-
PDF
- Split View
-
Views
-
Cite
Cite
Mingqian Liu, Mingmin Lu, Ziwei Zhao, Qin Luo, Feng Liu, Jing Zhao, Yubing He, Yanan Tian, Huadong Zhan, Rice ILI atypical bHLH transcription factors antagonize OsbHLH157/OsbHLH158 during brassinosteroid signaling, Plant Physiology, Volume 194, Issue 3, March 2024, Pages 1545–1562, https://doi.org/10.1093/plphys/kiad635
- Share Icon Share
Abstract
Brassinosteroids (BRs) are a group of steroid hormones that play crucial roles in plant growth and development. Atypical bHLH transcription factors that lack the basic region for DNA binding have been implicated in BR signaling. However, the underlying mechanisms of atypical bHLHs in regulation of rice (Oryza sativa) BR signaling are still largely unknown. Here, we describe a systematic characterization of INCREASED LEAF INCLINATION (ILI) subfamily atypical bHLH transcription factors in rice. A total of 8 members, ILI1 to ILI8, with substantial sequence similarity were retrieved. Knockout and overexpression analyses demonstrated that these ILIs play unequally redundant and indispensable roles in BR-mediated growth and development in rice, with a more prominent role for ILI4 and ILI5. The ili3/4/5/8 quadruple and ili1/3/4/7/8 quintuple mutants displayed tremendous BR-related defects with severe dwarfism, erect leaves, and sterility. Biochemical analysis showed that ILIs interact with OsbHLH157 and OsbHLH158, which are also atypical bHLHs and have no obvious transcriptional activity. Overexpression of OsbHLH157 and OsbHLH158 led to drastic BR-defective growth, whereas the osbhlh157 osbhlh158 double mutant developed a typical BR-enhanced phenotype, indicating that OsbHLH157 and OsbHLH158 play a major negative role in rice BR signaling. Further transcriptome analyses revealed opposite effects of ILIs and OsbHLH157/OsbHLH158 in regulation of downstream gene expression, supporting the antagonism of ILIs and OsbHLH157/OsbHLH158 in maintaining the balance of BR signaling. Our results provide insights into the mechanism of BR signaling and plant architecture formation in rice.
Introduction
Rice (Oryza sativa L.) is one of the most important food crops in the world. Increasing grain yield has always been the primary goal of rice breeding to meet the demands of a growing global population. Rice yield is an important and complex agronomic trait controlled by multiple factors, including plant height, leaf angle, grain size, and so on. Brassinosteroids (BRs), as a group of polyhydroxylated steroidal hormones, play essential roles in modulating plant architecture and grain yield. Uncovering the underlying mechanisms of BR signaling holds the potential for crop production in rice (Zhang et al. 2014; Tong and Chu 2018).
Over the past decades, genetic and biochemical characterization of numerous BR-related mutants has established the framework of BR signaling in plant. In brief, BRs are perceived at the plasma membrane by the receptor kinase BRASSINOSTEROID-INSENSITIVE 1 (BRI1) (Li and Chory 1997; He et al. 2000; Kinoshita et al. 2005; Hothorn et al. 2011; She et al. 2011). In the absence of BRs, BRI1 KINASE INHIBITOR 1 (BKI1) binds and inhibits the function of BRI1 (Wang and Chory 2006; Jaillais et al. 2011). Upon BRs binding, BRI1 is dissociated from BKI1 and forms an active receptor complex with BRI1-ASSOCIATED RECEPTOR KINASE 1 (BAK1) through a series of autophosphorylation and transphosphorylation (Li et al. 2002; Nam and Li 2002; Wang et al. 2008; Wang et al. 2014). The activated BRI1 can further sequentially phosphorylate and activate the BR SIGNALING KINASE 1 (BSK1), CONSTITUTIVE DIFFERENTIAL GROWTH 1 (CDG1), and the phosphatase BRI1-SUPPRESSOR 1 (BSU1), which leads to the dephosphorylation and inactivation of the negative regulator GSK3/Shaggy-like kinase BRASSINOSTEROID-INSENSITIVE 2 (BIN2) (Li et al. 2001; Li and Nam 2002; Mora-Garcia et al. 2004; Tang et al. 2008; Kim et al. 2009; Kim et al. 2011). Inhibition of BIN2 leads to the accumulation of nonphosphorylated forms of transcription factors BRASSINAZOLE-RESISTANT 1 (BZR1) and BRI1-EMS-SUPPRESSOR 1 (BES1, also named OsBZR2), which move into the nucleus and regulate the expression of numerous BR-responsive genes (He et al. 2002; Wang et al. 2002; Yin et al. 2002, 2005; Gampala et al. 2007; Ryu et al. 2007; Wang et al. 2012; Nosaki et al. 2022). The BR signaling is evolutionarily conserved between monocotyledonous and dicotyledonous plants, as many key components of rice BR signaling cascade, for example, OsBRI1, OsBAK1, OsGSK2, and OsBZR1, share strong sequence and functional similarities to their Arabidopsis (Arabidopsis thaliana) orthologs (Yamamuro et al. 2000; Bai et al. 2007; Li et al. 2009; Tong et al. 2012; Zhang et al. 2016).
The basic helix–loop–helix (bHLH) proteins, one of the largest transcription factor families in plant, play important roles in modulating BR signaling. BZR1/BES1 family proteins, the master transcription factors of BR signaling, contain a noncanonical bHLH motif in their DNA-binding region (Yin et al. 2005; Nosaki et al. 2018). A large number of bHLHs, for example, BRASSINOSTEROID-ENHANCED EXPRESSIONs (BEE1 to BEE3), BES1-INTERACTING MYC-LIKEs (BIM1 to BIM3), ACTIVATORS OF CELL ELONGATIONs (ACE1 and ACE2), HOMOLOG OF BRASSINOSTEROID-ENHANCED EXPRESSION2 INTERACTING WITH IBH1 (HBI1), and CESTA (CES), have been demonstrated to be implicated in BR signaling in Arabidopsis (Friedrichsen et al. 2002; Yin et al. 2005; Poppenberger et al. 2011; Bai et al. 2012; Ikeda et al. 2012). Among these, BIM proteins directly interact with BES1 to synergistically bind the E-box of the BR-induced genes to activate their expression (Yin et al. 2005). OsbHLH79, also designated as BRASSINOSTEROID RESPONSIVE LEAF ANGLE REGULATOR 1 (OsBLR1), positively regulates leaf angle and grain size in rice. Overexpression of OsbHLH79/OsBLR1 leads to increased leaf angle and grain length, whereas osblr1 knockout mutants develop erect leaves and shorter grains (Seo et al. 2020; Wang et al. 2020). OsbHLH98 negatively regulates leaf angle by counteracting BR-induced cell elongation via repressing OsBUL1 transcription (Guo et al. 2021).
In addition to the abovementioned typical bHLH factors, another kind of bHLH proteins, the atypical bHLHs, is also involved in BR signaling. Structurally, the bHLH domain is composed of 2 distinct functional regions, the basic region and the helix–loop–helix region (Atchley et al. 1999; Toledo-Ortiz et al. 2003). The basic region is required for DNA binding, while the HLH region is responsible for homo- or heterodimerization. Accordingly, the bHLH factors are classified into 2 categories: DNA-binding bHLH and non–DNA-binding atypical bHLH (HLH). The atypical bHLH is unable to bind DNA but retains the ability to form a heterodimer (Massari and Murre 2000). Hence, it is generally believed that the atypical bHLH proteins function as an inhibitor of other HLH or bHLH proteins through heterodimerization (Toledo-Ortiz et al. 2003). INCREASED LEAF INCLINATION 1 (ILI1) and ILI1 binding bHLH (OsIBH1) are a pair of antagonistic atypical bHLH proteins. The ili1-D activation mutant is hypersensitive to BR and develops remarkably increased leaf angle, while OsIBH1 overexpression causes erect leaf. ILI1 physically interacts with OsIBH1 to form a heterodimer that inhibits the function of OsIBH1 (Zhang et al. 2009). BR UPREGULATED 1 (OsBU1) also encodes a HLH protein. Rice plants overexpressing OsBU1 develop enhanced bending of the lamina joint, increased grain size, and resistance to BR biosynthesis inhibitor brassinazole (BRZ) (Tanaka et al. 2009). BRASSINOSTEROID UPREGULATED 1-LIKE 1 (OsBUL1) is a homolog of OsBU1, and likewise, overexpression of OsBUL1 produces leaves with a larger angle (Jang et al. 2017). OsBUL1 interacts with the mediator LO9-177, an uncharacterized KxDL motif-containing protein. In the presence of LO9-177, OsBUL1 further interacts with the typical bHLH transcriptional activator OsBUL1 COMPLEX 1 (OsBC1) to form a trimeric complex for the lamina joint cell elongation in rice (Jang et al. 2017). Another 3 atypical bHLH transcription factors, OsbHLH153, OsbHLH173, and OsbHLH174, are identified through genome-wide association studies (GWAS) and have been shown to positively regulate rice leaf angle (Dong et al. 2018).
