-
PDF
- Split View
-
Views
-
Cite
Cite
Lei Xue, Xiaofen Liu, Wenli Wang, Dan Huang, Chuanhong Ren, Xiaorong Huang, Xueren Yin, Kui Lin-Wang, Andrew C Allan, Kunsong Chen, Changjie Xu, MYB transcription factors encoded by diversified tandem gene clusters cause varied Morella rubra fruit color, Plant Physiology, Volume 195, Issue 1, May 2024, Pages 598–616, https://doi.org/10.1093/plphys/kiae063
- Share Icon Share
Abstract
Chinese bayberry (Morella rubra) is a fruit tree with a remarkable variation in fruit color, ranging from white to dark red as determined by anthocyanin content. In dark red “Biqi” (BQ), red “Dongkui” (DK), pink “Fenhong” (FH), and white “Shuijing” (SJ), we identified an anthocyanin-related MYB transcription factor-encoding gene cluster of four members, i.e. MrMYB1.1, MrMYB1.2, MrMYB1.3, and MrMYB2. Collinear analysis revealed that the MYB tandem cluster may have occurred in a highly conserved region of many eudicot genomes. Two alleles of MrMYB1.1 were observed; MrMYB1.1-1 (MrMYB1.1n) was a full-length allele and homozygous in “BQ”, MrMYB1.1-2 (MrMYB1.1d) was a nonfunctional allele with a single base deletion and homozygous in “SJ”, and MrMYB1.1n/MrMYB1.1d were heterozygous in “DK” and “FH”. In these four cultivars, expression of MrMYB1.1, MrMYB1.2, and MrMYB2 was enhanced during ripening. Both alleles were equally expressed in MrMYB1.1n/MrMYB1.1d heterozygous cultivars as revealed by a cleaved amplified polymorphic sequence marker. Expression of MrMYB1.3 was restricted to some dark red cultivars only. Functional characterization revealed that MrMYB1.1n and MrMYB1.3 can induce anthocyanin accumulation while MrMYB1.1d, MrMYB1.2, and MrMYB2 cannot. DNA–protein interaction assays indicated that MrMYB1.1n and MrMYB1.3 can directly bind to and activate the promoters of anthocyanin-related genes via interaction with a MYC-like basic helix-loop-helix protein MrbHLH1. We concluded that the specific genotype of MrMYB1.1 alleles, as well as the exclusive expression of MrMYB1.3 in some dark red cultivars, contributes to fruit color variation. The study provides insights into the mechanisms for regulation of plant anthocyanin accumulation by MYB tandem clusters.
Introduction
Anthocyanins are natural water-soluble plant pigments present in many plant tissues to produce the pink, red, blue, and purple colors (Koes et al. 2005; Grotewold 2006). Anthocyanins not only have multiple physiological functions in plants such as attracting pollinating insects and animals (Winkel-Shirley 2001; Hoballah et al. 2007), defense against biotic and abiotic stresses (Chalker-Scott 1999), and protecting against UV-B radiation damage (Forkmann and Martens 2001; Gould 2004), but also their presence in edible fruits and vegetables are advantageous to human health as well via their efficient antioxidant properties (Kong et al. 2003; He and Giusti 2010).
The biosynthesis of anthocyanins has been extensively studied in plants and its biosynthetic pathway is highly conserved (Holton and Cornish 1995; Jaakola 2013). Anthocyanins are produced through a sub-route of the flavonoid biosynthesis pathway, which utilizes a group of catalytic enzymes, including phenylalanine ammonia lyase, chalcone synthase (CHS), chalcone isomerase (CHI), flavanone 3-hydroxylase (F3H), flavonoid 3′-hydroxylase (F3′H), dihydroflavonol 4-reductase (DFR), leucoanthocyanidin dioxygenase/anthocyanidin synthase, and (UDP)-glucose:flavonoid-3-O-glycosyltransferase (UFGT; Boss et al. 1996; Winkel-Shirley 2001; Koes et al. 2005). After biosynthesis, anthocyanins are transported into vacuoles for storage through the activity of glutathione S-transferase (GST) and other presumed anthocyanin transporters (Gonzalez et al. 2008; Zhao et al. 2020; Xue et al. 2022).
The expression of anthocyanin biosynthesis and transport genes are coordinately induced by a transcriptional regulatory MYB-bHLH-WDR complex consisting of DNA-binding R2R3 MYB transcription factors (TFs), MYC-like basic helix-loop-helix (bHLH) TFs, and WD40-repeat proteins. The most specific members of the complex are the MYB regulators, which have been shown to be transcriptional activators of anthocyanin biosynthetic genes in numerous plant species (e.g. Allan et al. 2007; Jaakola 2013; Lai et al. 2014; Xu et al. 2015; Allan and Espley 2018; Wang et al. 2022; Zhao et al. 2023).
Chinese bayberry (Morella rubra) is a species with a large variation in fruit color and can be divided into four types distinguished by the color of its ripe fruit: dark red, red, pink, and white. In previous studies, Niu et al. (2010) identified the TF MrMYB1 regulating anthocyanin accumulation in bayberry fruits, while Huang et al. (2013) confirmed its function through stable overexpression in Arabidopsis (Arabidopsis thaliana) and tobacco (Nicotiana tabacum). Liu et al. (2013a, 2013b) identified MrbHLH1 and MrWD40-1 TFs involved in regulating anthocyanin accumulation in bayberry fruits. Analysis of mRNA sequence of MrMYB1 from different colored bayberry cultivars reveals that MrMYB1d, an MrMYB1 allele with a 1 bp deletion at nucleotide 30 of coding sequence (CDS), is present in red and white cultivars. mRNA of normal allele, MrMYB1, is not detectable in white cultivar “Shuijing” (SJ; Niu et al. 2010). However, it is unclear whether MrMYB1 exists in the genomic DNA (gDNA) of this white cultivar, and whether MrMYB1 genomic sequence varies among different colored cultivars. For this purpose, we analyzed sequences of MrMYB1 alleles from different cultivars at the genomic level and unexpectedly more than two sequences of MrMYB1 were observed in an individual cultivar. This means that MrMYB1 must be duplicated and then diversified since Chinese bayberry is a diploid species. Such duplication of anthocyanin-related MYBs has been reported in grape (Vitis vinifera; Kobayashi et al. 2004; Walker et al. 2007), peach (Prunus persica; Zhou et al. 2014), citrus (Citrus spp.; Butelli et al. 2017; Huang et al. 2018), kiwifruit (Actinidia chinensis; Wang et al. 2022), etc.
Recently, while exploring the genetic basis of sex determination in bayberry, the genomes of male and female trees were sequenced (Jia et al. 2019). With the availability of whole genome, we identified a MYB gene cluster at a 40.20 kb interval on chromosome 6, including MrMYB1 and MrMYB2 reported previously (Niu et al. 2010), and two other genes with substantial sequence similarity to MrMYB1. In this study, these two genes were designated MrMYB1.2 and MrMYB1.3 and accordingly, the original MrMYB1 and MrMYB1d were renamed as MrMYB1.1n, normal allele, and MrMYB1.1d, mutated allele with a single base deletion, respectively. We analyzed the sequence, expression, and functional differences of the four MYBs in this gene cluster with different colored fruits. Color diversity was shown to be attributable to the genotypes of MrMYB1.1 alleles in various cultivars as well as exclusive expression of MrMYB1.3, another transcriptional activator, in some dark red cultivars.
Results
Genomic structure of MrMYB cluster in four cultivars with different ripe fruit colors
Chinese bayberry (M. rubra) is a fruit crop with high anthocyanin accumulation in ripe fruit of most cultivars, where cyanidin 3-O-glucoside (C3G) accounts for 97% of the total anthocyanin content (Fang et al. 2006). “Biqi” (BQ), “Dongkui” (DK), and “Fenhong” (FH) deepened in color during fruit development, turning into dark red, red, and pink, respectively, when fully ripe (stage 4, S4), while “SJ” remained white during ripening (Fig. 1A). Anthocyanin content increased quickly at the end of fruit development (Fig. 1B), reaching 110.85, 64.01, and 13.74 mg/100 g fresh weight (FW) for “BQ”, “DK”, and “FH”, respectively. Anthocyanin was not detectable in “SJ” fruit.

