-
PDF
- Split View
-
Views
-
Cite
Cite
Charles Roussin-Léveillée, Guilherme Silva-Martins, Peter Moffett, ARGONAUTE5 Represses Age-Dependent Induction of Flowering through Physical and Functional Interaction with miR156 in Arabidopsis, Plant and Cell Physiology, Volume 61, Issue 5, May 2020, Pages 957–966, https://doi.org/10.1093/pcp/pcaa022
- Share Icon Share
Abstract
Flowering time is a finely tuned process in plants, in part controlled by the age-regulated microRNA156 (miR156), which functions by suppressing the transcripts of SQUAMOSA-PROMOTER BINDING LIKE (SPL) transcription factors. ARGONAUTE (AGO) proteins are essential effectors of miRNA-mediated gene regulation. However, which AGO(s) mediate(s) the control of flowering time remains unclear. Here, we demonstrate a role of AGO5 in controlling flowering time by modulating the expression of SPL transcription factors. We show that AGO5 interacts physically and functionally with miR156 and that ago5 mutants present an early flowering phenotype in Arabidopsis. Furthermore, in ago5 mutants, the repression of flowering caused by miR156 overexpression is largely reversed, whereas leaf morphology remains unaffected. Our results thus indicate a specific role for AGO5 in mediating miR156 activity in meristematic, but not vegetative, tissue. As such, our data suggest a spatiotemporal regulation of the miR156 aging pathway mediated through different AGO proteins in different tissues.
Introduction
In plants, flowering time is tightly regulated by multiple genetic pathways, some of which are controlled by the expression of small non-coding RNAs (Wu et al. 2009, Teotia and Tang 2015, Fouracre and Poethig 2016). These include microRNAs, which are processed into 20–24 nucleotide small RNAs from endogenously encoded microRNA precursors (pri-miRNA) by DICER-LIKE (DCL) proteins and are subsequently bound by ARGONAUTE (AGO) proteins (Baulcombe 2004, Borges and Martienssen 2015, Weiberg and Jin 2015). The latter proteins are guided through sequence complementarity to cleave or repress the translation of specific mRNAs (Jones-Rhoades et al. 2006, Zhang et al. 2015, Fang and Qi 2016). Among the miRNAs regulating developmental phase transition, microRNA156 (miR156) regulates key developmental transition genes by repressing the translation of SQUAMOSA PROMOTER BINDING PROTEIN (SBP) transcription factors (Poethig 2013, Xu et al. 2016, He et al. 2018). Arabidopsis thaliana (Arabidopsis hereafter) encodes 16 SBP-LIKE (SPL) genes, of which 10 are post-transcriptionally regulated by miR156 (Rhoades et al. 2002, Schwab et al. 2005, Wu and Poethig 2006, Gandikota et al. 2007). Studies of shoot apex development suggest that SBP transcription factors regulate subsets of genes, such as APETALA1 (AP1), LEAFY (LFY), SUPPRESSOR OF CONSTANS1 (SOC1), FRUITFULL (FUL) and AGAMOUS-LIKE42 (AGL42) (Wang et al. 2009, Xu et al. 2016). High levels of miR156 expression can be observed early in development, gradually decreasing in expression over time, allowing the translation of multiple transition-promoting proteins (Wu and Poethig 2006, Wu et al. 2009, Wang 2014).
An increase in the levels of SPL transcript abundance due to declining miR156 level drives vegetative development and flowering in many plant species (Fornara and Coupland 2009, Hibara et al. 2016, Wei et al. 2017). Previous studies have shown that miR156 targets the mRNAs encoding SPL3, SPL4 and SPL5, which are involved in floral meristem identity, as well as those encoding SPL2, SPL9, SPL10, SPL11 and SPL15, which control shoot maturation and floral induction (Schwarz et al. 2008, Wang et al. 2009, Xu et al. 2016). Consistent with this, overexpression of miR156 dramatically delays juvenile-to-adult and reproductive phase transition, including flowering, through the cleavage of mRNA and translational repression of SPL proteins (Wu and Poethig 2006, Chuck et al. 2007).
Although multiple reports have investigated the effects of miR156 on SPL-encoding mRNAs, less is known about which AGO proteins functionally interact with miR156 in different developmental processes. The Arabidopsis genome encodes 10 AGO proteins, which function in multiple regulatory mechanisms, determined at least in part by their expression patterns and by the nature of the small RNAs they bind (Vaucheret 2008).
