPFD (20 µmol m−2 s−1 and R:FR:B ratio = 1:1:1), (ii) the PPFD and the R photons (PPFD = 20 µmol m−2 s−1 and R:FR:B ratio = 0.5:1:1) and (iii) the PPFD and the B photons (PPFD = 20 µmol m−2 s−1 and R:FR:B ratio = 1:1:0.4). Both bbx28-4 and bbx28-5 mutant lines showed shorter hypocotyls than Col-0 under SS with reduced R photons (Fig. 1C), while no differences were observed with reduced B photons or PAR (Fig. 1, D and E). In opposition, both BBX28 overexpressing lines showed significantly larger hypocotyls than Col-0 under the three SS conditions (Fig. 1C). Taken together, these results suggest that BBX28 is necessary for the promotion of hypocotyl elongation in response to low R:FR ratios while the overexpression of BBX28 leads a constitutive SAR.
BBX28 promotes the expression of shade-induced genes
Several members of the BBX family are involved in the SAR of Arabidopsis seedlings (Crocco et al. 2010, 2015; Gangappa et al. 2013; Gómez-Ocampo et al 2023; Saura-Sanchez et al. 2023). For example, BBX21 and BBX24 contribute to the rapid induction of transcriptional PIF target genes such as ATHB2, HFR1, PAR1, or PIL1 under shade (Crocco et al. 2010, 2015). In our experimental conditions, these four genes were strongly induced by SS in Col-0 seedlings (Fig. 2A). The shade-induced expression of PAR1 was significantly lower in the bbx28-5 mutant compared to Col-0 seedlings after 1 and 3 h of SS condition, while the expression of ATHB2 and PIL1 was only affected in bbx28-5 after 3 h of shade (Fig. 2A). To better understand the function of BBX28 in the temporal regulation of shade-induced gene expression, we monitored the PIL1 expression using a transgenic reporter line expressing luciferase (LUC) under the PIL1 promoter (pPIL1:LUC) in Col-0, bbx28-5 and 35S:YFP-BBX28(#b). As expected, the pPIL1:LUC activity was low under WL independently of the genotype (Fig. 2B). Under SS, the pPIL1:LUC activity increased in Col-0 seedlings with a maximum peak after 4 h, and then its expression was maintained high until the end of the photoperiod (Fig. 2B). As expected for the positive function of BBX28 under shade, the bbx28-5 mutant line showed a lower pPIL1:LUC activity than Col-0, while the BBX28 overexpressing line displayed the opposite response to SS (Fig. 2B). These results suggest a prominent function of BBX28 under prolonged shade.
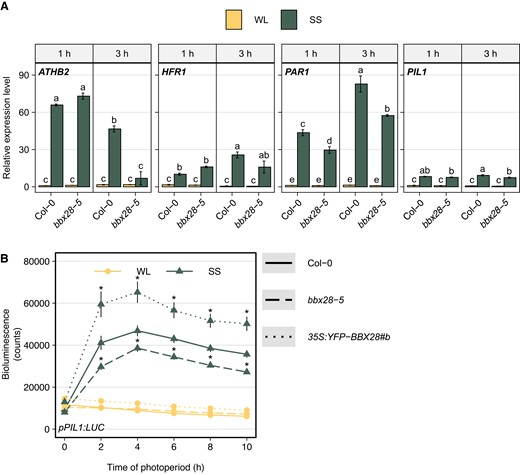
BBX28 promotes the expression of shade-responsive genes. A) Transcript levels of ATHB2, HFR1, PAR1, and PIL1 genes were determined in Col-0 and bbx28-5 seedlings grown in a short-day photoperiod (L10:D14) at 22 °C for 2 d and maintained in white light (WL) or transferred to simulated shade (SS) treatment for 1 h or 3 h. Expression levels are relative to UBQ10 and normalized to the gene expression of Col-0 in WL at 1 h. Data represent the mean of three biological replicates and standard deviation. Different letters indicate significantly different means obtained by three-way-ANOVA followed by the post-hoc Tukey's HSD (P < 0.05). B) Time course analysis of pPIL1:LUC activity in Col-0, bbx28-5, and 35S:YFP-BBX28#b seedlings grown in a short-day photoperiod (L10:D14) at 22 °C for 2 and maintained in white light (WL) or transferred to simulated shade (SS) treatment during 10 h. Measurements were taken every 2 h (n = 64). Asterisks indicate that the difference is statistically significant with Col-0 at the same time point and light treatment obtained by a three-way ANOVA followed by a post-hoc test (Bonferroni correction, P < 0.05).
BBX28 sustains the hypocotyl growth at the end of the shade photoperiod
To have a better understanding of BBX28 activity in the SAR, we studied the hypocotyl growth rate after the 10-h-photoperiod Under SS, the hypocotyl growth rate was significantly reduced in the two null mutant lines while the hypocotyl growth rate in both overexpressing lines was 2-folds higher than Col-0 seedlings (Supplementary Fig. S3). Then, we evaluated the hypocotyl growth rate at different time intervals when seedlings were exposed to WL and SS during the first day. We calculated the hypocotyl growth rate between 0 and 4 h, 4 and 7 h, 7, and 10 h and at night in Col-0, two mutants and two overexpressing lines of BBX28. Under WL, the growth rate was low and statistically similar between genotypes during the photoperiod, and gradually decreasing at night (Fig. 3). Under SS, the hypocotyl growth rate was significantly affected by BBX28. The bbx28-4 and bbx28-5 seedlings showed a similar hypocotyl growth rate than Col-0 between 0 and 7 h, but later, they showed a strong reduction of the growth at the end of the photoperiod (i.e. between 7 and 10 h, Fig. 3). In contrast, seedlings overexpressing BBX28 showed a higher hypocotyl growth rate than Col-0 at the beginning of the photoperiod, and then maintained a higher growth at the end of the photoperiod (Fig. 3). At night, the growth rate was similar between light conditions and genotypes (Fig. 3). These results support the idea that BBX28 is necessary to ensure the expression of the SAR at the end of the photoperiod.