Changes in fruit pigmentation and anthocyanin content during fruit development and ripening in Chinese bayberry. A) Photograph of four cultivars at four different fruit development stages. “BQ”, “DK”, “FH”, and “SJ” represent “Biqi”, “Dongkui”, “Fenhong”, and “Shuijing”, respectively. S1 (stage 1), S2, S3, and S4 represent 60 DAFB, 70 DAFB, 74 DAFB, and 86 DAFB, respectively. Images were digitally extracted for comparison. B) Anthocyanin content of Chinese bayberry fruit. Averaged data (± SE) from three independent biological replicates are shown. For each replicate, 20 fruits at early developmental stages (S1 and S2) or 5 fruits at late developmental stages (S3 and S4) were collected. Statistical analysis was performed using one-way ANOVA test and same letter indicates no significant difference (P < 0.05). FW, fresh weight.
Analysis of the bayberry genome sequence (Jia et al. 2019) revealed the presence of a MYB cluster within a span of 40.20 kb from the 27,172,068 to 27,214,638 bp region of chromosome 6 (Fig. 2A). MrMYB1.1, MrMYB2, and MrMYB1.3 occurred in tandem, with MrMYB1.2 being the product of proximal duplication. The gene, with ID KAB1211295.1, between MrMYB1.2 and MrMYB1.1 was annotated as a major latex protein-like protein. To evaluate the presence of the whole MrMYB cluster, we divided this 40.20 kb region into three overlapping fragments, i.e. fragment 1 (F1), 2 (F2), and 3 (F3). All three fragments were successfully amplified from the gDNA of all four cultivars (Supplementary Fig. S1) with primers listed in Supplementary Table S1.
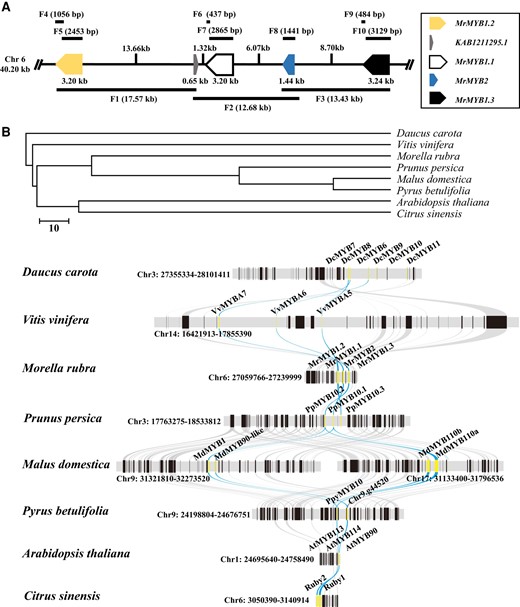
MYB gene cluster in Chinese bayberry and other eudicots. A) Physical locations of four MrMYBs in a cluster of 40.20 kb in size on chromosome 6 of the Chinese bayberry genome. B) Conserved collinear blocks at the MYB cluster loci in Chinese bayberry and other eudicots. Yellow lines represent the MYB genes in different species. Blue lines depict the collinear relationship of MYBs among various species.
The presence of all four MrMYBs was further evaluated by PCR, with primers listed in Supplementary Table S1, and sequencing of fragments 4 through 10 (Fig. 2A). For MrMYB1.1, MrMYB1.2, and MrMYB1.3, each member was cloned as two fragments (Fig. 2A) since the sequences near the start and stop codons were highly similar. All fragments were successfully amplified from four cultivars at genomic level (Supplementary Fig. S2), indicating that all four MrMYBs (Fig. 2A) were present in these four cultivars.
The genomic synteny and collinearity analysis of anthocyanin-related MYB clusters across eudicot plants were conducted (Fig. 2B). Our results showed that in most eudicots, eight of nine species with the exception of tomato (Solanum lycopersicum), MYBs located in genomic collinear regions across different species (Fig. 2B), implying the genomic conservation of anthocyanin-related MYB clusters following the divergence of eudicots.
Sequences of MrMYB cluster members in four cultivars with different ripe fruit colors
Chinese bayberry is a diploid species with a high level of heterozygosity, such as 0.56% and 0.70% for the two cultivars sequenced (Jia et al. 2019). Therefore, different alleles may present within an individual cultivar as well as between cultivars. With cloning strategies as illustrated previously (Fig. 2A), PCR analyses and sequencing were performed on four above-mentioned cultivars with different ripe fruit colors (Fig. 1A). As a result, it was found that two, three, three, and two alleles were present for MrMYB1.1, MrMYB1.2, MrMYB1.3, and MrMYB2, respectively (Table 1).
Member . | Allele . | Presence . | |||
---|---|---|---|---|---|
BQ . | DK . | FH . | SJ . | ||
MrMYB1.1 | MrMYB1.1-1 (MrMYB1.1n) | + | + | + | − |
MrMYB1.1-2 (MrMYB1.1d) | − | + | + | + | |
MrMYB1.2 | MrMYB1.2-1 | + | + | + | − |
MrMYB1.2-2 | + | − | − | − | |
MrMYB1.2-3 | − | + | + | + | |
MrMYB1.3 | MrMYB1.3-1 | + | + | + | + |
MrMYB1.3-2 | − | + | − | − | |
MrMYB1.3-3 | − | − | + | + | |
MrMYB2 | MrMYB2-1 | + | + | + | + |
MrMYB2-2 | + | − | − | − |
Member . | Allele . | Presence . | |||
---|---|---|---|---|---|
BQ . | DK . | FH . | SJ . | ||
MrMYB1.1 | MrMYB1.1-1 (MrMYB1.1n) | + | + | + | − |
MrMYB1.1-2 (MrMYB1.1d) | − | + | + | + | |
MrMYB1.2 | MrMYB1.2-1 | + | + | + | − |
MrMYB1.2-2 | + | − | − | − | |
MrMYB1.2-3 | − | + | + | + | |
MrMYB1.3 | MrMYB1.3-1 | + | + | + | + |
MrMYB1.3-2 | − | + | − | − | |
MrMYB1.3-3 | − | − | + | + | |
MrMYB2 | MrMYB2-1 | + | + | + | + |
MrMYB2-2 | + | − | − | − |
Note. “BQ”, “DK”, “FH”, and “SJ” represent “Biqi”, “Dongkui”, “Fenhong”, and “Shuijing”, respectively.
Member . | Allele . | Presence . | |||
---|---|---|---|---|---|
BQ . | DK . | FH . | SJ . | ||
MrMYB1.1 | MrMYB1.1-1 (MrMYB1.1n) | + | + | + | − |
MrMYB1.1-2 (MrMYB1.1d) | − | + | + | + | |
MrMYB1.2 | MrMYB1.2-1 | + | + | + | − |
MrMYB1.2-2 | + | − | − | − | |
MrMYB1.2-3 | − | + | + | + | |
MrMYB1.3 | MrMYB1.3-1 | + | + | + | + |
MrMYB1.3-2 | − | + | − | − | |
MrMYB1.3-3 | − | − | + | + | |
MrMYB2 | MrMYB2-1 | + | + | + | + |
MrMYB2-2 | + | − | − | − |
Member . | Allele . | Presence . | |||
---|---|---|---|---|---|
BQ . | DK . | FH . | SJ . | ||
MrMYB1.1 | MrMYB1.1-1 (MrMYB1.1n) | + | + | + | − |
MrMYB1.1-2 (MrMYB1.1d) | − | + | + | + | |
MrMYB1.2 | MrMYB1.2-1 | + | + | + | − |
MrMYB1.2-2 | + | − | − | − | |
MrMYB1.2-3 | − | + | + | + | |
MrMYB1.3 | MrMYB1.3-1 | + | + | + | + |
MrMYB1.3-2 | − | + | − | − | |
MrMYB1.3-3 | − | − | + | + | |
MrMYB2 | MrMYB2-1 | + | + | + | + |
MrMYB2-2 | + | − | − | − |
Note. “BQ”, “DK”, “FH”, and “SJ” represent “Biqi”, “Dongkui”, “Fenhong”, and “Shuijing”, respectively.
Two alleles for MrMYB1.1, MrMYB1.1-1, and MrMYB1.1-2 were observed. The former was a normal full-length allele and therefore we named it MrMYB1.1n as well, while the latter was a mutated one with a single base deletion at nucleotide 30 of CDS and thus was named MrMYB1.1d as well (Fig. 3 and Table 1). MrMYB1.1d was homozygous in “SJ” and heterozygous in “DK” and “FH”, while MrMYB1.1n was homozygous in “BQ”. The premature termination of the predicted protein of MrMYB1.1d at amino acid residue 14 prevents the synthesis of a full-length MrMYB1.1n. In silico translation analysis suggested that if a translation was initiated from the second alternative start codon (ATG*), it may encode a truncated protein lacking R2 domain and the first helix of the R3 domain (Fig. 3 and Supplementary Fig. S3). Since both R2 and R3 domains are essential for DNA-binding activity (Gabrielsen et al. 1991; Jia et al. 2004), the first two α-helices of the R3 domain are engaged in the interactions with a bHLH protein (Zimmermann et al. 2004; Lin-Wang et al. 2010), the putative truncated MrMYB1.1d may cannot bind the promoters of the anthocyanin biosynthetic/transport-related genes or interact with bHLH TFs, thus, may lose function in regulating anthocyanin biosynthesis/accumulation.
