-
PDF
- Split View
-
Views
-
Cite
Cite
Guoren He, Yuwei Cao, Jing Wang, Meng Song, Mengmeng Bi, Yuchao Tang, Leifeng Xu, Jun Ming, Panpan Yang, WUSCHEL-related homeobox genes cooperate with cytokinin to promote bulbil formation in Lilium lancifolium, Plant Physiology, Volume 190, Issue 1, September 2022, Pages 387–402, https://doi.org/10.1093/plphys/kiac259
- Share Icon Share
Abstract
The bulbil is an important vegetative reproductive organ in triploid tiger lily (Lilium lancifolium). Based on our previously obtained transcriptome data, we screened two WUSCHEL-related homeobox (WOX) genes closely related to bulbil formation, LlWOX9 and LlWOX11. However, the biological functions and regulatory mechanisms of LlWOX9 and LlWOX11 are unclear. In this study, we cloned the full-length coding sequences of LlWOX9 and LlWOX11. Transgenic Arabidopsis (Arabidopsis thaliana) showed increased branch numbers, and the overexpression of LlWOX9 and LlWOX11 in stem segments promoted bulbil formation, while the silencing of LlWOX9 and LlWOX11 inhibited bulbil formation, indicating that LlWOX9 and LlWOX11 are positive regulators of bulbil formation. Cytokinin type-B response regulators could bind to the promoters of LlWOX9 and LlWOX11 and promote their transcription. LlWOX11 could enhance cytokinin pathway signaling by inhibiting the transcription of type-A LlRR9. Our study enriches the understanding of the regulation of plant development by the WOX gene family and lays a foundation for further research on the molecular mechanism of bulbil formation in lily.
Introduction
Lilium lancifolium, also known as tiger lily, is an important Lilium species of the Liliaceae family. Liliumlancifolium shows high adaptability and is widely cultivated in China for its edible bulbs and medicinal applications (Liang and Tamura, 2000; China Pharmacopoeia Committee, 2005; Yu et al., 2015), with a production value of ∼6 billion Yuan per year. Liliumlancifolium is a natural triploid and cannot be propagated sexually, but its leaf axils can form a large number of purple-black bulbils (Bach and Sochacki, 2012; Chung et al., 2015). Bulbils grow on leaf axils and can naturally fall off the mother plant and develop into a new complete individual after maturity (Yang et al., 2017). The bulbil propagation strategy has the advantages of high efficiency and better retention of maternal genetic characteristics and is therefore the main reproductive strategy for L. lancifolium.
Bulbils are a special and important type of reproductive organ in plants and are only formed in a few plant species, such as yam (Dioscorea batatas), garlic (Allium sativum), Titanotrichum oldhamii, Pinellia (Pinellia ternate), tequila (Agave tequilana), and Lilium species (Wang et al., 2004; Bell and Bryan, 2008; Abraham-Juárez et al., 2010; Sandoval et al., 2012; Yang et al., 2017). The formation of bulbils is a complex developmental process that is regulated by genetic and environmental factors and phytohormones.
Plant hormones, especially auxin and cytokinin, have been proven to be involved in the regulation of bulbil formation, in which auxin inhibits bulbil formation, whereas cytokinin promotes the bulbil formation (Wang and Cronk, 2003; Peng et al., 2005; Abraham-Juárez et al., 2015; Navarro et al., 2015; He et al., 2020). Before bulbil initiation in Disocorea polystachya, auxin rapidly accumulates in the leaf axil, followed by the expression of auxin transport genes, such as AUXIN RESPONSE FACTORs (ARF9 and ARF18), AUXIN-INDUCED PROTEIN 15A (AX15A), and AUXIN-INDUCED PROTEIN 22D (AUX22D), resulting in auxin outflow from the leaf axil and bulbil initiation (Wu et al., 2020). AUXIN EFFLUX CARRIER COMPONENT 1 (AtqPIN1 and AtqSoPIN1) participates in auxin outflow in A. tequilana (Abraham-Juárez et al., 2015). In D. polystachya, the expression of CYTOKININ OXIDASE/DEHYDROGENASE genes (CKX1, CKX3, CKX9, and CKX11) is decreased before bulblet initiation and leads to the accumulation of cytokinin in the leaf axil (Wu et al., 2020). In a previous study, we revealed that iP-type cytokinins were the most important cytokinins during bulbil formation and showed that the accumulation of iP-type cytokinins was mainly due to the upregulation of cytokinin biosynthesis genes (ADENYLATE ISOPENTENYLTRANSFERASEs: IPT1 and IPT5) and cytokinin activation genes (CYTOKININ RIBOSIDE 5'-MONOPHOSPHATE PHOSPHORIBOHYDROLASEs: LOG1, LOG3, LOG5, and LOG7) and the substantial downregulation of cytokinin degradation gene (CKX4) expression (He et al., 2020).
As a special type of axillary organ, bulbils originate from the axillary meristem (AM). A recent study revealed that cytokinins can promote AM initiation through cytokinin type-B RESPONSE REGULATORs (type-B RRs) (Wang et al., 2017). Type-B RRs are positive regulatory transcription factors in cytokinin signaling and mostly modulate the transcription of cytokinin-regulated genes by directly binding target DNA sequences at their C-terminal MYB domains (Hosoda et al., 2002; Kieber and Schaller, 2014). In Arabidopsis (Arabidopsis thaliana), cytokinin signaling is mainly mediated by five members of type-B RR subfamily I: ARR1, ARR2, ARR10, ARR11, and ARR12 (Mason et al., 2004, 2005; Schaller et al., 2007; Yokoyama et al., 2007; Ishida et al., 2008; Tsai et al., 2012). In regulating axillary bud formation, type-B RRs act as key transcriptional regulators involved in AM initiation. ARR1 can directly bind to the WUSCHEL (WUS) promoter and activate the transcription of WUS; ARR2, ARR10, ARR11, and ARR12 can also activate the expression of WUS, indicating that type-B ARRs show functional redundancy in regulating the expression of WUS, in which ARR1 is the key regulatory factor (Wang et al., 2014a, 2014b, 2017).
Regarding the molecular regulation of bulbil formation, however, only a small number of genes related to bulbil formation have been identified to date, and the associated regulatory mechanism is not clear. In A. tequilana, HOMEOBOX PROTEIN KNOTTED-1-LIKE 1 (AtqKNOX1) and AtqKNOX2 are expressed at the beginning of globular bud formation and are specifically expressed during meristem development (Abraham-Juárez et al., 2010). The expression of some AtqMADS genes is decreased during bulbil formation, indicating that AtqMADS genes may be negatively related to bulbil formation in this species (Sandoval et al., 2012). In T. oldhamii, the expression of Gesneriaceae-FLORICAULA (GFLO) is also downregulated during bulbil formation, indicating that GFLO acts as a negative regulator during bulbil formation (Wang et al., 2004). The AGO protein mediates the silencing of downstream genes through miRNA. In L. lancifolium, LlAGO1 is specifically expressed in the bulbil and upregulated during bulbil formation, which indicates that the miRNA pathway may also be involved in the regulation of bulbil formation (Yang et al., 2018).
The WUS-related homeobox (WOX) proteins are a plant-specific family within the eukaryotic homeobox transcription actor superfamily characterized by a conserved N-terminal homeodomain consisting of 60–66 amino acids (Mayer et al., 1998; Haecker et al., 2004). Functional studies have revealed that the WOX transcription factors play important roles in promoting cell division, preventing immature cells from differentiating, embryonic development, stem cell niche maintenance in the meristem, and organ formation (Stahl et al., 2009; Van Der Graaff et al., 2009; Yadav et al., 2011). Based on the phylogenetic analysis and the distribution of WOX genes in the plant kingdom, they have been classified into three clades: a modern/WUS clade (found in seed plants), an intermediate/WOX9 clade (found in vascular plants including lycophytes), and an ancient/WOX13 clade (found in vascular and nonvascular plants, including mosses and green algae; Nardmann et al., 2009; Van Der Graaff et al., 2009).
