-
PDF
- Split View
-
Views
-
Cite
Cite
Dongjie Jia, Yuchen Li, Kun Jia, Benchang Huang, Qingyuan Dang, Huimin Wang, Xinyuan Wang, Chunyu Li, Yugang Zhang, Jiyun Nie, Yongbing Yuan, Abscisic acid activates transcription factor module MdABI5–MdMYBS1 during carotenoid-derived apple fruit coloration, Plant Physiology, Volume 195, Issue 3, July 2024, Pages 2053–2072, https://doi.org/10.1093/plphys/kiae188
- Share Icon Share
Abstract
Carotenoids are major pigments contributing to fruit coloration. We previously reported that the apple (Malus domestica Borkh.) mutant fruits of “Beni Shogun” and “Yanfu 3” show a marked difference in fruit coloration. However, the regulatory mechanism underlying this phenomenon remains unclear. In this study, we determined that carotenoid is the main factor influencing fruit flesh color. We identified an R1-type MYB transcription factor (TF), MdMYBS1, which was found to be highly associated with carotenoids and abscisic acid (ABA) contents of apple fruits. Overexpression of MdMYBS1 promoted, and silencing of MdMYBS1 repressed, β-branch carotenoids synthesis and ABA accumulation. MdMYBS1 regulates carotenoid biosynthesis by directly activating the major carotenoid biosynthetic genes encoding phytoene synthase (MdPSY2-1) and lycopene β-cyclase (MdLCYb). 9-cis-epoxycarotenoid dioxygenase 1 (MdNCED1) contributes to ABA biosynthesis, and MdMYBS1 enhances endogenous ABA accumulation by activating the MdNCED1 promoter. In addition, the basic leucine zipper domain TF ABSCISIC ACID-INSENSITIVE5 (MdABI5) was identified as an upstream activator of MdMYBS1, which promotes carotenoid and ABA accumulation. Furthermore, ABA promotes carotenoid biosynthesis and enhances MdMYBS1 and MdABI5 promoter activities. Our findings demonstrate that the MdABI5–MdMYBS1 cascade activated by ABA regulates carotenoid-derived fruit coloration and ABA accumulation in apple, providing avenues in breeding and planting for improvement of fruit coloration and quality.
Introduction
Carotenoids are major pigments contributing to the yellow, orange, red, and other coloration of horticultural fruits and vegetables (Yuan et al. 2015). In plants, carotenoids play vital roles in plant growth and development, and biotic interactions such as photoprotections (Domonkos et al. 2013; Sandmann 2021), precursors of abscisic acid (ABA), strigolactones and bioactive apocarotenoids (Ramel et al. 2012; Moreno et al. 2021). Furthermore, carotenoids are antioxidants that benefit human health (Fiedor and Burda 2014). Therefore, as our emphasis on quality of life has increased, so has the need for cultivating new plant varieties rich in carotenoids via plant genetic engineering.
Carotenoids are isoprenoid compounds synthesized by various metabolic enzymes (Sun et al. 2018). The diversity of carotenoid pigments is influenced by carotenoid biosynthetic genes. Multiple phytoene synthases influence carotenoid content in several species such as tomato (Solanum lycopersicum) (Fraser et al. 2002), maize (Zea mays) (Li et al. 2008), Arabidopsis thaliana (Rodriguez-Villalon et al. 2009), rice (Oryza sativa) (Bai et al. 2016), and pepper (Capsicum spp.) (Jang et al. 2020; Jeong et al. 2022). Overexpressing MdPSY2-1 and MdPSY1 increases carotenoid contents in apple (Malus domestica Borkh.) (Dang et al. 2021; Ampomah-Dwamena et al. 2022). Moreover, downregulating lycopene β-cyclase (ClLCYB) contributes to lycopene accumulation and red flesh coloration in watermelon (Citrullus lanatus) (Zhang et al. 2020).
The MYB transcription factor (TF) superfamily is grouped into 4 subfamilies including R1/R2-MYB, R2R3-MYB, R1R2R3-MYB, and R1R2R3R1/R2-MYB depending on the number of MYB repeats. MYB TFs play vital roles in growth, development, phytohormone synthesis, and stress in diverse plant species (Dubos et al. 2010; Wu et al. 2022). Several MYB TFs modulate carotenoid biosynthesis. The reduced carotenoid pigmentation 1 as an R2R3-MYB positively modulates carotenoid biosynthesis in Mimulus lewisii flowers (Sagawa et al. 2016). WHITE PETAL1 as an R2R3-MYB enhances carotenoid production by promoting lycopene Ɛ-cyclase (MtLYCe) and lycopene β-cyclase (MtLYCb) expression in Medicago truncatula flowers (Meng et al. 2019). SlMYB72 and SlMYB117 regulate carotenoid biosynthesis in tomato fruits by modulating carotenogenic genes (Wu et al. 2020; Tyagi et al. 2022). CrMYB68 inhibits α- and β-branch carotenoid biosynthesis via repressing β-carotene hydroxylases 2 (CrBCH2) and 9-cis-epoxycarotenoid dioxygenase 5 (CrNCED5) expression in citrus (Zhu et al. 2017). By contrast, AdMYB7 promotes carotenoid production by directly enhancing AdLCY-β expression in kiwifruit (Actinidia deliciosa) (Ampomah-Dwamena et al. 2019). Therefore, MYB subfamilies have multiple roles in regulating carotenoid biosynthesis in diverse plant species.
ABA, which is produced by 9-cis-epoxycarotenoid dioxygenase (NCED) cyclizing of violaxanthin or neoxanthin, is an important phytohormone that plays key roles in plant growth and development (Chen et al. 2020; Kavi Kishor et al. 2022). The enzyme NCED is the vital rate-limiting step for ABA biosynthesis (Frey et al. 2012; Lang et al. 2021). Silencing SlNCED1 reduces ABA levels and increases levels of lycopene and β-carotene in tomato fruits (Sun et al. 2012a, 2012b). Overexpressing PpNCED1 and PpNCED5 enhances ABA levels in peach (Prunus persica L. Batsch) calli (Wang et al. 2021). Several TFs participate in ABA-mediated carotenoid biosynthesis. ABA-DEFICIENT4 (ABA4) controls the accumulation of 9-cis-violaxanthin in Arabidopsis (Perreau et al. 2020). However, little is known about ABA-regulated carotenoid biosynthesis in apple fruit.
Fruit flesh color is mainly determined by amounts of carotenoids (Ampomah-Dwamena et al. 2012; Dang et al. 2021). Little is known about the regulatory network of carotenoid biosynthesis and accumulation in apple fruit. We previously reported that there was a marked difference in total carotenoid content of apple mature fruits between “Beni Shogun” and “Yanfu 3” (Dang et al. 2021). Here, we identify an R1-type MdMYBS1 that promotes carotenoid accumulation and fruit coloration by directly activating MdPSY2-1 and MdLCYb expression. Furthermore, MdMYBS1 activates MdNCED1 expression to enhance ABA accumulation, and ABA induces MdMYBS1 transcription via a basic leucine zipper TF ABSCISIC ACID-INSENSITIVE5 (MdABI5). Our findings thus provide insights into the regulatory mechanism of the “MdABI5–MdMYBS1” module underlying carotenoid biosynthesis and new opportunities for genetic improvement of fruit quality and coloration.
Results
Carotenoid content in fruits showing different coloration
We previously reported that total carotenoid content of ripening fruit flesh was markedly higher in “Beni Shogun” than in “Yanfu 3” (Dang et al. 2021). The flesh of ripening “Beni Shogun” fruit was orange, whereas that of “Yanfu 3” was yellow (Fig. 1A). Carotenoids and flavonoids play vital roles in fruit flesh color (Dang et al. 2021; Han et al. 2022). The total carotenoid content in fruit flesh significantly differed between “Beni Shogun” and “Yanfu 3” during fruit development at 30 and 0 days before fruit ripening (DBFR) but total flavonoid content did not (Fig. 1A and Supplementary Fig. S1), indicating that carotenoid is the main factor influencing flesh color of apple fruit.
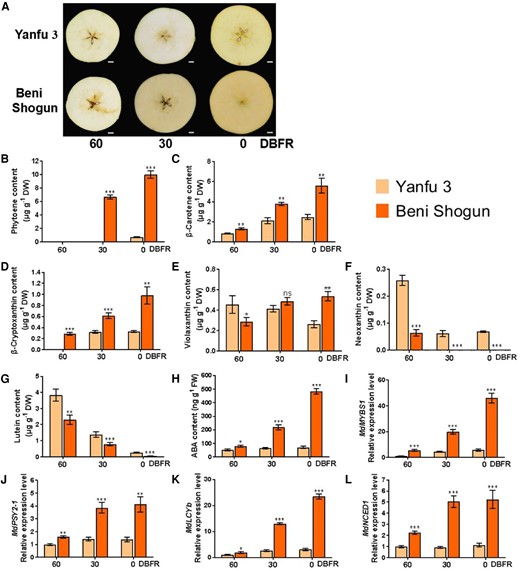
Identification of carotenoid compounds and carotenoid-related regulators in “Yanfu 3” and “Beni Shogun” fruits. A) Phenotypes of “Yanfu 3” and “Beni Shogun” fruits harvested at 60, 30, and 0 d before fruit ripening (DBFR). Scale bars, 1 cm. Fruit images were digitally extracted for comparison. B to H), Levels of the carotenoid compounds phytoene B), β-carotene C), β-cryptoxanthin D), violaxanthin E), neoxanthin F), and lutein G), and of ABA H) were measured using LC–MS/MS. I to L), Relative expression levels of MdMYBS1I), MdPSY2-1J), MdLCYbK), and MdNCED1L) were determined using RT-qPCR. At each harvesting time, 18 fruits were divided into 3 sets, each containing 6 fruits, and each set was considered to be 1 biological replicate. The data bars represent mean ± standard deviation. The error bars represent standard deviations of 3 independent biological replicates, and 6 fruits measured for each replicate. Asterisks indicate significant differences analyzed by Student's t-test (*P < 0.05, **P < 0.01, ***P < 0.001); ns, not significant.