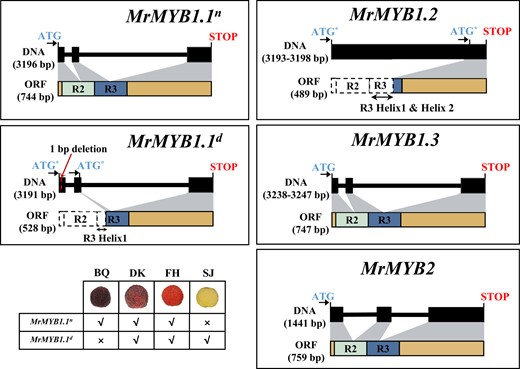
Schematic diagram showing the DNA and protein sequences of four MrMYBs as well as two MrMYB1.1 alleles in Chinese bayberry. “BQ”, “DK”, “FH”, and “SJ” represent “Biqi”, “Dongkui”, “Fenhong”, and “Shuijing”, respectively. ATG* stands for alternative start codon.
Three alleles were observed for MrMYB1.2 (Table 1 and Supplementary Fig. S3). The size of these three alleles of MrMYB1.2 transcript was similar to MrMYB1.1; however, unlike MrMYB1.1, the regions corresponding to two introns were not spliced. In silico translation analysis suggested that these three alleles may encode a same MYB protein with only part of the R3 domain (Fig. 3 and Supplementary Fig. S3), and thus may also lose function in regulating anthocyanin biosynthesis/accumulation.
MrMYB1.3 had three alleles, with deduced amino acid sequences possessing complete R2 and R3 domains. The deduced amino acid sequences of MrMYB1.3-2 and MrMYB1.3-3 were consistent, and with seven amino acid differences from MrMYB1.3-1, but all these differences occurred outside of the helices or the bHLH binding motif (Table 1 and Supplementary Fig. S3).
MrMYB2 had two alleles, with deduced amino acid sequences of both possessing complete R2 and R3 domains. Two amino acid residue differences were observed but none of them were located in critical motifs (Table 1 and Supplementary Fig. S3).
Phylogenetic analysis of deduced amino acid sequences showed that the predicted MrMYB proteins were clustered with other plant MYB TFs that have been reported to activate anthocyanin biosynthesis (Supplementary Fig. S4 and Table S2). Their roles in regulating anthocyanin biosynthesis/accumulation need to be further investigated.
Development of cleaved amplified polymorphic sequence markers for discrimination of MrMYB1.1n and MrMYB1.1d sequences
Since MrMYB1.1n is functional as characterized previously (Niu et al 2010; Huang et al 2013), while MrMYB1.1d was nonfunctional as predicted, a tool for measuring the presence and expression of these two alleles in “DK” and “FH” needs to be established. For this purpose, several methods were tried, such as TaqMan probe-based assay and allele-specific PCR, which were unsuccessful. As the single deletion in MrMYB1.1d removes one Hha I recognition site (5′-GCGC-3′; Fig. 4A), cleaved amplified polymorphic sequence (CAPS) markers can be developed based on the MrMYB1.1n/MrMYB1.1d polymorphism to discriminate three different genotypes, i.e. MrMYB1.1n/MrMYB1.1n, MrMYB1.1n/MrMYB1.1d, and MrMYB1.1d/MrMYB1.1d at genomic and transcript levels.
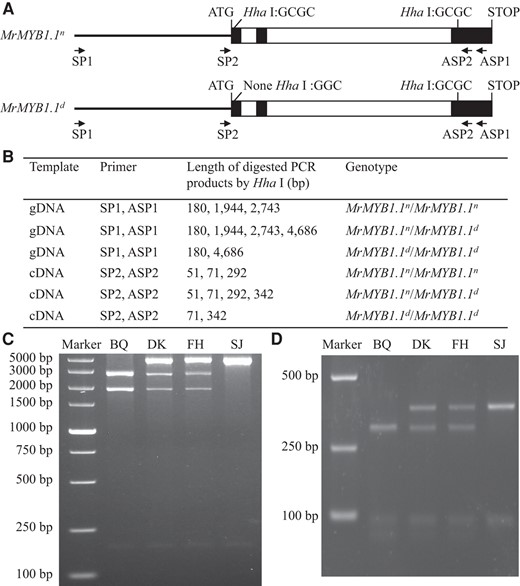
Genotyping of MrMYB1.1 using CAPS markers. A) Schematic diagram of CAPS markers for gDNA and cDNA genotyping. Black lines represent promoters. Solid black boxes represent exons. Hollow black boxes represent introns. B) Basic information on CAPS markers for gDNA and cDNA genotyping. C and D) CAPS genotyping of four cultivars at genomic (C) and transcript (D) levels. “BQ”, “DK”, “FH”, and “SJ” represent “Biqi”, “Dongkui”, “Fenhong”, and “Shuijing”, respectively.
At genomic level, we designed an upstream primer (SP1) at 1,912 bp before start codon of MrMYB1.1n/MrMYB1.1d and downstream primer (ASP1) near the stop codon. The length of PCR product was 4,867/4,866 bp. After Hha Ⅰ digestion, the MrMYB1.1n genotype produced three bands: 2,743, 1,944, and 180 bp, while MrMYB1.1d had two bands: 4,687 and 180 bp (Fig. 4, B and C). Four cultivars with different ripe fruit colors were selected for cloning and verification, where it was found that “BQ” was homologous for MrMYB1.1n, “SJ” was homologous for MrMYB1.1d, while “DK” and “FH” had both alleles (Fig. 4C). The results were consistent with those from direct sequencing of PCR products (Fig. 3 and Supplementary Fig. S5).
At transcript level, it was difficult to design specific primers due to the high sequence similarity between MrMYB1.1 and MrMYB1.2. However, owing to the length difference of their transcript sequences, the PCR products, from amplification with primers SP2 and ASP2, of these two members can be easily discriminated, with 414/413 bp for MrMYB1.1n/MrMYB1.1d and 2,865 bp for MrMYB1.2 (Supplementary Fig. S6). The 414/413 bp band was recovered from agarose gel and digested with Hha Ⅰ, and the MrMYB1.1n genotype had three bands: 292, 71, and 51 bp, while MrMYB1.1d had two: 342 and 71 bp (Fig. 4, B and D). Four cultivars with different ripe fruit colors were selected for cloning and verification, and it was found that “BQ” had only transcript of MrMYB1.1n, “SJ” had only MrMYB1.1d, while “DK” and “FH” had both (Fig. 4D). The results were consistent with those from direct cloning sequencing results (Fig. 3 and Supplementary Fig. S7).
Differential expression patterns of the MrMYB cluster members in four cultivars with different ripe fruit colors
The transcript levels of the MrMYB cluster were analyzed in fruit of four cultivars at different developmental stages. Because of the high transcript sequence similarity between members and especially the alleles of each MrMYB, it was not feasible to design allele-specific qPCR primers. The qPCR primers used (Supplementary Fig. S8) could not discriminate alleles. Therefore, the expression was the sum of both alleles for the members with two alleles inside an individual cultivar (Fig. 5A). Expression of four MrMYBs in the early fruit developmental stages (S1 and S2) of four cultivars was either undetectable or quite weak. Expression of MrMYB1.1, MrMYB1.2, and MrMYB2 in later fruit developmental stages (S3 and S4) was higher than those in early stages and at similar abundance in four cultivars. MrMYB1.3 exclusively expressed in “BQ” and the transcript was undetectable in other three cultivars (Fig. 5A).

Expression of MrMYB cluster members in Chinese bayberry fruit. A) Relative expression of four MrMYBs during fruit development of four Chinese bayberry cultivars. Averaged data (± SE) from three independent biological replicates are shown. For each replicate, 20 fruits at early developmental stages (S1 and S2) or 5 fruits at late developmental stages (S3 and S4) were collected. Statistical analysis is based on simultaneous comparisons of the four developmental stages for individual members, and was performed using one-way ANOVA test. The same letter indicates no significant difference (P < 0.05). B and C)MrMYB1.1 genotyping of “DK” (B) and “FH” (C) cDNA using CAPS. “BQ”, “DK”, “FH”, and “SJ” represent “Biqi”, “Dongkui”, “Fenhong”, and “Shuijing”, respectively. S1 (stage 1), S2, S3, and S4 represent 60 DAFB, 70 DAFB, 74 DAFB, and 86 DAFB, respectively.