Sequence analysis revealed that ILI1, OsBU1, OsBUL1, OsbHLH153, OsbHLH173, and OsbHLH174 belong to the same subfamily. Ectopic expression assays indicate their involvement in regulation of leaf angle and grain size in rice (Tanaka et al. 2009; Zhang et al. 2009; Jang et al. 2017; Dong et al. 2018). However, multifaceted knowledge about these atypical bHLHs remains poorly understood. For instance, the member composition of this atypical bHLH subfamily is still not definite. Zhang et al (2009) identified 7 ILI homologs (ILI1 to ILI7) through phylogenic and sequence analyses. Nevertheless, only 5, ILI1/OsbHLH154, ILI3/OsbHLH153, ILI4/OsBU1/OsbHLH172, ILI5/OsBUL1/OsbHLH170, and ILI7/OsbHLH173, were recognized as bHLH family members (Carretero-Paulet et al. 2010). On the other hand, current information about ILIs is predominantly derived from overexpression studies. Due to the lack of knockout mutants, the functional specificity, diversity, and redundancy about ILI subfamily members is almost completely unclear. Furthermore, the downstream molecular targets of ILIs also need to be elucidated.
In this study, we describe a systematic characterization of rice ILI subfamily. A total of 8 members (ILI1 to ILI8) with obvious sequence similarity and domain conservation were found in rice. Knockout and overexpression analysis demonstrated that ILIs are indispensable components of rice BR signaling. Moreover, we screened 2 ILI-interacting proteins, OsbHLH157 and OsbHLH158. OsbHLH157 and OsbHLH158 also belonged to atypical bHLHs but negatively regulated rice BR signaling. Further transcriptome analyses revealed opposite effects of ILIs and OsbHLH157/OsbHLH158 in regulation of downstream gene expression, supporting the antagonism of ILIs and OsbHLH157/OsbHLH158 in maintaining the balance of BR signaling in rice.
Results
Overexpression analysis reveals the functional conservation of ILI subfamily in modulating BR signaling
The atypical bHLH factor ILI1, also known as OsbHLH154, was originally identified in the screen of activation tagging mutants with increased leaf inclination in rice (Zhang et al. 2009). To further determine the composition and molecular characteristics of the ILI subfamily, the number, phylogeny, and functional domain of ILI proteins were analyzed. A total of 8 members (ILI1 to ILI8) with obvious sequence similarity and domain conservation were retrieved (Supplemental Fig. S1, A to C). Among these, ILI4 (OsbHLH172) and ILI5 (OsbHLH170) were previously reported OsBU1 and OsBUL1 (Tanaka et al. 2009; Jang et al. 2017). ILI3, ILI7, and ILI8 were named OsbHLH153, OsbHLH173, and OsbHLH174, respectively. ILI2 and ILI6 did possess conserved HLH domain but were not recognized as bHLH family members according to the existing classification (Supplemental Fig. S1C; Carretero-Paulet et al. 2010), rendering uncertainty about the subfamily of rice ILI.
To evaluate and compare the role of these ILIs, we first constructed overexpressing transgenic rice plants of individual ILI genes. For each transgene, more than 20 T0 (first generation) plants were obtained through Agrobacterium tumefaciens–mediated rice transformation. Noteworthily, a high proportion (>40%) of ILI1, ILI2, ILI3, ILI4/OsBU1, ILI5/OsBUL1, and ILI8 overexpressing plants developed sterility with enlarged leaf angle, twisted culm, and dwarfed stature at T0 generation. In comparison, the fertility and seed set of ILI6 and ILI7 overexpression transgenic lines was not obviously affected, implying a possible functional differentiation among these ILIs in regulation of reproduction in rice.
Two independent and fertile transgenic lines with representative growth performance were chosen for further analysis. RT-qPCR confirmed the significantly elevated expression of target ILI gene (Supplemental Fig. S2). As expected, BR-increased growth and development was observed in these ILIOx plants (Fig. 1). Compared with the wild-type, the leaf angle, grain length, width, and weight of ILI overexpression transgenic lines were tremendously increased (Fig. 1; Supplemental Fig. S3, B to E). Decreased plant height is another typical feature of BR-enhanced plant. As shown in Fig. 1, A to Q, the ILIOx plants developed obviously reduced height at maturity. In line with this shorter stature, the extension of upper internodes, especially the first internode, was distinctively inhibited in the ILIOx transgenic lines (Fig. 1R; Supplemental Fig. S3A). Altogether, these results reveal a conserved role of ILI subfamily members in modulating BR signaling. Intriguingly, unlike the wild-type control, the growth of the bottom fifth internode was remarkably increased in ILI1Ox-1, ILI2Ox-2, ILI3Ox-2, ILI4Ox-6, ILI5Ox-2, and ILI7Ox-2 transgenic plants (Fig. 1R; Supplemental Fig. S3A), suggesting the putative dual effect of the ILIs in regulation of rice internode growth.
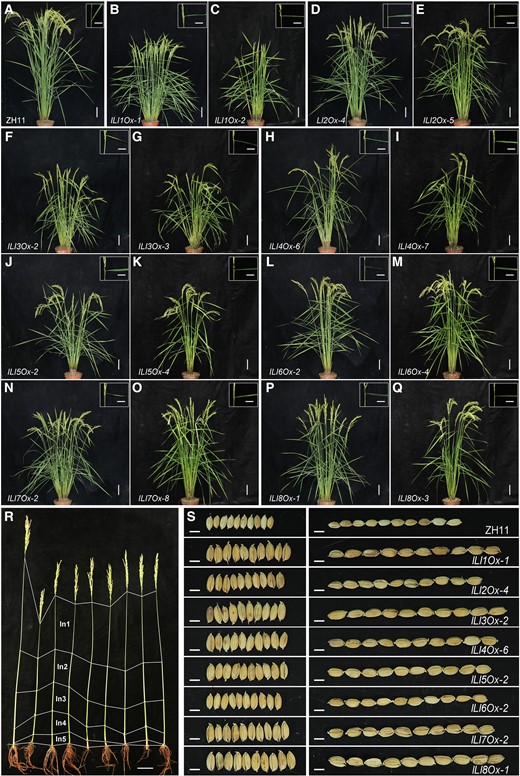
Phenotypic characterization of transgenic rice plants overexpressing ILI genes. A to Q) Plant morphologies of wild-type (A) and ILI overexpression rice plants (B to Q) at the mature stages. Bars, 10 cm. The pictures at the top right show the flag leaf inclinations (bars, 5 cm). R) Culm structures of wild-type and overexpression plants at the mature stage (approximate 95 days after sowing). From left to right: wild-type, ILI1Ox-1, ILI2Ox-4, ILI3Ox-2, ILI4Ox-6, ILI5Ox-2, ILI6Ox-2, ILI7Ox-2, and ILI8Ox-1. In, internode. Bar, 10 cm. S) Grain morphologies of wild-type and ILI overexpression lines. Bars, 0.5 cm.
ILIs are indispensable for BR-mediated growth and development
As HLH proteins, the peptide chains of ILIs are usually composed of less than or just about 100 amino acid residues (Supplemental Fig. S1A). The small exons of ILI genes impose a major obstacle to the acquisition of T-DNA loss-of-function mutants. In fact, only a single insertion knockout mutant line of ILI5/OsBUL1 has been isolated so far (Supplemental Fig. S1A; Jang et al. 2017). For this reason, the endogenous function and genetic relationship of these ILIs is almost completely unknown. To overcome this hurdle, CRISPR/Cas9 gene editing tool was employed to induce directional mutations in this study. Specifically, 8 single gene knockout vectors were constructed to mutate the individual ILI gene, as well as 4 paired sgRNAs targeting to ILI1/ILI7, ILI2/ILI6, ILI3/ILI8, and ILI4/ILI5, respectively, were selected for double gene knockout (see Supplemental Table S1 for the information of the target sites). Moreover, taking advantage of the sequence homology within the conserved HLH domain, sgRNA sites that simultaneously target to multiple ILI genes were designed to generate the higher-order ili3/4/5/8 quadruple and ili1/3/4/7/8 quintuple mutants (Supplemental Table S1).
Over 350 seedlings of T0 generation were collected for above editing vectors utilizing the Agrobacterium-mediated genetic transformation. Through genotypic PCR and sequencing analysis, we successfully obtained a series of independent single and double mutant alleles. For quadruple gene knockout, 5 T0 plants (5/34) were found to develop severely dwarfed stature. Only 3 plants survived after transferring to the field and were confirmed as ili3/4/5/8 quadruple loss-of-function mutants through Sanger sequencing (Supplemental Fig. S4 and Table S1). Twenty-eight T0 transformants were obtained for ili1/3/4/7/8 quintuple knockout, and they were divided into 3 groups based on their growth and mutation feature. The majority was partial gene knockouts and exhibited overall normal growth. Three plants (3/28) with complete knockout of ILI1, ILI3, ILI4, ILI7, and ILI8 genes developed severe dwarfism. One plant (1/28) displayed semidwarfism and should be a weak allele of quintuple knockout mutant, because of the presence of 1 bp insertion/6 bp deletion in ILI4 and heterozygous 1 bp insertion in ILI7 (Supplemental Table S1). The severely dwarfed ili3/4/5/8 quadruple and ili1/3/4/7/8 quintuple mutants were totally sterile (Supplemental Fig. S4). The fertility of the relatively mild weak quintuple mutant was also seriously impaired; nevertheless, a small amount of seeds were able to be obtained.