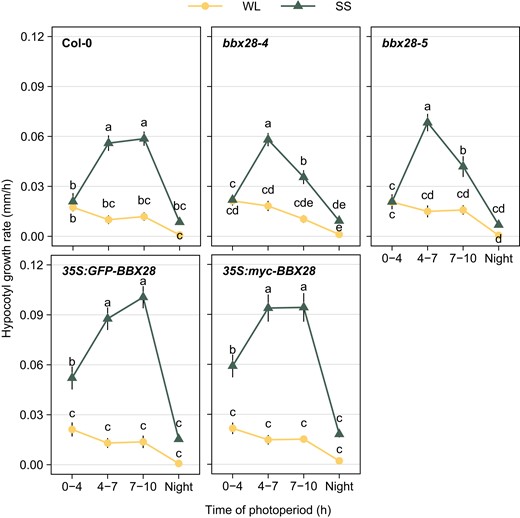
BBX28 sustains the growth rate to the end of the photoperiod under shade. Time course analysis of hypocotyl growth rate in Col-0, bbx28-4, bbx28-5, 35S:GFP-BBX28, and 35S:myc-BBX28 seedlings grown in a short-day photoperiod (L10:D14) at 22 °C for 2 d and maintained in white light (WL) or transferred to simulated shade (SS) treatment for 1 d. Measurements were taken at the beginning and at the end of each time interval between 0 and 4 h, 4 and 7 h, 7 and 10 h, and 10 and 24 h to calculate the hypocotyl growth rate (mm/h). Data represent mean (n > 32) and standard deviation. Different letters indicate significantly different means obtained by two-way-ANOVA followed by the post-hoc Tukey's HSD (P < 0.05).
We reasoned that the gene expression or the protein levels of BBX28 should be high at the end of the photoperiod to maintain the hypocotyl growth of seedlings under SS. To evaluate this hypothesis, we measured the gene expression of BBX28 at 2, 4, and 7 h of the first day in seedlings cultivated under WL and SS. Under WL, the BBX28 expression was low at 2 h and then increased at 4 h to decrease to the basal levels at 7 h, while the BBX28 expression is low and stable under SS (Supplementary Fig. S4). Then, we studied the time course of BBX28 protein stability in epidermal cells in the hypocotyls of 35S:GFP-BBX28 seedlings. The nuclear fluorescence intensity and the percentage of fluorescent nuclei of GFP-BBX28 were higher in seedlings exposed to SS compared to WL after 2 h, and the differences between light treatments increased after 6 h (Fig. 4, A to C). Consistently with these results, the immunoblots showed that the GFP-BBX28 protein abundance in seedlings grown under SS was significantly higher than WL after 6 h (Fig. 4D). Together, these results strongly suggest that BBX28 protein accumulates under prolonged shade to sustain the hypocotyl growth at the end of the photoperiod.
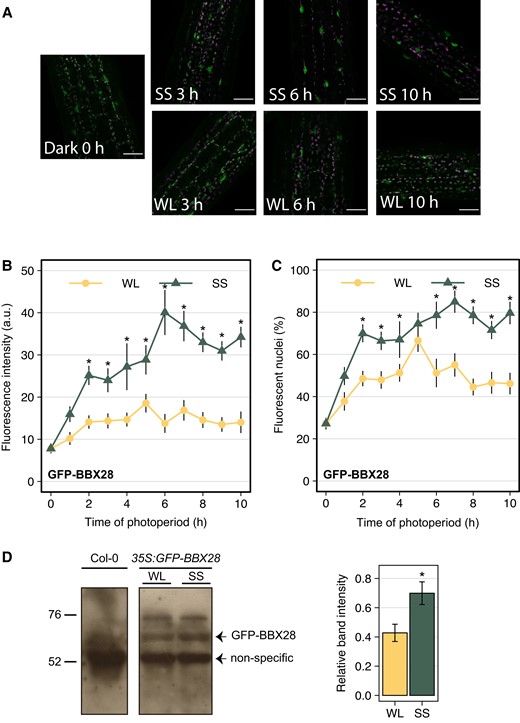
BBX28 protein accumulates in nuclei in response to shade. BBX28 protein abundance in 35S:GFP-BBX28 seedlings grown in a short-day photoperiod (L10:D14) at 22 °C for 2 d and maintained in white light (WL) or transferred to simulated shade (SS) for 10 h. A) Representative confocal images showing hypocotyl epidermal cells. The GFP signal is shown in green, and magenta represents the chlorophyll. Scale bars: 40 μm. B) Mean fluorescence intensity, and C) percentage of fluorescence nuclei. Data represent the mean and standard deviation. Measures were taken every hour of photoperiod (n = 9). Asterisks indicate that the difference is statistically significant compared to white light (WL) at the same time point by a two-way ANOVA followed by a post-hoc test (Bonferroni correction, P < 0.05). D) Immunoblot analysis of GFP-BBX28 in seedlings grown as indicated in (A) and kept under WL or SS during 6 h. The BBX28 band and the non-specific band, which was used as a loading control, are indicated. Position of the marker with known molecular weight are indicated on the left. The GFP-BBX28 protein levels are also showed as the intensity band relative to the loading control under WL and SS. Data represent the mean of four biological replicates and standard deviation. The asterisk indicates that the difference is statistically significant compared to the WL treatment by a one-way ANOVA (P < 0.05).