In Arabidopsis, the AGO1 and AGO10 proteins have been extensively characterized for their roles in plant development (Vaucheret et al. 2004, Zhu et al. 2011). AGO1 is mainly known for its importance in mediating mRNA cleavage through its interaction with miRNAs, as well as in generating trans-acting small-interfering RNA, which together regulate a plethora of physiological and developmental phenomena (Vaucheret et al. 2004, Baumberger and Baulcombe 2005, Fei et al. 2013, Rogers and Chen 2013). Given the large number of genes regulated by AGO1, null mutants are lethal and many of the developmental phenotypes caused by hypomorphic alleles may be pleiotropic in nature. AGO10 has also been identified as a key player in meristem development through its antagonism with AGO1 by sequestering miR165/166, which negatively regulates the HD-ZIP III gene family (Zhu et al. 2011). With respect to shoot development, AGO1 has been shown to bind miR156 in seedlings (Ronemus et al. 2006, Azevedo et al. 2010) and regulates SPL2/9/11 during abiotic stress (Stief et al. 2014). However, the identity of the AGO protein(s) that mediate(s) the effects of miR156 in flowering time has not been specifically addressed.
Here, we show that ago5 mutant plants display an early bolting phenotype, suggesting a role for AGO5 in flowering time. This conclusion was supported by the observation that SBP/SPL gene expression occurred earlier in ago5 plants. Accordingly, we found that AGO5 binds to and stabilizes miR156. Furthermore, we show that the late-flowering phenotype caused by constitutive mir156 overexpression is largely abrogated in ago5 mutants, as are the effects of SPL and other developmental genes.
Results
Arabidopsis ago5 mutants display an early flowering phenotype
The AGO5 protein is involved in antiviral defense (Brosseau and Moffett 2015). In the course of studying this phenomenon, we first observed (regardless of virus infection) a marked difference in flowering time in two independent Arabidopsis ago5 mutant lines. Under a long day (LD) photoperiod, we found that ago5 mutants displayed an early flowering time compared to wild-type (WT) Columbia-0 (Col-0) (Fig. 1A). To quantify this phenomenon, we assessed the difference in bolting time between Col-0 and two ago5 mutant lines, ago5-1 and ago5-5. Bolting started on average at 23 d post-germination (dpg) in ago5 mutants, while Col-0 bolted approximately 5 d later, at 28 dpg (Fig. 1B). Similar results were observed for days-to-bolting in a 12-h/12-h light/dark photoperiod (Supplementary Fig. S1D).
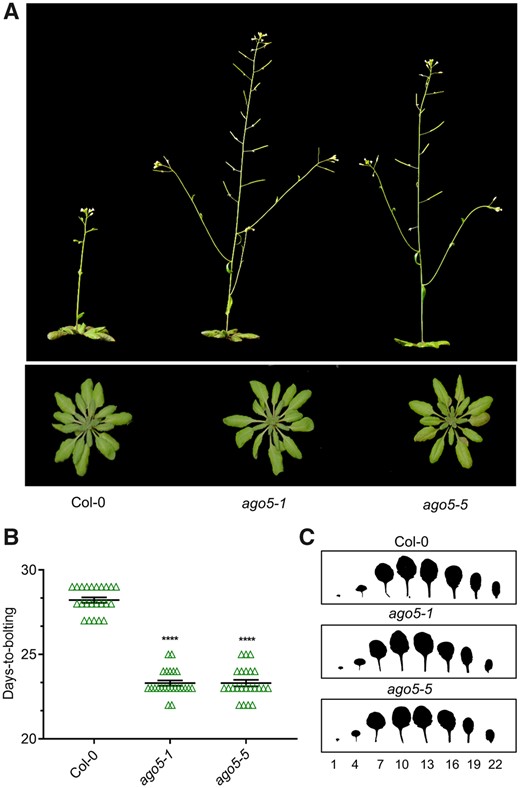
Arabidopsis ago5 mutant plants display an early flowering time phenotype. (A) Phenotypes of Col-0 and ago5 T-DNA insertion mutants (ago5-1 and ago5-5). (B) Days-to-bolting of the aforementioned genotypes was evaluated when bolts were 1 cm tall (n = 25). (C) Representative scans of fully expanded 20-day-old rosettes. Numbers represent leaves starting from the base of the rosette. Asterisks indicate significant differences from Col-0 in a Student’s t-test (*P < 0.05, **P < 0.01, ***P < 0.001, ****P < 0.0001). Error bars indicate standard deviation.