The expression levels of MrMYB1.1n and MrMYB1.1d were discriminated using the developed CAPS marker via determining the distribution of MrMYB1.1n/MrMYB1.1d among MrMYB1.1 PCR products. For the two cultivars with both alleles, “DK” and “FH”, the PCR products from the amplification with primers SP2 and ASP2, a mixture of MrMYB1.1n and MrMYB1.1d, were ligated into a cloning vector and transformed into Escherichia coli. Transformed colonies for each were randomly picked, amplified with CAPS marker primers SP2 and ASP2, and the products from individual plasmids containing MrMYB1.1n/MrMYB1.1d, with MrMYB1.2 excluded, were digested with Hha I. The digestion results of PCR products of 12 single colonies of “DK” and “FH” were shown in Fig. 5, B and C, respectively. The counts of MrMYB1.1n and MrMYB1.1d in “DK” transcripts were 5 and 7, respectively, and in “FH” transcripts, the counts were 6 and 6, respectively, suggesting that both alleles were expressed unbiasedly in “DK” and “FH”.
Association of MrMYB1.1 genotype and MrMYB cluster member expression with fruit color in other cultivars
Association of fruit color with MrMYB1.1 genotype and the expression of MrMYB cluster members were analyzed in further 10 cultivars with the assistance of the developed CAPS markers (Fig. 6). Anthocyanin contents matched well ripe fruit color (Fig. 6A). Genotyping results were consistent at genomic and transcript levels (Fig. 6, B and C; and Supplementary Figs. S5 and S7). Dark red cultivars (Fig. 6A) were homozygous for MrMYB1.1n, the red cultivar “Luozi” and pink cultivar “Dayezhong” were heterozygous for MrMYB1.1n/MrMYB1.1d, and the white cultivar “Longhaishuijing” was homozygous for MrMYB1.1d (Fig. 6, B and C; and Supplementary Figs. S5 and S7).
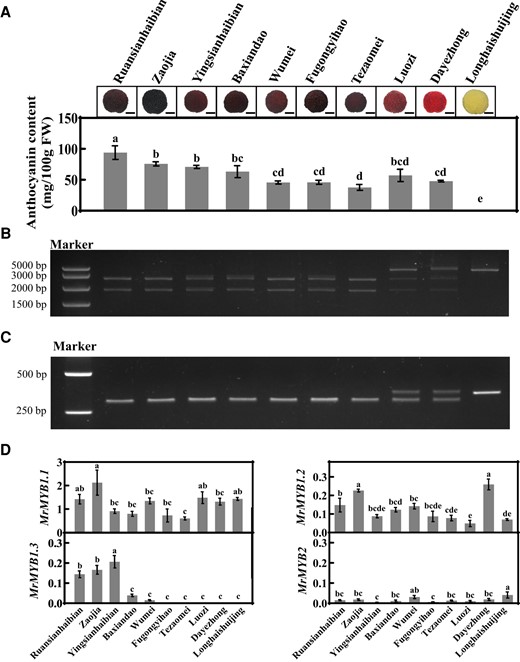
MrMYB cluster members in 10 Chinese bayberry cultivars with different ripe fruit colors. A) Anthocyanin content in ripe fruit. FW, fresh weight. Bar, 1 cm. B and C)MrMYB1.1 genotyping at genomic (B) and transcript (C) levels using CAPS. D) Relative expression of MrMYB cluster members in ripe fruit. Averaged data (± SE) from three independent biological replicates, with five fruits for each replicate, are shown. Statistical analysis was performed using one-way ANOVA test and same letter indicates no significant difference (P < 0.05).
MrMYB1.1 showed the highest expression levels among the four MrMYBs, which was around 10 times of that for MrMYB1.2 and MrMYB1.3, while the expression of MrMYB2 was lowest. MrMYB1.3 was only expressed in some dark red fruit cultivars to different extents (Fig. 6D). The CAPS genotyping at the transcript level of the red cultivar “Luozi” and pink cultivar “Dayezhong” showed that expression levels of MrMYB1.1n and MrMYB1.1d were comparable (Supplementary Fig. S9), as was “DK” and “FH” (Fig. 4, C and D). This further validated the close association of the MrMYB1.1 genotype as well as expression of MrMYB1.1 and MrMYB1.3 to the color of ripe fruit in different cultivars.
Functional characterization of MrMYB cluster members in tobacco (N. tabacum)
Function of MrMYB cluster members in regulating anthocyanin accumulation was assessed by transient and stable expressions in tobacco (N. tabacum; Fig. 7). It was found that MrMYB1.1n could induce anthocyanin accumulation, and a much more intense pigmentation was detected when co-infiltrated with MrbHLH1 (Fig. 7, A and B). However, MrMYB1.1d could neither induce anthocyanin accumulation, with or without MrbHLH1, nor repress anthocyanin accumulation when co-infiltrated with MrMYB1.1n (Supplementary Fig. S10). This indicated that the deletion of a single base resulted in loss of function, but did not promote a negative regulatory function.

Transient expression and stable transformation of MrMYBs in tobacco (N. tabacum). A) Digital images of tobacco leaves taken at 3 d after infiltration. Images were digitally extracted for comparison. B and C) Total anthocyanin content (B) and relative expression of anthocyanin-related genes (C) of leaf tissues at infiltration sites. Anthocyanins are quantified as cyanidin-3-O-glucoside (C3G) equivalents. Three independent biological replicates, with each replicate including three leaves taken from one plant, were performed. The experiments were repeated three times independently, with similar results. D) Phenotypes of transgenic tobacco full-boom flower and young fruit overexpressing MrMYB1.3-1. Images were digitally extracted for comparison. E and F) Total anthocyanin content (E) and expression (F) of MrMYB1.3 in transgenic tobacco petals and pericarps. Averaged data (± SE) from three independent biological replicates, with each replicate including one transgenic line, are shown. Statistical analysis was performed using one-way ANOVA test and same letter indicates no significant difference (P < 0.05). FW, fresh weight. WT, wild type.
Deduced amino acid sequences of all three MrMYB1.2 alleles were identical, losing complete R2 and partial R3 domain (Fig. 3 and Supplementary Fig. S3). Ectopic transient expression in tobacco (N. tabacum) leaves showed that MrMYB1.2-1 could not induce red patches (Fig. 7A).
Deduced amino acid sequences of all three MrMYB1.3 alleles had complete R2 and R3 domains (Fig. 3 and Supplementary Fig. S3). There were seven deduced amino acid differences among alleles but all these occurred outside of the helices or the bHLH binding motif (Supplementary Fig. S3). In addition, MrMYB1.3-1 was the only allele expressed in “BQ” (Fig. 5 and Table 1). MrMYB1.3-1 was selected for subsequent transient expression analysis and an induction of anthocyanin accumulation was observed (Fig. 7A). Similar to MrMYB1.1n, the anthocyanin accumulation was much stronger in MrMYB1.3-1 + MrbHLH1 injected areas than MrMYB1.3-1 alone (Fig. 7, A and B).
Deduced amino acid sequences of two MrMYB2 alleles had complete R2 and R3 domains; a difference of two amino acid residues was observed but none of them located in critical motifs (Supplementary Fig. S3). The results of ectopic transient expression in tobacco (N. tabacum) leaves showed that MrMYB2-1 could not induce red patches (Fig. 7, A and B).
We quantified the transcript levels of several tobacco (N. tabacum) genes encoding enzymes of the anthocyanin biosynthesis pathway, including NtCHS, NtCHI, NtF3H, NtF3′H, NtDFR1, NtANS, and NtUFGT in the injected areas of tobacco (N. tabacum) leaves (Fig. 7C). These genes were expressed at higher levels in MrMYB1.1n and MrMYB1.3-1 injected areas, and further increased in co-infiltrations included MrbHLH1. These results indicated that MrMYB1.1n and MrMYB1.3-1 could activate endogenous pathway genes of tobacco (N. tabacum) to facilitate anthocyanin production.