Transcriptome of BBX28-regulated gene expression under shade
Our previous results suggest that BBX28 transcription factor can have a higher impact on the expression of target genes under prolonged shade. Then, we performed a whole-transcriptome sequencing (RNA-seq) experiment with Col-0 and bbx28-5 seedlings exposed to WL or SS for 4 h. In Col-0 seedlings, we found that 3,608 genes were differentially expressed between WL and SS (Supplementary Data Set 1). The gene ontology (GO) analysis showed that the up-regulated genes under SS were enriched in processes related to auxin signaling, response to heat, response to light intensity, and response to BR (Supplementary Fig. S5 and Supplementary Data Set 2). Down-regulated genes under SS were associated with glycosinolate biosynthesis, phenylpropanoid biosynthesis, pigment biosynthesis, photosynthesis electron transport, and wounding response (Supplementary Fig. S5 and Supplementary Data Set 3). These results are in agreement with previous transcriptomes (Hornitschek et al. 2012; Kohnen et al. 2016; Pacín et al. 2016).
Then, we compared the genes differentially expressed between Col-0 and bbx28-5 under SS. We found 1,592 genes differentially expressed by BBX28 (Supplementary Fig. S6A). Among them, 399 genes were differentially expressed under WL, 1,283 genes were differentially expressed under shade, and 556 genes showed a significant Genotype × Light interaction (Supplementary Data Set 4 to 6, Supplementary Fig. S6B). The 1,592 genes regulated by BBX28 were grouped in six clusters (Fig. 5A, Supplementary Data Set 7). Cluster I grouped 743 genes promoted by BBX28, and they were related to cell wall growth (XTH17, EXPA1, CALS5, EXPA5, XTH20), auxin biosynthesis (YUC9, CYP79B2, CYP79B3), auxin transport (PIN5, PIN6), and cell cycle regulation (CYCA1; 1, CYCA3; 2, WEE1). Cluster II and IV grouped genes inhibited by BBX28. Cluster II contained 133 genes related to glucosinolate biosynthesis (CYP79F2, MAM1, IMD1) and cluster IV included 264 genes enriched in heat shock proteins (HSP90.1, HSP70, HSP17.6A, HOP3). The clusters III, V, and VI grouped genes associated with light and genotype interaction. Among them, we found genes related to auxin synthesis and signaling (SAV4, IAA6, SAUR71, ARF11, Supplementary Data Set 7). From those genes regulated by BBX28, we selected genes related to auxin signaling to confirm the gene expression by RT-qPCR analysis in our light conditions. In Col-0 seedlings, IAA6 was promoted, while ARF11 and PIN5 were significantly inhibited under SS (Fig. 5B). The expression of the 3 auxin-related genes was completely impaired in the bbx28-5 mutant in response to shade (Fig. 5B). These results demonstrate that BBX28 controls the gene expression of auxin signaling elements to enhance the hypocotyl growth under shade.
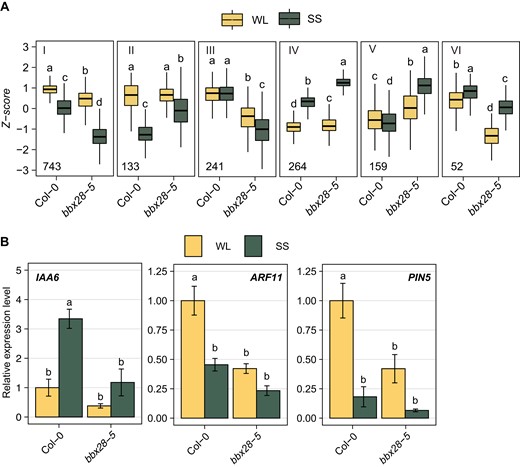
BBX28 regulated transcriptome under shade. A) RNA-Seq experiment performed in Col-0 and bbx28-5 seedlings grown in a short-day photoperiod (L10:D14) at 22 °C for 2 and maintained in white light (WL) or transferred to simulated shade (SS) for 4 h. Mean normalized expression data (Z-score) of genes for the 6 clusters that group BBX28 regulated genes depending on their expression pattern. The number of each cluster is indicated with a roman number. The boxplots show the median as a solid line, the hinges representing the 25th and 75th percentiles, and the whiskers that extend up to a maximum of 1.5 times the interquartile range from the hinges. Different letters indicate significant differences between means by two-way ANOVA and Tukey post-hoc test (P < 0.05). B)IAA6, ARF11, and PIN5 gene expression in Col-0 and bbx28-5 seedlings grown as in (A). Gene expression is relative to UBQ10 and normalized to the expression of Col-0 under WL. Data represent the mean of three biological replicates and standard deviation. Different letters indicate significant differences between means by two-way ANOVA and Tukey post-hoc test (P < 0.05).