The earlier flowering time in ago5 mutants did not alter floral architecture or number of seeds per silique, compared to Col-0 (Supplementary Fig. S2). However, ago5 mutants produced fewer flowers [138.6 ± 2.95, 138.1 ± 2.87 (mean ± SEM) for ago5-1 and ago5-5, respectively] compared to WT [184.9 ± 2.26 (mean ± SEM)] (Supplementary Fig. S1). This difference was not due to the final height reached by the different genotypes since both ago5 mutants and Col-0 ultimately grew to approximately the same height (data not shown). In plants, flowering is associated with juvenile-to-adult phase transition, which also involves leaf development (Xu et al. 2016). However, we found no striking difference in leaf shape between Col-0 and ago5 mutants (Fig. 1C). Furthermore, a number of mature, juvenile and cauline leaves were unchanged between Col-0 and ago5 mutants in a 12-h light/dark photoperiod (Supplementary Fig. S1A–C). Thus, the early bolting phenotype of ago5 mutants is likely due to important changes in the shoot apical meristem (SAM) rather than a more global effect on plant maturation.
AGO5 is highly expressed in the SAM
To further study the role of AGO5 in the regulation of flowering time, we investigated the levels of AGO5 expression in different tissues. AGO5 expression was assessed in 20 dpg plants under an LD photoperiod in both leaves and the SAM. A striking difference was found between these tissues in that AGO5 was expressed 60-fold higher in SAM tissue compared to leaves (Fig. 2A). Since the control of flowering time is a finely regulated phenomenon, we also assessed AGO5 expression in the same tissues of 14 dpg plants. As expected, in 14 dpg plants, AGO5 was expressed 24-fold higher in SAM tissues, compared to leaves (Fig. 2A). Interestingly, AGO5 was 10-fold more expressed in the SAM of 20 dpg plants as compared to 14 dpg plants (Fig. 2A), suggesting a developmentally regulated progression in AGO5 expression levels.
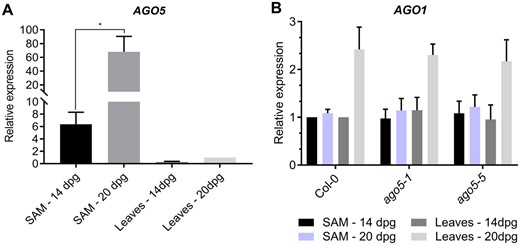
AGO5 is highly expressed in the SAM. (A) RT-qPCR analysis of AGO5 gene expression in leaves and the SAM of Col-0. (B) RT-qPCR analysis of AGO1 gene expression in leaves and the SAM of Col-0 and ago5 mutants. Values are mean ± SD of three biological replicates and are given as fold change compared to Col-0. Asterisks indicate significant differences from Col-0 in a Student’s t-test (*P < 0.05). Error bars indicate standard deviation.
AGO1 is an important player in developmental phase transition in plants and is presumed to have a similar miRNA binding capacity to AGO5, based on their miRNA sorting profiles (Mi et al. 2008). Potential crosstalk between the two AGO proteins is also supported by the expression profile of AGO1 and AGO5 during meristem development (Figs. 1, 2). Thus, we investigated AGO1 expression in ago5 mutants to confirm that AGO5 does not alter AGO1 expression. Expression of AGO1 was unaltered in both the SAM and the leaves of ago5 mutants, as compared to WT plants (Fig. 2B). Therefore, the early flowering phenotype of ago5 mutants is unlikely to be caused by altered expression of AGO1.
Altered expression of SPL transcription factors in ago5 mutants
SPL gene transcripts are tightly regulated by the miR156/157 aging pathway. Both SPLs and miR156 are expressed in the SAM and in leaves, where their abundances are negatively correlated (Schmid et al. 2003, Xu et al. 2016). Based on this information, we hypothesized that the role of AGO5 in SAM maturation and flowering is likely due to miR156-dependent targeting of SPL transcripts. To investigate this, we collected the SAMs of 20 dpg plants and quantified the relative abundance of six SPL gene transcripts by reverse transcriptase quantitative PCR (RT-qPCR) in ago5-1 and ago5-5 mutants, as well as WT plants. Transcripts of SPL genes involved in meristem identity (SPL3, 4, 5), as well as shoot maturation and floral induction (SPL9, 11, 15), were significantly more abundant in the SAMs of ago5 mutants, as compared to Col-0 (Fig. 3A, B). We also investigated the expression of the same genes at an earlier stage of development, when miR156 levels are higher. Significant differences in SPL expression levels were not apparent in ago5 mutants relative to WT in the SAM of 14 dpg plants (Supplementary Fig. S4A, B). We also measured the expression of SPL-targeted genes (AP1, LFY, FUL and SOC1) in the same samples. LFY, FUL and SOC1 showed no significant difference between WT plants and ago5-5 mutant, while AP1 was 2.5-fold more expressed in the ago5-5 mutant (Supplementary Fig. S4C). This suggests that the differences in expression levels of SPL and SPL-targeted genes are not constitutive and that AGO5 is likely important for the fine-tuning of developmentally regulated genes in flowering. However, it is possible that AGO5 could act in additional pathways controlling flowering time since AP1 showed a slight increase in expression in ago5-5 mutant at 14 dpg SAM.