Through genetic segregation, homozygous single, double, and quintuple loss-of-function mutants of ILI genes were harvested (Supplemental Table S1). Among the 8 single mutants, ili4 and ili5 showed visible BR-related phenotypes, including slight dwarfism, erect leaves, and small grains, whereas other ili single mutant plants only displayed relatively small and topical morphological change, suggesting a dominant role of ILI4 and ILI5 in the subfamily (Fig. 2; Supplemental Fig. S5). The growth of ili5 was consistent with previous T-DNA insertional mutant (Jang et al. 2017). Notably, ili7 plants developed increased leaf angle (Fig. 2, H and S). This might be caused by feedback regulation of BR signaling as common in BR-related mutants. No more serious abnormalities were further detected in the various combinations of ili double mutants. The ili4 ili5 had similar growth with the ili4 and ili5 single mutant plants (Fig. 2, J to M; Supplemental Fig. S5). In contrast, the ili3/4/5/8 quadruple and ili1/3/4/7/8 quintuple mutants developed much more severe growth defects (Fig. 2, N to S; Supplemental Fig. S4). The average plant height of ili1/3/4/7/8 was only about 20 cm at the mature stage (Fig. 2, N to Q; Supplemental Fig. S5A). Much shorter internodes, tortuous and dark green leaf blades, and completely erect leaves were visualized in ili1/3/4/7/8 (Fig. 2, P to S). We further assessed the BR sensitivity of ili1/3/4/7/8 quintuple mutants by lamina inclination assay. With exogenous application of 24-epibrassinolide (epiBL), the lamina joint inclination was increased significantly in wild-type, but not altered in ili1/3/4/7/8-c1, mutant, indicating that ili1/3/4/7/8 was insensitive to BL (see Fig. 6, A and B). All together, these results suggested that ILIs are indispensable component of BR signaling. ILIs take part in BR signaling and show redundant roles in regulation of BR-mediated growth and development in rice, with a more prominent role for ILI4 and ILI5.

Phenotypic analyses of ili knockout mutants. A to O) Plant morphologies of wild-type, ili1 to ili8 single mutants, ili double mutants, and ili1/3/4/7/8 quintuple mutants at the mature stage. Bars, 10 cm. The inserted pictures show the flag leaf inclinations (Bars, 5 cm). P and Q) Culm structures of ili1/3/4/7/8 quintuple mutants. Bars, 5 cm. The red arrows indicate the node positions. R) The dark green and tortuous flag leaf blades of ili1/3/4/7/8 quintuple mutants. Bars, 2 cm. S) Quantification of the angles of flag leaf of wild-type and ili mutants. Values are means ± Sd (n = 12). Asterisks indicate significant differences between ZH11 and ili mutants (**P < 0.01, Student's t test). T) Grain morphologies of wild-type and ili mutants. Bars, 0.5 cm.
ILIs interact with OsbHLH157 and OsbHLH158
Previously, ILI1 was reported to interact with and inhibit the negative HLH factor OsIBH1 to modulate leaf angle in rice. Nevertheless, overexpression of OsIBH1 only results in a weak phenotypical alteration with erect leaf and slightly reduced plant height (Zhang et al. 2009). Considering the extremely severe defects of ili3/4/5/8 quadruple and ili1/3/4/7/8 quintuple mutants (Fig. 2, N and O; Supplemental Fig. S4), we inferred that there should be additional HLH or bHLH target(s) to maintain the balance of ILI-mediated BR signaling in rice. To this end, yeast 2-hybrid assay was performed to identify the possible interacting downstream targets. As a result, 2 bHLH family proteins OsbHLH157 and OsbHLH158 were screened out. Compared with the empty binding domain (BD) and activation domain (AD) controls, yeast colonies harboring the plasmids OsbHLH157/OsbHLH158-BD and ILIs-AD grew well on synthetic defined medium lacking Trp, Leu, His, and Ade (SD-TLHA, quadruple dropout) (Fig. 3A; Supplemental Fig. S6). The interaction was further confirmed in plant cell by bimolecular fluorescent complementation (BiFC) and split-luciferase (LUC) complementation imaging assay. Coinjection of OsbHLH157/OsbHLH158-nYFP with ILIs-cYFP or OsbHLH157/OsbHLH158-nLUC with ILIs-cLUC into Nicotiana benthamiana leaves led to obvious fluorescence signals (Fig. 3, B and C). Intriguingly, OsbHLH157 seemed not to interact with ILI3 in yeast 2-hybrid system but they showed interaction in plant cell. A similar phenomenon was observed for OsbHLH158 and ILI7. This might be related with the weak interaction in certain combinations. Overall, these results demonstrated that OsbHLH157 and OsbHLH158 interact with ILI subfamily members.
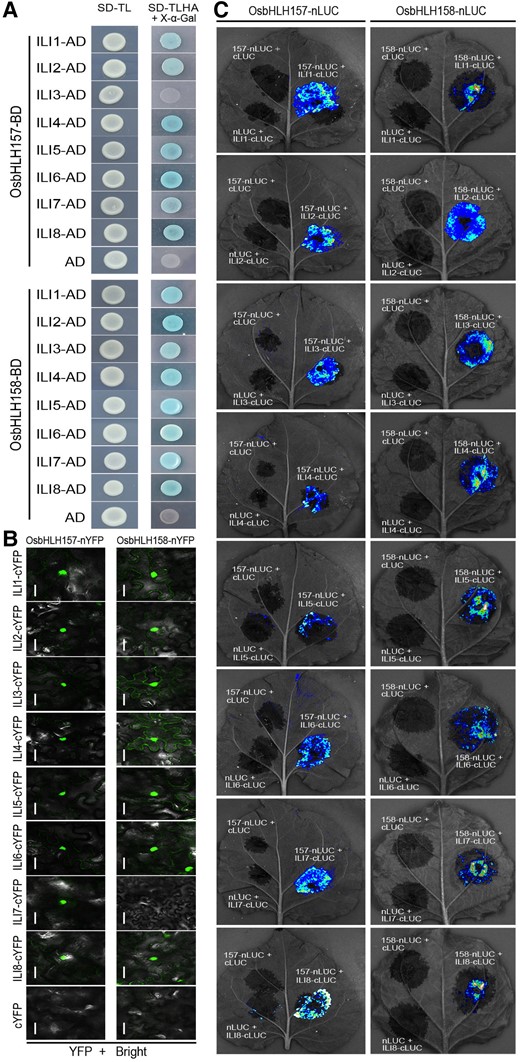
Interaction between ILIs and OsbHLH157/OsbHLH158. A) Interaction between ILIs and OsbHLH157/OsbHLH158 in yeast 2-hybrid assay. SD/-TL, selective medium lacking Trp and Leu. SD/-TLHA, lacking Trp, Leu, His, and Ade. X-α-Gal (5-bromo-4-chloro-3-indolyl-α-d-galactopyranoside) was added to indicate the β-galactosidase activity of transformed yeast cells. Yeast transformations of the empty prey (AD) and OsbHLH157/OsbHLH158-BD were used as negative control. B) Interaction between ILIs and OsbHLH157/OsbHLH158 using BiFC assay. Bars, 20 μm. C) Split-LUC complementation analysis of the interaction between ILIs and OsbHLH157/OsbHLH158.
The C-terminus fusion constructs of ILIs and OsbHLH157/OsbHLH158 with the green fluorescent protein (GFP) driven by cauliflower mosaic virus (CaMV) 35S promoter were generated to determine their subcellular localization. Transient expression in N. benthamiana leaves revealed that the rice ILI proteins were localized in both the nucleus and the cytoplasm like their Arabidopsis counterparts (Fig. 4A; Hong et al. 2013; Buti et al. 2020). ILI6 was a little distinctive as only relatively faint GFP signals could be observed in nucleus (Fig. 4A). The GFP fluorescence of OsbHLH157 was specifically confined in the nucleus, whereas OsbHLH158 localized in both the nucleus and the cytoplasm (Fig. 4A). The nuclear distribution of ILIs and OsbHLH157/OsbHLH158 was not only in accordance with their identity of transcriptional regulators but also enabled direct physical interaction between these proteins.