Some members of the WOX gene family have been shown to be involved in the regulation of AM. In A. thaliana, WUS is essential for the initiation and maintenance of AM (Wang et al., 2014a, 2014b). Unlike the situation in A. thaliana, the AM of rice (Oryza sativa) is coregulated by OsWUS and OsWOX4. OsWUS is expressed only before meristem formation and not in the established AM, and OsWOX4 is expressed only in the established AM, indicating that OsWOX4 functions only in maintaining meristem activity (Ohmori et al., 2013; Lu et al., 2015; Tanaka et al., 2015). WOX9 and WOX11 are members of the intermediate clade and regulate the shoot meristem or AM. In the A. thaliana wox9 mutant, the development of the embryo, apical meristem, and root meristem is abnormal, and the growth and development of the axillary buds and roots is substantially inhibited (Skylar et al., 2010; Skylar and Wu, 2010). In addition, the loss of WUS expression in the wox9 mutant indicates that WOX9 can positively regulate the expression of WUS (Wu et al., 2005). In O. sativa, OsWOX9 (Dwarftiller1 [DWT1]) plays an important role in the development of rice tillers, and the dwt1 mutant shows shorter tillers and a reduced tiller number (Wang et al., 2014c). In A. thaliana and O. sativa, WOX11 mainly regulates the crown root primordium (Liu et al., 2014; Hu and Xu, 2016). wox11 mutants show crown root number and growth rate deficiencies, a dwarf phenotype, and delayed flowering (Zhao et al., 2009). In crown and root development, OsWOX11 mediates the cytokinin pathway by inhibiting the expression of type-A OsRR2, thus enhancing cytokinin signaling to promote crown and root formation (Nardmann and Werr, 2006; Zhao et al., 2009). A recent study revealed that in addition to its function in crown root development, OsWOX11 is also required for rice shoot development and can activate gene expression during the development of the rice shoot apical meristem by recruiting the H3K27me3 demethylase JMJ705 (Cheng et al., 2018).
On the basis of transcriptome data (accession number: SRP103184), we screened the expression of all annotated WOX genes during bulbil formation and identified two WOX genes closely related to bulbil formation, LlWOX9 and LlWOX11 (Supplemental Figure S1). In this study, our results showed that LlWOX9 and LlWOX11 were members of the intermediate clade and that their expression increased continuously during bulbil formation. The overexpression of LlWOX9 and LlWOX11 promoted bulbil formation, while the silencing of LlWOX9 and LlWOX11 inhibited bulbil formation, indicating that LlWOX9 and LlWOX11 are positive regulators of bulbil formation. Cytokinin type-B LlRRs can bind to the promoters of LlWOX9 and LlWOX11 to promote their transcription. In addition, LlWOX11 can enhance cytokinin signaling by inhibiting the transcription of type-A LlRR9. Our study enriches the understanding of the roles of the WOX gene family in regulating plant development. We also show that WOX genes cooperate with cytokinins to regulate the formation of bulbils. Our study lays a foundation for further research on the molecular mechanism of bulbil formation in lily.
Results
Full-length cloning and sequence analysis of LlWOX9 and LlWOX11
On the basis of transcriptome data (accession number: SRP103184), we cloned the full-length sequences of LlWOX9 (1,008 bp) and LlWOX11 (699 bp) by RNA ligase-mediated rapid amplification of 5' and 3' cDNA ends (RLM-RACE) and found that they encoded 335 and 232 amino acids, respectively (Figure 1A). Amino acid sequence analysis showed that both LlWOX9 and LlWOX11 contained HOX domains at the N-terminus (Figure 1A). Sequence alignment confirmed a conserved HOX domain at the N-terminus in LlWOX9 and LlWOX11 (Supplemental Figure S2). A phylogenetic tree of LlWOX9, LlWOX11, and the members of the WOX transcription factor family in A. thaliana was constructed, and the results showed that LlWOX9 and LlWOX11 belonged to the intermediate evolutionary branch of the WOX family (Supplemental Figure S3). Phylogenetic tree of WOX9 and WOX11 from different species showed that LlWOX9 and LlWOX11 were clustered with the sequences of other monocotyledonous species and were closely related to the WOX9 and WOX11 amino acid sequences of Palmaceae plants (Figure 1, B and C).
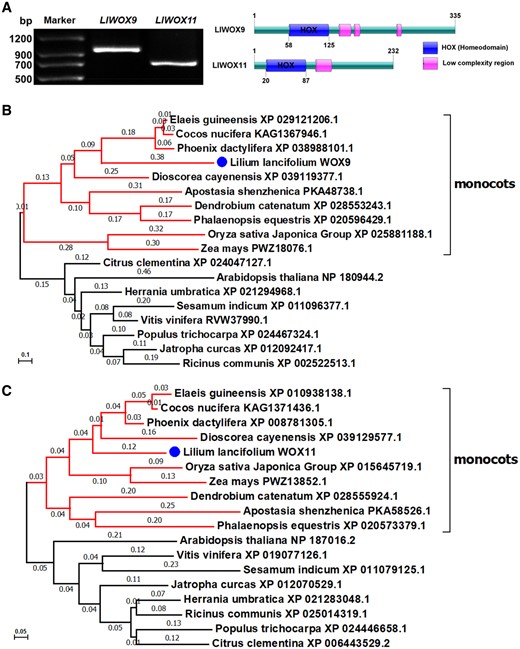
Full-length cloning and phylogenetic tree of LlWOX9 and LlWOX11. A, Full-length cloning and domain prediction of LlWOX9 and LlWOX11. B, Neighbor-joining tree of the LlWOX9 amino acid sequence of L. lancifolium and WOX9 amino acid sequences from other species. C, Neighbor-joining tree of the LlWOX11 amino acid sequence of L. lancifolium and WOX11 amino acid sequences from other species. Bootstrap values from 1,000 replicates were used to assess the robustness of the tree.
Expression pattern and subcellular localization of LlWOX9 and LlWOX11
To study the subcellular localization of LlWOX9 and LlWOX11, we fused the LlWOX9 and LlWOX11 proteins with a green fluorescent protein (GFP) tag and introduced them into the leaves of L. lancifolium. The subcellular localization results showed that the GFP signals of the LlWOX9-GFP and LlWOX11-GFP fusion proteins were located in the nuclei of L. lancifolium leaf epidermal cells (Figure 2A), indicating that LlWOX9 and LlWOX11 may function as transcription factors in the nucleus.
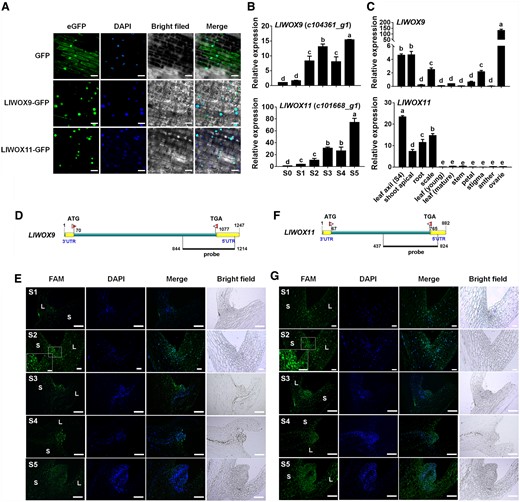
Subcellular localization, expression patterns, and FISH of LlWOX9 and LlWOX11. A, Subcellular localization of LlWOX9-GFP and LlWOX11-GFP proteins in L. lancifolium leaf epidermal cells with DAPI staining. Scale bars = 50 μm. B, LlWOX9 and LlWOX11 expression during bulbil formation. C, LlWOX9 and LlWOX11 expression in different tissues. Values are means ± sd (n = 3). Lowercase letters (a–d in B; a–e in C) indicate significantly different values (Student’s t test, P < 0.05). D, Gene-specific probe of LlWOX9 used in FISH. E, FISH of LlWOX9 during bulbil formation. F, Gene-specific probe of LlWOX11 used in FISH. G, FISH of LlWOX11 during bulbil formation. L, leaf; S, stem. Scale bar in (A) S2 and (B) S1 and S2, 100 μm. Scale bar in (A) S1 and S3–S5 and (B) S3–S5, 500 μm.