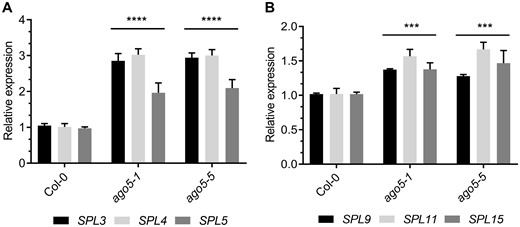
Altered expression of SPL transcription factors in ago5 mutants. (A) RT-qPCR analysis of SPL3/4/5 gene expression in the SAM of 20-day-old Col-0, ago5-1, ago5-5 plants. (B) RT-qPCR analysis of SPL9/11/15 gene expression in the SAM of 20-day-old Col-0, ago5-1, ago5-5 plants. Values are mean ± SD of three biological replicates and are given as fold change compared to Col-0. Asterisks indicate significant differences from Col-0 in a Student’s t-test (*P < 0.05, **P < 0.01, ***P < 0.001, ****P < 0.0001). Error bars indicate standard deviation.
Levels of pri-miR156 are reduced near the onset of reproductive phase change to allow the translation of developmentally regulated proteins (Xu et al. 2016). We thus explored whether pri-miR156 levels were different in 20 dpg Arabidopsis Col-0 and ago5 mutants. Interestingly, we observed that levels of pri-miR156 were approximately 35% lower in ago5 mutants relative to Col-0 (Supplementary Fig. S5). This result would be consistent with a scenario whereby meristem identity in ago5 mutants shifts more rapidly from a vegetative to reproductive phase.
AGO5 physically interacts with miR156 in planta
In Arabidopsis, miRNAs with a 5′ terminal uridine, such as miR156, are preferentially loaded onto AGO1 (Mi et al. 2008). To investigate whether AGO5 and miR156 interact physically, we immunoprecipitated (IP) AGO5 from total extracts from 1-week-old seedlings. Subsequent stem-loop reverse transcription PCR amplification with miRNA156-specific primers indicated the presence of miR156 in immunoprecipitates from Col-0 and miR156 overexpressing plants but not the ago5-5 mutant (Fig. 4A, B).
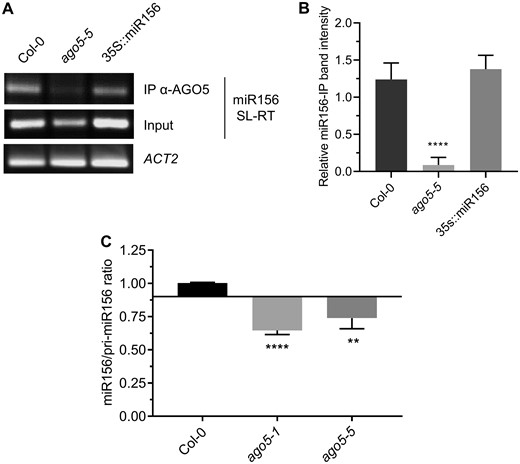
AGO5 binds to, and stabilizes, miR156. (A) RNA extracted from AGO5 immunoprecipitates from 1-week-old seedlings was subjected to SL-RT and PCR using miR156-specific primers. Primers specific to ACTIN2 (ACT2) were used as a control in amplification from total input RNA. (B) PCR band intensities were measured using ImageJ software. Band intensities from miR156 IP samples were normalized to miR156 input bands, and the resulting values were normalized to ACT2 bands amplified from the input. (C) Ratios of miR156 to pri-miR156, based on Cq values obtained by RT-qPCR performed on RNA extracted from the SAMs of 20-day-old Col-0 and ago5 mutant plants, as indicated. Values are mean ± SD of three biological replicates and are given as fold change compared to Col-0. Asterisks indicate significant differences from Col-0 in a Student’s t-test (*P < 0.05, **P < 0.01, ***P < 0.001, ****P < 0.0001). Error bars indicate standard deviation.
Many miRNAs are known to be more stable when they are bound by AGO proteins (Vaucheret et al. 2004). We thus quantified and compared the ratios of miR156 to pri-miR156 transcript levels in Col-0, ago5-1 and ago5-5 by qRT-PCR. We found a decrease in the ratio of miR156 to pri-miR156 in both ago5 mutant lines compared to WT (Fig. 4C). These results suggest that miR156 is less stable in ago5 mutants compared to Col-0, suggesting that AGO5 may be the major binding partner for miR156.