We detected 6 carotenoid compounds including phytoene, β-carotene, β-cryptoxanthin, violaxanthin, neoxanthin, and lutein in apple fruit flesh using LC–MS/MS (Supplementary Fig. S2A). However, zeaxanthin, antheraxanthin, α-carotene, and α-cryptoxanthin were not detected (Supplementary Fig. S3). The major carotenoids in fruit flesh of “Beni Shogun” were phytoene and β-carotene, and of “Yanfu 3” were β-carotene and β-cryptoxanthin (Fig. 1B to G). Phytoene level was high in “Beni Shogun” fruit flesh at 30 and 0 DBFR, but this compound was not detected in “Yanfu 3” fruit flesh at 60 or 30 DBFR or in “Beni Shogun” fruit flesh at 60 DBFR (Fig. 1B). Levels of β-carotene (Fig. 1C) and β-cryptoxanthin (Fig. 1D) were markedly higher in “Beni Shogun” than in “Yanfu 3” at 60, 30, and 0 DBFR, with differences of 2- to 4-fold. Neoxanthin (Fig. 1F) and lutein (Fig. 1G) contents were much lower in “Beni Shogun” than in “Yanfu 3” during fruit ripening, whereas violaxanthin level was lower at 60 DBFR and higher at 0 DBFR in “Beni Shogun” than in “Yanfu 3” (Fig. 1E).
In addition, because ABA is derived from the cleavage of carotenoid precursors, we examined endogenous ABA content using LC–MS/MS (Supplementary Fig. S2B). ABA level was significantly (3- to 6-fold) higher in “Beni Shogun” than in “Yanfu 3” fruit flesh at 60, 30, and 0 DBFR (Fig. 1H).
MdMYBS1 is a candidate regulator of carotenoid and ABA accumulation
To identify the key regulators of carotenoid biosynthesis and ABA accumulation in apple fruit, 18 RNA sequencing (RNA-seq) libraries were sequenced from the flesh of “Beni Shogun” and “Yanfu 3” fruits at 60, 30, and 0 DBFR (Supplementary Fig. S4A to C). Differentially expressed genes (DEGs) were defined with false discovery rate < 0.05, and expression variation ratio > 2.0. There were 2,569 total DEGs, 446 upregulated DEGs and 441 downregulated DEGs for the 3 time points of fruit development (Supplementary Fig. S4D to F). We identified 1 upregulated MYB-related TF gene (MdMYBS1, MD00G1169600) from 446 upregulated DEGs (Supplementary Fig. S4E) and 2 downregulated MYB-related TF genes (PHL11, MD00G1000500; MYB88, MD16G1076100) from 441 downregulated DEGs (Supplementary Fig. S4F). Moreover, because there was a significant difference in carotenoids and ABA levels between “Beni Shogun” and “Yanfu 3” fruits at 30 and 0 DBFR, we identified 3 upregulated MYB-related TF genes from 2,736 upregulated DEGs (Supplementary Fig. S4E) and 10 downregulated MYB-related TF genes from 3,886 downregulated DEGs (Supplementary Fig. S4F) in the 30 DBFR vs. 0 DBFR comparison (Supplementary Fig. S5).
Further RT-qPCR assays showed that MdMYBS1 transcript level was more highly correlated with total carotenoid, phytoene, β-carotene, β-cryptoxanthin and ABA contents compared to the transcript levels of other MYB TF genes (Fig. 1I and Supplementary Table S1). MdMYBS1 was also expressed in the leaf and flower (Supplementary Fig. S6). Furthermore, expression of MdMYBS1, which is high in orange/yellow fruit flesh (i.e. “Gala”) and low in white fruit flesh (i.e. “Granny Smith”), was related with carotenoid abundance and fruit coloration in 13 Malus accessions (Supplementary Fig. S7). We therefore chose MdMYBS1 for further analysis.
MdMYBS1 positively regulates carotenoid and ABA accumulation
MdMYBS1, an R1-type MYB TF, comprises 328 amino acids with a central SANT/MYB DNA-binding domain and an ZnF_C2HC domain, as predicted by the SMART website (Fig. 2A). Phylogenetic analysis indicated the MdMYBS1 amino acid sequence was most similar to that of the Arabidopsis MYB TF KUODA1 (KUA1) (71.56% similarity) (Fig. 2B) (Lu et al. 2014; Huang et al. 2015). The DNA-binding region of MdMYBS1 contains an SHAQKYF motif, which is conserved in R1-type MYB TFs such as AtKUA1, OsMYBS3, and OsMYBS2. AtKUA1 and OsMYBS3 function as transcriptional repressors and contain an EAR motif (LxLxL), whereas OsMYBS2 is a transcriptional activator and lacks an EAR motif (Lu et al. 2002); MdMYBS1 also lacks an EAR motif (Fig. 2C). Subcellular localization analysis using MdMYBS1-GFP fusion protein showed that MdMYBS1 is localized in the nucleus (Fig. 2D).
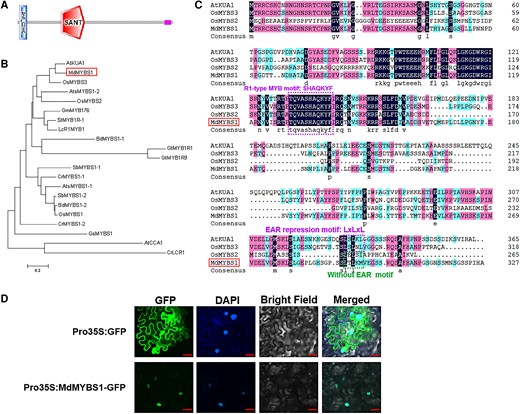
Characterization and subcellular localization of MdMYBS1. A) MdMYBS1 contains a central SANT/MYB DNA-binding domain and an ZnF_C2HC domain, determined on the SMART website (http://smart.embl-heidelberg.de/). B) Phylogenetic analysis of MdMYBS1 indicated that the amino acid sequence of MdMYBS1 was most similar to that of an Arabidopsis protein KUA1. The horizontal branch length represents the proportional to the estimated number of amino acids substitutions per residue (bar = 0.2 aa substitution per residue). Protein sequences of R1-type MYBs deposited in GenBank of NCBI were used to construct the phylogenetic tree are listed in Supplementary Table S4. C) The DNA-binding region of MdMYBS1 includes a conserved SHAQKYF motif of R1-type MYB TFs like AtKUA1, OsMYBS3, and OsMYBS2. AtKUA1 and OsMYBS3 contain an EAR motif (LxLxL), and MdMYBS1 and OsMYBS2 without the EAR motif. D) The MdMYBS1-GFP fusion protein derived from the 35S promoter (Pro35S:MdMYBS1-GFP) and the control 35S:GFP were transiently expressed in N. benthamiana leaves. DAPI was used to stain the nucleus. The fluorescent images were observed and obtained using confocal microscopy. Scale bars = 50 μm.
We performed a transient expression assay to overexpress or silence MdMYBS1 in “Granny Smith” fruit to test whether MdMYBS1 is involved in carotenoid and ABA accumulation. We constructed 35S:MdMYBS1 (MdMYBS1-OVX) vectors for gene overexpression and TRV:MdMYBS1 (MdMYBS1-TRV) vectors for gene silencing; the empty vector pRI101-flag (P101F) served as an overexpression control, and TRV1/TRV2 (TRV) served as a silencing control (Fig. 3A). Deep yellow coloration, an indicator of carotenoid content, was observed in MdMYBS1-OVX fruits (Fig. 3B). MdMYBS1 was highly overexpressed in MdMYBS1-OVX fruits and highly silenced in MdMYBS1-TRV fruits (Fig. 3C). Significantly higher levels of total carotenoid, phytoene, β-carotene, β-cryptoxanthin, violaxanthin and ABA were detected in MdMYBS1-OVX yet much lower in MdMYBS1-TRV fruits (Fig. 3D to F) than those of the controls, whereas the neoxanthin level followed an opposite trend (Fig. 3E).