BBX28 requires COP1 to promote hypocotyl growth under shade
It has been demonstrated that BBX28 interacts physically with COP1 during seedling skotomorphogenesis (Lin et al. 2018). We compared our BBX28 transcriptome with the previous COP1-regulated transcriptome expressed under SS (Pacín et al. 2016). We found a statistically significant overlap of 193 genes commonly regulated by BBX28 and COP1 (P = 3.35 × 10−4) (Fig. 6A and Supplementary Data Set 8). To know if BBX28 is involved in the COP1 signaling pathway induced by SAR, we studied the hypocotyl growth under WL and SS in Col-0, bbx28-5 cop1-4, and 35S:YFP-BBX28 cop1-6 mutants. As expected, the hypocotyl growth response to SS was strongly reduced or impaired in both cop1-4 and cop1-6 single mutants, respectively (Fig. 6B, Crocco et al. 2010; Gangappa et al. 2013). Interestingly, the enhanced SAR of 35S:YFP-BBX28 was strongly repressed in the cop1-6 background, while bbx28-5 cop1-4 seedlings did not show a statistically significant response to shade (Fig. 6B). These results strongly suggest that BBX28 is involved, at least partially, in the COP1 signaling to promote the SAR.
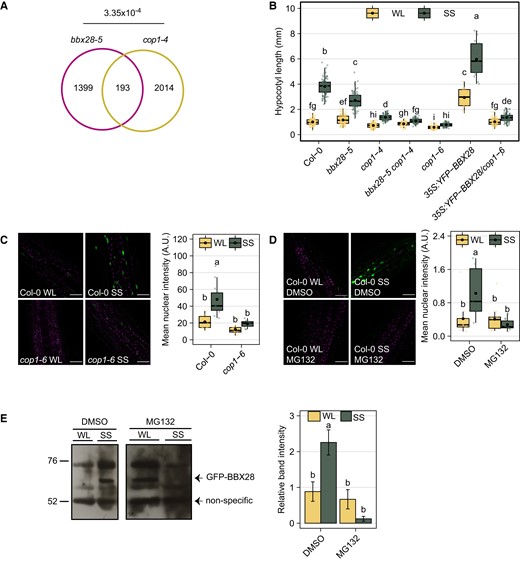
BBX28 requires COP1 to promote hypocotyl growth under shade. A) The Venn diagram shows co-regulated genes between BBX28 and COP1 under shade. The P-value of the intersection is indicated. B) Hypocotyl length of Col-0, bbx28-5, cop1-4, bbx28-5 cop1-4, cop1-6, 35S:YFP-BBX28 and 35S:YFP-BBX28/cop1-6 seedlings grown in a short-day photoperiod (L10:D14) at 22 °C for 2 d and maintained in white light (WL) or transferred to simulated shade (SS) treatment for 4 d. The boxplots show the median as a solid line and the mean as a black point, the hinges representing the 25th and 75th percentiles, and the whiskers that extend up to a maximum of 1.5 times the interquartile range from the hinges. Individual observations are represented by colored points (n > 50). Different letters indicate significantly different means obtained by two-way-ANOVA followed by the post-hoc Tukey's HSD (P < 0.05). C) Nuclear fluorescence in hypocotyl cells of YFP-BBX28 in Col-0 and cop1-6 seedlings grown in white light (WL) or simulated shade (SS) for 6 h. D) Nuclear fluorescence in hypocotyl cells of YFP-BBX28 in Col-0 seedlings grown in white light (WL) or simulated shade (SS) with DMSO or MG132 (100 μM) for 6 h. C and D) On the left, representative confocal images showing hypocotyl epidermal cells. The YFP signal is shown in green, and magenta represents the chlorophyll. Scale bars: 40 μm. On the right, mean fluorescence intensity. The boxplots show the median as a solid line and the mean as a black point, the hinges representing the 25th and 75th percentiles, and the whiskers that extend up to a maximum of 1.5 times the interquartile range from the hinges. Individual observations are represented by colored points (n = 12). Different letters indicate significantly different means obtained by two-way-ANOVA followed by the post-hoc Tukey's HSD (P < 0.05). E) Immunoblot analysis of GFP-BBX28 in seedlings grown as in (D). The BBX28 protein and the non-specific band used as a loading control are indicated. The position of the marker with known molecular weight are indicated on the left. The GFP-BBX28 protein levels are shown as the intensity band relative to the loading control under WL and SS. Data represents the mean of three biological replicates and the standard deviation. Different letters indicate a significant difference between means by two-way ANOVA followed by the post-hoc Tukey's HSD (P < 0.05).
COP1 and BBX28 accumulate in the nuclei to promote hypocotyl growth under SS (Fig. 4, Pacín et al. 2013). We hypothesized that COP1 contributes to the accumulation of BBX28 at the end of the photoperiod under SS. To test this hypothesis, we quantified the nuclear fluorescence intensity of YFP-BBX28 in Col-0 and cop1-6 seedlings grown under WL and SS for 6 h. We found that the response to SS in fluorescence levels in Col-0 was abolished in cop1-6 seedlings (Fig. 6C). To confirm if the BBX28 stability is dependent on the activity of COP1 under SS, we quantified the fluorescence intensity of the fusion protein YFP-BBX28 in the presence or absence of MG132, which is a potent inhibitor of the 26S proteasome. As expected, the MG132 completely abolished the increase of YFP-BBX28 nuclear fluorescence in Col-0 seedlings under SS (Fig. 6D). In agreement with the later results, the immunoblot analysis showed that GFP-BBX28 protein abundance in the presence of MG132 was reduced significantly when seedlings were exposed to SS (Fig. 6E). These results strongly support that BBX28 protein is stabilized by COP1 under prolonged SS.