AGO5 is required for the delay in flowering induced by miR156 overexpression
In WT Arabidopsis plants, constitutive overexpression of miR156 from the CaMV 35S promoter leads to a delay in juvenile-to-adult and flowering time due to its negative regulation of SPL transcripts (Wu et al. 2009). To explore the role of AGO5 in the miR156-dependent regulation of SPL genes, we introduced a previously described 35S::miR156 transgene (Wu et al. 2009) into an ago5-5 mutant background. These 35S::miR156/ago5-5 plants displayed morphological characteristics similar to those of the 35S::miR156 alone, including altered leaf shape and stem length (Fig. 5A). However, 35S::miR156/ago5-5 plants bolted earlier than the 35S::miR156 under LD photoperiod, with flowering times not significantly different from Col-0 (Fig. 5D). Thus, the effect of miR156 overexpression on bolting time is abrogated in the absence of AGO5. However, 35S::miR156/ago5-5 plants did not bolt as early as ago5-5 mutants, possibly because some miR156 is able to load onto other AGO when artificially overexpressed.
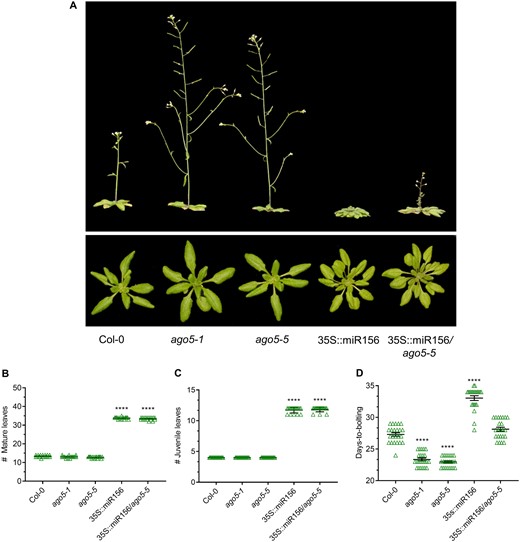
AGO5–miR156 interaction controls flowering time. Phenotypes of Col-0, ago5 T-DNA insertion mutants (ago5-1 and ago5-5), 35S::miR156 and 35S::miR156/ago5-5 plants. (A) Whole plant phenotypes. Photos were taken at 30 dpg. (B) Number of mature leaves (n = 23 plants). (C) Number of juvenile leaves (n = 23 plants). (D) Days-to-bolting of the aforementioned genotypes was evaluated when bolts were 1 cm tall (n = 23 plants). Experiments were repeated at least three times, and similar results were obtained. Asterisks indicate significant differences from Col-0 in a Student’s t-test (*P < 0.05, **P < 0.01, ***P < 0.001, ****P < 0.0001). Error bars indicate standard deviation.
We characterized additional morphological aspects of the juvenile-to-adult phase transition in the 35S::miR156/ago5-5 plants. Despite the marked difference in flowering times, no difference was observed between the 35S::miR156 and the 35S::miR156/ago5-5 lines with respect to either aerial architecture (Fig. 5A) or mature and juvenile leaf counts, as defined by the presence of abaxial trichomes (Fig. 5B, C), which are present in mature, but not juvenile, leafs (Xu et al. 2016). These observations are consistent with the fact that AGO5 expression in the leaves is virtually undetectable (Supplementary Fig. S6). Thus, miR156 may act through AGO5 in meristems, but in leaves its effects may be mediated through other AGO proteins, such as AGO1, which has previously been shown to modulate juvenile-to-adult phase transition in leaves.
AGO5 is essential for a conventional regulation of the miR156 aging pathway in the SAM
To support a functional relationship at the molecular level between AGO5 and mir156 in regulating the flowering time, we compared the expression levels of SPL genes and developmentally regulated genes (AP1, SOC1, FUL and LFY) at 20 dpg in Col-0, ago5-1, ago5-5, 35S::miR156 and 35S::miR156/ago5-5. As expected, ago5 mutants showed higher levels of expression of all SPL genes (Fig. 6A), as well as SPL-regulated genes (Fig. 7A), compared to all other genotypes. In contrast, both 35S::miR156/ago5-5 and 35S::miR156 plants, which have not bolted at this time point, showed dramatically reduced expression levels of the same genes compared to Col-0 at 20 dpg (Figs. 6A, 7A).