The expression of LlWOX9 and LlWOX11 increased continuously during bulbil formation (Figure 2B). LlWOX9 was mainly expressed in the leaf axil (S4 stage), shoot apical meristem, scale, stigma, and ovary, with the highest relative expression in the ovary and the second highest in the leaf axil (Figure 2C). LlWOX11 was mainly expressed in the leaf axil (S4 stage), shoot apical tissue, root, and scale, and the highest relative expression was found in the leaf axil (S4 stage; Figure 2C). The relatively high expression of LlWOX9 and LlWOX11 in leaf axils further indicated that LlWOX9 and LlWOX11 might be involved in bulbil formation.
We further detected the expression of LlWOX9 and LlWOX11 during bulbil formation by fluorescence in situ hybridization (FISH). Gene-specific sequences containing the 3′-UTRs (untranslated region) of LlWOX9 and LlWOX11 were selected to synthesize the FAM-labeled fluorescent probes (Figure 2, D and F). Our results showed that although the LlWOX9 and LlWOX11 fluorescent signals could be detected throughout the analyzed tissue, the fluorescent signals of LlWOX9 and LlWOX11 were mainly located in the leaf axil and gradually increased during bulbil formation (Figure 2, E and G) and no fluorescent signal in negative controls (Supplemental Figure S4). In addition, the fluorescent signals of LlWOX9 and LlWOX11 appeared on a differentiated scale (S5 stage) (Figure 2, E and G). These results further indicated that LlWOX9 and LlWOX11 are involved not only in the formation of the bulbil primordium but also in the differentiation of the bulbil scale.
Overexpression of LlWOX9 and LlWOX11 increases the number of branches in A. thaliana
Lily bulbils form in leaf axils and belong to axillary organs, similar to branches of A. thaliana, which originate from the AM. Since a stable genetic system has not been established in L. lancifolium, to study whether LlWOX9 and LlWOX11 could play a role in axillary organ formation, we generated transgenic lines of overexpression LlWOX9 and LlWOX11 in A. thaliana. [AQ]The band of ∼1,200 or ∼800 bp was amplified from the genomic DNA of the transgenic lines, and no corresponding bands were amplified from control plants (Figure 3A). Our results demonstrated that overexpression of LlWOX9 or LlWOX11 in A. thaliana increased the number of branches and promoted the formation of accessory buds on inflorescences (Figure 3, B, C, E, and F). The number of branches was significantly higher in the 35S:LlWOX9 and 35S:LlWOX11 transgenic lines than in the wild-type (Figure 3, D and G). Interestingly, we found that the 35S:LlWOX9 transgenic lines showed some abnormal phenotypes, such as the development of the inflorescence branches into a single flower and the abnormal elongation of stem internodes in rosette leaves (Figure 3C).
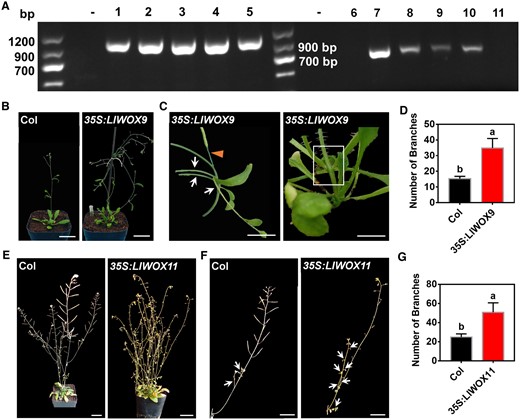
The phenotypes of 35S:LlWOX9 and 35S:LlWOX11 transgenic lines and wild-type A. thaliana plants. A, The transgenic plants of the T3 generation of A. thaliana were detected by PCR. “−” indicates the negative control. 1–5 represent different transgenic lines overexpressing LlWOX9, 6–11 represent different transgenic lines overexpressing LlWOX11. B, The branching phenotypes of wild-type Col and transgenic plants overexpressing LlWOX9. Scale bar is 3 cm. C, The phenotypes of transgenic plants overexpressing LlWOX9. The arrows represent multiple branches in one leaf axil; the triangle represents abnormal branches, and the white box represents abnormal elongation of stem internodes. Scale bar is 1 cm. D, The numbers of branches on wild-type Col and transgenic plants overexpressing LlWOX9. n = 10 plants for each repeat. Values are means ± sd (n = 3). Lowercase letters in (c) and (e) indicate significantly different values (Student’s t test, P < 0.05). E, The branching phenotypes of wild-type Col and transgenic plants overexpressing LlWOX11. Scale bar is 3 cm. F, The inflorescence branching phenotypes of wild-type Col and transgenic plants overexpressing LlWOX11. The arrows represent branches in leaf axil. Scale bar is 2 cm. Images in (B), (C), (E), and (F) were digitally extracted for comparison. G, The numbers of branches on wild-type Col and transgenic plants overexpressing LlWOX11. n = 10 plants for each repeat. Values are means ± sd (n = 3). Lowercase letters in (C) and (E) indicate significantly different values (Student’s t test, P < 0.05).
LlWOX9 and LlWOX11 overexpression promotes bulbil formation
To preliminarily understand the functions of LlWOX9 and LlWOX11 during bulbil formation, we further evaluated the functions of LlWOX9 and LlWOX11 via their transient overexpression in leaf axils through an in vitro bulbil induction system. Our results showed that after 6 days of culture, most of the developing leaf axils in the control group and the 35S:GUS treatment group were still in the S3 stage (Figure 4A), but the overexpression of LlWOX9 and LlWOX11 could substantially promote the formation of bulbils (Figure 4A), and the rate of bulbil induction was significantly higher than that in the control group and the 35S:GUS treatment group (Figure 4B). These results indicated that LlWOX9 and LlWOX11 play important roles during bulbil formation.
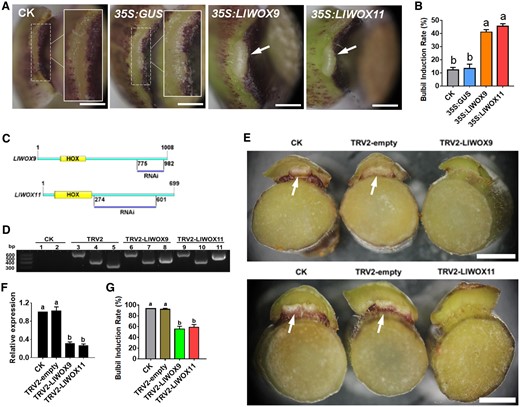
Phenotype and relative expression of LlWOX9 and LlWOX11 in leaf axils after overexpressing or silencing LlWOX9 and LlWOX11. A, The phenotype of the leaf axil after the transient overexpression of LlWOX9 and LlWOX11. The white boxes show an enlargement of the indicated portion of the leaf axil. The arrows show the bulbil has formed in leaf axil. Scale bar is 1 mm. B, The bulbil induction rate after the transient overexpression of LlWOX9 and LlWOX11. Values are means ± sd (n = 3). Lowercase letters indicate significantly different values (Student’s t test, P < 0.05). C, Specific fragments of genes used in VIGS experiments. D, PCR was used to detect the presence of the TRV1 and TRV2 viruses in the leaf axils. CK is the negative control, TRV2 is the positive control. Lanes 1, 3, 6, and 9 show TRV1 detection; 2, 4, 7, and 10 show the detection of coat proteins in TRV2; and lanes 5, 8, and 11 show the detection of inserts in TRV2. E, The phenotype of the leaf axil after silencing LlWOX9 and LlWOX11. The arrows show the bulbil has formed in leaf axil. Scale bar is 5 mm. F, The relative expression of LlWOX9 and LlWOX11 in leaf axils after silencing LlWOX9 and LlWOX11. n = 20 plants for each repeat. Values are means ± sd (n = 3). Lowercase letters in (B), (F), and (G) indicate significantly different values (Student’s t test, P < 0.05). G, The bulbil induction rate after silencing LlWOX9 and LlWOX11. n = 20 plants for each repeat. Values are means ± sd (n = 3). Lowercase letters indicate significantly different values (Student’s t test, P < 0.05).