The function of MrMYB1.1n in promoting anthocyanin accumulation has previously been verified via stable transformation of tobacco (N. tabacum; Huang et al. 2013). Here the function of MrMYB1.3-1 was evaluated. Compared to the wild type (WT), three lines of 35S::MrMYB1.3-1 transgenic plants displayed dark red color on the petals and showed a purple pigmentation of the pericarp, whereas the pericarp of the WT plants was white (Fig. 7D). The contents of anthocyanin of the petals and pericarps were higher in transgenic plants than those in WT (Fig. 7E). Reverse transcription quantitative PCR (RT-qPCR) experiments showed that expression levels of MrMYB1.3 were higher in transgenic lines than those in WT (Fig. 7F).
Roles of MrMYB cluster members in activation of the expression of anthocyanin biosynthesis/transport genes of Chinese bayberry
The effects of MrMYB cluster members on activating the transcription of anthocyanin pathway/transport genes of bayberry, alone or in combination with MrbHLH1, were investigated by conducting a dual-luciferase (LUC) assay (Fig. 8A). We performed activation experiments on the promoters of anthocyanin pathway genes MrCHS, MrCHI, MrF3H, MrF3′H, MrDFR1, MrANS, and MrUFGT, as well as the anthocyanin transporter gene MrGST1. MrMYB1.1n and MrMYB1.3-1 alone can significantly induce MrCHI, MrUFGT, and MrGST1 promoters, by 7–18-fold, and the induction fold was nearly doubled when MrMYB1.1n or MrMYB1.3-1 was coupled with MrbHLH1. The activities of MrF3′H, MrDFR1, and MrANS promoters were significantly induced by MrMYB1.1n or MrMYB1.3-1 when coupled with MrbHLH1, by 21–126-fold for the former and 23–143-fold for the latter (Fig. 8A). MrMYB1.2-1, alone or in combination with MrbHLH1, could not induce the promoter of MrCHI, MrDFR1, and MrUFGT (Fig. 8A). The activation fold of the promoters of MrCHI, MrF3′H, MrDFR1, MrANS, MrUFGT, and MrGST1 were proportional to the amount of Agrobacterium tumefaciens harboring MrMYB1.1n injected, which was not inhibited by the addition of A. tumefaciens harboring MrMYB1.1d (Supplementary Fig. S11). Neither MrCHS nor MrF3H can be activated by MrMYB cluster members in the presence or absence of MrbHLH1 (Supplementary Fig. S12).
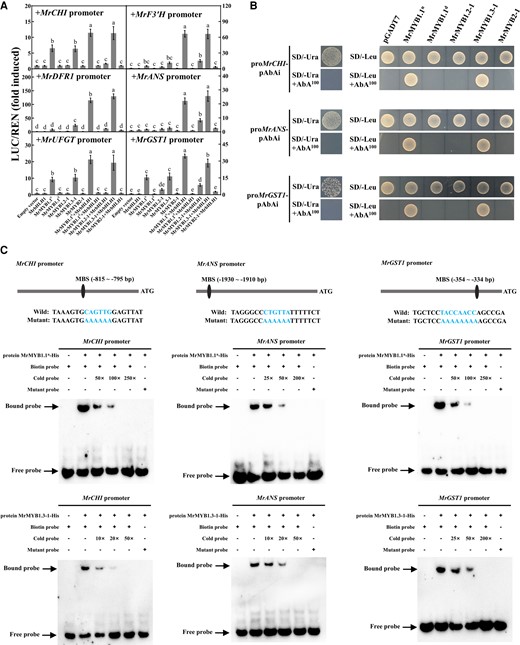
Interaction of MrMYB cluster members with the promoters of anthocyanin biosynthetic/transport-related genes. A) Effects of MrMYB cluster members, alone or in combination with MrbHLH1, on the promoter activity of anthocyanin biosynthetic/transport-related genes measured by dual-luciferase assays. Averaged data (± SE) from three independent biological replicates, with each replicate including six leaf discs (6 mm in diameter) taken from one plant, are shown. Statistical analysis was performed using one-way ANOVA test and same letter indicates no significant difference (P < 0.05). B) Yeast one-hybrid assays on the interactions of MrMYB cluster members with the promoters of MrCHI, MrANS, and MrGST1. C) EMSA showing the binding of MrMYB1.1n and MrMYB1.3-1 to the promoters of MrCHI, MrANS, and MrGST1.
We performed yeast one-hybrid assays to determine whether MrMYB cluster members can bind directly to the promoters of anthocyanin biosynthesis/transport-related genes. Results showed that MrMYB1.1n and MrMYB1.3-1 physically bind to the MrCHI, MrANS, and MrGST1 promoters (Fig. 8B). The interactions were further confirmed by electrophoretic mobility shift assay (EMSA). As presented in Fig. 8C, with the increase in the cold probe concentration, the binding of MrMYB1.1n and MrMYB1.3-1 to the MrCHI, MrANS, and MrGST1 promoters weakened, while the mutant probe lost the binding ability, indicating that MrMYB1.1n and MrMYB1.3-1 could bind at MYB binding sites (MBSs) specifically (Fig. 8C and Supplementary Fig. S13). Therefore, MrMYB1.1n and MrMYB1.3-1 can directly bind and activate the promoters of anthocyanin-related genes to regulate anthocyanin accumulation in bayberry.
Interaction between MrMYB cluster members and MrbHLH1 in vitro and in vivo
To test the interaction between MrMYB cluster members and the bHLH partner MrbHLH1, yeast two-hybrid (Y2H) and firefly luciferase complementation imaging (LCI) assays were performed. On the synthetic dextrose (SD)/-Trp medium containing X-α-Gal and 200 ng/mL aureobasidin A (AbA), there was no auto-activation of MrbHLH1-pGBKT7 vector. Physical interactions between either of MrMYB1.1n, MrMYB1.3-1, and MrMYB2-1 with MrbHLH1 were observed as evidenced by the growth and blue pigmentation of these three co-transformants on SD/-Trp-Leu-His-Ade (QDO, quadruple dropout supplements) medium containing AbA and X-α-Gal (Fig. 9A).
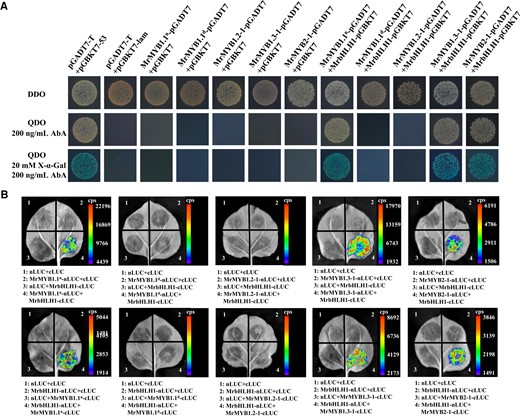
Interaction of MrMYB cluster members with MrbHLH1. A) Yeast two-hybrid assays revealed the interactions of MrMYB cluster members with MrbHLH1. The DDO (double dropout supplements) medium (SD/-Leu/-Trp) and QDO (quadruple dropout supplements) medium (SD/-Trp/-Leu/-His/-Ade) were used as selective media. AbA, aureobasidin. B) Firefly LCI on the direct interactions of MrMYB cluster members with MrbHLH1 in N. benthamiana leaves. Three independent biological replicates, with each replicate including three leaves taken from one plant, were performed. The experiments were repeated three times independently with similar results.
The interaction between MrMYB1.1n/MrMYB1.3-1/MrMYB2-1 and MrbHLH1 was also validated by an in vivo interaction experiment utilizing LCI. High fluorescence intensity (cps) was observed in areas injected with MrMYB1.1n + MrbHLH1, MrMYB1.3-1 + MrbHLH1, and MrMYB2-1 + MrbHLH1 (Fig. 9B). These results indicated that both MrMYB1.1n, MrMYB1.3 and MrMYB2, interacted with MrbHLH1 in vivo, although MrMYB2 was verified to be unable to induce anthocyanin accumulation.
Discussion
Multiple MYB members controlling anthocyanin accumulation in plants originate via both whole genome duplication and tandem duplication
The earliest MYB identified in plants to stimulate anthocyanin synthesis is ZmC1, found in monocot corn (Zea mays; Paz-Ares et al. 1987). Subsequently, an increasing number of MYBs activating anthocyanin accumulation in plants was reported (e.g. Allan et al. 2007; Jaakola 2013; Allan and Espley 2018). In 1993 in petunia, Quattrocchio et al. (1993) found that at least four MYB members are involved in regulation of anthocyanin accumulation. After that, multiple MYBs controlling anthocyanin accumulation in a single plant species are frequently reported, such as carrot (Daucus carota; Xu et al. 2019), grape (Matus et al 2017; Xie et al. 2020; Jiu et al. 2021), peach (P. persica; Zhou et al. 2014; Tuan et al. 2015), apple (Malus domestica; Chagné et al. 2013; Sun et al. 2021), pear (Pyrus betulifolia; Pierantoni et al. 2010; Yao et al. 2017), Arabidopsis (Zimmermann et al. 2004), citrus (Huang et al. 2018), and tomato (Sun et al. 2019; Yan et al. 2019). However, in the pre-genomics era, the precise origin and evolutionary relationships of these MYBs within a single species or across multiple species remain uncertain.