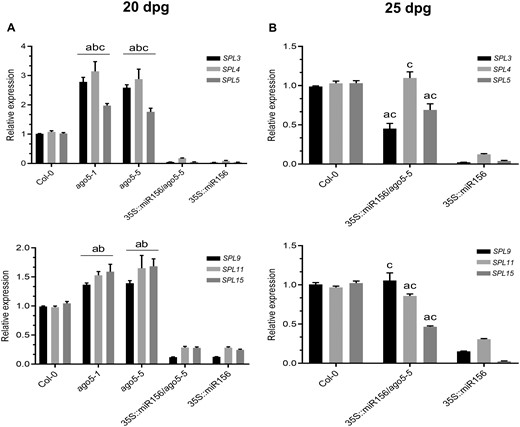
AGO5 regulates the fine-tuning of SPL transcription factor expression. (A) RT-qPCR analysis of SPL gene expression in the SAM of 20 dpg Col-0, ago5-1, ago5-5, 35S::miR156 and 35S::miR156/ago5-5 plants. (B) RT-qPCR analysis of SPL gene expression in the SAM of 25 dpg Col-0, 35S::miR156 and 35S::miR156/ago5-5 plants. Values are mean ± SD of three biological replicates and are given as fold change compared to Col-0. a: significantly different from Col-0; b: significantly different from 35S::miR156/ago5-5; and c: significantly different from 35S::miR156 (Student’s t-test; P < 0.01). Error bars indicate standard deviation.

Developmentally regulated gene expression in the 35S::miR156/ago5-5 hybrid. (A) RT-qPCR analysis at 20 dpg of AP1, LFY, FUL and SOC1 gene expression in the SAMs of Col-0, ago5-1, ago5-5, 35S::miR156 and 35S::miR156/ago5-5 plants. (B) RT-qPCR analysis at 25 dpg of AP1, LFY, FUL and SOC1 gene expression in the SAMs of Col-0, 35S::miR156 and 35S::miR156/ago5-5 plants. Values are mean ± SD of three biological replicates and are given as fold change compared to Col-0. a: significantly different from Col-0; b: significantly different from 35S::miR156/ago5-5; and c: significantly different from 35S::miR156 (Student’s t-test; P < 0.01). Error bars indicate standard deviation.
Since 35S::miR156/ago5-5 plants bolt at the same time as Col-0 plants, we evaluated the expression of SPL genes near the Col-0 bolting time (ago5 mutants were not included as these plants have already bolted at this time point). As expected, we found that expression levels of all of SPL genes analyzed in 35S::miR156/ago5-5, but not 35S::miR156 plants, were close, or equal to WT at 25 dpg (Fig. 6B). Expression levels of developmentally regulated genes were also reestablished in 35S::miR156/ago5-5 compared to 35S::miR156 plants (Fig. 7B). As such, these results further indicate a role for AGO5 in the fine-tuning of gene regulation during flowering.
Discussion
Aging pathway studies have shown important roles for microRNAs in floral induction, in particular miR156. The latter is known to regulate this pathway by targeting SPL transcription factors, which are in turn responsible for the transcription of genes involved in the appearance of adult and reproductive traits (Wang 2014). To affect gene expression, miRNAs must act through AGO proteins and our data suggest that AGO5 plays an important role in the miR156 aging pathway in meristematic tissues.
We observed an early flowering phenotype in ago5 mutant plants, supported by precocious SPL expression. Furthermore, we found that AGO5 was able to bind to miR156 in seedlings and this miRNA was less stable in the SAM of ago5 mutants compared to WT plants. In agreement with this, interactions between AGO5 and miR156 in inflorescent tissues of Arabidopsis have previously been reported in large-scale AGO–miRNA interaction studies (Mi et al. 2008, Takeda et al. 2008, Shao et al. 2014). In support of a major role of AGO5 in flowering, we found that the delay in bolting time was abolished in miR156 overexpressing plants lacking AGO5. Thus, this suggests that the late-flowering phenotype of miR156-overexpressing plants is due mainly to its interaction with AGO5. However, we do not rule out possible compensation by another AGO protein in the presence of artificial overexpression of miR156 in these lines. Likewise, it is possible that the actions of AGO5 are mediated through additional miRNAs. For example, AGO5 has been reported to bind to miR172 (Mi et al. 2008, Takeda et al. 2008, Shao et al. 2014), which functions downstream of miR156 in the age-dependent flowering pathway (Jung et al. 2011, Spanudakis and Jackson 2014, Xu et al. 2016). In addition, although SPL target genes LFY, FUL and SOC1 were not upregulated in ago5-5 mutants before the marked upregulation of SPL genes at 14 dpg, we observed a slight upregulation of AP1 at this time (Supplementary Fig. S4C). This may indicate an alternative pathway involving AGO5.