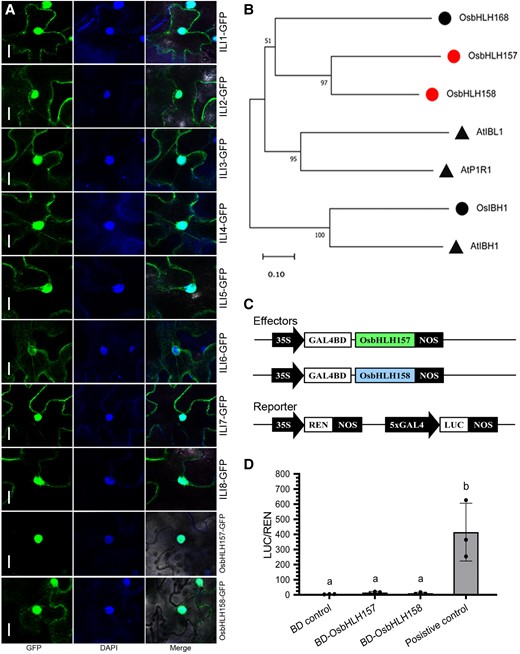
Subcellular localization, phylogenetic analysis, and transcriptional activity assay of ILIs and OsbHLH157/OsbHLH158. A) Subcellular localization of ILIs, OsbHLH157, and OsbHLH158 by transient expression in N. benthamiana. The green fluorescent signals visualized with confocal microscopy represent the 35S-ILIs-GFP, 35S-OsbHLH157-GFP, or 35S-OsbHLH158-GFP fusion protein. The fluorescent dye DAPI was added to mark the nuclei. Bars, 10 µm. B) Phylogenetic tree of OsbHLH157/OsbHLH158 and their homologs from rice and Arabidopsis. Circles and triangles represent proteins from rice and Arabidopsis, respectively. OsbHLH157 and OsbHLH158 are highlighted in red. The maximum likelihood tree was constructed using the MEGA 11.0 program. Bootstrapping was performed with 1,000 replicates. C) Diagram of the effector and reporter constructs of the dual-LUC transcriptional assay system. D) Transcriptional regulatory activity of OsbHLH157 and OsbHLH158. Values are means ± Sd (n = 3). Different lowercase letters represent significant differences (P < 0.05, 1-way ANOVA with Tukey's test).
OsbHLH157 and OsbHLH158 are atypical bHLH proteins and show no obvious transcriptional activity
OsbHLH157 and OsbHLH158 shared ∼47.67% (92/193) amino acid identity and 61.66% (119/193) amino acid similarity (Supplemental Fig. S7A), and they were previously classified as subfamily 20 atypical bHLHs (Carretero-Paulet et al. 2010). Sequence homologs of OsbHLH157 and OsbHLH158 were retrieved in rice and Arabidopsis through BLAST search (Fig. 4B; Supplemental Fig. S7B). Phylogenetic analysis showed that OsbHLH157 and OsbHLH158 cluster with the atypical bHLHs, AtP1R1, AtIBL1, AtIBH1, and OsIBH1 (Fig. 4B). Further sequence alignment revealed OsbHLH157 and OsbHLH158 possess the conserved HLH domain with these reported atypical bHLHs, though their sequence identity of the whole amino acid was at a low level (20% to 30%) (Supplemental Fig. S7, B and C).
Yeast 2-hybrid analysis was performed to learn about the transcriptional activity of OsbHLH157 and OsbHLH158. The full-length coding sequence (CDS) fragments of OsbHLH157 and OsbHLH158 were fused with the GAL4 DNA-binding domain and transferred into yeast strain Y2H Gold (Clontech). Unlike the positive control, the yeast transformants that expressed the OsbHLH157 or OsbHLH158 recombinant protein could not survive on the SD-Trp/-Leu/-His selective medium (Supplemental Fig. S8), indicating that they likely had no direct transcriptional activity. We additionally investigated their transcriptional activity using a dual-LUC reporter system in rice protoplasts (Fig. 4C). In comparison with the BD negative control, the relative LUC activities of OsbHLH157 and OsbHLH158 increased slightly, but no significant differences were observed (Fig. 4D), further supporting the nontranscriptional activation role for OsbHLH157 and OsbHLH158. Therefore, as non–DNA-binding atypical bHLHs, OsbHLH157 and OsbHLH158 should not likely function as transcription factors by themselves.
OsbHLH157 and OsbHLH158 negatively regulate rice BR signaling
To understand the physiological functions of OsbHLH157 and OsbHLH158 in rice, homozygous single and double loss-of-function mutants of OsbHLH157 and OsbHLH158 were generated using CRISPR/Cas9 gene editing tool after genetic segregation (Fig. 5A). No visible alterations were able to be detected in individual osbhlh157 and osbhlh158 single mutant plants during the whole period of growth. In comparison, osbhlh157 osbhlh158 double mutant demonstrated obvious BR-enhanced growth and development (Fig. 5, C to F; Supplemental Fig. S9). The average flag leaf angles of osbhlh157 osbhlh158 double mutant were close to 90 degrees, which were remarkably larger than those of wild-type and single mutant controls (Fig. 5, C to F, M). The grain length, width, and weight were also significantly increased in osbhlh157 osbhlh158 double mutant (Fig. 5N; Supplemental Fig. S9).
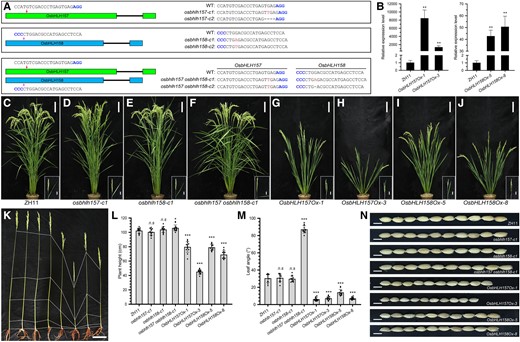
Phenotypic characterization of OsbHLH157 and OsbHLH158 knockout mutants and overexpression transgenic rice plants. A) sgRNA targets and mutation information of osbhlh157 and osbhlh158 mutants. The exons of OsbHLH157 and OsbHLH158 are labeled with green and blue boxes, respectively. The red and pink arrows indicate the position of target sgRNAs. PAM sequences (blue), insertions (red), and deletions (black dashes) are indicated. B) RT-qPCR analysis of OsbHLH157/OsbHLH158 expression in wild-type and overexpression transgenic lines. Values are means ± Sd (n = 3). Asterisks indicate significant differences between ZH11 and overexpression lines by Student's t test (**P < 0.01). C to J) Plant morphologies of wild-type, osbhlh157/osbhlh158 knockout mutants, and OsbHLH157/OsbHLH158 overexpression rice plants at the mature stages. Bars, 10 cm. The pictures at the bottom right show the flag leaf inclinations (bars, 5 cm). K) Culm structures of wild-type, osbhlh157/osbhlh158 mutants, and OsbHLH157/OsbHLH158 overexpression plants at the mature stage. Bar, 10 cm. From left to right: wild-type, osbhlh157-c1, osbhlh158-c1, osbhlh157 osbhlh158-c1, OsbHLH157Ox, and OsbHLH158Ox overexpression transgenic rice plants. L and M) Quantification of plant height and flag leaf angles of wild-type, osbhlh157/osbhlh158 mutants, and OsbHLH157/OsbHLH158 overexpression transgenic rice plants at the mature stage. Values are means ± Sd (n = 13 in L; n = 12 in M). Asterisks indicate significant differences between ZH11 and OsbHLH157/OsbHLH158 knockout mutants and overexpression plants by Student's t test (*P < 0.05, ***P < 0.001). n.s, no significance. N) Grain morphologies of wild-type, osbhlh157/osbhlh158 mutants, and OsbHLH157/OsbHLH158 overexpression transgenic rice plants. Bars, 0.5 cm.
In addition to above knockout mutants, we also constructed OsbHLH157 and OsbHLH158 overexpression transgenic rice materials. Two independent lines with significantly elevated expression levels of OsbHLH157 (OsbHLH157Ox-1 and OsbHLH157Ox-3) and OsbHLH158 (OsbHLH158Ox-5 and OsbHLH158Ox-8) were selected for further study (Fig. 5B). Contrary with the BR-enhanced phenotype in osbhlh157 osbhlh158 double mutant, overexpression of OsbHLH157 and OsbHLH158 led to a variety of BR-deficient growth defects (Fig. 5, C to N). First of all, the plant height was significantly reduced in OsbHLH157 and OsbHLH158 overexpression plants. The mean plant height of OsbHLH157Ox-3 was only about 45 cm at the mature stage (Fig. 5, G to L). Compared with the upper internodes, the extension of bottom internodes (In3 and In4) was more distinctively inhibited in these overexpression transgenic lines (Fig. 5K). Erect and dark green leaves were also observed in OsbHLH157 and OsbHLH158 overexpression plants (Fig. 5, G to J and M). The grain size and weight was significantly lowered in OsbHLH157 and OsbHLH158 overexpressing transgenic plants (Fig. 5N; Supplemental Fig. S9). Moreover, compared with wild-type, we observed greater increase of lamina joint angles in osbhlh157 osbhlh158 double mutants treated with 2,4-epiBL, whereas lamina joints of OsbHLH157 and OsbHLH158 overexpressing transgenic plants appeared largely insensitive to exogenous 2,4-epiBL (Fig. 6, A and B). Taken together, these results demonstrated that OsbHLH157 and OsbHLH158 are major negative regulators of BR signaling in rice, and they act redundantly to modulate rice growth and development.