The advancement of high-throughput sequencing technology has facilitated the analysis of genomes. The emergence of multiple anthocyanin-related MYBs has been observed as a consequence of both whole genome duplication (WGD) and tandem duplication events in some cases, such as four MYBs involved in regulation of anthocyanin accumulation in apple, i.e. MdMYB10 (with aliases MdMYB1 and MdMYBA), MdMYB90-like, MdMYB110a, and MdMYB110b, with the former two located tandemly at chromosome 9 and the latter two at chromosome 17 (Lin-Wang et al. 2010; Chagné et al. 2013; Daccord et al. 2017; Sun et al. 2021). However, in peach, PpMYB10.4, PpMYB10.5, and PpMYB10.6 on chromosome 6, and PpMYB10.1, PpMYB10.2, and PpMYB10.3 on chromosome 3 were not a result of WGD (Verde et al. 2013). Zhou et al. (2014) hypothesized that both MYB clusters in peach originated from the putative Rosaceae ancestor, but one of the tandem clusters was lost in the apple genome after apple and peach diverged, while the other cluster originated from the apple genome through WGD.
We performed synteny analysis among different plants with multiple MYBs reported pair-by-pair. Intriguingly, extensive collinearity analysis showed that anthocyanin-associated MYB clusters occurred in highly conserved regions of the genome of many eudicots. However, it is not clear whether the MYB tandem clusters predated the divergence of eudicots or underwent convergent evolution by independent tandem duplication. Future studies to more completely understand the genesis of the MYB gene clusters linked with anthocyanins can be feasible with the availability of more accurate genomic data and more reliable methodologies.
Expression differentiation and function diversification of tandem MYB clusters coordinately regulate anthocyanin accumulation
Gene duplication results in an increase in gene numbers, which then experience distinct evolutionary trajectories in response to selective pressure (Pennisi 2012; Panchy et al. 2016). Some repeated genes retain the original function, while others undergo subfunctionalization, neofunctionalization, or nonfunctionalization (Panchy et al. 2016; Qiao et al. 2019). Most of the plants reported to possess the MYB tandem clusters in relation to anthocyanin accumulation have several active MYBs with expression differentiation or function diversification.
In citrus, Huang et al. (2018) found that anthocyanins accumulate in the peel and young leaves of “purple pummelo”, a wild species, owing to the co-activation of Ruby1 and its tandem duplication, Ruby2. During citrus domestication, Ruby2 is converted into a negative regulator to suppress anthocyanin accumulation in both wild and domesticated species, whereas Ruby1 maintains its role as an activator of anthocyanin accumulation in wild species but is rendered inactive in most cultivated species. Therefore, the Ruby2–Ruby1 cluster subfunctionalization during domestication reflects the outcome of human selection and domestication (Huang et al. 2018). In grape, VvMYBA1 and VvMYBA2 located on chromosome 2 govern the synthesis of anthocyanins in berries, while VvMYBA1 on chromosome 2 and VvMYBA5, VvMYBA6, and VvMYBA7 located on chromosome 14 are primarily responsible for the accumulation of anthocyanins in vegetative tissues (Matus et al. 2017; Xie et al. 2020). Both an insertion of a retrotransposon into the promoter region of VvMYBA1 and two single nucleotide polymorphism (SNP) alterations in the coding region of VvMYBA2 were essential for impairment in anthocyanin accumulation in white grape berries (Kobayashi et al. 2004; Walker et al. 2007; Jiu et al. 2021). In carrot, DcMYB6 and DcMYB7, from a tandem cluster, regulate anthocyanin production in purple inner tissues and root outer phloem, respectively (Xu et al. 2017; Iorizzo et al. 2019). In peach, anthocyanin accumulation in the peel and flesh is mainly governed by PpMYB10.1 (Ravaglia et al. 2013), while PpMYB10.2 and PpMYB10.4 are responsible for the regulation of anthocyanin accumulation in flowers and leaves, respectively (Zhou et al. 2014, 2016).
Our study showed that MrMYB1.1n and MrMYB1.3 acted as the only anthocyanin activators in Chinese bayberry tandem gene cluster (Figs. 7–9). Differential accumulation of anthocyanin in ripe fruits among cultivars was closely associated with both MrMYB1.1 genotype and transcript abundance, as well as the expression of MrMYB1.3 found in some dark red cultivars (Figs. 5 and 6). The reason why MrMYB1.3 was not expressed in cultivars with low fruit anthocyanin content was not clear and worthy of future investigation. On the other hand, MrMYB1.1d and MrMYB1.2 cannot promote anthocyanin accumulation due to mutation or non-splicing of transcripts (Figs. 3, 7–9 and Supplementary Fig. S3), suggesting that they were diverted into nonfunctional genes. Surprisingly, although MrMYB2 had a complete R2R3 domain, clustered with MYBs that promote anthocyanin accumulation in other species, and interacted with MrbHLH1 (Figs. 2 and 9; and Supplementary Figs. S3 and S4), transient overexpression in tobacco (N. tabacum) leaves failed to induce anthocyanin accumulation (Fig. 7). It is possible that MrMYB2 may play roles in manipulating biosynthesis of other compounds.
Another interesting fact we noticed is that for promoters of some structural genes such as MrCHI and MrGST1, MrMYB1.1n and MrMYB1.3 can directly bind and activate them, though stronger activation effects were observed when MrbHLH1 was present (Fig. 8). However, for promoters of other genes such as MrANS, MrMYB1.1n and MrMYB1.3 can directly bind but fail to activate until under the assistance of MrbHLH1 (Fig. 8). This phenomenon has also previously been reported in other plants. For example, in Arabidopsis, the expression of the structural gene AtDFR cannot be activated by Production of Anthocyanin Pigment 1 (AtPAP1) or AtPAP2 alone, but can be substantially activated by either MYB member when coupled with bHLH protein AtTT8 (Zimmermann et al. 2004). Similarly, in pear, the promoter of PyANS was not activated by PyMYB10 alone but can be stimulated by around six times by PyMYB10 in coupling with PybHLH3 (Yao et al. 2017). In crape myrtle, LfiMYB75 directly bind to the promoters of LfiCHS, LfiANS, as well as LfiF3′H, and substantially activate the promoters of the former two but not LfiF3′H. However, when coupled with LfibHLH1, the LfiF3′H promoter was activated by seven times (Feng et al. 2023). The reasons for the issue of differential activation effects on different promoters by a same MYB might be attributed to structural variation owing to the sequence difference, especially from MBSs to transcription initiation site, among different structural genes. Furthermore, such structural variation might be changed following the addition of a bHLH partner in a way, with detail unveiled, of facilitating the activation of structural genes.
Allele discrimination is important for studies involving plants with high heterozygous backgrounds
Allelic variation, both across cultivars and within individuals, is prevalent in highly heterozygous species and is one of the most important factors contributing to the species diversity. In recent years, there has been a growing emphasis in anthocyanin research on the examination of alleles. For example, the allele sequence variations of MYB10 in different species of genus Fragaria leads to great color variation in strawberries (Castillejo et al. 2020). The inability of the majority of citrus plants to accumulate anthocyanins may be explained by disruptions of both two Ruby1 alleles, including point mutations, deletions, and insertions of transposable elements (Butelli et al. 2017). Similarly, simultaneous occurrence of homozygous nonfunctional alleles of two genes, VvMYBA2w and VvmybA1a, produces white berries in grape (Jiu et al. 2021).
Several techniques have been developed to discriminate alleles. For example, to identify the MYB10 allele responsible for color variation in strawberry (Fragaria vesca), Castillejo et al. (2020) developed high-resolution melting and “kompetitive allele specific PCR” markers. Distinguishing alleles becomes more challenging when the focus of allele investigation is directed toward tandemly repeated genes. In bayberry, the established CAPS markers targeting MrMYB1.1n/MrMYB1.1d exhibit the ability to quickly distinguish cultivars with different colored ripe fruits (Fig. 5 and Supplementary Figs. S5 and S7). This study provides a reference for similar work involving the discrimination of alleles under the background of tandemly duplicated members. With increasing accumulation of SNP data from second- and third-generation sequencing technology, as well as availability of several molecular markers based on SNPs, allele discrimination and characterization can be expected to be involved in more studies on plants with highly heterozygous backgrounds.