AGO5 appears to be involved in several plant development processes including seed pigmentation in Glycine max (Cho et al. 2017), sporogenesis in Oryza sativa (Nonomura et al. 2007), establishment of nodules in legume-rhizobia interactions (Reyero-Saavedra et al. 2017) as well as mega-gametogenesis (Tucker et al. 2012) and pollen development in Arabidopsis (Borges et al. 2011). In Arabidopsis, AGO5 is highly and almost exclusively expressed during all stages of flowers and seed formation (Schmid et al. 2005, Kapoor et al. 2008, Waese et al. 2017), including inflorescence meristems (Mantegazza et al. 2014), suggesting a primordial role for this protein in the reproductive phase of development. We find that AGO5 is also highly expressed in the SAM of adult plants where it appears to target SPL transcripts in a miR156-dependent manner. At the same time, AGO1 has previously identified as an important player in plant development (Vaucheret et al. 2004, Baumberger and Baulcombe 2005). Supporting this, AGO1 binds miR156 and miR157 in Arabidopsis seedlings, suggesting a role for AGO1 in the vegetative phase change (Azevedo et al. 2010, He et al. 2018). However, a role for AGO1 in miR156-dependant regulation of flowering time has not been demonstrated, possibly in part because Arabidopsis ago1 mutant phenotypes can be difficult to interpret due to dramatic pleiotropic developmental defects (Vaucheret et al. 2004). In our analysis, AGO1 shows a ubiquitous expression, as opposed to the tissue-specific expression of AGO5, which is restricted mainly to the SAM and reproductive tissues (Supplementary Fig. S3). The phenotypic aspect of 35S::miR156/ago5-5 plants suggests that AGO1 and AGO5 might act in the miR156 aging pathway but that they may have distinct functions in different tissues. While these plants displayed a flowering time comparable to WT plants, the leaf architecture resembled that of the miR156-overexpressing plants. Since miR156 is also known to be important for leaf development and morphology (Xie et al. 2012, Feng et al. 2016), but AGO5 is not expressed at significant levels in leaves (Fig. 2A and Supplementary Fig. S3), we suggest that the miR156 involvement in leaf development is likely due to an interaction with another AGO protein, probably AGO1. This would be consistent with the absence of a leaf morphology phenotype in ago5 mutants (Figs. 1A, 5B, C) and the fact that a lack of AGO5 does not affect the leaf morphology induced by miR156 overexpression (Fig. 5).
In later stages of plant development, other factors promote the expression of SPL genes above the threshold set by miR156, enabling phase transitions to occur (Xu et al. 2016). Expression levels of SPL3/4/5 are influenced by miR156-independent floral inductive signals such as CONSTANS (CO) and FLOWERING LOCUS T (FT) (Jung et al. 2012). Crosstalk between gibberellic acid (GA) and miR156-regulated pathways has also been reported. In both long- and short-day photoperiods, GA has been shown to play an important role in the expression of SPLs and in their targets, such as SOC1 and FUL (Moon et al. 2003, Galvao et al. 2012, Porri et al. 2012). Interestingly, miR156 levels are largely unaffected by the activity of the aforementioned pathways. Despite low levels of miR156 in late developmental stages, He et al. (2018) suggested that this miRNA may act in fine-tuning SPL expression in a threshold-dependent manner. Indeed, miR156-dependent regulation of SPL genes has been shown to be regulated at the translational level as well (He et al. 2018) and, thus, protein levels are likely to differ even more than mRNA levels. The expression of AGO5 specifically in the SAM and the higher abundance of SPL transcripts in ago5 mutants suggest that AGO5 plays a role in miR156-mediated flowering by acting on meristem identity. In agreement with this, when the expression of SPL and SPL-regulated genes was assessed in Col-0, 35S::miR156 and the 35S::miR156/ago5-5 near bolting time, we observed similar expression patterns between Col-0 and the 35S::miR156/ago5-5. In contrast, in 20-day-old 35S::miR156/ago5-5 plants, no significant difference was observed with respect to SPL and SPL-regulated gene expression as compared to the 35S::miR156 line alone. This observation reinforces the hypothesis that AGO5 mediates the fine-tuning of SPLs through its interaction with the miR156 in the SAM.
To conclude, we propose a model whereby a novel AGO–miRNA interaction mediates the fine-tuning of flowering time in Arabidopsis. Through genetic and biochemical approaches, we confirm that a physical and functional interaction between AGO5 and miR156 is necessary for regulating the miR156-aging pathway in the SAM but not in leaves. As such, this study highlights the complexity by which the RNA silencing machinery modulates different aspects of plant development by allowing different AGO proteins to act in tissue-specific manners.