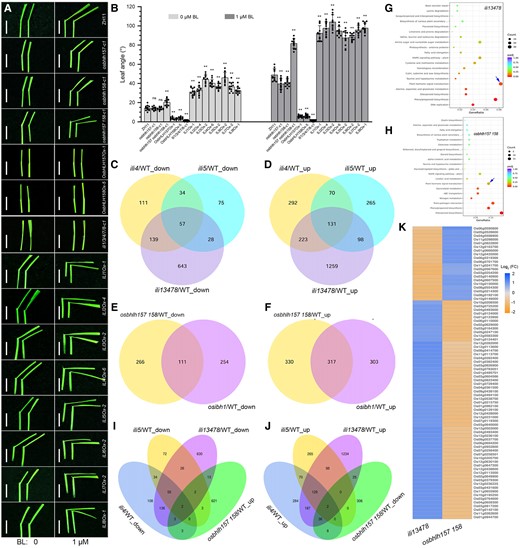
BR sensitivity and transcriptome analyses. A) Lamina joint bending response to exogenous 24-epiBL treatment of wild-type, knockout mutants, and overexpression transgenic rice plants of ILIs and OsbHLH157/OsbHLH158. Bars, 1 cm. B) Quantification of the lamina joint bending assay described in A). Data represent means ± Sd (n > 10). Asterisks indicate significant differences between ZH11 and knockout mutants or overexpression plants of ILIs and OsbHLH157/OsbHLH158 by Student's t test (**P < 0.01). C and D) Venn diagram for the overlap of DEGs between ili4, ili5, and ili1/3/4/7/8 quintuple mutants. E and F) Venn diagram for the overlap of DEGs between osbhlh157 osbhlh158 and osibh1 knockout mutants. G and H) KEGG term enrichment analysis of DEGs in ili1/3/4/7/8 (G) and osbhlh157 osbhlh158 mutants (H). The blue arrow indicates the enriched plant hormone signal transduction pathway. I and J) Venn diagram for the overlap of the reversely coregulated DEGs between ili1/3/4/7/8 and osbhlh157 osbhlh158 knockout mutants. K) Heatmap representation of the opposite expression of DEGs in ili1/3/4/7/8 and osbhlh157 osbhlh158 knockout mutants.
Transcriptome analyses reveal the antagonistic effects of ILIs and OsbHLH157/OsbHLH158 in regulation of gene expression
To learn about the molecular mechanism of ILIs and OsbHLH157/OsbHLH158 in regulation of BR signaling, RNA sequencing (RNA-seq) analyses were performed. Differentially expressed genes (DEGs) between the mutants and wild-type were identified using the standard of |Log2(fold change)|>1 and P-value < 0.05. We first analyzed the RNA-seq data of 2-wk-old wild-type, ili4-c1, ili5-c1, and ili1/3/4/7/8-c1 seedlings. A total of 341 and 194 downregulated genes were identified in ili4 and ili5, respectively, relative to the wild-type. Among these genes, 91 were downregulated in both mutants (Fig. 6C; Supplemental Fig. S10). A total of 716 and 564 genes were upregulated in ili4 and ili5, respectively, and of these, 201 genes were upregulated in both mutants (Fig. 6D; Supplemental Fig. S10). ILI4/OsBU1 and ILI5/OsBUL1 are 2 homologous proteins sharing over 98.8% amino acid similarity and 90.8% amino acid identity (Supplemental Fig. S1D). Nevertheless, the 26.67% (91/341) and 28.07% (201/716) common downregulated and upregulated DEGs in ili4 indicated that they might have functional divergence at the molecular level (Fig. 6, C and D). In comparison with ili4 and ili5 single mutants, many more DEGs were obtained in ili1/3/4/7/8 quintuple mutant. Specifically, 2,578 DEGs, including 1,711 upregulated and 867 downregulated genes, were identified in ili1/3/4/7/8-c1 (Fig. 6, C and D; Supplemental Fig. S10). This was in agreement with the more severe growth defects of ili1/3/4/7/8 plants. Venn diagram analysis revealed a high ratio of overlapping DEGs between ili4/ili5 single mutant and ili1/3/4/7/8 quintuple mutant. For example, 57.48% (196/341) and 44.5% (85/194) downregulated genes of ili4 and ili5 single mutants were commonly downregulated in ili1/3/4/7/8 (Fig. 6, C and D). The Kyoto Encyclopedia of Genes and Genomes (KEGG) and the gene ontology (GO) analysis showed that the DEGs related to pathways involved in plant hormone signal transduction, cell wall, cell division, cell cycle, and DNA-binding were significantly enriched in ili1/3/4/7/8 (Fig. 6G; Supplemental Fig. S11A).
We next investigated the RNA-seq data of osbhlh157 osbhlh158 double mutant. A total of 1,024 DEGs (377 downregulated genes and 647 upregulated genes) were detected in osbhlh157 osbhlh158-c1 (Supplemental Fig. S10D). KEGG and GO analysis showed that plant hormone signal transduction, defense response, and phenylpropanoid and diterpenoid biosynthesis were enriched in osbhlh157 osbhlh158 double mutant (Fig. 6H; Supplemental Fig. S11B). In addition to OsbHLH157 and OsbHLH158, OsIBH1 was another ILI-interacting atypical bHLH, but to date, only overexpression analysis of OsIBH1 was performed in rice (Zhang et al. 2009). In this study, we constructed osibh1 loss-of-function mutants using CRISPR/Cas9 gene editing tool (Supplemental Table S1). Contrast with the erect leaf of OsIBH1 overexpression rice plants (Zhang et al. 2009), the osibh1 knockout mutant had increased leaf angle (unpublished data). This endogenous evidence further supported its negative role in regulation of leaf angle. We additionally examined the transcriptome profile of osibh1. A total of 985 (620 upregulated genes and 365 downregulated genes) DEGs were found in osibh1-c1 seedlings (Supplemental Fig. S10E). Comparative analysis demonstrated the presence of a high ratio overlapping DEGs between osbhlh157 osbhlh158 and osibh1. About 51.13% (317/620) upregulated genes and 30.41% (111/365) downregulated genes of osibh1 had similar expression in osbhlh157 osbhlh158 double mutant (Fig. 6, E and F), suggesting that OsbHLH157/OsbHLH158 and OsIBH1 might share some common transcription modules in regulation of downstream gene expression.
As paired interacting atypical bHLHs, ILIs and OsbHLH157/OsbHLH158 displayed opposite effects on diverse aspects of plant growth and development as well as BR response. We inferred that this antagonism should also be occurring at the molecular level. To test this, DEGs with upregulated expression in osbhlh157 osbhlh158 but downregulated expression in ili1/3/4/7/8 or downregulated expression in osbhlh157 osbhlh158 but upregulated expression in ili1/3/4/7/8 were picked out and analyzed. As shown in Fig. 6, I and K, only ∼3.1% (20/647) upregulated genes of osbhlh157 osbhlh158 were downregulated in ili1/3/4/7/8. By comparison, a significantly high ratio (16.71%, 63/377) of downregulated genes of osbhlh157 osbhlh158 had upregulated expression in ili1/3/4/7/8 (Fig. 6, J and K), suggesting the presence of somewhat antagonistic effect between ILIs and OsbHLH157/OsbHLH158 in regulation of gene expression at the molecular level. This antagonism was also observed between ili1/3/4/7/8 and osibh1. About 2.74% (17/620) upregulated genes and 33.97% (124/365) downregulated genes of osibh1 were reversely coregulated in ili1/3/4/7/8 (Supplemental Fig. S12). These data suggested that OsbHLH157/OsbHLH158 and OsIBH1 likely synergistically antagonize ILIs to maintain the balance of BR signaling in rice.
Discussion
Knowledge about rice INCREASED LEAF INCLINATION (ILI) subfamily atypical bHLH transcription factors is still scarce and fragmentary. The member composition and nomenclature is indefinite and confusing, not to mention the endogenous biological function. On the basis of previous studies, as well as search against the rice genome database, a total of 8 ILI members, ILI1/OsbHLH154, ILI2, ILI3/OsbHLH153, ILI4/OsBU1/OsbHLH172, ILI5/OsBUL1/OsbHLH170, ILI6, ILI7/OsbHLH173, and ILI8/OsbHLH174, with substantial sequence similarity were identified (Supplemental Fig. S1, A to C). We and others showed that overexpression of the ILI subfamily genes in rice causes typical BR-enhanced phenotypes (Fig. 1; Supplemental Fig. S3; Tanaka et al. 2009; Zhang et al. 2009; Jang et al. 2017; Dong et al. 2018). Lamina inclination assay further confirmed the enhanced BR sensitivity of these ILIOx plants (Fig. 6, A and B). These data clearly demonstrate that the ILI subfamily members share conserved roles in modulation of BR signaling in rice. ILI2 and ILI6 were functional atypical bHLH transcription factors (Fig. 1); nevertheless, they had not been categorized as bHLH family proteins (Carretero-Paulet et al. 2010). This might be caused by sequence divergence of their HLH domain from the consensus. Notably, unlike the relatively high ratio of sterile plants in other ILIOx transgenes at T0 generation, the seed set of ILI6 and ILI7 overexpression transgenic lines were not remarkably affected. Also, the increased growth of the bottom fifth internode was differentially observed in these ILI overexpression plants (Fig. 1R; Supplemental Fig. S3A). Hence, in addition to the functional conservation, the members of ILI subfamily atypical bHLH also have some aspects of functional divergence during rice growth and development.