LlWOX9 and LlWOX11 silencing inhibits bulbil formation
To further understand the functions of LlWOX9 and LlWOX11 during bulbil formation, we constructed the TRV2-LlWOX9 and TRV2-LlWOX11 silencing vectors by selecting specific fragments of the LlWOX9 and LlWOX11 genes (Figure 4C). After 12 days of infection with the empty TRV2 vector and the recombinant TRV2-LlWOX9 or TRV2-LlWOX11 vector, leaf axil cDNAs were obtained, and TRV1-F/R and TRV2-F/R were used for polymerase chain reaction (PCR)-based detection. The results showed that in leaf axils infected with the empty TRV2 vector, TRV2-LlWOX9, or TRV2-LlWOX11, the target bands of pTRV1, the coat protein in pTRV2 and the insert fragment in pTRV2 could be detected (Figure 4D). These results indicated that TRV2, TRV2-LlWOX9, and TRV2-LlWOX11 were successfully inserted and expressed in the genome of L. lancifolium.
The silencing of the LlWOX9 and LlWOX11 genes in leaf axils was detected by reverse transcription-quantitative PCR (RT-qPCR). The results showed that the expression of LlWOX9 and LlWOX11 in leaf axils infected with TRV2-LlWOX9 or TRV2-LlWOX11 was significantly lower than that in the control and the leaf axils infected with TRV2 (Figure 4F). These findings indicated that LlWOX9 and LlWOX11 were effectively silenced in TRV2-LlWOX9- and TRV2-LlWOX11-infected leaf axils, respectively.
The silencing experiment results showed that after LlWOX9 and LlWOX11 silencing, the formation of bulbils was delayed (Figure 4E). In the control group, the induction rate of bulbils reached nearly 100% after 12 days of culture, while in the silence groups were only ∼50% (Figure 4G). Other stem segments in silence groups that did not form bulbils were delayed in forming bulbils after 20 days of culture. These results indicated that LlWOX9 and LlWOX11 play important roles by positively regulating bulbil formation.
Cytokinins induce the expression of LlWOX9 and LlWOX11
Our previous studies have revealed that cytokinins can promote bulbil formation through type-B RRs. To study whether the expression of LlWOX9 and LlWOX11 is regulated by cytokinins, we detected the expression of LlWOX9 and LlWOX11 after exogenous cytokinin treatment and the silencing of type-B LlRRs. The results showed that after treatment with 6-BA, the expression of LlWOX9 and LlWOX11 during bulbil formation was significantly higher than in the control group (Figure 5A). To further study whether the expression of LlWOX9 and LlWOX11 was directly induced by exogenous cytokinins, we treated leaf axils at the S4 stage with 6-BA. The results showed that after exogenous 6-BA treatment, the expression of LlWOX9 and LlWOX11 was rapidly induced (Figure 5B), indicating that exogenous cytokinins could induce the expression of LlWOX9 and LlWOX11. In addition, because cytokinins regulate downstream genes through type-B RRs, we detected the expression of LlWOX9 and LlWOX11 after the silencing of five type-B LlRRs in leaf axils. The results showed that the expression of LlWOX9 and LlWOX11 decreased significantly after the silencing of a single type-B LlRR gene, while after the silencing of five type-B LlRRs, the relative expression of LlWOX9 and LlWOX11 was almost undetectable (Figure 5C). These results suggest that cytokinins can induce the expression of LlWOX9 and LlWOX11 and that type-B LlRRs may directly regulate the expression of LlWOX9 and LlWOX11.
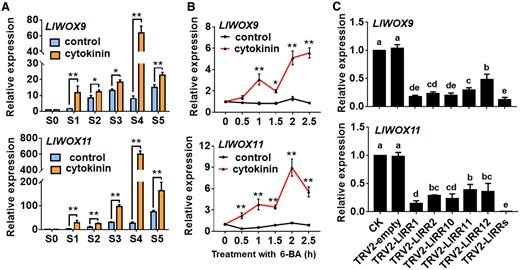
Expression of LlWOX9 and LlWOX11 after treatment with 6-BA and type-B LlRR silencing. A, Expression of LlWOX9 and LlWOX11 after treatment with 4-μM 6-BA during bulbil formation. Values are means ± sd (n = 3). Asterisks indicate significantly different values (Student’s t test, *P < 0.05 and **P < 0.01). B, Expression of LlWOX9 and LlWOX11 in leaf axils at stage S4 after 10-mM 6-BA treatment. Values are means ± sd (n = 3). Asterisks indicate significantly different values (Student’s t test, *P < 0.05 and **P < 0.01). C, Expression of LlWOX9 and LlWOX11 after type-B LlRR silencing. Values are means sd (n = 3). Lowercase letters indicate significantly different values (Student’s t test, P < 0.05).
Type-B LlRRs promote the transcription of LlWOX9 and LlWOX11
To study whether type-B LlRRs regulate the transcription of LlWOX9 and LlWOX11, we first cloned the promoter sequences of LlWOX9 and LlWOX11 via the chromosome walking technique and obtained promoters with lengths of 1,318 and 2,351 bp, respectively (Figure 6A). The use of the New Place and PlantCARE online element prediction tools showed that the promoters of LlWOX9 and LlWOX11 contained a large number of type-B RR-binding elements (NGATT/C) (Figure 6A). Furthermore, we studied the promoter activities of LlWOX9 and LlWOX11 in L. lancifolium stem segments. GUS staining results showed that both the LlWOX9 and LlWOX11 promoters were active and that their activity was weaker than that of the 35S promoter (Figure 6B). We also observed stronger GUS staining of the promoters of LlWOX9 and LlWOX11 in the leaf axils (Figure 6B), consistent with the higher expression of LlWOX9 and LlWOX11 in the leaf axils.
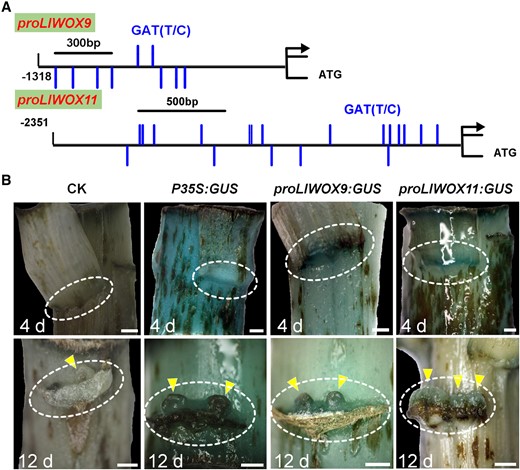
Detection of LlWOX9 and LlWOX11 promoter activity. A, The LlWOX9 and LlWOX11 promoters contain a large number of type-B RR binding elements. B, The expression of GUS was driven by the 35S, LlWOX9, and LlWOX11 promoters, and GUS staining was performed in L. lancifolium stems after cultured 4 and 12 days. The stems were digitally extracted for comparison. The circles indicate leaf axils and triangles indicate bulbils. Scale bar in (B) is 1 mm.