Materials and Methods
Plant material and growth conditions
All A. thaliana plants were grown in Agromix (Fafard, Saint-Bonaventure, QC, Canada) in growth chambers with either a 16-h light and 8-h dark photoperiod or a 12-h light and 12-h dark photoperiod at 22°C. Except for the 35S::miR156/ago5-5 hybrid, all Arabidopsis mutant lines have been previously described including ago5-1 (Katiyar-Agarwal et al. 2007), ago5-5 (Brosseau and Moffett 2015) and 35S::miR156 (Wu et al. 2009). The 35S::miR156/ago5-5 line was generated by crossing the 35S:miR156 transgenic line with ago5-5 mutant. Newly generated lines were genotyped using the primers listed in Supplementary Table S1.
RNA extraction and treatment
Total RNA was extracted using TRIzol (Invitrogen, Burlington, ON, Canada) and then treated with RNase-free DNase (Ambion, Burlington, ON, Canada) following the manufacturer’s instructions. Tissue was enriched for meristems (including meristem and leaf primordia) by removing the cotyledons as well as all leaves >5 mm, as previously described (Xu et al. 2016). This tissue enrichment is referred to as SAM throughout. The quantity and purity of total RNA samples were determined from 280/260 to 260/230 nm absorbance ratios measured using a Nanophotometer (Implen, Westlake Village, CA, USA). Ratios of 1.8 and 2.3, respectively, were accepted as pure RNA, and electrophoresis with agarose gel 2% was carried out to observe RNA integrity. Following RNA extraction, 2 μg of RNA was reverse transcribed with SuperScript II (Invitrogen, Burlington, ON, Canada) according to the manufacturer’s instructions.
RT-qPCR and gene expression analysis
RT-qPCR analysis was performed using a BioRad CFX96 real-time PCR system (Bio-Rad, Mississauga, ON, Canada) operated by CFX Manager software (version 3.0). In all assays, the samples analyzed included technical and biological triplicates. Each biological replicate included meristems from eight different plants. The specificity of each primer pair was verified from the dissociation (melt) curves acquired after 40 cycles using CFX Manager software (version 3.0). PCR amplification efficiency (E) was determined for the validation of primers according to the standard curve method after using a set of all cDNA samples with 5-fold serial dilution. The RT-qPCR was programmed with an initial step of 98°C for 2 min and 40 cycles of 98°C for 2 s and annealing/extension at 60°C for 5 s, with melt curve analyses from 65°C to 95°C in 0.5°C increments. ACTIN2 was used as a reference gene for the normalization of the relative expression data as described previously (Xu et al. 2016). Average Cq values were then normalized by ΔΔCT formula against the indicated reference gene. The specific primer efficiency was considered in the relative gene expression analysis as suggested previously (Pfaffl 2001). Primer sequences used in this study and their efficiency are listed in Supplementary Table S1.
miRNA immunoprecipitation
AGO–miRNA complexes were obtained by grinding 1-week-old plants with a mortar in cold extraction buffer and subjected to immunoprecipitation (IP) as described previously (Carbonell et al. 2012, Brosseau and Moffett 2015) using an anti-AGO5 antibody (AS10671, Agrisera, Vännäs, Sweden). Prior to IP, 100 μl of the total extract was set aside for the analysis of input miRNAs, from which small RNAs were extracted as previously described (Varkonyi-Gasic et al. 2007). RNA was extracted from AGO IP complexes using TRIzol (Invitrogen, Burlington, ON, Canada). Thereafter, miRNA stem-loop reverse transcription (SL-RT) was performed as described, with modifications (Varkonyi-Gasic et al. 2007). PCR was performed using Taq polymerase using the following cycles: 94°C for 2 min; 94°C for 15 s; 53°C for 25 s; 72°C for 20 s; 50 cycles. PCR primers are listed in Supplementary Table S1.
Microscopy assay
The floral architecture was verified under a Leica M165FC stereomicroscope with a LeicaM170HD camera (Leica Microsystems Inc., Concord, ON, Canada). All photos were taken with a 0.63× objective.
Acknowledgments
We are thankful to Daniel Garneau for help with stereomicroscopy and Marie Bernadette Dib Diam for providing the 5.8S rRNA primer.
Funding
Discovery grant from the Natural Sciences and Engineering Research Council (Canada) to P.M. and the Agrophytosciences CREATE program to C.R.-L. and G. S.-M.
References
Author notes
Charles Roussin-Léveillée and Guilherme Silva-Martins contributed equally to this work