In the current model of rice BR signaling, ILI has long been regarded as an essential component of the downstream transcriptional regulation network (Tong et al. 2009; Zhang et al. 2009; Zhang et al. 2014; Cao et al. 2022). However, loss-of-function genetic evidence supporting the importance of ILI subfamily atypical bHLHs in the BR signaling pathway has not been fully established. Paclobutrazol Resistance (PRE) is homologous to rice ILIs and consists of 6 members (PRE1, PRE2, PRE3/ATBS1, PRE4, PRE5, and PRE6/KDR) in Arabidopsis (Lee et al. 2006; Wang et al. 2009). Overexpression of these PRE genes rescues the defects of bri1-301, revealing the functional homogeneity of ILI and PRE in regulation of BR signaling (Wang et al. 2009). The endogenous role of PRE and functional importance of ILI/PRE type atypical bHLH in plant remains to be elucidated. Here, through construction and analyses of numerous ili knockout mutants, we demonstrate that ILIs redundantly play pivotal roles in rice BR signaling pathway (Fig. 2; Supplemental Figs. S4 and S5). Compared with the relatively weak phenotypical alterations in single and double mutants, the ili3/4/5/8 quadruple and ili1/3/4/7/8 quintuple mutants exhibited multiple and severe BR-related defects, including drastic dwarfism (about 20 cm at the mature stage), completely erect leaves, dark green and tortuous leaf blades, and sterility (Fig. 2; Supplemental Figs. S4 and S5A). Exogenous application of 24-epiBL had no effect on the lamina joint inclination in ili1/3/4/7/8, indicating the aborted BR response of quintuple mutants (Fig. 6, A and B). Together, our results provide direct and solid genetic evidences that ILIs are indispensable component of BR signaling, and they act redundantly to regulate BR-mediated growth and development in rice.
Among the 8 ILIs, only ili4/osbu1 and ili5/osbul1 single mutants displayed relatively weak but significant BR-related phenotypes (Fig. 2; Jang et al. 2017), suggesting a dominant role of ILI4 and ILI5 in the subfamily. ILI4 and ILI5 are 2 homologous proteins sharing over 98.8% amino acid similarity and 90.8% amino acid identity (Supplemental Fig. S1D). Nevertheless, transcriptome data indicated that they likely have functional divergence at the molecular level because of the relative low percentage of common DEGs (Fig. 6, C and D). Many more DEGs were detected in ili1/3/4/7/8 quintuple mutant, which was consistent with the significantly more severe growth defects of ili1/3/4/7/8 (Supplemental Fig. S10). KEGG and GO analyses showed that pathways involved in plant hormone signal transduction, cell division, DNA-binding, cell wall, metabolism, and so on were enriched in ili1/3/4/7/8 (Fig. 6G; Supplemental Fig. S11A). This associates with the diverse effects of BR on plant growth and development.
Due to the deficiency of basic region, the non–DNA-binding atypical bHLH proteins usually function as an inhibitor of other HLH or bHLH proteins through heterodimerization (Toledo-Ortiz et al. 2003; Ikeda et al. 2012; Buti et al. 2020). ILI1 has been reported to physically interacts with and counteract the negative atypical bHLH, OsIBH1, to modulate leaf inclination. Notably, transgenic rice lines overexpressing OsIBH1 only exhibited a weak phenotype with small leaf angle and slightly dwarf stature (Zhang et al. 2009). In this study, we additionally constructed CRISPR-edited mutants of OsIBH1 (Supplemental Table S1). Contrast with the erect leaf of OsIBH1 overexpression rice plants, the osibh1 knockout mutant had increased leaf angle (unpublished data). This endogenous evidence further supported its negative role in regulation of leaf angle. Based on these observations, it seems that OsIBH1 might be mainly specified for regulation of leaf angle formation rather than function as a core component of BR signaling pathway in rice. Notably, ectopic overexpression of AtIBH1 in Arabidopsis results in remarkable BR-deficient phenotype with drastic dwarfism and dark green leaves (Zhang et al. 2009; Ikeda et al. 2012), reflecting the functional differentiation of IBH1 protein in these 2 species.
Considering the phenotypical discordance between ili high-order mutants and OsIBH1 overexpression transgenic lines, OsIBH1 alone should not be sufficient enough to balance the effect of ILIs. In this study, 2 additional ILI-interacting atypical bHLH proteins OsbHLH157 and OsbHLH158 were identified through yeast 2-hybrid assay (Fig. 3). OsbHLH157 and OsbHLH158 were deficient of the basic region and displayed no obvious transcriptional activity (Fig. 4, C and D; Supplemental Figs. S7C and S8). BR-enhanced growth with enlarged leaf angle and grain size was visible in osbhlh157 osbhlh158 double mutant, whereas overexpression of OsbHLH157 and OsbHLH158 led to a variety of BR-deficient growth defects (Fig. 5, C to N; Supplemental Fig. S9). Lamina inclination assay further supported the negative role of OsbHLH157 and OsbHLH158 in regulation of BR-mediated development (Fig. 6, A and B). Although OsbHLH157 and OsbHLH158 phylogenetically clustered with OsIBH1, they demonstrated multiple obvious differences. Firstly, the amino acid identity between OsbHLH157/OsbHLH158 and OsIBH1/AtIBH1 is low, ranging from only 22% to 26% (Supplemental Fig. S7B). Secondly, ectopic overexpression of OsbHLH157 and OsbHLH158 led to more serious developmental defects. For example, the mean plant height of OsbHLH157Ox-3 was just about 45 cm at the mature stage (Fig. 5, G to L). Hence, OsbHLH157 and OsbHLH158 likely function as major negative regulators of BR signaling in rice. Thirdly, in additional to BR-defective phenotype, BR-unrelated growth, for example, enlarged tiller angle was able to be observed in OsbHLH157 and OsbHLH158 overexpression lines (Fig. 5, G to J). For these reasons, OsbHLH157 and OsbHLH158 were not designated as IBH-like proteins in this study. RNA-seq data demonstrated osbhlh157 osbhlh158 and osibh1 knockout mutants had a large set of common DEGs (Fig. 6, E and F), indicating that OsbHLH157/OsbHLH158 and OsIBH1 likely share certain common transcription module in regulating downstream gene expression.
Gas-and-brake model involving triantagonistic HLH/bHLH (positive HLH, negative HLH, and typical bHLH transcription factor) has been proposed in plant (Ikeda et al. 2012; Buti et al. 2020). Atypical bHLHs, which can be positive or negative growth regulators, interact in an antagonistic manner to regulate cell elongation. Similarly, as paired interacting atypical bHLHs, ILIs and OsbHLH157/OsbHLH158 displayed opposite effects on diverse aspects of plant growth and development as well as response to BR. This antagonism was also confirmed at the molecular level. Approximately 16.71% (63/377) downregulated genes of osbhlh157 osbhlh158 were upregulated in ili1/3/4/7/8 (Fig. 6J). All together, these results suggested ILIs and OsbHLH157/OsbHLH158 form antagonistic system to maintain the balance of BR signaling. Interestingly, compared with osbhlh157 osbhlh158, a higher proportion of reversely coregulated genes was found between osibh1 and ili1/3/4/7/8 (Supplemental Fig. S12). One explanation is the presence of additional redundant factor (such as OsbHLH168) for OsbHLH157 and OsbHLH158 (Fig. 4B). OsbHLH168 also seems to be involved in BR signaling, since overexpression of OsbHLH168 in rice resulted in BR-related phenotype (unpublished data). Based on these results, we infer that OsbHLH157/OsbHLH158 likely work together with other atypical bHLHs, i.e., OsIBH1 and OsbHLH168, to antagonize ILIs to maintain the optimal levels of BR signaling in rice.