Then, we divided the LlWOX9 and LlWOX11 promoters into three or five segments, respectively, according to the positions of GATT/C elements to construct yeast bait vectors (Figure 7A). Yeast one-hybrid (Y1H) results showed that five type-B LlRRs could strongly bind the promoter sequences of LlWOX9 and LlWOX11. Among these sequences, the proWOX9-I fragment could be bound by LlRR1, LlRR2, LlRR10, and LlRR12 (Figure 7B); the proWOX9-II fragment could be bound by LlRR2, LlRR11 and LlRR12 (Figure 7B); and the proWOX9-III fragment could not be bound by any LlRR because it contained no predicted binding element (Figure 7B). The proWOX11-I fragments could be bound by LlRR1, LlRR10, and LlRR12 (Figure 7C); the proWOX11-II fragments could be bound by LlRR1, LlRR2, and LlRR10 (Figure 7C); the proWOX11-III fragments could be bound by LlRR1, LlRR10, LlRR11, and LlRR12 (Figure 7C); the proWOX11-IV fragments could be bound by LlRR2, LlRR10, and LlRR11 (Figure 7C); and the proWOX11-V fragments could be bound by LlRR1, LlRR2, and LlRR12 (Figure 7C).
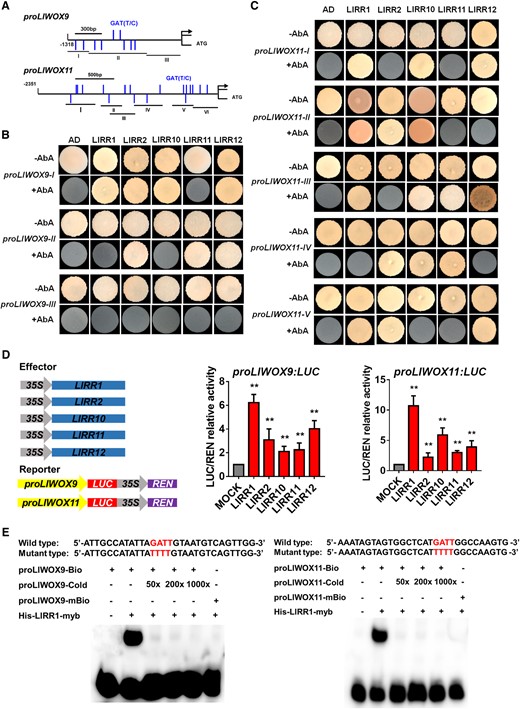
Yeast one-hybrid and dual-luciferase reporter assays and EMSAs of type-B LlRRs with the LlWOX9 and LlWOX11 promoters. A, Division of the LlWOX9 and LlWOX11 promoters into fragments according to the location of type-B RR binding elements (GATT/C). B, Y1H assays between five type-B LlRRs and LlWOX9 promoter fragments. C, Y1H assays between type-B LlRRs and LlWOX11 promoter fragments. D, The transient activation test in N. benthamiana leaves verified the transcriptional activation ability of the five type-B LlRRs toward the LlWOX9 and LlWOX11 promoters. Values are means ± sd (n = 3). Asterisks indicate significantly different values (Student’s t test, **P < 0.01). e, The binding ability of His-LlRR1 protein toward the proLlWOX9-1 and proLlWOX11-2 fragments was verified by EMSAs. The binding element GATT was mutated to TTTT in the mutant probe.
Furthermore, we studied the transcriptional activation ability of five type-B LlRRs toward the LlWOX9 and LlWOX11 promoters in Nicotiana benthamiana leaves. The results showed that compared with the control group, all five type-B LlRRs could significantly activate the transcription of the LlWOX9 and LlWOX11 promoters (Figure 7D). In addition, we selected 30-bp fragments of the proWOX9-I and proWOX11-II fragments containing GATT/C elements to synthesize biotin-labeled probes for electrophoretic mobility shift assays (EMSAs). The results showed that the His-LlRR1 protein could directly bind the proWOX9-I and proWOX11-II fragments (Figure 7E).
LlWOX11 mediates the cytokinin pathway by inhibiting the transcription of LlRR9
LlRR9 is a type-A RR gene whose product is a negative regulator of cytokinin signaling. Our previous studies have revealed that LlRR9 is involved in bulbil formation and transcriptional regulation by LlRR1. In this study, we found a WOX-binding element (TTAATGAG) 2097-bp upstream of ATG in the promoter of LlRR9 (Figure 8A). To determine whether LlRR9 is a downstream gene directly regulated by LlWOX9 or LlWOX11, we carried out dual-luciferase reporter and Y1H assays and EMSAs. Our results showed that LlWOX9 did not affect transcription from the LlRR9 promoter, but LlWOX11significantly inhibited transcription from the LlRR9 promoter (Figure 8B). In addition, we detect the expression of LlRR9 decreased after overexpression of LlWOX11, interestingly, LlRR9 expression also decreased after overexpression LlWOX9 (Figure 8C). The results of Y1H assays showed that LlWOX11 could bind the LlRR9 promoter fragment containing the TTAATGAG element (Figure 8D). Furthermore, a biotin-labeled probe was synthesized by selecting a 30-bp fragment of the LlRR9 promoter containing the TTAATGAG element for EMSA. The EMSA results showed that LlWOX11 could directly bind the LlRR9 promoter sequence (Figure 8E).
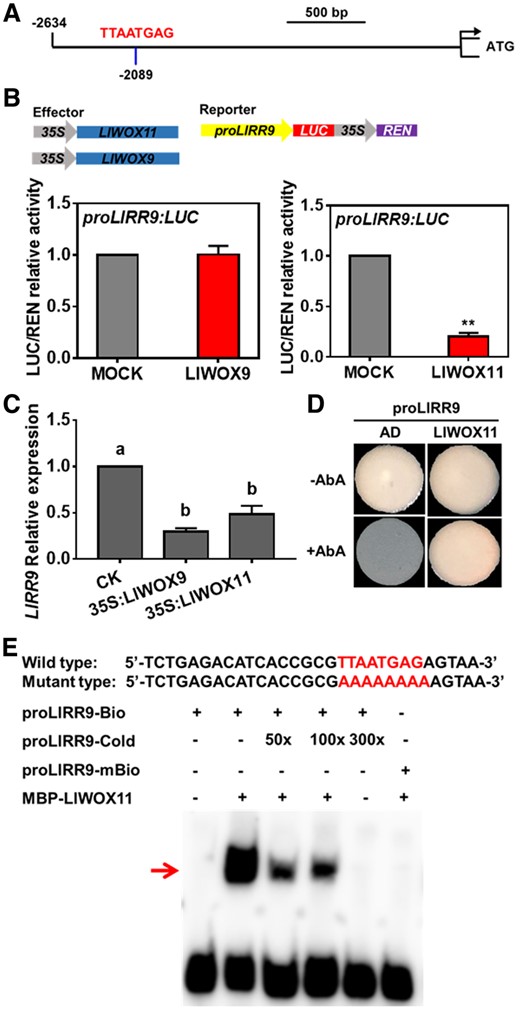
The interaction between LlWOX11 and the LlRR9 promoter was verified by dual-luciferase reporter and Y1H assays and EMSA. A, A WOX-binding element (TTAATGAG) in the promoter of LlRR9. B, The transient activation test in N. benthamiana leaves verified the transcriptional activation ability of LlWOX9 and LlWOX11 toward the LlRR9 promoter. Values are means ± sd (n = 3). Asterisks indicate significantly different values (Student’s t test, **P < 0.01). C, The expression of LlRR9 after overexpression LlWOX9 and LlWOX11. Values are means ± sd (n = 3). Lowercase letters indicate significantly different values (Student’s t test, P < 0.05). D, The binding ability of LlWOX11 toward the LlRR9 promoter was verified by Y1H assay. E, The binding ability of the MBP-LlWOX11 protein toward the proLlRR9 fragment was verified by EMSA. The binding element TTAATGA was mutated to AAAAAAA in the mutant probe.