Discussion
The COP1-HY5 is a central regulatory hub in light signaling, and BBX proteins are cofactors for the fine-tuning of seedling photomorphogenesis (Gangappa and Botto 2014; Yadav et al. 2020). Here, we demonstrated that BBX28 operates in another light signaling pathway previously ignored. We found that (i) BBX28 induces the hypocotyl length of seedlings grown under shade by the perception of R:FR changes (Fig. 1), (ii) BBX28 has a stronger contribution in sustaining the hypocotyl growth response under prolonged shade (Fig. 3), (iii) BBX28 promotes the SAR-induced gene expression including auxin signaling components principally under prolonged shade (Figs. 2 and 5), (iv) BBX28 protein stability increases at the end of the photoperiod of shade (Fig. 4) and requires the activity of COP1 (Fig. 6).
Under shade, phyB perceives the low R:FR ratios, while cryptochrome 1 is responsible for the perception of the reduction of PAR and B photons (Keller et al. 2011; Pedmale et al. 2016). We found that BBX28 is involved in response to reduced R photons, but not in the reduction of the PAR and B photons mediated by cryptochrome 1 under shade (Fig. 1). This observation contrasts with the function of BBX28 in the phytochrome and cryptochrome signaling pathways in seedling photomorphogenesis (Lin et al. 2018). Furthermore, BBX28 has a strong contribution in maintaining the hypocotyl growth at the end of the photoperiod of shade (Fig. 3), sustained by the promotion of gene expression in prolonged shade (Figs. 2 and 5). Low R:FR ratios typical of shade environments trigger rapid elongation hypocotyl growth (Kohnen et al. 2016), but additional mechanisms are required to maintain the growth for the fine-tuning of the SAR (Ciolfi et al. 2013; Pucciariello et al. 2018). These findings add information on the functional dynamics of BBX proteins for the fine-tuning regulation of growth plant responses under shade.
We found that the BBX28 function is dependent on COP1 to fully express the SAR. COP1 is required to stabilize BBX28 protein at the end of the photoperiod of shade (Figs. 4 and 6), and the genetic analysis of bbx28 and cop1 mutants showed that they act redundantly in the same signaling pathway (Fig. 6, A and B). Furthermore, BBX28 accumulation in the nuclei requires the 26S proteasome activity of COP1 for its stabilization to sustain the growth under shade (Fig. 6C to E). These results are in agreement with previous reports showing that other BBX members of the structural group IV are involved in the COP1 signaling of the SAR (Crocco et al. 2010; Gangappa et al. 2013). BBX21 and BBX22 inhibit, while BBX24 and BBX25 promote the SAR. Interestingly, the bbx21 bbx22 double mutant restores the SAR in the background of cop1 (Crocco et al. 2010), while BBX24 and BBX25 are completely epistatic to COP1 under shade (Gangappa et al. 2013). In the SAR, COP1 targets negative transcriptional regulators for degradation, such as HFR1 (Pacín et al. 2016), DELLA proteins (Blanco-Touriñán et al. 2020), and BBX21 and BBX22 proteins (Crocco et al 2010); while stabilizes positive regulators such as PIF4 and PIF5 (Pham et al. 2018), and BES1 (Costigliolo Rojas et al 2022). The results of our work with cop1-6 mutant and MG132 inhibitor of COP1 activity suggest that the COP1 stabilizes BBX28 under shade probably by the COP1-mediated degradation of negative regulators of the SAR through the 26S proteosome (Fig. 6C to E). Further investigations are needed to elucidate additional molecular mechanisms of BBX transcription factors in COP1 signaling under shade.
COP1 and BBX28 signaling showed a significant convergence at the transcriptional level (Fig. 5). BBX28 regulates gene expression of well-known direct targets of PIF proteins under shade (Fig. 2). In addition, BBX28 strongly controls the gene expression of auxin signaling elements including SAV4, YUC9, PIN5, PIN6, IAA6, and ARF11 under shade (Fig. 5, Supplementary Table S5). Consistently, the up-regulation of expression of biosynthesis, transport, and signaling of auxin-related genes requires the contribution of COP1 for the stability of PIF under shade (Hornitschek et al. 2012; Pacín et al. 2016). The genome-wide scale transcriptome analysis also shows that the bbx28-5 mutant showed lower expression of genes related to cell wall modification than Col-0 (e. g. XTH, AGP, and EXP gene families, Supplemental Datasets 5 to 6). These results agree with previous reports showing that the auxin signaling dependent of PIF under shade involves the transcriptional regulation of XTH and AGP family members (Hornitschek et al. 2012; Kohnen et al. 2016).