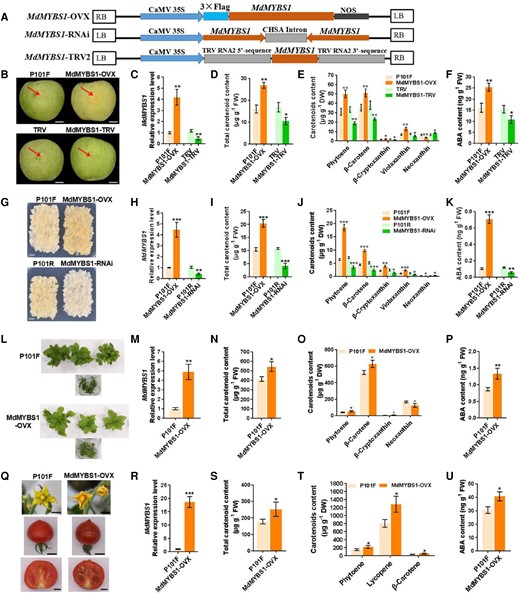
MdMYBS1 promotes carotenoid biosynthesis and endogenous ABA accumulation in transient transgenic apple fruits, transgenic apple calli and plants, and tomato fruits. A) T-DNA region of the vectors MdMYBS1-OVX, MdMYBS1-RNAi, and MdMYBS1-TRV2 used for transformation. B)MdMYBS1 was overexpressed (MdMYBS1-OVX) and silenced (MdMYBS1-TRV) in transient transgenic “Granny Smith” apple fruits. P101F or TRV transient transgenic apple fruits transformed with empty vector PRI101-flag or pTRV2. Scale bars, 1 cm. Fruit images were digitally extracted for comparison. C to F) Levels of MdMYBS1 expression, total carotenoid, phytoene, β-carotene, β-cryptoxanthin, violaxanthin, neoxanthin, and ABA in transient transgenic apple fruits. G)MdMYBS1 was overexpressed and silenced (MdMYBS1-RNAi) in apple calli. P101F or P101R transgenic apple calli transformed with empty vector PRI101-flag or PRI101-AN. Scale bars, 1 cm. H to K) Levels of MdMYBS1 expression, total carotenoid, phytoene, -carotene, β-cryptoxanthin, violaxanthin, neoxanthin, and ABA in transgenic apple calli. L)MdMYBS1 was overexpressed in apple plants. M to P) Levels of MdMYBS1 expression, total carotenoid, phytoene, β-carotene, β-cryptoxanthin, neoxanthin, and ABA in transgenic apple leaves. Q) MdMYBS1 was heterologously overexpressed in tomato fruits. Scale bars, 1 cm. R to U) Levels of MdMYBS1 expression, total carotenoid, phytoene, lycopene, β-carotene, and ABA in transgenic tomato fruits. The data bars represent mean ± standard deviation and the error bars represent standard deviations of 3 independent biological replicates. Asterisks indicate significant differences analyzed by Student's t-test (*P < 0.05, **P < 0.01, ***P < 0.001); ns, not significant.
We also overexpressed or silenced MdMYBS1 in apple calli and plants. We constructed the RNAi vector pRI101-AN-MdMYBS1 (MdMYBS1-RNAi) and used it for stable transformation; the empty vector pRI101-AN (P101R) served as a control (Fig. 3A). MdMYBS1-OVX apple calli (Fig. 3G) and apple plants (Fig. 3L) showed a deeper yellow color than controls. MdMYBS1 was highly overexpressed in MdMYBS1-OVX calli (Fig. 3H) and leaves (Fig. 3M) and highly silenced in MdMYBS1-RNAi calli (Fig. 3H). Levels of total carotenoid, phytoene, β-carotene, β-cryptoxanthin, violaxanthin, and ABA were markedly higher in MdMYBS1-OVX calli (Fig. 3I to K) and apple leaves (Fig. 3N to P), and much lower in MdMYBS1-RNAi calli than in the controls (Fig. 3I to K). However, the neoxanthin level was markedly lower in MdMYBS1-OVX calli (Fig. 3J) and apple leaves (Fig. 3O), and higher in MdMYBS1-RNAi calli than in the controls (Fig. 3J).
Furthermore, we transformed MdMYBS1 into Micro-Tom tomato for heterologous expression. MdMYBS1-OVX tomato flower and fruits showed a deeper yellow color than controls (Fig. 3Q). MdMYBS1 was highly expressed in MdMYBS1-OVX tomato fruits (Fig. 3R). Levels of total carotenoid, phytoene, lycopene, β-carotene, and ABA were markedly higher in MdMYBS1-OVX tomato fruits (Fig. 3S to U). Together, these results demonstrate that MdMYBS1 activates accumulation of β-branch carotenoids and endogenous ABA.
MdMYBS1 regulates carotenoid biosynthesis by activating MdPSY2-1 and MdLCYb
Next, we reasoned that MdMYBS1 might regulate carotenoid accumulation by modulating carotenoid biosynthetic genes. We identified carotenoid biosynthesis genes in RNA-seq data (Supplementary Fig. S8A and B), and found that the expression levels of MdPSY2-1 (MD09G1146800) and MdLCYb (MD06G1049200) were significantly higher in “Beni Shogun” than in “Yanfu 3” at 60, 30, and 0 DBFR (Fig. 1J and K and Supplementary Fig. S8B). In addition, the MdPSY2-1 expression level was positively correlated with phytoene content and MdMYBS1 expression level, and the MdLCYb expression level was positively correlated with β-carotene content and MdMYBS1 expression level (Supplementary Fig. S9A). Moreover, expression levels of MdPSY2-1 and MdLCYb were high in MdMYBS1-overexpressing apple fruits, calli, and leaves, and low in MdMYBS1-silenced apple fruits and calli (Fig. 4A). These results suggest MdMYBS1 might influence carotenoids biosynthesis by regulating MdPSY2-1 and MdLCYb.
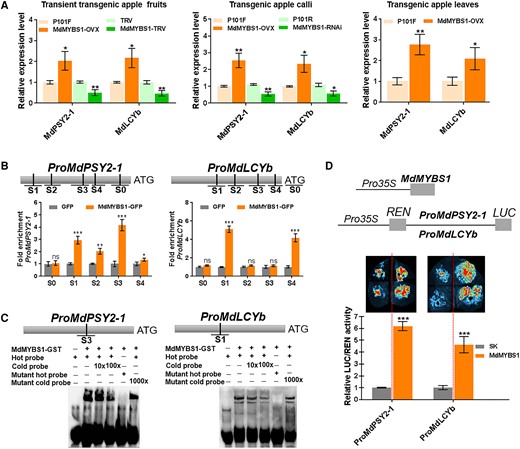
MdMYBS1 promotes the transcription of the carotenoid biosynthetic genes MdPSY2-1 and MdLCYb. A) Expression levels of MdPSY2-1 and MdLCYb in MdMYBS1-overexpressing (MdMYBS1-OVX) or MdMYBS1-silenced (MdMYBS1-RNAi or MdMYBS1-TRV) apple fruits, calli, and leaves. P101F, P101R, or TRV transgenic lines transformed with empty vector PRI101-flag, PRI101-AN or pTRV2. B) ChIP-PCR showing that MdMYBS1 binds to the promoters of MdPSY2-1 and MdLCYb containing the major motif TTATC(YD) or (HY)TATCCA in vivo. Five regions (S0–S4) in MdPSY2-1 and 5 regions (S0–S4) in MdLCYb were investigated. C) EMSA of MdMYBS1 direct binding to the TTTATCTA motif of the MdPSY2-1 promoter, and the CTTATCCA motif of the MdLCYb promoter. D) Dual-LUC assay showing that MdMYBS1 positively regulates MdPSY2-1 and MdLCYb promoter activity. The data bars represent mean ± standard deviation and the error bars represent standard deviations of 3 independent biological replicates. Asterisks indicate significant differences analyzed by Student's t-test (*P < 0.05, **P < 0.01, ***P < 0.001); ns, not significant.
Previous study demonstrated that overexpressing MdPSY2-1 increases levels of total carotenoid, phytoene, phytofluene, and β-carotene (Dang et al. 2021). Here, overexpressing MdLCYb in apple calli enhanced levels of β-carotene, β-cryptoxanthin, violaxanthin, and neoxanthin, whereas it decreased phytoene content (Supplementary Fig. S10). These results indicate that MdPSY2-1 and MdLCYb contribute to carotenoid biosynthesis.
We predicted the cis-elements of MdMYBS1 using JASPAR 2020. Previous studies reported that several R1-type proteins could specifically bind to a core sequence (HY)TATC(YD) (Lu et al. 2002, 2014; Liu et al. 2022). The main cis-elements of “ATTATCTT” (−1,741 bp, S1), “CTTATCGT” (−1,613 bp, S2), “TTTATCTA” (−865 bp, S3), and “AACTATCT” (−760 bp, −600 bp S4) were identified within the MdPSY2-1 promoter. The main cis-elements of “CTTATCCA” (−1,031 bp, S1), “GCTATCTA” (−735 bp, S2), “AAATATCG” (−415 bp, S3), and “TTTATCTG” (−108 bp, S4) were identified within the MdLCYb promoter (Fig. 4B). Y1H assay confirmed that MdMYBS1 binds to the promoters of MdPSY2-1 and MdLCYb (Supplementary Fig. S11).
Next, we confirmed the binding of MdMYBS1 to these major cis-elements in vivo using a chromatin immunoprecipitation (ChIP-PCR) assay. We obtained MdMYBS1-GFP fusion protein from MdMYBS1-overexpressing apple calli; PRI101-GFP (GFP) calli were used as a negative control. MdMYBS1 enhanced the PCR-based detection of gene promoters with the major motif TTATC(YD) or (HY)TATCCA, including the promoters of MdPSY2-1 and MdLCYb (Fig. 4B). In addition, we found that MdMYBS1 directly bound to the TTTATCTA motif of the MdPSY2-1 promoter or the CTTATCCA motif of the MdLCYb promoter using an electrophoretic mobility shift assay (EMSA) (Fig. 4C). Furthermore, we explored how MdMYBS1 influences promoter activity in vivo using a luciferase (LUC) transactivation assay. MdMYBS1 can significantly facilitate the promoter activities of MdPSY2-1 and MdLCYb (Fig. 4D). These results suggest MdMYBS1 acts as a transcriptional activator of MdPSY2-1 and MdLCYb by directly binding to their promoters to regulate carotenoid biosynthesis.