Discussion
LlWOX9 and LlWOX11 are key regulators during bulbil formation
The development of axillary buds can be divided into the AM initiation and the formation of axillary buds. At present, studies in the AM regulation have revealed WUS-clade members are key regulators. In Arabidopsis, WUS is involved in the AM initiation and maintenance (Wang et al., 2014a, 2014b, 2017), OsWUS is involved in AM initiation, and OsWOX4 in AM maintenance in rice (Tanaka et al., 2015). Some studies have shown WOX9, member of the intermediate-clade in WOX family genes, can affect the formation of axillary buds. In the A. thaliana wox9 mutant, the growth of the axillary buds is substantially inhibited (Skylar et al., 2010; Skylar and Wu, 2010). In O. sativa, the dwt1 mutant shows shorter tillers and a reduced tiller number (Wang et al., 2014c). However, there is a lack of in-depth research on how members of the intermediate-clade participate in the formation of axillary buds. In our study, we identified two intermediate-clade members, LlWOX9 and LlWOX11 are the key regulators during AM initiation, AM maintenance and bulbil development. In our transcriptome data, we did not find WUS, besides, based on the conservation of WUS in different species, we tried to clone WUS through degenerate primers in L. lancifolium, however, the WUS gene has not been amplified. Since the lily genome has not been sequenced, we speculate that WUS may not be involved in the bulbil formation, and LlWOX9 and LlWOX11 may play a more important role.
Members of the intermediate clade are widely expressed in plants and usually play a role in maintaining meristem cell division (Wu et al., 2007; Breuninger et al., 2008; Zhao et al., 2009). In A. thaliana, when WOX9 function is lost, cells divide abnormally, and the development of the shoot meristem is defective (Wu et al., 2005; Skylar et al., 2010). In the Oswox11 mutant, the crown root number and plant height are decreased, and the growth rate and flowering time are delayed, while in OsWOX11-overexpressing lines, the number of crown roots is increased, ectopic crown roots form at the base of the spikelets, and the growth rate increases substantially (Zhao et al., 2009). After the overexpression of LlWOX9 and LlWOX11, we also observed the abnormal proliferation of axillary tissue cells and the development of large purple-black bulbils on leaf axils (Supplemental Figure S5), which indicated that LlWOX9 and LlWOX11 maintain the normal division of the meristem.
Cytokinins induce the transcription of LlWOX9 and LlWOX11 through type-B LlRRs
Many studies have shown that WOX family genes can be induced by plant hormones, such as auxin, cytokinin, and gibberellin (GA) (Gonzali et al., 2005; Leibfried et al., 2005; Weijers et al., 2006; Sarkar et al., 2007; Skylar et al., 2010; Cheng et al., 2014). Some studies have revealed cytokinins could regulate WUS expression through type-B RRs. During AM initiation, type-B RRs can directly bind to the WUS promoter and activate the transcription of WUS (Wang et al., 2014a, 2014b, 2017). Although promoters of WOX family genes contain a large number of cytokinin binding elements, it is still unclear whether cytokinins widely regulate WOX transcription through type-B RRs. In our study, we also found a large number of cytokinin response elements in the LlWOX9 and LlWOX11 promoters, and we demonstrated that the expression of LlWOX9 and LlWOX11 could be induced by cytokinin through type-B LlRRs.
LlWOX11 mediates cytokinin signaling by inhibiting the transcription of LlRR9
Some studies have shown that both WOX9 and WOX11 can mediate cytokinin pathways to regulate plant development (Wu et al., 2005, 2007; Zhao et al., 2009; Wang et al., 2014c; Jiang et al., 2017). In rice crown root formation, OsWOX11 can directly bind and inhibit the transcription of OsRR2 to mediate cytokinin signaling (Zhao et al., 2009). OsRR2 is specifically expressed in the crown root and is a member of the type-A RRs, which are negative regulators of cytokinin signaling. Therefore, after the transcription of OsRR2 is inhibited, cytokinin signaling is enhanced to induce crown root formation (Zhao et al., 2009). The ERF3 protein can bind to the OsWOX11 protein and further enhance the transcriptional inhibition of OsRR2 by OsWOX11 (Zhao et al., 2015). We identified a similar mechanism, as our results showed that LlWOX11 can directly bind the promoter of LlRR9, a type-A LlRR gene, and inhibit its transcription to enhance cytokinin signaling and thus promote bulbil formation.
Previous studies have shown that WOX9 is involved in the cytokinin pathway. In Arabidopsis, WOX9 inhibits cytokinin signaling through type-A RRs (Skylar et al., 2010), while in rice, WOX9 can promote the cytokinin pathway (Wang et al., 2014a, 2014b, 2014c). During A. thaliana seedling development, WOX9 seems to promote the expression of type-A RRs. In the wox9 mutant, ARR5 expression is decreased, which causes shoot meristem development termination (Skylar et al., 2010; Skylar and Wu, 2010). In rice, OsWOX9 plays a negative role in regulating the expression of type-A RRs (Wang et al., 2014c). A study in rice revealed that OsWOX9 modulates the cytokinin pathway to regulate the growth height and flowering time of tillers and main branches (Wang et al., 2014c). In the Oswox9 mutant, tiller elongation is inhibited, and in the shortened internodes, the expression of OsCKX4, OsCKX9, and several type-A OsRR genes (OsRR6, OsRR9, and OsRR10) is increased (Wang et al., 2014c). However, it has not been reported that WOX9 could directly bind to type-A RRs promoter to regulate type-A RRs transcription. In our study, we proved that LlWOX9 could not bind to LlRR9 promoter, but decreased LlRR9 expression was detected after overexpression of LlWOX9, suggesting that LlWOX9 may mediate the cytokinin pathway indirectly.
LlWOX9 may mediate GA signaling
In the Oswox9 mutant, the tillers have shorter internodes with cells that are fewer in number and unelongated relative to those of the wild-type, and OsWOX9 activity in internode elongation is directly or indirectly associated with GA signaling (Wang et al., 2014c). The organ boundary gene ARABIDOPSIS THALIANA HOMEOBOX GENE 1 (ATH1) and the GA signaling DELLA genes maintain the compressed rosette growth habit of Arabidopsis. The loss of ATH1 and DELLA function causes a change from a rosette to caulescent growth habit (Ejaz et al., 2021). The phenotypes of LlWOX9-overexpressing Arabidopsis lines show elongated internodes, and we speculate that LlWOX9 may regulate the internode elongation associated with GA signaling. So we evaluated two GA biosynthetic genes GIBBERELLIN 2-BETA-DIOXYGENASE 1 (LlGA20OX1, c120709_g1) and LlGA3OX2 (c129136_g2), and one GA oxidase gene LlGA2OX2 (c81052_g1) in LlWOX9 transient overexpression leaf axils. We found that after overexpression LlWOX9, the expression of LlGA3OX2 increased significantly, while the expression of LlGA2OX2 decreased significantly (Supplemental Figure S6). Therefore, LlWOX9 may be associated with altered GA homeostasis or signaling.
LlWOX9 and LlWOX11 may be involved in scale development and anthocyanin synthesis
A recent study on the genome of garlic bulb plants showed that two WOX family genes (Asa7G00799.1 and Asa3G03517.1) are involved in bulb development, among which Asa7G00799.1 is expressed specifically in bulbs and positively associated with bulb weight (Sun et al., 2020). Our results showed that LlWOX9 and LlWOX11 were highly expressed in scales and that the expression of LlWOX9 and LlWOX11 reached the highest level at the stage of bulbil scale development (S5), indicating that LlWOX9 and LlWOX11 may be involved in the development of lily bulbs.
Interestingly, we found that the overexpression of LlWOX9 and LlWOX11 not only promoted bulbil formation but also resulted in abnormal purple-black bulbils on the leaf axils (Supplemental Figure S5). Although bulbils may gradually turn purple-black during development under normal circumstances, the overexpression of LlWOX9 and LlWOX11 substantially advanced this change. Therefore, we overexpression LlWOX9 and LlWOX11 in young leaves of L. lancifolium to verify the role of LlWOX9 and LlWOX11 in anthocyanin synthesis. We found that anthocyanins accumulated significantly after overexpression LlWOX11 (Supplemental Figure S7), and the expression levels of DIHYDROFLAVONOL 4-REDUCTASE (LlDFR, c4316912_g1 and c124872_g1) and ANTHOCYANIDIN SYNTHASE (LlANS, c235432_g1) genes increased significantly both in overexpression LlWOX9 and LlWOX11 leaves (Supplemental Figure S7). Therefore, we speculate that LlWOX9 and LlWOX11 may be involved in the synthesis of anthocyanins.