Conclusions
The anthocyanin-related MYBs in Chinese bayberry exist as a tandem cluster of four members, with function and expression diversified. Because of high heterozygosity, a feature of perennial fruit crops, the MYB members in Chinese bayberry come as alleles not only between cultivars but within individual cultivar as well. MrMYB1.1-1 (MrMYB1.1n) is functional on activation of anthocyanin biosynthesis and transport genes while MrMYB1.1-2 (MrMYB1.1d), an allele with a single base deletion, is not. MrMYB1.1n is homozygous in dark red fruit cultivars and MrMYB1.1d is homozygous in white fruit cultivars while MrMYB1.1n/MrMYB1.1d are heterozygous in red/pink fruit cultivars. MrMYB1.3-1 is functional and its expression is restricted to some dark red cultivars exclusively. In conclusion, in Chinese bayberry, anthocyanin-related MYBs were tandemly duplicated and then diversified, in function and expression pattern, as well as allele-specifically mutated, contributing to the differential anthocyanin accumulation and ripe fruit color variation among cultivars.
Materials and methods
Plant material
Fourteen Chinese bayberry (M. rubra Sieb. et Zucc.) cultivars were involved in this study with names and color of ripe fruit indicated in Figs. 1A and 6A. Young leaves and fruit were sampled from commercial orchards located in Zhejiang Province and the Chinese bayberry germplasm repository in Fruit Research Institute of Fujian Academy of Agriculture Science, China. Fruit of BQ, DK, FH, and SJ were sampled at stage 1 (S1), S2, S3, and S4, representing 60, 70, 74, and 86 days after full-bloom (DAFB), respectively, as shown in Fig. 1A, and those of the other 10 cultivars were picked at full maturity (Fig. 6A). For fruit, three biological replicates were collected, with each replicate including 20 fruits at early developmental stages (S1 and S2) or five fruit at late developmental stages (S3 and S4 or full maturity). All samples were instantly frozen in liquid nitrogen and kept at −80 °C until use.
Anthocyanin extraction and high-performance liquid chromatography analysis
Anthocyanin extraction was carried out as reported previously (Zhao et al. 2017). Briefly, powdered samples were extracted with 0.05% (v/v) HCl in methanol, and supernatants were combined and dried at 30 °C using a rotary evaporator. The residue was dissolved with 1 M HCl in methanol and filtered through a 0.22 nm Millipore membrane. Using a Waters Alliance 2695 system (Waters Corp., United States) with a reverse-phase C18 column (4.6 × 250 mm, 5 μm; Waters Corp., United States), high-performance liquid chromatography analysis was conducted with conditions as specified by Cheng et al. (2014). The anthocyanin cyanidin 3-O-glucoside (C3G), which makes up to 97% of bayberry's total anthocyanins (Fang et al. 2006), was quantified by comparing with its authentic standard.
gDNA and total RNA extraction and cDNA synthesis
gDNA was extracted from young bayberry leaves using the Plant Genomic DNA Kit (TIANGEN, Beijing, China) following manufacturer's protocols. Total RNA was isolated from bayberry fruits following a modified cetyltrimethylammonium bromide approach (Chang et al. 1993) and from tobacco (N. tabacum) tissues using the TRIzol Reagent Kit (Ambion, Hopkinton, MA, United States). Subsequently, TransScript One-Step gDNA Removal and cDNA Synthesis SuperMix (TransGen, Beijing, China) were used to remove gDNA contamination from total RNA and to synthesize the first-strand cDNA according to the manufacturer's instructions.
Gene cloning
To confirm the authenticity of the MYB cluster, 2× Vazyme LAmp Master Mix (Dye Plus; Vazyme, Nanjing, China) was used to amplify the long genomic fragments. Genomic and transcript sequence cloning were performed using Phanta Max Super-Fidelity DNA Polymerase (Vazyme, Nanjing, China) with gDNA and cDNA as templates, respectively. Primer information is given in Supplementary Table S1.
MrMYB1.1 genotyping by CAPS
CAPS markers were designed to distinguish the genotypes of MrMYB1.1 at the genomic and transcript levels. PCR was conducted with primers SP1 and ASP1 for genomic, as well as SP2 and ASP2 for transcript amplification. Primer information is given in Supplementary Table S1. The PCR products were separated by electrophoresis on 1% (w/v) agarose gel, and the band with the correct size, 414/413 bp, was excised and the DNA was recovered with MiniBEST Agarose Gel DNA Extraction Kit Ver.4.0 (TAKARA, Beijing, China) to exclude the interference of MrMYB1.2. The purified PCR products were subsequently digested with Hha I (Thermo scientific) for 2 h at 37 °C. The digestion products were separated by electrophoresis on 1% (w/v) agarose gel stained with ethidium bromide and photos taken under ultraviolet light.
In order to distinguish the occurrence of MrMYB1.1n/MrMYB1.1d at the transcript level in heterozygous cultivars, PCR products were ligated to pEASY-Blunt Simple Cloning Vector (TIANGEN, Beijing, China), and after transformation in E. coli, 12 single colonies were randomly selected and subjected to PCR, Hha I digestion, and electrophoresis.
RT-qPCR analysis
RT-qPCR was performed using ChamQ Universal SYBR qPCR Master Mix (Vazyme, Nanjing, China) in a CFX96 equipment (Bio-Rad, CA, United States) according to the manufacturer's instructions. Actin (ACT) genes, MrACT (GenBank accession number GQ340770) and NtACT (GenBank accession number AJ421411), were utilized as internal controls for normalizing the expression of bayberry and tobacco (N. tabacum) target genes, respectively. Primer information is given in Supplementary Table S3. For each sample, quantifications were made in triplicate. The RT-qPCR data were analyzed using the 2−ΔCt analysis method.
Transient ectopic expression in tobacco (N. tabacum) leaves
The full-length mRNA sequences of MrMYB1.1n, MrMYB1.1d, MrMYB1.2-1, MrMYB1.3-1, MrMYB2-1, and MrbHLH1 (from “BQ”) were separately cloned into the pGreenII 62-SK vectors. Information for the primers involved is given in Supplementary Table S1. Transient ectopic expression tests were performed in tobacco (N. tabacum) leaves via A. tumefaciens infiltration (EHA105) using the previously described approach (Sainsbury et al. 2009). Three biological replicates, each consisting of three leaves taken from one tobacco (N. tabacum) plant, were set for transient expression analysis. The experiments were repeated three times independently. The infiltrated plants were placed in a growth room (25 °C, 75% humidity, 16 h light and 8 h dark) for phenotypic identification captured digitally one week after infiltration.
Stable transformation in tobacco (N. tabacum)
The full-length mRNA sequences of MrMYB1.3-1 were cloned into the pGreenII 62-SK vectors and transformed into A. tumefaciens strain EHA105. Information for the primers involved is given in Supplementary Table S1. Stable transformation in tobacco (N. tabacum) was performed using a leaf disc co-cultivation method (Horsch et al. 1985). T0 and T1 transgenic plants were screened on selected medium for kanamycin resistance, transplanted to soil, and kept in a growth room (25 °C, 75% humidity, 16 h light and 8 h dark). The petal of full-bloom flower and the pericarp were sampled for subsequent experiments. Three tobacco (N. tabacum) transgenic lines were served as three biological replicates.
Dual-luciferase assay
The promoters of MrCHS, MrCHI, MrF3H, MrF3′H, MrANS, MrDFR1, MrUFGT, and MrGST1, with fragments 2,000, 1,466, 2,000, 598, 2,000, 1,981, 2,000, and 2,229 bp upstream of the predicted start codon, respectively, were introduced into pGreenII 0800-LUC vector. All promoter sequences were cloned from gDNA of “BQ”. Primer information is given in Supplementary Table S1. Following a previously described approach, dual-luciferase experiments were conducted on Nicotiana benthamiana leaves (Zeng et al. 2015). Three biological replicates, each consisting of six leaf discs (6 mm in diameter) taken from one plant, were set for dual-luciferase assays.
Yeast one-hybrid assay
The promoters, all from “BQ”, of MrCHI, MrF3′H, MrANS, MrDFR1, MrUFGT, and MrGST1, with fragments from 1,301, 598, 2,000, 1,447, 2,000, and 2,229 bp upstream of the predicted start codon, respectively, were cloned into pAbAi vector. The CDS regions of MrMYB1.1n, MrMYB1.1d, MrMYB1.2-1, MrMYB1.3-1, and MrMYB2-1, from the start codon or the first alternative start codon (ATG*) as illustrated in Fig. 3, were cloned into pGADT7 vectors. Primer information is given in Supplementary Table S1. The interactions were screened on SD/-Leu agar plate supplemented with AbA. All transformations and screenings were carried out for at least three times.