MdMYBS1 regulates ABA accumulation by activating MdNCED1
We reasoned that MdMYBS1 might regulate ABA accumulation by modulating ABA biosynthetic genes. We identified ABA biosynthesis genes in RNA-seq data (Supplementary Fig. S8A and C) and showed that the MdNCED1 (MD05G1282700) expression level was significantly higher in “Beni Shogun” than in “Yanfu 3” at 60, 30, and 0 DBFR (Fig. 1L). In addition, the MdNCED1 expression level was positively correlated with ABA content and MdMYBS1 expression level (Supplementary Fig. S9B). Moreover, the MdNCED1 expression level was high in MdMYBS1-overexpressing apple fruits, calli, and leaves, and was low in MdMYBS1-silenced apple fruits and calli (Fig. 5A). These results suggest MdMYBS1 might influence ABA accumulation by regulating MdNCED1.
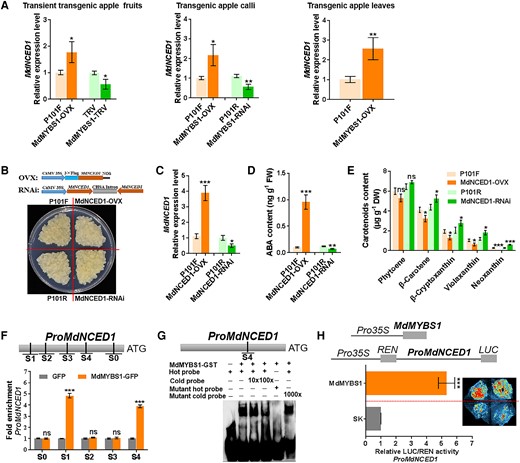
MdMYBS1 promotes the transcription of the ABA biosynthetic gene MdNCED1. A) Expression levels of MdNCED1 in MdMYBS1-overexpressing (MdMYBS1-OVX) or MdMYBS1-silenced (MdMYBS1-RNAi or MdMYBS1-TRV) apple fruits, calli, and leaves. P101F, P101R, or TRV transgenic lines transformed with empty vector PRI101-flag, PRI101-AN, or pTRV2. B)MdNCED1 was overexpressed (MdNCED1-OVX) and silenced (MdNCED1-RNAi) in apple calli. P101F or P101R transgenic apple calli transformed with empty vector PRI101-flag or PRI101-AN. C to E) Levels of MdNCED1 expression, ABA, phytoene, β-carotene, β-cryptoxanthin, violaxanthin, and neoxanthin in transgenic apple calli. F) ChIP-PCR showing that MdMYBS1 binds to the MdNCED1 promoter containing the major motif TTATC(YD) or (HY)TATCCA in vivo. Five regions (S0 to S4) in MdNCED1 were investigated. G) EMSA of MdMYBS1 direct binding to the ATTATCCT motif of the MdNCED1 promoter. H) Dual-LUC assay showing that MdMYBS1 positively regulates the MdNCED1 promoter activity. The data bars represent mean ± standard deviation and the error bars represent standard deviations of 3 independent biological replicates. Asterisks indicate significant differences analyzed by Student's t-test (*P < 0.05, **P < 0.01, ***P < 0.001); ns, not significant.
Here, we overexpressed or silenced MdNCED1 in apple calli (Fig. 5B and C). The ABA level was much higher, and levels of neoxanthin, violaxanthin, β-cryptoxanthin, and β-carotene were much lower in MdNCED1-overexpressing calli, while silencing of MdNCED1 had the opposite effect (Fig. 5D and E). These results indicate that MdNCED1 contributes to ABA accumulation.
The main cis-elements bound by MdMYBS1 of “ACTATCCA” (−1,794 bp, S1), “AAGTATCT” (−1,637 bp, S2), “ATATATCT” (−1,317 bp, S3) and “ATTATCCT” (−1,033 bp, S4) were identified within the MdNCED1 promoter. Y1H assay confirmed that MdMYBS1 binds to the MdNCED1 promoter (Supplementary Fig. S11). Next, we confirmed the binding of MdMYBS1 to these major cis-elements using a ChIP-PCR assay. MdMYBS1 enhanced the PCR-based detection of MdNCED1 promoter with the major motif TTATC(YD) or (HY)TATCCA (Fig. 5F). In addition, we found that MdMYBS1 directly bound to the ATTATCCT motif of MdNCED1 promoter using an EMSA (Fig. 5G). Furthermore, we found that MdMYBS1 can significantly facilitate MdNCED1 promoter activity using a LUC transactivation assay (Fig. 5H). These results suggest MdMYBS1 acts as a transcriptional activator of MdNCED1 by directly binding to its promoter to enhance ABA accumulation.
The MdMYBS1 promoter was activated by exogenous ABA and MdABI5
We identified 3 cis-acting ABA-responsive element (ABRE) motifs in the MdMYBS1 promoter (S1: ‒1,978 bp, CCACGTGG; S2: ‒1,579 bp, CCACGTGG; S3: ‒784 bp, TACGTG). We reasoned that ABA acts on these motifs to regulate MdMYBS1 expression. To examine the impact of ABA on MdMYBS1 transcription, we generated ProMdMYBS1:LUC/GUS fusion constructs containing the 2,016-bp promoter sequence of MdMYBS1 fused to the LUC or GUS reporter. When we expressed the ProMdMYBS1:LUC construct in Nicotiana benthamiana leaves, LUC activity significantly increased under 0.5 mM ABA treatment compared to in the control (ddH2O treatment) (Fig. 6A). Furthermore, we transformed apple calli with the ProMdMYBS1:GUS construct. Significantly higher GUS activity was detected in transgenic calli under 30 µM ABA treatment than in the control (Fig. 6B). These results suggest exogenous ABA promotes MdMYBS1 transcription by enhancing its promoter activity.
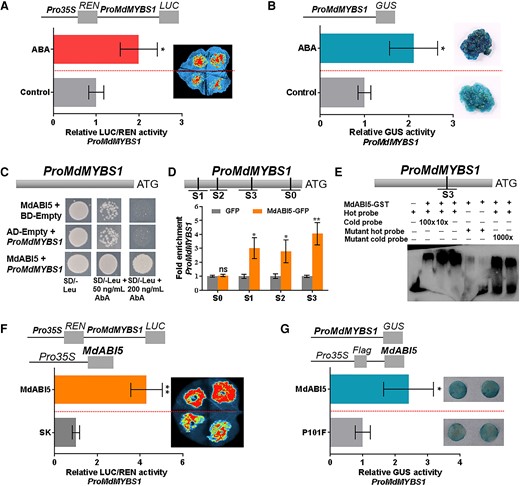
The MdMYBS1 promoter was activated by exogenous ABA and MdABI5. A) Dual-LUC assay showing that the relative LUC/REN activity of the MdMYBS1 promoter increased significantly in N. benthamiana leaves under 0.5 mM ABA treatment compared to the control (ddH2O treatment). B) GUS assay showing that the relative GUS activity of the MdMYBS1 promoter increased significantly in transgenic apple calli under 30 µM ABA treatment compared to the control. C) Y1H assay confirming that MdABI5 binds to the MdMYBS1 promoter. D) ChIP-PCR confirming that MdABI5 binds to the promoter of MdMYBS1 containing the major ABRE motif in vivo. Four regions (S0 to S3) in MdMYBS1 promoter were investigated. E) EMSA confirming that MdABI5 directly binds to the ABRE motif TACGTG (S3) in the MdMYBS1 promoter. F and G) Dual-LUC assay (F) and GUS activity assay (G) showing that MdABI5 positively regulates MdMYBS1 promoter activity. The data bars represent mean ± standard deviation and the error bars represent standard deviations of 3 independent biological replicates. Asterisks indicate significant differences analyzed by Student's t-test (*P < 0.05, **P < 0.01); ns, not significant.
Next, we searched for the TFs that specifically bind to ABRE motif in the MdMYBS1 promoter. The ABRE/G-box motif is recognized by ABI5 TFs (Zinsmeister et al. 2016; Zhao et al. 2020; Song et al. 2022). Using Y1H screening (Jia et al. 2021) and RNA-seq data, 1 MdABI5 (MD14G1021600) was identified. MdABI5 transcript level was significantly higher in “Beni Shogun” than in “Yanfu 3” at 60, 30, and 0 DBFR (Supplementary Fig. S12A), and was correlated with total carotenoid and ABA content (Supplementary Fig. S12B and C). Y1H assay validated that MdABI5 binds the MdMYBS1 promoter (Fig. 6C).
Next, we confirmed the binding of MdABI5 to the ABRE motif in MdMYBS1 promoter using a ChIP-PCR assay. We obtained the MdABI5-GFP fusion protein from MdABI5-overexpressing apple calli; P101-GFP calli were used as a negative control. MdABI5 heightened the PCR-based detection of the MdMYBS1 promoter with ABRE/G-box motifs (Fig. 6D), and EMSA demonstrated MdABI5 bound to the TACGTG motif of ABRE/G-box in the MdMYBS1 promoter (Fig. 6E). Furthermore, we found that MdABI5 can significantly facilitate the MdMYBS1 promoter activity using a LUC transactivation assay (Fig. 6F) and a GUS reporter assay (Fig. 6G). These results suggest MdABI5 acts as a transcriptional activator of MdMYBS1 by directly binding to its promoter.
MdABI5 regulates carotenoids and ABA accumulation, and responds to ABA
To investigate the role of MdABI5 in carotenoid biosynthesis, we separately transiently transformed “Granny Smith” fruit with the 35S:MdABI5 (MdABI5-OVX) and TRV:MdABI5 (MdABI5-TRV) constructs (Fig. 7A and B). Deep yellow coloration was observed in MdABI5-OVX fruit skin (Fig. 7B). Levels of total carotenoid and endogenous ABA were markedly higher in MdABI5-OVX fruit skin yet lower in MdABI5-TRV fruit skin vs. the in controls (P101F and TRV, respectively) (Fig. 7C and D). Furthermore, MdABI5, MdMYBS1, MdPSY2-1, MdLCYb, and MdNCED1 were expressed at much higher levels in MdABI5-OVX fruit skin yet at much lower levels in MdABI5-RNAi fruit skin than in the controls (Fig. 7E). These findings suggest that MdABI5 promotes carotenoid and endogenous ABA accumulation.