Conclusion
In conclusion, we revealed the molecular mechanism by which WOX genes cooperate with cytokinin signaling to regulate bulbil formation. Type-B LlRRs promote the transcription of LlWOX9 and LlWOX11, and LlWOX11 inhibits the transcription of type-A LlRR9 to enhance cytokinin signaling, thus promoting bulbil formation (Figure 9).
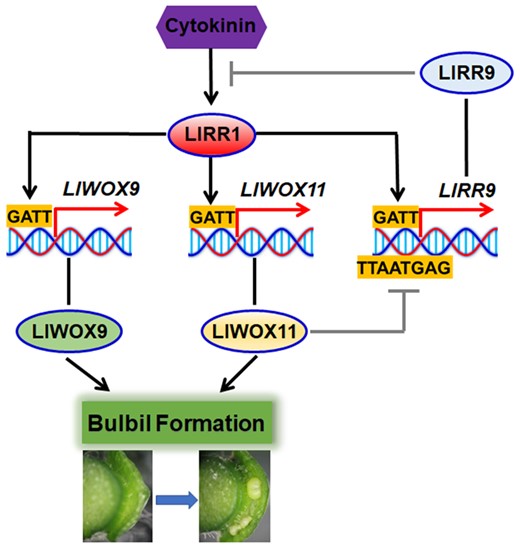
Model of WOX gene cooperation with cytokinin signaling to regulate the bulbil formation.
Materials and methods
Plant materials and treatments
Bulbs of tiger lily (L.lancifolium) of uniform size were harvested and buried in soil at 4°C at the Institute of Vegetables and Flowers, Chinese Academy of Agricultural Sciences, Beijing, China, in November 2019. Well-grown stems with a height of 10 cm were selected according to an in vitro bulbil induction system (He et al., 2020), and stem segments were cultured on Murashige and Skoog (MS) medium for bulbil induction. The stages of bulbil formation were divided into the bulbil initiation stage (S0–S2), bulbil primordium formation stage (S3–S4), and bulbil structure formation stage (S5; He et al., 2020). Different stages of developing bulbils and different tissues (leaf axils at stage S4, shoot apex, leaf, stem, root, scale, stigma, ovary, anther, and petal tissues) were collected for RNA extraction.
To determine whether LlWOX9 and LlWOX11 are immediately induced by cytokinins, 4-mM 6-BA was added to Murashige and Skoog (MS) medium during bulbil formation, and stem segments at the S4 stage were treated with 10-mM 6-BA or with 0.05-mM NaOH as a control. Leaf axils were harvested at the S0–S5 stages and after 0, 0.5, 1.0, 1.5, 2.0, or 2.5 h of treatment.
Isolation of LlWOX9 and LlWOX11 genes and promoters
According to our transcriptome data (accession number: SRP103184), we designed primers by using Primer 6 to clone the full-length sequences and promoters of LlWOX9 and LlWOX11. The full-length sequences of LlWOX9 and LlWOX11 were cloned via RLM-RACE using the GeneRacer Kit (Invitrogen, Carlsbad, CA, USA) according to the kit protocol. To obtain the promoter sequences of LlWOX9 and LlWOX11, three gene-specific reverse primers were designed and a nested PCR program was used according to the protocol of a genome walking kit (Takara, Shiga, Japan). The sequences of the primers used for amplification are shown in Supplemental Table S1. Conserved protein domains were analyzed using SMART (http://smart.embl.de/). Phylogenetic analysis was performed using MEGA6 (http://mega6.software.informer.com/). Multiple sequence alignments were analyzed using the DNAMAN software package. New PLACE (https://www.dna.affrc.go.jp/PLACE/?action=newplace) and PlantCARE (http://bioinformatics.psb.ugent.be/webtools/plantcare/html/) were used to analyze the LlWOX9 and LlWOX11 promoters.
RT-qPCR
Total RNA from the different tissue and leaf axil specimens was extracted with an RNAprep Pure Plant Kit (TIANGEN, Beijing, China) according to the kit protocol, and DNA contamination was removed with RNase-free DNase I. First-strand cDNA was synthesized with a Hifair® 1st Strand cDNA Synthesis Kit (gDNA digester plus) (Yeasen, China) according to the kit protocol. Gene-specific primers for RT-qPCR were designed with Primer version 6.0 (Supplemental Table S2). The LilyActin primer was used as an internal control (Xu et al., 2017), and SYBR Green Master Mix (No Rox) (Yeasen, Shanghai, China) was used in the reaction mixture according to the manufacturer’s instructions. RT-qPCR was conducted using the CFX96 Real-Time System (Bio-Rad, Hercules, CA, USA), with an initial denaturation step at 95°C for 3 min, followed by 40 cycles of denaturation at 95°C for 10 s, annealing at 60°C for 20 s, and extension at 72°C for 1 min. The 2−ΔΔCt method was used to calculate the relative expression levels of the different genes (Livak and Schmittgen, 2001). Three biological and three technical replicates were performed to reduce error.
Subcellular localization
The full-length cDNAs of LlWOX9 and LlWOX11 under the control of the 35S cauliflower mosaic virus promoter were cloned into the pCAMBIA 2300 vector using the pEASY-Basic Seamless Cloning and Assembly Kit (Transgen Biotech, Beijing, China). The sequences of the primer pairs used for amplification are shown in Supplemental Table S3. The resulting plasmids were transferred into Agrobacterium tumefaciens strain EHA105. Agrobacterium cells were collected and suspended in infiltration buffer (10 mM methylester sulfonate, 10 mM MgCl2, and 150 μM acetosyringone, pH 5.7) at optical density (OD600) = 1.0 and infiltrated into L. lancifolium leaves. About 3 days after infiltration, the leaves were harvested and treated with 150 μg/mL 4ʹ,6-diamidino-2-phenylindole (DAPI; Sigma). A Zeiss LSM 510 confocal scanning microscope was used to collect images. GFP and DAPI fluorescence was observed at 488 and 340 nm excitation, respectively.
RNA FISH
Leaf axils in S1–S5 were fixed with FAA, and after dehydration, clearing, and embedding, paraffin sections of the leaf axils were sliced at a thickness of 0.8 μm. The obtained slides were rehydrated with xylene, digested with protease K (20 μg/mL) at 37°C, blocked with a 3% (w/v) methanol–H2O2 solution for 25 min, with avidin (0.07%, w/v) at 37°C for 25 min and with biotin (0.005%, w/v) at 37°C for 15 min. Hybridization with the probes was performed at 37°C overnight in a moist chamber. After hybridization, the slides were washed in 2× SSC for 10 min, 3 times in 1× SSC for 5 min, and once in 0.5× SSC for 10 min at 37°C. The avidin-labeled probe was detected with a streptavidin Alexa Fluor 405 conjugate (1:250; Invitrogen). Antibodies were diluted in PBS containing 3% (w/v) BSA, and the slides were incubated with the antibodies for 30 min at 37°C. After antibody incubation, the slides were washed 3 times with 4× SSC containing 0.1% Tween-20 (v/v), stained with 100-ng/mL DAPI in PBS for 30 min, and dehydrated with ethanol. Confocal images were obtained using a Zeiss LSM 510 confocal scanning microscope. GFP and 4',6-diamidino-2-phenylindole (DAPI) fluorescence was observed at 488 nm and 340-nm excitation, respectively.