EMSA
The CDS regions of MrMYB1.1n and MrMYB1.3-1 were respectively cloned into pCold-TF vector and transformed into E. coli Rossetta (DE3). Primer information is given in Supplementary Table S1. Expression and purification of the recombinant proteins were carried out as reported previously (Ren et al. 2022). The EMSA was conducted using the LightShiftTM Chemiluminescent EMSA Kit (Thermo Fisher Scientific, MA, United States) according to the manual for the kit. The probes used for EMSAs are listed in Supplementary Table S1.
Yeast two-hybrid assay
The CDS regions of MrbHLH1 were cloned into pGBKT7 vector. Primer information is given in Supplementary Table S1. The MrbHLH1-pGBKT7 construct was transformed into yeast (Saccharomyces cerevisiae) strain Y2HGold cells and the background expression level of AbA resistant gene (AbAr) was monitored on SD/-Trp supplemented with 200 ng/mL AbA. Subsequently, the previously constructed recombinant pGADT7 vectors harboring either of MrMYB1.1n, MrMYB1.1d, MrMYB1.2-1, MrMYB1.3-1, or MrMYB2-1 were transformed into the yeast. Then the yeast was selected on SD/-Leu/-Trp supplemented with 200 ng/mL AbA at 30 °C for 3–4 d. The interactions were tested on SD/-Ade/-His/-Leu/-Trp supplemented with 200 ng/mL AbA, with or without X-α-Gal. All transformations and screenings were carried out for at least three times.
Firefly LCI assay
The CDS regions of MrMYB1.1n, MrMYB1.3-1, and MrbHLH1 were separately cloned into the pCAMBIA1300-nLUC (nLUC) and pCAMBIA1300-cLUC (cLUC) vectors. Primer information is given in Supplementary Table S1. A. tumefaciens cultures (strain EHA105) containing nLUC and cLUC were mixed in a 1:1 (v/v) ratio in infiltration buffer (10 mM MgCl2, 10 mM MES, 150 mM acetosyringone; pH 5.6), setting the final A600 of each suspension to 1.0, and were then injected into N. benthamiana leaves. The LUC activity was determined 2 d after infiltration. Before imaging, 100 μL of 0.2 mM luciferin was injected into the leaves and kept in the dark for 15 min. The fluorescence intensity imaging was carried out using the NightSHADE LB 985 system. Three biological replicates, each consisting of three leaves taken from one plant, were set for LCI assays. The experiments were repeated three times independently.
Phylogenetic and genomic syntenic analysis
Phylogenetic tree was constructed in molecular evolutionary genetics analysis 11 using the maximum likelihood approach and 1,000 bootstrap replicates (Tamura et al. 2021) with the MYBs from various plants as listed in Supplementary Table S2. For genomic syntenic analysis, MCscanX (v.0.8) (Wang et al. 2012) were used to identify the syntenic and collinear blocks between each two species based on core putatively orthologous gene sets discovered by BlastP (E-value ≤ 1e−5), and the minimum number of genes required to call a syntenic block was set to five. Evolutionary relationship among eight species, with the exception of tomato (S. lycopersicum) because of the absence of collinearity, was obtained from the TimeTree database (http://www.timetree.org/; Kumar et al. 2022). The results were visualized using TBtools (Chen et al. 2020). The whole genome sequence data involved were downloaded from Phytozome (carrot or D. carota; peach or P. persica; and tomato; Goodstein et al. 2012), Genome Warehouse at the National Genomics Data Center Chinese Academy of Sciences (P. betulifolia), National Center for Biotechnology Information (grape or V. vinifera, and Chinese bayberry), The Arabidopsis Information Resource database (A. thaliana), “Hanfu” apple genome data repository (https://github.com/moold/Genome-data-of-Hanfu-apple; apple or M. domestica), and Citrus Pan-genome to Breeding Database (sweet orange or Citrus sinensis).
Statistical analysis
The mean ± standard error was calculated for experiments involving biological replicates. Statistical analysis was performed by IBM SPSS Statistics 23 (IBM Corp., Armonk, NY, United States) using one-way analysis of variance (ANOVA). Graphs were drawn using GraphPad Prism 9.0.2 for Windows (GraphPad Software, San Diego, CA, United States; www.graphpad.com).
Accession numbers
Sequence data of MrMYBs obtained in this study have been deposited in GenBank under following accession numbers: MrMYB1.1-1 with alias MrMYB1.1n (OQ224663), MrMYB1.1-2 with alias MrMYB1.1d (OQ224664), MrMYB1.2-1 (OQ224665), MrMYB1.2-2 (OQ224666), MrMYB1.2-3 (OQ224667), MrMYB1.3-1 (OQ224668), MrMYB1.3-2 (OQ224669), MrMYB1.3-3 (OQ224670), MrMYB2-1 (OQ224671), and MrMYB2-2 (OQ224672).
Acknowledgments
We would like to thank Mr. Zehuang Zhang, Mr. Qihua Lin, and Ms. Qiuzhen Zhong (Fruit Research Institute of Fujian Academy of Agriculture Science) for providing Chinese barberry cultivars' fruits, as well as colleagues Mr. Changqing Zhu and Ms. Rong Jin for assistance on anthocyanin measurement and tobacco (N. tabacum) plant maintenance.
Author contributions
C.X. designed the study; C.X. obtained funding; L.X. performed the experiments and analyses with help from X.L., W.W., D.H., C.R., and X.H.; L.X. and C.X. wrote the manuscript; X.Y., A.C.A., K.L.-W., and K.C. contributed to revision and language enhancement. All authors read and approved the final manuscript.
Supplementary data
The following materials are available in the online version of this article.
Supplementary Figure S1. PCR amplification of the three intergenic fragments in four Chinese bayberry cultivars.
Supplementary Figure S2. PCR amplification of genomic sequences of four MYBs in four Chinese bayberry cultivars.
Supplementary Figure S3. Sequence alignment of predicted proteins from all alleles of MrMYB1.1, MrMYB1.2, MrMYB1.3, and MrMYB2.
Supplementary Figure S4. Phylogenetic relationships between MrMYBs and other MYBs involved in anthocyanin biosynthesis in other plant species.
Supplementary Figure S5. Sanger sequence-based confirmation of MrMYB1.1 genotypes in 14 Chinese bayberry cultivars at the genomic level.
Supplementary Figure S6. PCR amplification of transcript sequences using CAPS marker primers SP2 and ASP2.
Supplementary Figure S7. Sanger sequence-based confirmation of MrMYB1.1 genotypes in 14 Chinese bayberry cultivars at the transcript level.
Supplementary Figure S8. Sequences of RT-qPCR primers for MrMYB1.1, MrMYB1.2, MrMYB1.3, and MrMYB2.
Supplementary Figure S9.MrMYB1.1 genotyping of “Luozi” (LZ) and “Dayezhong” (DYZ) using CAPS.
Supplementary Figure S10. Functional analysis of MrMYB1.1n with MrMYB1.1d.
Supplementary Figure S11. Validation of activation effects of MrMYB1.1n with MrMYB1.1d on the promoter of anthocyanin biosynthetic genes measured by dual-luciferase assays.
Supplementary Figure S12. Effects of MrMYB cluster members, alone or in combination with MrbHLH1, on the promoter activity of MrCHS and MrF3H measured by dual-luciferase assays.
Supplementary Figure S13. SDS-PAGE analysis of MrMYB1.1n and MrMYB1.3-1 protein.
Supplementary Table S1. Primers/probes used in this study.
Supplementary Table S2. Gene IDs for plant MYBs used in phylogenetic analysis.
Supplementary Table S3. RT-qPCR primers for expression analysis of anthocyanin-related genes in Chinese bayberry (M. rubra) and tobacco (N. tabacum).
Funding
This work was supported by the Natural Science Foundation of Zhejiang Province, China (LZ17C150001), and Pao Yu-Kong International Fund, Zhejiang University.
Data availability
The data underlying this article are available in the article and in its online supplementary material.
Dive Curated Terms
The following phenotypic, genotypic, and functional terms are of significance to the work described in this paper:
References
Author notes
The author responsible for distribution of materials integral to the findings presented in this article in accordance with the policy described in the Instructions for Authors (https://dbpia.nl.go.kr/plphys/pages/General-Instructions) is Changjie Xu.
Conflict of interest statement. None declared.