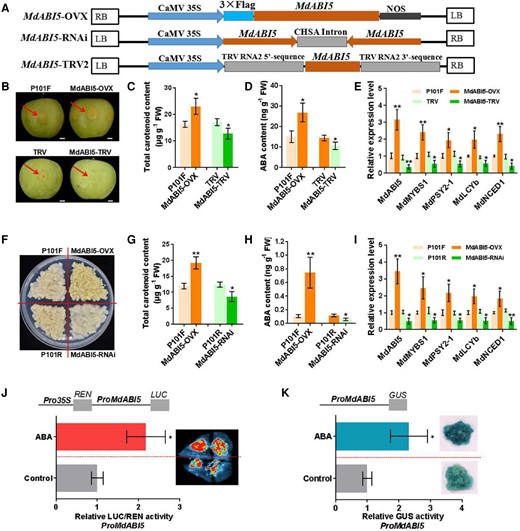
MdABI5 promotes carotenoid and ABA accumulation and responds to ABA. A) T-DNA region of the vectors MdABI5-OVX, MdABI5-RNAi, and MdABI5-TRV2 used for transformation. B)MdABI5 was overexpressed (MdABI5-OVX) and silenced (MdABI5-TRV) in transient transgenic “Granny Smith” apple fruits. P101F or TRV transient transgenic apple fruits transformed with empty vector PRI101-flag or pTRV2. Fruit images were digitally extracted for comparison. Scale bars, 1 cm. C and D) Levels of total carotenoid and ABA in transient transgenic apple fruits. E) Expression levels of MdABI5, MdMYBS1, MdPSY2-1, MdLCYb, and MdNCED1 in transient transgenic apple fruits. F)MdABI5 was overexpressed and silenced (MdABI5-RNAi) in apple calli. P101F or P101R transgenic apple calli transformed with empty vector PRI101-flag or PRI101-AN. G and H) Levels of total carotenoid and ABA in transgenic apple calli. I) Expression levels of MdABI5, MdMYBS1, MdPSY2-1, MdLCYb and MdNCED1 in transgenic apple calli. J) Dual-LUC assay showing that the relative LUC/REN activity of the MdABI5 promoter significantly increased in N. benthamiana leaves under 0.5 mM ABA treatment compared to the control. K) GUS assay showing that the relative GUS activity of the MdABI5 promoter significantly increased in transgenic apple calli under 30 µM ABA treatment compared to the control. The data bars represent mean ± standard deviation and the error bars represent standard deviations of 3 independent biological replicates. Asterisks indicate significant differences analyzed by Student's t-test (*P < 0.05, **P < 0.01).
MdABI5 was also overexpressed (MdABI5-OVX) or silenced (MdABI5-RNAi) in apple calli (Fig. 7A and F). Significantly higher levels of total carotenoid and endogenous ABA were detected in MdABI5-OVX calli yet lower in MdABI5-RNAi calli vs. the controls (Fig. 7G and H). In addition, overexpressing MdABI5 upregulated MdMYBS1, MdPSY2-1, MdLCYb, and MdNCED1, whereas silencing MdABI5 had the opposite effect (Fig. 7I). Taken together, these findings suggest that MdABI5 promotes carotenoid and endogenous ABA accumulation by activating MdMYBS1 transcription.
Next, we amplified the MdABI5 promoter (2,002 bp) and identified several ABRE motifs within it. We reasoned that ABA acts on the ABRE motifs to regulate MdABI5 expression. When we transiently transformed N. benthamiana leaves with the ProMdABI5:LUC fusion construct, LUC activity increased significantly under 0.5 mM ABA treatment compared to in the control (ddH2O treatment) (Fig. 7J). Moreover, we transformed apple calli with the ProMdABI5:GUS fusion construct. GUS activity was significantly higher in transgenic calli under 30 µM ABA treatment compared to in the control (Fig. 7K). These results demonstrate that MdABI5 could be activated by exogenous ABA.
MdABI5–MdMYBS1 cascade regulates carotenoid and ABA accumulation
We explored how the MdABI5–MdMYBS1 transcriptional cascade influences carotenoid biosynthesis by silencing MdMYBS1 (MdMYBS1-RNAi) in apple calli overexpressing MdABI5 (MdABI5-OVX) and silencing MdABI5 (MdABI5-RNAi) in apple calli overexpressing MdMYBS1 (MdMYBS1-OVX) (Fig. 8A). MdMYBS1 was significantly silenced in MdABI5-OVX/MdMYBS1-RNAi calli, and MdABI5 was significantly silenced in MdMYBS1-OVX/MdABI5-RNAi calli (Fig. 8B). The silencing of MdMYBS1 significantly suppressed total carotenoid and ABA production in MdABI5-OVX calli; however, total carotenoid and ABA levels were not significantly affected when MdABI5 was silenced in MdMYBS1-OVX calli (Fig. 8C and D). Furthermore, the silencing of MdMYBS1 significantly suppressed expression levels of MdPSY2-1, MdLCYb and MdNCED1 in MdABI5-OVX calli; however, these were not significantly affected when MdABI5 was silenced in MdMYBS1-OVX calli (Fig. 8E). These results demonstrate that MdABI5 regulates the transcription of MdMYBS1, and MdMYBS1 activates MdPSY2-1, MdLCYb, and MdNCED1 expression. Thus, the MdABI5–MdMYBS1 cascade regulates carotenoid biosynthesis and ABA accumulation.
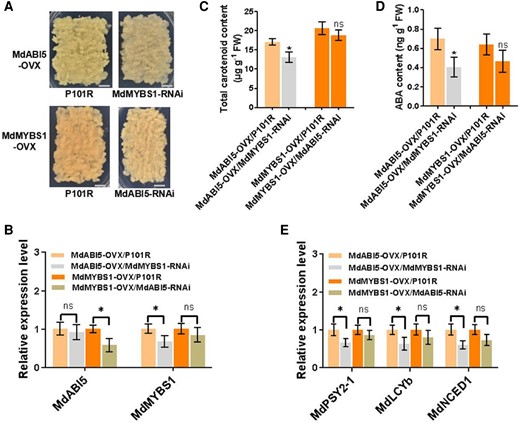
MdABI5–MdMYBS1 cascade regulates carotenoid and ABA accumulation. A) The phenotypes of MdABI5-OVX apple calli with silencing of MdMYBS1 (MdMYBS1-RNAi) and MdMYBS1-OVX apple calli with silencing of MdABI5 (MdABI5-RNAi). Scale bars, 1 cm. B) Transcription levels of MdABI5 and MdMYBS1 in transgenic apple calli. C and D) Total carotenoid content and ABA content in transgenic apple calli. E) Expression levels of MdPSY2-1, MdLCYb, and MdNCED1 in transgenic apple calli. The data bars represent mean ± standard deviation and the error bars represent standard deviations of 3 independent biological replicates. Asterisks indicate significant differences analyzed by Student's t-test (*P < 0.05); ns, not significant.
Exogenous ABA promotes carotenoid accumulation
ABA can promote carotenoid accumulation in tomato and citrus fruits (Wu et al. 2018; Sun et al. 2023). In this study, ABA content was markedly higher in “Beni Shogun” than in “Yanfu 3” during fruit ripening (Fig. 1H), and MdMYBS1 activated MdNCED1 expression to promote ABA accumulation (Fig. 5). We reasoned that ABA could influence carotenoid accumulation in apple fruit. To decipher the potential relationship between ABA and carotenoid accumulation in apple, we harvested “Orin” fruits and treated them with exogenous ABA (0, 0.1, 0.5, or 1 mM) via vacuum injection, and we also vacuum-injected fruits with the ABA inhibitor NDGA (0.5 mM). Treatment with 0.5 or 1 mM ABA accelerated yellow color development in apple fruit, whereas treatment with NDGA suppressed yellow color development (Fig. 9A). ABA treatment strongly induced accumulation of ABA and total carotenoid in fruit flesh, whereas these levels were much lower in fruit flesh under NDGA treatment compared to in controls (Fig. 9B and C). MdABI5, MdMYBS1, MdPSY2-1, MdLCYb, and MdNCED1 were markedly upregulated in fruit flesh treated with 0.5 or 1 mM ABA (Fig. 9D). These results indicate that exogenous ABA promotes carotenoid production by enhancing the expression of carotenoid-related TF genes and carotenoid biosynthetic genes.
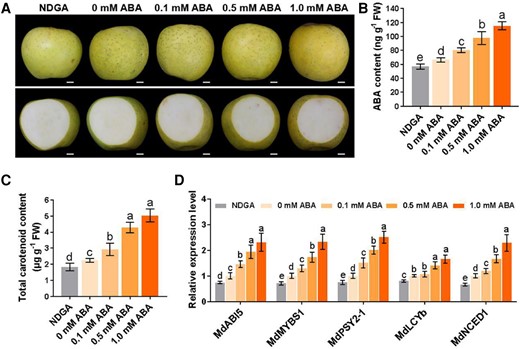
Exogenous ABA promotes carotenoid accumulation in apple fruit. A) “Orin” apple fruits were treated with different concentrations (0, 0.1, 0.5, and 1 mM) of exogenous ABA; 0.5 mM nordihydroguaiaretic acid (NDGA) was used as an ABA inhibitor. Fruit images were digitally extracted for comparison. Scale bars, 1 cm. B and C) Levels of ABA B) and total carotenoid content C) in treated fruit flesh. D) The relative expression levels of MdABI5, MdMYBS1, MdPSY2-1, MdLCYb, and MdNCED1 in treated fruit flesh. The data bars represent mean ± standard deviation and the error bars represent standard deviations of 3 independent biological replicates. Different letters represent significant differences at P < 0.05 by a Tukey's multiple range test using one-way ANOVA.