The manipulation of the BBX28 activity in plant tissues would be a profitable strategy to generate desirable agronomic traits in crops. The sub-expression of BBX28 may have positive effects reducing the elongation of vegetative structures at high plant densities through the auxin signaling pathway in prolonged shade. Changes in the plant architecture by shortness of petioles and stems would increase the translocation of photosynthetic resources to leaves and grains (Carriedo et al. 2016). This is true for other BBX members overexpressed in crops. For example, the ectopic expression of Arabidopsis BBX21 in transgenic potatoes resulted in increased photosynthetic rates and higher tuber yields without penalty in the water use efficiency (Crocco et al. 2018; Gómez-Ocampo et al. 2021), while the overexpression of BBX32 in soybean significantly increased the grain yield by extending the reproductive for the grain fill (Preuss et al. 2012). The biotechnological manipulation of the expression of BBX28 together with other BBX members of the same structure group, such as BBX29, by CRISPR/CAS9 genome editing could be an opportunity to stack higher order of mutations to gain insight into the complex functional picture of these transcription factors in crops.
Materials and methods
Plant material
All the experiments were carried out with Arabidopsis (A. thaliana (L.) Heynh.) Columbia (Col-0). bbx28-4, 35S:YFP-BBX28, 35S:myc-BBX28, and 35S:YFP-BBX28/cop1-6 (Lin et al. 2018), cop1-4 and cop1-6 (McNells et al. 1994), pPIL1:LUC (Li et al. 2014) genotypes were previously described. The T-DNA insertion mutant bbx28-5 (SAIL_412_A09) was obtained from the Arabidopsis Biological Resource Center (ABRC), and the homozygous lines were identified by PCR using the primers listed in Supplementary Table S1. To confirm the bbx28-5 mutant line (SAIL_412_A09), cDNA was obtained from 7-d-old seedlings from Col-0 and bbx28-5. Full-length BBX28 CDS was amplified with specific primers by PCR and the ribosomal protein L14B gene (RPL14B, AT4G27090) was used as a loading control (Supplementary Table S1). The bbx28-5 cop1-4 double mutant, and the bbx28-5 mutant bearing pPIL1:LUC, were obtained in this work by simple crossing, and the materials selected by PCR using the primers listed in Supplementary Table S1.
To obtain 35S:GFP-BBX28 and 35S:YFP-BBX28(#b)/pPIL1:LUC transgenic lines, the pTOPO·BBX28 plasmid harboring BBX28 CDS sequence was recombined by LR reaction with pB7WGF2 (Karimi et al. 2002) or pEarleyGate104 (Earley et al. 2006) to generate GFP-BBX28 or YFP-BBX28, respectively. Agrobacterium tumefaciens GV3101 cells were transformed with the corresponding construct and introduced into Col-0 or pPIL1:LUC plants via the floral dip method. Transgenic plants were selected on MS medium containing 15 mg/L Basta (T0 plants). Plants of the next generation (T1 plants) were selected in the same conditions and only the lines with a 3:1 resistance ratio were kept ensuring a unique copy of the transgene. Homozygous plants of the T3 or T4 generation were used for the experiments.
Growth conditions and light treatments
Seeds were sown in clear plastic boxes on 0.8% agar/water (w/v) and incubated in darkness at 4°C. After 4 d, imbibed seeds were exposed to a white light pulse for 5 h, and then kept in darkness for 14 h at 22°C to induce homogenous germination. Then, the boxes were transferred to white light with a short-day photoperiod of 10 h light:14 h of dark (L10:D14) at 22°C for 2 d to ensure the complete de-etiolation. Then, the boxes with de-etiolated seedlings were transferred to different light conditions with L10:D14 photoperiod at 22°C for the time indicated in each experiment. The white light (WL) treatment consisted of a mixture of fluorescent and incandescent lamps (PPFD = 90 μmol m−2 s−1, R:FR:B ratio = 1.1:1:1, R-660 nm = 9.13 μmol m−2 s−1, FR-730 nm = 8.3 μmol m−2 s−1, B-470 nm = 8.1 μmol m−2 s−1). The simulated shade (SS) treatment was obtained with a green acetate filter (#089; LEE Filters, http://www.leefilters.com) located above the boxes with seedlings to reduce the PPFD and filter R and B photons (PPFD = 20 μmol m−2 s−1, R:FR:B ratio = 0.14:1:0.23, R = 0.8 μmol m−2 s−1, FR = 5.5 μmol m−2 s−1, B = 1.3 μmol m−2 s−1). We also used different filters to reduce (i) the PPFD (20 µmol m−2 s−1 and R:FR:B ratio = 1:1:1, R = 3 μmol m−2 s−1, FR = 2.8 μmol m−2 s−1, B = 2.8 μmol m−2 s−1), (ii) the PPFD and the R photons (PPFD = 20 µmol m−2 s−1 and R:FR:B ratio = 0.5:1:1, R = 1 μmol m−2 s−1, FR = 2 μmol m−2 s−1, B = 2 μmol m−2 s−1) and (iii) the PPFD and the B photons (PPFD = 20 µmol m−2 s−1 and R:FR:B ratio = 1:1:0.4, R = 5 μmol m−2 s−1, FR = 5 μmol m−2 s−1, B = 0.2 μmol m−2 s−1). The light treatments were provided by WL source in combination with a white sheet of paper to reduce the PAR, with a blue acetate filter (#363; LEE Filters) to reduce the R photons, and a red acetate filter (#106; LEE Filters) to reduce the B photons.
During the experiment under different light treatments, the boxes with seedlings were placed vertically and then photographed with a digital camera (PowerShot; Canon, http://www.canon.com). To measure the cotyledon area, seedlings were arranged manually on transparent tape so that the cotyledons would stick together on it and fixed on a sheet of white paper and scanned (EPSON, https://www.epson.es). Hypocotyl length and cotyledon area were determined using the Fiji distribution of ImageJ (Schindelin et al. 2012; Schneider et al. 2012).