The negative atypical HLHs in the triantagonistic system mainly function through inhibiting other typical bHLH transcription factors, such as ACEs, BEEs, and HBI1 (Ikeda et al. 2012; Buti et al. 2020). The heterologous binding of the negative atypical HLHs to the typical bHLH transcription factors leads to the inhibition of their DNA-binding activities. On the other hand, the positive atypical HLH can further suppress the inhibitory activity of negative atypical HLH through forming heterodimers, which leads to the release of the typical bHLH transcription factor (Ikeda et al. 2012; Buti et al. 2020). As paired counteracting HLHs, ILIs and OsbHLH157/OsbHLH158 antagonistically play crucial roles in BR-mediated growth and development in rice. Nevertheless, it must be noted that both ILIs and OsbHLH157/OsbHLH158 are non–DNA-binding atypical bHLHs. They should not likely modulate rice development and gene expression through direct binding or competing to bind to the cis-activation elements. As mentioned, the antagonism of the positive and the negative atypical HLHs results in the release and derepression of the downstream typical bHLH transcription factor(s). In a sense, the BR-enhanced phenotype of osbhlh157 osbhlh158 double mutant to some extent could be attributed to the activation of downstream bHLH transcription factor(s). We analyzed the promoter sequence of the reversely coregulated DEGs between ili1/3/4/7/8 and osbhlh157 osbhlh158 knockout mutants (Fig. 6, I to K) using the Plant Promoter Analysis Navigator (PlantPAN4.0; Chow et al. 2019). The overwhelming majority of these common DEGs (78/83) contains at least 1 bHLH binding site in their 1.5 kb promoter region upstream of the start codon, supporting a potential association between ILI-OsbHLH157/OsbHLH158 module and bHLH transcription factor. Thus, we infer that the eliminations of OsbHLH157 and OsbHLH158 could bring about the derepression of the bHLH transcription factor(s) inhibited by them. Future characterization of OsbHLH157/OsbHLH158 inhibited typical bHLH(s) will further advance our understanding of the sophisticated BR signaling network in rice.
We focused on the characterization of ILIs-OsbHLH157/OsbHLH158 module in regulation of BR signaling in this study. Nevertheless, BR-independent phenotypes were apparently observed in knockout mutants or overexpression plants of ILIs and OsbHLH157/OsbHLH158. One of the most striking is the enlarged tiller angle in the high-order mutants of ILIs and OsbHLH157/OsbHLH158 overexpression transgenic rice plants (Figs. 2 and 5). Increasing studies suggest that BR participates in the regulation of rice tiller angle. For example, GWAS revealed that the BR biosynthetic enzyme gene DWARF2 (D2) contributes to the natural variation in the tiller angle during the domestication of japonica rice accessions (Dong et al. 2016). BR biosynthesis and signaling mutants, like d2, d11, oscpd1 oscpd2, d61, dlt, and rla1, usually develop more compact plant architecture with smaller tiller angle (Yamamuro et al. 2000; Sakamoto et al. 2006; Tong et al. 2012; Dong et al. 2016; Qiao et al. 2017; Zhan et al. 2022). On the other hand, overexpression of BR negative regulator, like OsGSK2, also leads to similar phenotype (Tong et al. 2012). These genetic evidences support a positive role of BR in tiller angle formation. Based on these observations, the enlarged tiller angle in ili1/3/4/7/8 quintuple mutants, as well as OsbHLH157 and OsbHLH158 overexpression rice plants, conceivably could not be explained by inactivation of BR signaling. During the past decades, identification of the wider tiller angle mutants revealed that auxin plays a fundamental role in rice tiller angle formation (Wang et al. 2022). RNA interference of OsPIN1 or overexpression of OsPIN2 increases tiller angle in rice (Xu et al. 2005, Chen et al. 2012). Three auxin response factors, OsARF12, OsARF17 and OsARF25, also have been demonstrated to control the rice tiller angle by fine-tuning the auxin asymmetric distribution (Li et al. 2020). OsbZIP49 positively regulates rice tiller angle by activating the transcription of indole-3-acetic acid–amido synthetase genes OsGH3-2 and OsGH3-13 (Ding et al. 2021). We analyzed the RNA-seq data of ili1/3/4/7/8 mutant, and multiple auxin related genes, including OsYUCCA2, OsPIN9, OsGH3-1, OsIAA20, and OsIAA21, were found to be differentially expressed in ili1/3/4/7/8 (Supplemental Table S2). On these grounds, we inferred that ILIs and OsbHLH157/OsbHLH158 module might integrate auxin pathway to control tiller angle.
Another interesting finding is the elongated fifth internode of ILI overexpression lines (Fig. 1R; Supplemental Fig. S3A). This phenomenon was also described and confirmed in the characterization of OsBU1/ILI4 overexpression plants, and it was proposed to be a characteristic BR effect on rice culm growth (Tanaka et al. 2009). However, the comprehensive comparison of the overexpression transgenic plants of ILI subfamily genes did not support this hypothesis, as ectopically overexpression of ILI6 and ILI8 resulted in marked BR-enhanced phenotype without promoting the extension of the fifth internode (Fig. 1R). More importantly, this increased fifth internode was not observed in Flag-aOsBZR1 overexpression transgenic rice plants either (Supplemental Fig. S13; Liu et al. 2021). Hence, the elongation of the fifth internode might likely be BR-unrelated and specific for ILI overexpression. Compared with the accumulating reports about the development of the first and second internode of the culm (Zhu et al. 2006; Gao et al. 2016; Niu et al. 2022), knowledge regarding the regulation of bottom internodes is quite limited. The genetic analysis of ILIs in this study is a step toward understanding the underlying mechanism of bottom internode growth. Moreover, in many rice germplasms, like ZH11, the contribution of the fifth internode to plant height is usually negligible because of its shortness (1∼2 cm at the mature stage). Here, our results suggested that fine-tune of plant height through manipulation of bottom internode is potentially feasible.
In conclusion, we report the systematic characterization of ILI subfamily atypical bHLHs in this study. Additionally, we identified 2 ILI-interacting downstream components OsbHLH157 and OsbHLH158. Our results demonstrate that ILIs redundantly play indispensable role in maintenance of plant architecture through BR signaling by counteracting OsbHLH157 and OsbHLH158 in rice.
Materials and methods
Plant materials and growth conditions
Rice (O. sativa) japonica cultivar ‘Zhonghua 11’ (ZH11) was used as the wild-type control in this study. Rice plants were cultivated in the field under natural conditions in Nanjing, China, as described previously (Zhan et al. 2022). Seedlings were germinated and grown in a growth chamber with 16-h light (30 °C)/8-h dark (28 °C) photoperiod and an approximate 300 μmol photons/m2/s light intensity. The relative humidity was maintained at ∼70%. Kimura's B solutions were used for seedling culture and changed twice per week.
Phylogenetic analysis
The full-length protein sequences of ILIs and OsbHLH157/OsbHLH158 homologs in rice and Arabidopsis (A. thaliana) were used to construct the phylogenetic tree by the neighbor-joining method with 1,000 bootstrap replicates using the software MEGA 11.0 (http://www.megasoftware.net/).
Plasmid construction, plant transformation, and characterization of targeted mutations by CRISPR/Cas9
To produce the CRISPR-knockout mutants, designed sgRNAs were synthesized and fused into the transgene killer CRISPR (TKC) gene editing system (He et al. 2018). For construction of overexpressing transgenic plants, the full-length open reading frame (ORF) of ILI genes and OsbHLH157/OsbHLH158 were amplified and cloned into the plasmid pU2301-TEV under the control of the maize ubiquitin (UBQ) promoter.
The target plasmids were transformed into calli of ZH11 through Agrobacterium-mediated rice transformation. T0 plants were transplanted to the normal condition rice field, and seeds were harvested separately.
Rice genomic DNA was extracted from CRISPR/Cas9 edited mutant plants by the cetyltrimethylammonium bromide (CTAB) method. Genomic regions of targeted sites were amplified with specific primers and sequenced (Supplemental Table S3). The sequence chromatograms were analyzed by web-based tool to check the genotype of the tested plants.
RNA extraction and RT-qPCR analysis
Total RNAs were extracted using the SteadyPure Plant RNA Extraction Kit (Accurate, Hunan, China) according to the manufacturer's instructions. The first-strand cDNAs were synthesized by reverse transcription using HiScript II 1st Strand cDNA Synthesis Kit (Vazyme, Nanjing, China). A RT-qPCR assay was performed with AceQ qPCR SYBR Green Master Mix (Vazyme, Nanjing, China) and amplification in the CFX Connect Real-Time PCR Detection System (Bio-Rad). OsActin1 was used as an internal control. The primers used are listed in Supplemental Table S3.
Yeast 2-hybrid assay
To create bait and prey constructs, the coding sequences of OsbHLH157, OsbHLH158, and ILI genes were cloned into the pGBKT7 or pGADT7 vector (Clontech). The resulting constructs and the corresponding empty vectors were transformed into Saccharomyces cerevisiae strain Golden Yeast with different combinations. Interactions were detected on dropout media without Trp/Leu/His/Ade (SD/-TLHA) medium. The transformation was performed as described in the Clontech Yeast Two-Hybrid System User Manual. The primers used for the yeast 2-hybrid assay are listed in Supplemental Table S3.