Discussion
Carotenoid is an important pigment for fruit coloration with considerable nutritional value and has become a major target trait in apple breeding (Ampomah-Dwamena et al. 2012, 2022; Dang et al. 2021). Carotenoid content and compounds display substantial variability in different Malus genotypes. In the current study, we identified carotenoid as the main factor influencing apple flesh color in “Red Fuji” bud mutants “Beni Shogun” and “Yanfu 3” (Fig. 1 and Supplementary Fig. S1). Among carotenoid compounds, lutein content decreases whereas phytoene and β-carotene contents increase during fruit ripening in several plants such as carrot (Daucus carota ssp. sativus) (Clotault et al. 2008), orange (Guzman et al. 2010), and apple (Ampomah-Dwamena et al. 2012, 2022); we observed a similar pattern in “Yanfu 3” and “Beni Shogun” (Fig. 1). The predominant compounds in ripening apple fruits are β-carotene and β-cryptoxanthin in “Aotea”, violaxanthin and neoxanthin in “Royal Gala”, and lutein in “Granny Smith” (Ampomah-Dwamena et al. 2012). Here, we detected 6 carotenoid compounds in ripening apple fruits. The major compounds were β-carotene and phytoene in “Beni Shogun”, β-carotene and β-cryptoxanthin in “Yanfu 3”, and lutein and β-carotene in “Granny Smith” (Figs. 1 and 3). The different predominant compounds in different Malus genotypes might be attributed to differential transcriptional or posttranscriptional regulation (Dang et al. 2021; Ampomah-Dwamena et al. 2022). Analyzing the genetic variations in carotenoid biosynthesis could help to decipher the regulatory mechanism of carotenoid biosynthesis in apple fruit. Furthermore, multiple Malus genotypes with different carotenoid contents could be used to produce new apple varieties with high carotenoid contents.
Carotenoids are isoprenoid compounds that are synthesized by metabolic enzymes (Yuan et al. 2015). The diversity of carotenoid pigmentation is influenced by carotenoid biosynthetic genes (Jang et al. 2020; Zhang et al. 2020). Our previous research demonstrated overexpressing MdPSY2-1 increases the phytoene and total carotenoid contents in apple (Dang et al. 2021). Here, the MdPSY2-1 or MdLCYb expression was highly positively correlated with phytoene or β-carotene content in apple fruits (Fig. 1 and Supplementary Fig. S9), and we determined that overexpressing MdLCYb enhanced levels of β-carotene, β-cryptoxanthin, violaxanthin, and neoxanthin, whereas it decreased phytoene content (Supplementary Fig. S10), indicating that MdPSY2-1 and MdLCYb are major biosynthetic genes contributing to carotenoid biosynthesis in apple.
Previous studies reported that violaxanthin and neoxanthin are precursors of ABA (Perreau et al. 2020; Kavi Kishor et al. 2022). In the study, Neoxanthin content was much lower in “Beni Shogun” fruit with higher levels of carotenoids and ABA than in “Yanfu 3” fruit with lower levels of carotenoids and ABA (Fig. 1). Moreover, the neoxanthin level highly declined in MdMYBS1-overexpressing apple fruits, calli, and leaves, and highly increased in MdMYBS1-silenced apple fruits and calli (Fig. 3). These results indicate that the neoxanthin is the main precursor of ABA synthesis.
MYB TFs play vital roles in growth and development in diverse plants (Dubos et al. 2010; Wu et al. 2022). Several MYBs activate or repress carotenoid biosynthesis by directly modulating the transcription of carotenogenic genes (Sagawa et al. 2016; Meng et al. 2019; Wu et al. 2020). For example, CrMYB68 inhibits α- and β-branch carotenoid biosynthesis in citrus (Zhu et al. 2017), whereas AdMYB7 promotes carotenoid production by directly enhancing AdLCY-β expression in kiwifruit (Ampomah-Dwamena et al. 2019). Here, we found that MdMYBS1 contributes to fruit flesh color of apple and tomato (Fig. 3). MdMYBS1 acts as an activator of the carotenogenic genes MdPSY2-1 and MdLCYb by directly binding to their promoters (Fig. 4). These findings indicate that different subclasses of MYB TFs play multiple roles in modulating carotenoid biosynthesis via controlling the expression of different carotenogenic genes in diverse plant species.
In perennial fruits, somatic mutations known as “bud sports” can arise from epigenetic or genetic alterations (Kobayashi et al. 2004; El-Sharkawy et al. 2015; Jia et al. 2020; Li et al. 2020). Both “Yanfu 3” and “Beni Shogun” are bud mutants of Red Fuji. The MdMYBS1 transcript level was significantly higher in “Beni Shogun” than in “Yanfu 3” during fruit ripening (Fig. 1); however, we could not find the genetic DNA variants of MdMYBS1 including 2,100 bp promoter, exons and introns between “Beni Shogun” and “Yanfu 3” (Supplementary Table S2). Previous studies indicated that epigenetic regulation, such as methylation and histone acetylation, is involved in carotenoid synthesis (Arango et al. 2016; Li et al. 2022). We speculate that the epigenetic regulation of MdMYBS1 is involved in regulating carotenoid biosynthesis; however, this requires further study.
The phytohormone ABA plays key roles in fruit development, coloration, and ripening (Leng et al. 2014). ABA is derived from the cleavage of carotenoid precursors. ABA biosynthesis is restricted by 1 rate-limiting step catalyzed by the enzyme NCED (Frey et al. 2012; Sato et al. 2018; Lang et al. 2021; Wang et al. 2021). Silencing SlNCED1 reduces endogenous ABA levels and increases contents of lycopene and β-carotene in tomato fruits (Sun et al. 2012a, 2012b). In our study, MdNCED1 was positively related to ABA accumulation during the ripening of apple fruit flesh (Fig. 1 and Supplementary Fig. S9), indicating that MdNCED1 is related to ABA accumulation during the ripening of apple fruit flesh. Moreover, overexpressing MdNCED1 in apple calli leads to a higher ABA level and lower levels of neoxanthin, violaxanthin, β-cryptoxanthin, and β-carotene (Fig. 5), indicating that MdNCED1 is the major protein contributing to ABA accumulation.
NCED gene expression is regulated by a series of TFs (Ma et al. 2018; Lang et al. 2021; Martín-Pizarro et al. 2021). Ethylene response TF PpERF3 modulates ABA biosynthesis by enhancing PpNCED2/3 expression in peach (Wang et al. 2019). Here, MdNCED1 expression and ABA levels were much higher in MdMYBS1-overexpressing fruits and calli and much lower in MdMYBS1-silenced fruits and calli (Fig. 5). In addition, we demonstrated that MdMYBS1 enhances MdNCED1 expression via binding to its promoter (Fig. 5), indicating that MdMYBS1 promotes ABA accumulation via activating MdNCED1 expression.
Exogenous ABA treatment induces fruit ripening in grape (Vitis vinifera) berries (Giribaldi et al. 2010). The interaction between ABA and ethylene modulates fig (Ficus carica) fruit ripening (Qiao et al. 2021). Apple fruit ripening is promoted by ethylene. However, apple fruit ripening is also regulated by the dynamic interaction between phytohormones (e.g. ethylene and ABA) (Ji and Wang 2021). Both “Yanfu 3” and “Beni Shogun” are bud mutants of Red Fuji. However, “Beni Shogun” fruits ripened 1 month earlier than “Yanfu 3” fruits, and ABA content was significantly higher in “Beni Shogun” fruit flesh compared to in “Yanfu 3” (Fig. 1). We propose that the higher endogenous ABA content induces fruit ripening in “Beni Shogun” and that the MdMYBS1–MdNCED1 module or MdMYBS1-interaction proteins are involved in regulating the early maturity of “Beni Shogun” fruit; these ideas warrant further study.
ABRE motifs are present in numerous genes and respond to ABA (Fujita et al. 2005; Yoshida et al. 2010; Liu et al. 2022). We determined that ABA acts on ABRE motifs in the MdMYBS1 and MdABI5 promoters to influence their expressions (Figs. 6 and 7). Therefore, MdMYBS1 and MdABI5 promotes ABA accumulation; in turn, higher ABA levels enhance MdMYBS1 and MdABI5 expression to promote carotenoid accumulation. The ABRE/G-box motif is recognized by ABI5 TFs (Zinsmeister et al. 2016; Zhao et al. 2020; Song et al. 2022). In this study, we identified MdABI5 as a transcriptional activator that specifically binds to the ABRE motif in the MdMYBS1 promoter and enhances its transcription (Fig. 6). ABI5 is a bZIP TF whose disruption increases carotenoid and chlorophyll biosynthesis in the green alga (Bai et al. 2021). Here, we demonstrated that MdABI5 responds to ABA and activates carotenoid accumulation (Fig. 7). These findings suggest that additional TFs might be involved in ABA-regulated carotenoid biosynthesis, a notion that requires further study.
ABA influences carotenoid biosynthesis in diverse plants (Khaleghnezhada et al. 2019; Liu et al. 2020). For example, ABA promotes the accumulation of carotenoid during fruit color development in tomato (Barickman et al. 2014; Wu et al. 2018). The CsHB5-CsbZIP44 module regulates ABA-induced carotenoid biosynthesis in citrus (Sun et al. 2023). Here, we established that ABA treatment strongly induced carotenoid accumulation in apple fruit, and MdMYBS1 and MdABI5 were upregulated in ABA-treated fruits (Fig. 9). These results confirm that ABA promotes carotenoid production by enhancing the transcription of genes encoding carotenoid-related TFs.