The growth rate was measured during the first day of WL and SS treatments. Measurements were taken at the beginning and at the end of different time intervals (0 to 3 h, 3 to 7 h, 7 to 10 h, 10 to 24 h). The difference in the hypocotyl length between the end and the beginning of each interval was estimated and divided by the hours of the interval to obtain the hypocotyl growth rate of each time interval (mm/h).
For the MG132 treatment, seeds were sown on a paper filter above the 0.8% agar/water medium in a 96-well plate. At the beginning of the light treatments, the filter papers with seedlings were transferred carefully to 0.8% agar/water medium supplemented with DMSO or MG132 (100 μM) and kept in this medium until the end of the experiment.
Luciferase activity
For luminescence assays, the same experimental procedure as indicated above was followed with some changes. Seeds were sown on 0.8% agar/water medium in a 96-well plate. At the beginning of the previous night before the starting of WL and SS treatments, 20 μl of a luciferin solution (0.5 mM) was added to each well. Luciferase activity was measured with the LB 960 Microplate Luminometer Center (Berthold, https://www.berthold.com). The luminescence signal was recorded every 2 h as counts per second, for three successive seconds per well.
RNA-Seq and comparative transcriptome analysis
Seedling samples for RNA-seq analysis were harvested after 4 h of the beginning of WL and SS on the first day of light treatment. Total RNA from three independent biological samples was extracted using the Spectrum Plant Total RNA Kit (Sigma-Aldrich, https://www.sigmaaldrich.com). The preparation of libraries and their sequencing was carried out in the Genomics Unit of the Center for Genomic Regulation (CRG, Barcelona). Single-end libraries were prepared using the TruSeq mRNA Sample Prep Kit (Illumina) and sequenced on the HiSeq 2500 platform (Illumina) to obtain 50 pb length reads. Data quality was assessed with FastQC. Reads were aligned to the A. thaliana TAIR10 (v. 45) genome with HISAT (Kim et al. 2015) and sorted with SAM tools (Li et al. 2009). Counts per gene were obtained with HTseq (Anders et al. 2015). Only these genes with CPM > 0.8 in at least 3 samples were retained for further analysis. Count genes were normalized to library length with the limma package in R (Ritchie et al. 2015). Differential gene expression was estimated using the EdgeR package in R (Robinson et al. 2009). Genes with absolute log2FC > 1.2 and q-value < 0.05 were considered as differentially expressed genes. Genes selected as regulated by BBX28 were hierarchically clustered based on the Euclidean distance of Z-score expression values across all the samples using the pheatmap package in R (Kolde 2019).
COP1-regulated genes were selected and reanalyzed from previously published transcriptomes (Pacín et al. 2016). Normalized expression data were obtained from Pacín et al. (2016). Genes differentially expressed were determined using the limma package in R (Ritchie et al. 2015). In all cases, genes regulated by a genotype were determined as genes differentially expressed in genotype and the interaction genotype:treatment (q-value < 0.05). The statistical significance of the overlap between groups was calculated at http://nemates.org/MA/progs/overlap_stats.html.
Gene ontology (GO) category terms were assigned using the BioMaps tool of Virtualplant3.1 software (Katari et al. 2010). The adjusted P-value associated with each term corresponds to the P-value obtained for a Fisher's test corrected for multiple contrasts. The enrichment factor was calculated as the ratio between the proportion of genes associated with a GO term within the group of interest and the proportion of genes expected for that term in the entire set of genes.
Confocal microscopy
Confocal microscopy images were acquired with an LSM 880 microscope (Zeiss, http://www.zeiss.com) with a Plan-Apochromat objective lens (20×/0.8 NA). For the detection of the YFP-BBX28 fusion protein, probes were excited with a 514 nm laser (0.75 Airy units), and the signal was detected between 522 and 598 nm. For the GFP-BBX28 fusion protein, the molecule was excited with a 488 nm laser (1 Airy unit), and the signal was detected between 500 and 561 nm. The chlorophyll signal was obtained with excitation at 543 nm and detection between 639 and 736 nm. For fluorescence intensity measurement, fluorescent nuclei were identified as regions of interest (ROIs), and the fluorescence intensity of each nucleus was quantified using ImageJ's Fiji software. The average nuclear fluorescence intensity was obtained and multiplied by the number of nuclei, obtaining the total fluorescence intensity. For each image, the area of all cells was obtained, and the number of cells was estimated by dividing the total area of the hypocotyl section by the average cell area. Fluorescence intensity (arbitrary units) was calculated as the total fluorescence intensity divided by the number of cells. The percentage of fluorescent nuclei was obtained by dividing the number of fluorescent nuclei by the estimated number of cells in the hypocotyl section.
RT-qPCR analysis
Total RNA from A. thaliana seedlings was extracted using the Spectrum Plant Total RNA Kit (Sigma-Aldrich, https://www.sigmaaldrich.com) according to the manufacturer's instructions. Plant tissue was previously crushed with a mortar and liquid nitrogen. For the elimination of residual genomic DNA, the purified total RNA was treated with DNaseI following the protocol of the DNase kit (Promega, https://worldwide.promega.com/). cDNA synthesis was performed starting with 2 μg of total RNA and following the protocol of the M-MLV Reverse Transcriptase kit (Promega) using oligo dT as a primer for the reverse transcription reaction. In all cases, 50 ng of cDNA was used and amplified with FastStart Universal SYBR Green Master (Roche, http://www.roche.com) in a 7500 Fast-Real Time qPCR thermal cycler (Applied Biosystems). The sequence of the primers used was designed using the Primer3Plus software or previously published sequences were used (Supplementary Table S1). The UBQ10 gene was used as a loading control. The 7500 Software v2.0.6 program (Applied Biosystems) was used to analyze the data. Relative mRNA levels were determined by the 2(-Delta Delta C(T)) method (Livak and Schmittgen 2001).