BiFC assay
For BiFC assays, the full-length coding sequences of ILIs, OsbHLH157, and OsbHLH158 were amplified and fused with the split GFP to generated ILIs-cYFP and OsbHLH157/OsbHLH158-nYFP. The primers used are listed in Supplemental Table S3. The resulting vectors were transiently expressed in leaves of N. benthamiana via A. tumefaciens infiltration. YFP fluorescence was assayed at 2 d after infiltration. The fluorescence signals were observed using a confocal laser scanning microscope (Nikon C2+). The YFP fluorescence was excited using a 513 nm laser beam, and emission was detected between 520 and 540 nm.
LUC complementation imaging assay
The full-length cDNA sequences of OsbHLH157/OsbHLH158 and ILIs were fused to the JW771-nLUC and JW772-cLUC vectors, respectively. The constructed plasmids were transformed into GV3101 Agrobacterium strain and further injected into N. benthamiana leaves via a needleless syringe. After 48 h growth, luciferin (Yeasen, Shanghai, China) was infiltrated into the leaves, and the firefly LUC signal was visualized using the Tanon-5500 Chemiluminescent Imaging System (Tanon, Shanghai, China).
Subcellular localization
The full-length CDS of ILIs, OsbHLH157, and OsbHLH158 were amplified by PCR with gene-specific primers (Supplemental Table S3) and cloned into GFP-containing plasmid under the control of CaMV 35S promoter. The GFP fusion plasmids were first transformed into Agrobacterium strain GV3101 and then injected into the leaf epidermal cells of N. benthamiana using 1 mL needless syringe. The DAPI (1 μg/mL) was added to visualize the nuclei. The fluorescence signals were observed using a confocal laser scanning microscope (Nikon C2+). The GFP fluorescence was excited using a 488 nm laser beam and emission was detected using a 510 nm bandpass filter.
Transcription activation analysis
To investigate the transcriptional activities of OsbHLH157 and OsbHLH158, the full-length ORF of OsbHLH157 and OsbHLH158 were cloned into pGBKT7 vector and transferred into yeast strain Y2H using the high-efficiency lithium acetate transformation procedure. The yeast transformants were then plated on selective SD-Trp/Leu/His medium to test transcriptional activity.
Transactivation analysis was also performed in rice protoplasts using the dual-LUC reporter system. The coding sequence of OsbHLH157 and OsbHLH158 gene were amplified and fused to GAL4-BD to generate the effector plasmids. The reporter vector containing the firefly LUC was driven by the 35S minimal TATA box and 5 copies of the GAL4-binding element (5 × GAL4). Renilla LUC (REN) in the same vector driven by the 35S promoter was used as the internal control. The reporter and effector plasmids were cotransfected into rice protoplasts by PEG-mediated transfection. Transfected protoplasts were then suspended in W5 buffer and kept in darkness for 12 h. The dual-LUC activity was determined using the Dual-Luciferase Reporter Assay System Kit (E1910, Promega, USA) according to the manufacturer's instructions.
BR sensitivity test
24-epiBL (Sigma Aldrich, E1641) was dissolved in DMSO to prepare the stock solution. For leaf bending assay, germinated seeds were cultivated in Kimura's B solution, and the third leaf laminas from 14-d-old seedlings with uniform growth were cut. All segments, containing about 1 cm of the leaf blade, the lamina joint and the leaf sheath, were immersed in 24-epiBL solutions for another 2 d in the dark.
RNA-Seq and data analysis
The wild-type ZH11, ili4-c1, ili5-c1, ili1/3/4/7/8-c1, osbhlh157 osbhlh158-c1, and osibh1-c1 mutant seedlings were germinated and cultured in Kimura's B solution for 2 wks. The aerial parts of the plant were then harvested for RNA extraction, and 3 biological replicates of each sample were prepared. The RNA-seq analysis was performed by the Novogene Bioinformatics Technologies Co. Ltd (Beijing). Total amounts and integrity of RNA were assessed using the RNA Nano 6000 Assay Kit of the Bioanalyzer 2100 system (Agilent Technologies, CA, USA). RNA sequencing was conducted on Illumina NovaSeq platform. Differential expression analysis was performed using the DESeq2 R package (1.20.0), and |log2(foldchange)|>1 and P value < 0.05 were set as the threshold for significantly differential expression.
Gene Ontology (GO) enrichment analysis of DEGs was implemented by the clusterProfiler R package (3.8.1), in which gene length bias was corrected. GO terms with corrected P value < 0.05 were considered significantly enriched by DEGs. The clusterProfiler R package (3.8.1) was used to test the statistical enrichment of DEGs in KEGG pathways (http://www.genome.jp/kegg/).
Statistical analysis
Statistical significance was determined by Student’s t test and 1-way ANOVA with Tukey's honestly significant differences test using GraphPad Prism 8.0 software. Data for quantitative analyses are presented as means ± Sd.
Accession numbers
Sequence data from this article can be found in the GenBank/EMBL data libraries under the following accession numbers: ILI1 (LOC_Os04g54900); ILI2 (LOC_Os11g39000); ILI3 (LOC_Os03g07540); ILI4 (LOC_Os06g12210); ILI5 (LOC_Os02g51320); ILI6 (LOC_Os03g07510); ILI7 (LOC_Os10g26460); ILI8 (LOC_Os10g26410), OsbHLH157 (LOC_Os02g08220); OsbHLH158 (LOC_Os06g44320); OsbHLH168 (LOC_Os02g54870); OsIBH1 (LOC_Os04g56500); OsACTIN1 (LOC_Os03g50885); AtP1R1 (AT5G57780); AtIBL1 (AT4G30410); and AtIBH1 (AT2G43060).
Acknowledgments
We are grateful to Prof. Zhigang Zhao (Nanjing Agricultural University, China) for helpful discussion and revision. We thank Prof. Hongning Tong (Chinese Academy of Agricultural Sciences, China) for providing the Flag-aOsBZR1 overexpression transgenic rice seeds and Prof. Chunhao Jiang (Nanjing Agricultural University, China) for providing the BiFC vectors.
Author contributions
H.Z. conceived the project and designed the study. H.Z., Mingq.L., Mingm.L., and Z.Z. performed the experiments with the help from Q.L., F.L., J.Z., Y.H., and Y.T. H.Z., Mingq.L., and Z.Z. analyzed the data. H.Z. wrote the manuscript. All authors read and approved the manuscript.
Supplemental data
The following materials are available in the online version of this article.
Supplemental Figure S1. Member information of ILI subfamily atypical bHLHs.
Supplemental Figure S2. RT-qPCR analysis of ILI expression in wild-type and overexpression transgenic lines.
Supplemental Figure S3. Phenotypical quantification of the ILI overexpression transgenic rice.
Supplemental Figure S4. Construction of ili3/4/5/8 quadruple mutant.
Supplemental Figure S5. Phenotypical quantification of ili knockout mutants.
Supplemental Figure S6. Growth of yeast transformants with the ILIs-AD and empty-BD on SD-TL (lacking Trp and Leu) and SD-TLHA (lacking Trp, Leu, His, and Ade) selective medium.
Supplemental Figure S7. Sequence analysis of OsbHLH157/OsbHLH158 and their homologs in rice and Arabidopsis.
Supplemental Figure S8. Transcription activation of OsbHLH157 and OsbHLH158 in yeast cells.
Supplemental Figure S9. Quantification of grain length, width, and weight of OsbHLH157/OsbHLH158 knockout mutant and overexpression rice plants.
Supplemental Figure S10. Volcano plots of the DEGs.
Supplemental Figure S11. GO enrichment analysis of DEGs in ili1/3/4/7/8 and osbhlh157 osbhlh158 mutants.
Supplemental Figure S12. Venn diagram for the overlap of the reversely coregulated DEGs between ili4, ili5, ili1/3/4/7/8, and osibh1 knockout mutants.
Supplemental Figure S13. Morphologies of wild-type and Flag-aOsBZR1 overexpression transgenic rice plants.
Supplemental Table S1. Sequence information of CRISPR-edited knockout mutants used in this study.
Supplemental Table S2. List of DEGs in ili4-c1, ili5-c1, ili1/3/4/7/8-c1, osbhlh157 osbhlh158-c1, and osibh1-c1 knockout mutants.
Supplemental Table S3. Primers used in this study.
Funding
This work was supported by grants from the National Natural Science Foundation of China (31700277), the Key Research and Development Program of Jiangsu Province (BE2022365), the Jiangsu Agricultural Science and Technology Innovation Fund (JASTIF) (CX(21)3105), the Natural Science Foundation of Jiangsu Province (61HB200032), and the National Transgenic Science and Technology Program of China (2019ZX08010-001).
Data availability
The data underlying this article are available in the article and in its online supplementary material.
Dive Curated Terms
The following phenotypic, genotypic, and functional terms are of significance to the work described in this paper:
References
Author notes
These authors contributed equally.
The author responsible for distribution of materials integral to the findings presented in this article in accordance with the policy described in the Instructions for Authors (https://dbpia.nl.go.kr/plphys/pages/General-Instructions) is Huadong Zhan.
Conflict of interest statement. None declared.