In summary, MdMYBS1 contributes to carotenoid accumulation via activating carotenogenic genes MdPSY2-1 and MdLCYb. MdMYBS1 enhances ABA accumulation by activating MdNCED1 expression. In addition, MdABI5 activates MdMYBS1 expression by binding its promoter to increase carotenoid and ABA accumulation. Furthermore, ABA promotes carotenoid biosynthesis and enhances the MdMYBS1 and MdABI5 promoter activities (Fig. 10). Overall, our findings promise to be a major advance in the regulatory mechanism that the MdABI5–MdMYBS1 cascade activated by ABA regulates carotenoids and ABA production, and lead the way to other avenues in the breeding and planting for genetic improvement of fruit coloration and quality.
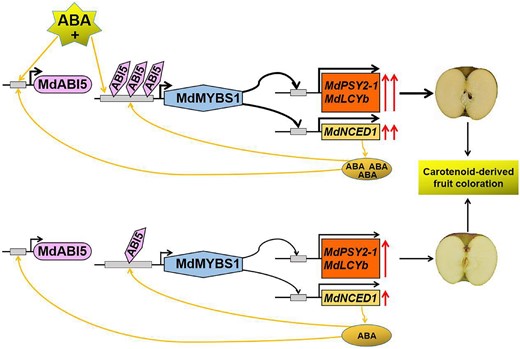
Proposed model for the roles of the MdABI5–MdMYBS1 cascade activated by ABA in regulating carotenoid-derived fruit coloration and ABA accumulation in apple. Carotenoid is the main factor influencing fruit flesh color. MdMYBS1 contributes to carotenoid accumulation via activating carotenogenic genes MdPSY2-1 and MdLCYb. MdMYBS1 enhances ABA accumulation by activating MdNCED1 expression. In addition, MdABI5 activates MdMYBS1 expression by binding its promoter to increase carotenoid and ABA accumulation. Furthermore, exogenous ABA promotes carotenoid biosynthesis and enhances MdMYBS1 and MdABI5 transcription by activating their promoter activities.
Materials and methods
Plant materials
“Yanfu 3” is a red skin mutant and “Beni Shogun” is an early mature mutant of “Red Fuji” apple (M. domestica Borkh.). “Yanfu 3” and “Beni Shogun” apple fruits were harvested and collected from the Laixi breeding farm (Laixi City, Qingdao City, Shandong Province, China) in 2019. The apple trees were grown under identical conditions using standard cultivation and management practices. Fruits were harvested every 30 d beginning at 60 d before fruit ripening (DBFR) and ending with mature fruit at 0 DBFR (Beni Shogun, September 28, 2019; Yanfu 3, October 28, 2019). At each harvesting time, 18 fruits were collected from 8 trees and were divided into 3 sets, each containing 6 fruits; each set was considered to be 1 biological replicate. The fruit flesh of each set was collected and frozen in liquid nitrogen, and stored at −78 °C to analyze carotenoid contents, plant hormone contents, and gene expression. “Orin” calli were incubated at 25 °C under dark conditions (Dang et al. 2021). “Micro-Tom” tomato (S. lycopersicum) and N. benthamiana plantlets were incubated under a 16-h light/8-h dark cycle at 23 °C (Jia et al. 2021; Zhu et al. 2023).
Exogenous ABA treatment in “Orin” fruits
“Orin” fruits were harvested at 130 days after full blossom (DAFB) from the Laixi Gaosong farm (Laixi City, Qingdao City, Shandong Province, China) in 2021. For ABA treatment, the fruits were vacuum-injected with 0.1, 0.5, or 1 mM ABA (catalog no. 862169, Sigma-Aldrich, USA). For ABA inhibitor treatments, the fruits were vacuum-injected with 0.5 mM nordihydroguaiaretic acid (NDGA) (catalog no. IN0590, Solarbio Life Science, China) (Yue et al. 2023). The fruits were vacuum-injected with H2O (0 mM ABA) as a control. All treated fruits were incubated under a 16-h light (600 μmol m−2 s−1)/8-h dark cycle at 25 °C. Flesh samples from 30 fruits per treatment were collected at 14 d after infiltration and divided into 3 sets, each containing 10 fruits; each set was considered to be 1 biological replicate. The fruit flesh of each set was collected and frozen in liquid nitrogen, and stored at −78 °C for experiments.
Carotenoid measurement
Carotenoid was extracted from fruits, leaves or calli with acetone:hexane:ethanol (1:1:1, v/v/v). Total carotenoid was quantified using a UV–vis spectrophotometer. Carotenoids were measured and analyzed by MetWare Company (http://www.metware.cn/), and the extracts of carotenoids were measured and determined by an LC–MS/MS system (Jia et al. 2019; Dang et al. 2021). Three biological replicates were executed. A standard curve was used to quantify carotenoid levels via changing the pertinent peak area to concentration. The authentic standards of carotenoids included phytoene (CAS no. 13920-14-4, BOC, USA), phytofluene (CAS no. 27664-65-9, 13920-14-4, BOC, USA), α-carotene (CAS no. 7488-99-5, BOC, USA), β-cryptoxanthin (catalog no. ZES-0317S, ExtraSynthese, France), violaxanthin (catalog no. 52444, Sigma, USA), neoxanthin (CAS no. 14660-91-4, BOC, USA), β-carotene (catalog no. RO19713, RHAWN, China), zeaxanthin (catalog no. BD2134, Bide, China), and lutein (catalog no. X109574, Aladdin, China).
Quantification of ABA
ABA was extracted from samples using a mixed solution of methanol:water:formic acid (15:4:1, v/v/v) supplemented with 1 ng mL−1 of the internal standard d6-ABA (catalog no. HY-100560S, MCE, USA) for quantitation. The extracts were evaporated to dryness and then dissolved in a mixed solution of methanol:water (4:1, v/v). Three biological replicates of each set of samples were executed. The extracts of ABA were measured and determined by the LC–MS/MS system (Niu et al. 2014). A standard curve was used to quantify carotenoid levels via changing the pertinent peak area to concentrations. The ABA standard (catalog no. 862169) was purchased from Sigma-Aldrich.
RNA-seq analysis
Total RNA was extracted from flesh of Yanfu 3 and Beni Shogun fruit at 3 developmental stages, 60, 30, and 0 DBFR by means of an RNAprep Pure Plant Plus Kit (catalog no. DP441, Tiangen, China) (Jia et al. 2021). Three biological replicates of each set of samples were executed, and 18 RNA-seq libraries were generated and sequenced. RNA-seq was carried out by Novogene Co. (Beijing, China). The Illumina HiSeq 2000 platform (Illumina, San Diego, CA, USA) was used for library sequencing, and RNA-seq analysis was executed as described by Jia et al. (2021). Clean reads were mapped to the apple genome sequence (https://www.rosaceae.org/) (Daccord et al. 2017). The RNA-seq data were submitted to GenBank of NCBI (PRJNA985276).
RT-qPCR assay
Total RNA was extracted and RT-qPCR was executed as described in previous study (Dang et al. 2021; Jia et al. 2021). First-strand cDNA was synthesized by a PrimeScript RT reagent kit, and qPCR assay was executed using SYBR Premix Ex Taq (Takara, Japan). The relative expression levels were analyzed and determined by the 2−ΔΔCT method. MdEF1α (MD04G1011000) and MdActin7 (MD01G1001600) were used as internal control genes (Jia et al. 2021; Ampomah-Dwamena et al. 2022). Three biological replicates were executed. The RT-qPCR primers were designed online (https://www.ncbi.nlm.nih.gov/tools/primer-blast/) and are shown in Supplementary Table S3.
Cloning of gene sequences and phylogenetic analysis
The full-length coding sequence (CDS) of MdMYBS1, MdbABI5, MdLCYb, or MdNCED1 were cloned from the cDNA of “Beni Shogun” fruit flesh. The promoter sequences (listed in Supplementary Table S2) of genes including MdMYBS1, MdABI5, MdPSY2-1, MdLCYb, and MdNCED1 were cloned from the DNA of young “Beni Shogun” leaves that were collected in late spring. The prediction of cis-acting regulatory elements in gene promoters was conducted using PlantCARE database (http://bioinformatics.psb.ugent.be/webtools/plantcare/html/). The cis-elements of TFs were predicted using JASPAR 2020 (https://jaspar.genereg.net//) (Fornes et al. 2019; Liu et al. 2022). Protein sequences of R1-type MYBs (listed in Supplementary Table S4) deposited in GenBank of NCBI were used to construct the phylogenetic tree using MEGA version 6.0 software (Jia et al. 2021). The primers are shown in Supplementary Table S3.
Vector construction and stable transformation of apple and tomato
The CDS of MdMYBS1, MdbABI5, MdLCYb, or MdNCED1 was cloned into the previously generated overexpression vector PRI101-FLAG (Dang et al. 2021). The RNAi constructs were obtained by cloning a 435-bp CDS fragment (between +475 and +909 bp) of MdMYBS1, a 398-bp CDS fragment (between +301 and +698 bp) of MdABI5, and a 429-bp CDS fragment (between +4 and +432 bp) of MdNCED1 into the RNAi vectors PRI101-AN and pTRV2 (Patade et al. 2014; Yue et al. 2020). Agrobacterium tumefaciens strain EHA105 harboring these constructs were transformed into apple Orin calli, apple GL-3 leaves, and “Micro-Tom” tomato plants (Jia et al. 2019, 2021; Zhu et al. 2023). The primers are shown in Supplementary Table S3.