For the quantification of known shade-induced genes, the cDNA was obtained from seedlings after 1 and 3 h of the beginning of WL or SS on the first day. For quantification of the expression of selected BBX28 target genes from the RNA-seq assay, the cDNA was obtained from seedlings after 4 h in WL or SS on the first day. For quantification of the time-course BBX28 expression, the cDNA of Col-0 seedlings was extracted after 2, 4, and 7 h in WL or SS on the first day.
Immunoblots assays
Seedlings were harvested after 6 in WL or SS on the first day. Samples were introduced in liquid nitrogen and ground into a mortar. Extraction was performed with a denaturing buffer (50 nM Tris/HCL pH 7.5, 100 nM EDTA, 1 mM DTT, 0.1% Triton X100, and protease inhibitor cocktail). Then, the samples were centrifuged for 20 min at 10,000 g at 4°C and the supernatant was saved. Proteins were separated on a 12% SDS-polyacrylamide gel and transferred to a nitrocellulose membrane. The membrane was incubated with the corresponding antibody and the bands were visualized with an ECL chemiluminescence kit (Amersham Biosciences), according to the manufacturer’s protocol.
Accession numbers
Sequence data from this article can be found in the GenBank/EMBL data libraries under accession numbers GSE217723. The records are accessible at: https://www.ncbi.nlm.nih.gov/geo/query/acc.cgi? acc=GSE217723.
BBX28: AT4G27310, COP1: AT2G32950, ATHB2: AT4G16780, HFR1: AT1G02340, PAR1: AT2G42870, PIL1: AT2G46970, IAA6: AT1G52830, ARF11: AT1G19220, PIN5: AT5G16530.
Acknowledgments
We thank Marcos Castellanos from the Nottingham Arabidopsis Stock Centre (NASC) for the provision of seeds. We also thank José Pruneda-Paz for the plasmid pTOPO·BBX28 containing the CDS of the BBX28 gene and Dongqing Xu for providing seeds of bbx28-4, 35S:myc-BBX28, 35S:YFP-BBX28, and 35S:YFP-BBX28/cop1-6. We also thank Jorge Casal for his comments on the manuscript, and Roberto Tornese for his technical assistance in the adjustment of light chambers for the experiments.
Author contributions
MSS and JFB conceived and designed the experiments; MSS performed most of the experiments. GGO, MEP, and CEB performed the protein blot experiments. MSS, GGO, MEP, AHR, and JPC performed the confocal microscopy imaging. MSS, GGO, and MEP analyzed the data and performed the statistical analysis. JFB and MSS wrote the manuscript. All the authors read and accepted the manuscript.
Supplementary data
The following materials are available in the online version of this article.
Supplementary Figure S1. Characterization of bbx28-5 mutant line and 35S:GFP-BBX28 transgenic line.
Supplementary Figure S2. BBX28 function in cotyledon expansion under shade.
Supplementary Figure S3. BBX28 promotes hypocotyl growth rate during the first day of shade.
Supplementary Figure S4.BBX28 gene expression is not induced in response to shade.
Supplementary Figure S5. GO enrichment for shade-regulated genes in Col-0.
Supplementary Figure S6. BBX28-regulated genes under white light (WL) and simulated shade (SS).
Supplementary Table S1. Primers used in this study.
Supplementary Data Set 1. List of genes regulated by simulated shade (SS) in Col-0 seedlings.
Supplementary Data Set 2. GO enrichment of genes up-regulated by simulated shade (SS) in Col-0 seedlings (FDR < 0.05).
Supplementary Data Set 3. GO enrichment of genes down-regulated by simulated shade (SS) in Col-0 seedlings (FDR < 0.05).
Supplementary Data Set 4. List of genes regulated by BBX28 in white light (WL).
Supplementary Data Set 5. List of genes regulated by BBX28 in the simulated shade (SS).
Supplementary Data Set 6. List of genes that show an expression dependent on genotype/light interaction.
Supplementary Data Set 7. List of genes regulated by BBX28 by cluster.
Supplementary Data Set 8. List of genes regulated by BBX28 and COP1.
Funding
This work was supported by grants from the University of Buenos Aires (UBACYT 20020170100265BA, PIDAE2020), and the Agencia Nacional de Promoción Científica y Tecnológica of Argentina (PICT2017-0583 and PICT2019-2807) to JFB.
Data availability
The raw transcriptomic data are deposited in NCBI GEO and can be found under accession number GSE217723.
Dive Curated Terms
The following phenotypic, genotypic, and functional terms are of significance to the work described in this paper:
References
Author notes
To whom correspondence shall be addressed.
The author responsible for the distribution of materials integral to the findings presented in this article in accordance with the policy described in the Instructions for Authors (https://dbpia.nl.go.kr/plphys/pages/General-Instructions) is JFB.
Conflict of interest statement None declared.