Transformation of A. thaliana and L. lancifolium
The full-length cDNAs of LlWOX9 and LlWOX11 were amplified by PCR and inserted into the pCAMBIA 3301 vector using the pEASY-Basic Seamless Cloning and Assembly Kit (Transgen Biotech, China). All primers used are listed in Supplemental Table S1. Arabidopsisthaliana was transformed using A. tumefaciens strain GV3101 and the floral dip method (Clough and Bent, 1998). Transgenic A. thaliana plants were selected on 1/2 MS medium with 30 mg/L kanamycin. Transgenic A. thaliana plants were grown in climate-controlled boxes at 24°C under a 12/12-h light/dark cycle. The transgenic lines were identified using the 35S-F and LlWOX9-R or LlWOX11-R primers.
Liliumlancifolium was transformed using A. tumefaciens strain EHA105 via Agrobacterium-mediated vacuum infiltration. Agrobacterium cells were collected and suspended in infiltration buffer that contained 10 mM MgCl2, 200-μM acetosyringone, and 10-mM MES (pH 5.6). The small stem segments of L. lancifolium were submerged in infiltration solution and then subjected to −50-kPa vacuum for 10 min. The infiltrated segments were washed with distilled water 3 times and then were grown on MS medium with 30-g L−1 sucrose and 6-g L−1 agar (pH 5.8) in the dark at 20°C for 1 day, followed by growth at 22°C under a 16-/8-h light/dark cycle. The rate of bulbil formation was assessed after 1 week of culture, and RNA was extracted from leaf axils to measure the expression of the target genes. Each treatment consisted of three experimental replicates, with 30 leaf axils per replicate.
VIGS
For the generation of pTRV2-LlWOX9 and pTRV2-LlWOX11, gene-specific fragments of ∼300 bp were cloned into the pTRV2 vector using the pEASY-Basic Seamless Cloning and Assembly Kit (Transgen Biotech, China). Five pTRV2-LlRR vectors were constructed as previously described (He et al., 2021). The primer pairs used to generate the TRV vectors are shown in Supplemental Table S3. Virus-induced gene silencing (VIGS) was performed using A. tumefaciens strain EHA105 and vacuum infiltration method (He et al., 2021). The rate of bulbil formation was assessed after 2 weeks of culture, and RNA was extracted from leaf axils to measure the expression of the target genes. Each treatment consisted of three experimental replicates, with 30 leaf axils per replicate.
GUS staining
A 1,318-bp fragment upstream of the start codon of LlWOX9 and a 2,351-bp fragment upstream of the start codon of LlWOX11 were introduced into the pCAMBIA 3301 vector, and the 35S promoter was replaced using the pEASY-Basic Seamless Cloning and Assembly Kit (Transgen Biotech, China). The constructed plasmids were transferred into A. tumefaciens strain EHA105. Stem segments at S0 and S5 were used for vacuum infiltration according to the method described above. Three days after infiltration, the leaves and stem segments were harvested and treated with GUS staining solution (Solarbio, China) according to the kit protocol. After staining, the leaves and stem segments were washed and cleared with 70% ethanol for more than 24 h before image capture using a Leica Microsystems DM5500B instrument (Wetzlar, Germany).
Y1H assay
Y1H analysis was performed according to the method described by Lin et al. (2013). Briefly, the full-length coding regions of five LlRRs and LlWOX11 were cloned into the pGADT7 vector to generate the pGADT7-LlRRs and pGADT7-LlWOX11 constructs. Various truncated versions of the promoter regions of LlWOX9 and LlWOX11 were amplified and ligated into the pABAi reporter vector. The constructs were then cotransformed into the yeast strain EGY48. Transformants were grown on SD-Trp/-Ura plates for 3 days at 28°C. The interactions were determined based on the growth ability of the cotransformants on medium supplemented with aureobasidin A.
Dual-luciferase reporter assay
The coding sequence of LlWOX11 was cloned into the pCAMBIA 3301 vector using the pEASY-Basic Seamless Cloning and Assembly Kit (Transgen Biotech, China). Five pCAMBIA 3301-LlRR vectors were constructed as previously described (He et al., 2021). A 1,318-bp fragment upstream of the start codon of LlWOX9 and a 2,351-bp fragment upstream of the start codon of LlWOX11 were introduced into the pluc-35Rluc vector using the pEASY-Basic Seamless Cloning and Assembly Kit (Transgen Biotech, China). The primers used to generate the constructs are listed in Supplemental Table S3. The constructed plasmids were transformed into A. tumefaciens strain GV3101. Different effectors were subsequently coinfiltrated with the reporter into N.benthamiana leaves using a syringe. At 3 days after infiltration, 2-cm-diameter leaf discs were harvested and ground in liquid nitrogen. The activities of firefly and Renilla luciferase were measured with a Dual-Luciferase Reporter Assay System (Promega, Madison, WI, USA) using a GloMax 20/20 luminometer (Promega).
EMSAs
To construct plasmids for the expression of the recombinant LlWOX11 protein in Escherichia coli, the full-length cDNA was amplified and cloned into the pMal-c2X vector, which was expressed in the E.coli strain BL21 (DE3) cell line. The pET32a-LlRR1 vector was constructed as previously described (He et al., 2021). The primers are listed in Supplemental Table S3. Protein expression was induced by incubation in 1-mM IPTG at 16°C at 160 rpm for 24 h. Protein purification was carried out using an amylose resin purification system (NEB, Ipswich, MA, USA) following the manufacturer’s instructions. Double-stranded oligonucleotide probes were synthesized and labeled with biotin at the 5′-end. EMSA was carried out using the LightShift Chemiluminescent EMSA Kit (Thermo Fisher Scientific, Waltham, MA, USA). Competition experiments were performed with different amounts of nonlabeled oligonucleotides. The mutated competitors were generated by replacing eight base pairs in the WOX binding elements (TTAATGAG to AAAAAAAA).
Accession numbers
RNA-seq raw reads from this article can be found in the NCBI SRA data under accession number SRP103184. Gene accession numbers used in this study: LlRR1 (MW509629), LlRR2 (MW509630), LlRR10 (MW509631), LlRR11 (MW509632), LlRR12 (MW509633), and LlRR9 (MW509634).
Supplemental data
The following materials are available in the online version of this article.
Supplemental Figure S1. Heat map of WOX genes expression at different bulbil formation stages.
Supplemental Figure S2. Sequence alignment of LlWOX9 and LlWOX11.
Supplemental Figure S3. Phylogenetic tree of LlWOX9 and LlWOX11.
Supplemental Figure S4. FISH of LlWOX9 and LlWOX11 with sense probe.
Supplemental Figure S5. The phenotype of abnormal proliferation in leaf axil after overexpression of LlWOX9 and LlWOX11.
Supplemental Figure S6. Expression of LlGA20OX1, LlGA3OX2, and LlGA2OX2 after overexpression LlWOX9.
Supplemental Figure S7. The L. lancifolium leaves phenotype, anthocyanin content, and LlDFRs and LlANS expression after overexpression of LlWOX9 and LlWOX11.
Supplemental Table S1. Primers used in full-length and promoter sequence cloning of LlWOX9 and LlWOX11.
Supplemental Table S2. Primers used in RT-qPCR.
Supplemental Table S3. Primers used in vector construction.
P.P.Y. and J.M. designed the research. G.R.H., Y.W.C., J.W., M.S., M.M.B., Y.C.T., and L.F.X. conducted the experiments. G.R.H. analyzed the data and wrote the manuscript. All authors read and approved the manuscript.
The author responsible for distribution of materials integral to the findings presented in this article in accordance with the policy described in the Instructions for Authors (https://dbpia.nl.go.kr/plphys/pages/general-instructions) are Panpan Yang ([email protected]) and Jun Ming ([email protected]).
Acknowledgments
We acknowledge Xia Cui (Chinese Academy of Agricultural Sciences, China) for the pluc-35Rluc vector and technical assistance.
Funding
This work was supported by National Natural Science Foundation of China (31902043, 32172612), National key R & D program of China (2019YFD1001002), Science and technology projects of Guizhou Province (20201Y121), and the Central Public-interest Scientific Institution Basal Research Fund (IVF-BRF2021017).
Conflict of interest statement. None declared.
References
Author notes
Senior authors