Transient transformation of apple fruit
Agrobacterium tumefaciens strain GV3101 cells harboring the overexpression constructs or interference constructs of MdMYBS1 and MdbABI5 were collected by centrifugation and resuspended in mixed infiltration buffer (pH 5.6) containing 10 mM MES, 10 mM MgCl2, and 200 μM acetosyringone. Fruits of green apple “Granny Smith” were harvested at 140 DAFB and used for transient transformation. These Agrobacterium cultures were injected into the space between the fruit skin and flesh (An et al. 2021; Dang et al. 2021). All treated fruits were incubated at 25 °C and were collected 14 d after infiltration. The infiltrated areas of the fruits were separately divided into 3 groups, frozen in liquid nitrogen, and stored at −78 °C to analyze carotenoid content, plant hormone content, and gene expression.
Subcellular localization
The fusion construct 35S:MdMYBS1‒GFP was gained by cloning the CDS of MdMYBS1 (without stop codon) into the pRI101-GFP vector. Agrobacterium strain GV3101 cells containing the 35S:MdMYBS1-GFP construct were infiltrated into N. benthamiana leaves and incubated for 3 d at 25 °C. The GFP fluorescent signals were excited at 488 nm and detected at 495 to 545 nm with approximately 8% laser value and the around 700 master gain value under a laser scanning confocal microscope (Leica, Wetzlar, Germany). 4′,6-diamidino-2-phenylindole (DAPI; Invitrogen, USA) was used to stain the nucleus (Dang et al. 2021; Jia et al. 2021).
GUS activity analysis
The Pro:GUS fusion vector ProMdMYBS1:GUS or ProMdABI5:GUS was generated by cloning the promoter fragment of MdMYBS1 (2,016 bp) or MdABI5 (2,005 bp) into pCAMBIA1301 (primers are shown in Supplementary Table S3). Agrobacterium GV3101 cells harboring the Pro:GUS fusion constructs were infiltrated into apple calli and N. benthamiana leaves (Jia et al. 2021; Zhu et al. 2023). Three biological replicates were executed. GUS staining was conducted using a β-Galactosidase Reporter Gene Staining Kit (Solarbio, China).
Dual-LUC assay
A series of promoter-LUC reporter constructs was obtained by cloning the promoter fragment of MdABI5, MdMYBS1, MdPSY2-1, MdLCYb, or MdNCED1 into pGreenII 0800-LUC vector. The effector construct 35S:MdMYBS1 or 35S:MdABI5 was obtained by cloning the CDS of MdMYBS1 or MdABI5 into the effector vector pGreenII 62-SK (primers are shown in Supplementary Table S3). The effectors with their corresponding reporters were coinfiltrated into the N. benthamiana leaves. The LUC activities of firefly and Renilla (LUC/REN ratios) were analyzed at 72 h after the infiltration using the Dual-Luciferase Reporter Assay System (Promega, USA) (Dang et al. 2021; Jia et al. 2021; Zhu et al. 2023). Six biological replicates were executed. For ABA treatment, the infiltrated leaves were sprayed with 0.5 mM ABA 12 h before analyzing.
Y1H screening of the cDNA library
Y1H screening was performed using a previously constructed apple fruit cDNA library (Jia et al. 2021). The BD-MdMYBS1 construct was obtained by cloning a 473-bp promoter fragment (between −2,016 and −1,544 bp) of MdMYBS1 into the reporter vector pAbAi (primers are shown in Supplementary Table S3) and introduced into Y1H-susceptible yeast. Next, the AD-cDNA library was introduced into susceptible yeast with BD-MdMYBS1 to screen and identify the specific binding of TFs.
Protein–promoter interaction validation
For the Y1H assay (Dang et al. 2021; Jia et al. 2021), the CDS of MdMYBS1 or MdABI5 was cloned into the effector vector pJG4-5 or pGADT7 (Clontech, USA). The promoter fragment of MdPSY2-1, MdLCYb, or MdNCED1 was cloned into the reporter vector pLacZi (Clontech, USA), and the MdMYBS1 promoter fragment was cloned into the reporter vector pAbAi (Clontech, USA). The primers are listed in Supplementary Table S3.
For the EMSA, the fusion construct MdMYBS1-GST or MdABI5-GST with GST-tag were obtained by cloning the CDS of MdMYBS1 or MdABI5 into pGEX-4T-1 vector, and then separately introduced into Escherichia coli cells BL21 (DE3) for protein production. The 5ʹ and 3ʹ Biotin end-labeled DNA probes for EMSA were synthesized by the Sangon Biotech (China). The EMSA was carried out by a LightShift Chemiluminescent EMSA Kit (Thermo Fisher Scientific, USA) as described in previous study (Dang et al. 2021; Jia et al. 2021). The primers and probe sequences for EMSA are shown in Supplementary Table S3.
For the ChIP-PCR analysis, the fusion construct 35S:MdMYBS1‒GFP or 35S:MdABI5‒GFP with GFP tag were obtained by cloning the CDS of MdMYBS1 or MdABI5 into the pRI101-GFP vector. GFP-tag transgenic calli were used for the ChIP-PCR assay (Dang et al. 2021; Jia et al. 2021; Zhu et al. 2023), which was carried out using an EpiQuik Plant ChIP Kit (EpiGentek, USA). The chromatin DNA fragments were incubated with anti-GFP antibody (Abcam, UK), and then qPCR was used to examine the enrichment of immunoprecipitated chromatin. MdACTIN served as the internal control to normalize the ChIP enrichment. Three biological replicates were executed. Primers used for ChIP-PCR are shown in Supplementary Table S3.
Statistical analyses
The data from the experiments in this study were obtained using 3 independent biological replicates. Significant differences between samples were analyzed by Student's t-test (*P < 0.05, **P < 0.01, ***P < 0.001), and differences among samples were analyzed by one-way ANOVA (P < 0.05) using GraphPad 9.0 software and Microsoft Excel 2019.
Accession numbers
The accessions of the sequence of the genes and proteins used in this study are deposited in M. domestica genome (GDDH13 v1.1) database and GenBank database of NCBI: MdMYBS1 (MD00G1169600), MdPSY2-1 (MD09G1146800), MdLCYb (MD06G1049200), MdNCED1 (MD05G1282700), MdABI5 (MD14G1021600). Protein sequences of R1-type MYBs are listed in Supplementary Table S4.
Acknowledgments
We thank the Laixi breeding farm and Laixi Gaosong farm (Qingdao City, China) for providing experimental resources. We thank Planteditors (www.planteditors.com) for editing this manuscript.
Author contributions
D.J. and Y.Y. designed the experiments and analysis; D.J., Y.L., K.J., B.H., Q.D., H.W., X.W., and C.L. performed the experiments; D.J., Y.Z., and J.N. carried out data analyses; Y.Y., Y.Z., and J.N. contributed materials/analysis tools; D.J., and Y.Y. wrote the article. All authors read and approved the manuscript.
Supplementary data
The following materials are available in the online version of this article.
Supplementary Figure S1. The concentrations of total flavonoid and total carotenoid.
Supplementary Figure S2. The mass spectrum of carotenoid compounds and abscisic acid (ABA) using LC–MS/MS.
Supplementary Figure S3. Levels of carotenoid compounds identified by LC–MS/MS of “Yanfu 3” and “Beni Shogun” fruits at 60, 30, and 0 d before fruit ripening (DBFR).
Supplementary Figure S4. RNA-seq analysis for fruit flesh of “Yanfu 3” and “Beni Shogun” at three ripening stages of 60, 30, and 0 d before fruit ripening (DBFR).
Supplementary Figure S5. The screening of candidate MYB-related transcription factors.
Supplementary Figure S6. The expression pattern of MdMYBS1 in apple leaf, flower and fruit of “Yanfu 3” and “Beni Shogun”.
Supplementary Figure S7. The expression pattern of MdMYBS1 in ripened fruit flesh of 13 Malus genotypes.
Supplementary Figure S8. The screening of carotenoid and abscisic acid (ABA) biosynthetic genes.
Supplementary Figure S9. Correlation analysis.
Supplementary Figure S10. Overexpressing MdLCYb in apple calli influences carotenoid biosynthesis.
Supplementary Figure S11. The Y1H assay was performed to show that MdMYBS1 binds to the promoters of MdPSY2-1, MdLCYb and MdNCED1.
Supplementary Figure S12. The identification of candidate MdABI5 transcription factor.
Supplementary Table S1. Pearson’s correlation between MYB TFs transcript levels and content of total carotenoid, phytoene, β-Carotene, β-Cryptoxanthin or ABA in “Yanfu 3” and “Beni Shogun” fruit flesh.
Supplementary Table S2. Promoter sequences analyzed in the study.
Supplementary Table S3. Primer and probe sequences used in the study.
Supplementary Table S4. Protein sequences of R1-type MYB TFs were used to construct the phylogenetic tree.
Funding
This work was supported by the National Natural Science Foundation of China (31972362, U22A20493, 32272657, 32001993), the Project of the Shandong Natural Science Foundation (ZR2020QC143), the Taishan Scholar Foundation of Shandong Province (tstp20221134), and the Shandong Key R&D Plan (Agricultural Variety Project) (2022LZGCQY008).
Data availability
The data that support the findings of this study are available from the corresponding author upon request.
Dive Curated Terms
The following phenotypic, genotypic, and functional terms are of significance to the work described in this paper:
References
Author notes
The author responsible for distribution of materials integral to the findings presented in this article in accordance with the policy described in the Instructions for Authors (https://dbpia.nl.go.kr/plphys/pages/General-Instructions) is: Yongbing Yuan ([email protected]).
Conflict of interest statement. None declared.