-
PDF
- Split View
-
Views
-
Cite
Cite
Martin Gritter, Kuang-Yu Wei, Rosa D Wouda, Usha M Musterd-Bhaggoe, Kyra L Dijkstra, Jesper Kers, Christian Ramakers, Liffert Vogt, Martin H de Borst, Alexander H J Danser, Ewout J Hoorn, Joris I Rotmans, Chronic kidney disease increases the susceptibility to negative effects of low and high potassium intake, Nephrology Dialysis Transplantation, Volume 39, Issue 5, May 2024, Pages 795–807, https://doi.org/10.1093/ndt/gfad220
- Share Icon Share
ABSTRACT
Dietary potassium (K+) has emerged as a modifiable factor for cardiovascular and kidney health in the general population, but its role in people with chronic kidney disease (CKD) is unclear. Here, we hypothesize that CKD increases the susceptibility to the negative effects of low and high K+ diets.
We compared the effects of low, normal and high KChloride (KCl) diets and a high KCitrate diet for 4 weeks in male rats with normal kidney function and in male rats with CKD using the 5/6th nephrectomy model (5/6Nx).
Compared with rats with normal kidney function, 5/6Nx rats on the low KCl diet developed more severe extracellular and intracellular K+ depletion and more severe kidney injury, characterized by nephromegaly, infiltration of T cells and macrophages, decreased estimated glomerular filtration rate and increased albuminuria. The high KCl diet caused hyperkalemia, hyperaldosteronism, hyperchloremic metabolic acidosis and severe hypertension in 5/6Nx but not in sham rats. The high KCitrate diet caused hypochloremic metabolic alkalosis but attenuated hypertension despite higher abundance of the phosphorylated sodium chloride cotransporter (pNCC) and similar levels of plasma aldosterone and epithelial sodium channel abundance. All 5/6Nx groups had more collagen deposition than the sham groups and this effect was most pronounced in the high KCitrate group. Plasma aldosterone correlated strongly with kidney collagen deposition.
CKD increases the susceptibility to negative effects of low and high K+ diets in male rats, although the injury patterns are different. The low K+ diet caused inflammation, nephromegaly and kidney function decline, whereas the high K+ diet caused hypertension, hyperaldosteronism and kidney fibrosis. High KCitrate attenuated the hypertensive but not the pro-fibrotic effect of high KCl, which may be attributable to K+-induced aldosterone secretion. Our data suggest that especially in people with CKD it is important to identify the optimal threshold of dietary K+ intake.
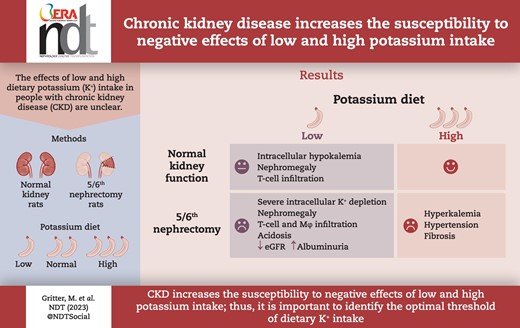
What was known:
The general population and people with chronic kidney disease (CKD) consume less dietary K+ than recommended.
Increasing K+ intake to recommended levels improves cardiovascular and kidney health in the general population, but the effects in people with CKD are less clear.
This study adds:
In rats with normal kidney function, low dietary K+ intake caused mild hypokalemia and nephromegaly, whereas high dietary K+ intake had no adverse effects.
In rats with CKD induced by 5/6th nephrectomy, low dietary K+ intake caused severe intracellular and extracellular K+ depletion and kidney injury characterized by nephromegaly and T-cell infiltration.
High dietary K+ intake caused hyperkalemia, hyperaldosteronism and hypertension with kidney fibrosis.
Potential impact:
CKD increases susceptibility to the negative effects of low and high dietary K+ intake, suggesting that especially in people with CKD it is important to identify the optimal threshold of dietary K+ intake.
INTRODUCTION
In the general population dietary potassium (K+) intake is ∼54 mmol/day [1] which is below the recommended intake of 90–120 mmol/day [2, 3]. Low dietary K+ intake is associated with salt-sensitive hypertension [4] and with a higher incidence of stroke [5], chronic kidney disease (CKD) [6] and mortality [7]. Increasing K+ intake through K+ supplementation or salt substitution decreases blood pressure and improves cardiovascular outcomes [5, 8]. People with CKD have a similar dietary K+ intake to the general population [9]. At present it is unclear whether the health benefits of adequate dietary K+ intake in the general population also apply to people with CKD [10]. Several studies have shown that higher estimated dietary K+ intake is associated with better kidney outcomes [11], cardiovascular outcomes [12] or lower mortality in people with CKD [13]. On the other hand, people with CKD are prone to hyperkalemia which could also have untoward effects [14]. Our recent study showed that short-term KChloride (KCl) supplementation in people with CKD normalized dietary K+ intake and caused overt hyperkalemia in 11% of the participants [15].
In addition to the health effects of dietary K+ in people with CKD, another relevant and more physiological question is how CKD modifies the response to dietary K+. Several previous studies in animals have characterized the effects of low and high K+ diets [16–22], but few of these studies were conducted in animal models of CKD. In addition, no studies have directly compared the effects of low and high K+ diets in animals with normal kidney function and animals with CKD. From these previous studies, it is clear that a low K+ diet causes a form of kidney injury that is characterized by inflammation and fibrosis (“kaliopenic nephropathy”) [23]. In addition, a low K+ diet activates the so-called K+ switch, which increases the activity of the sodium-chloride cotransporter (NCC) [10]. This response helps to conserve K+ but can also cause salt-sensitive hypertension [10, 17]. In the 5/6th nephrectomy (5/6Nx) model of CKD, a K+-deficient diet exaggerated proximal tubular hypertrophy compared with normal rats [16]. A high K+ diet is able to relieve part of the kidney injury induced by a low K+ or high sodium chloride diet [20, 24]. Moreover, a high K+ diet inactivates NCC leading to K+-induced natriuresis with lowering of blood pressure [25, 26]. However, a chronic high K+ diet in mice can cause hypertension even in the presence of NCC inhibition [25, 27]. The same hypertensive response to a high K+ diet has been reported in 5/6Nx rats [28]. In contrast, Wang et al. found that a high K+ diet decreased blood pressure and kidney injury compared with a normal K+ diet in 5/6Nx rats [29]. It is important to emphasize that different percentages of KCl were used in these previous studies, including ranges of 0%–0.5% for the low K+ diet, 0.4%–1.0% for the normal K+ diet and 2.6%–7.6% for the high K+ diet. Of note, if the recommendations for adequate dietary K+ intake in humans (90–120 mmol/day) are translated to rodents, a normal K+ diet would be 0.1% KCl.
The aim of the present study was to compare the effects of a low, normal and high K+ diet in normal rats and rats with CKD using the 5/6Nx model. The effects of these diets on plasma electrolyte and acid–base levels, intracellular K+ concentrations, blood pressure, kidney function and kidney histology were analyzed.
MATERIALS AND METHODS
Study design
All animal procedures were approved by the Animal Welfare Body of the Erasmus Medical Center (license AVD101002016790). Male Sprague–Dawley rats (Envigo, UK) were housed in individual cages with free access to water and their designated diets. CKD was established through a two-step surgical 5/6th nephrectomy procedure as described previously [30]. The first surgery (right nephrectomy) was combined with telemetry implantation (Data Sciences International, St Paul, MN, USA) in the abdominal aorta. Four weeks after the second surgery, baseline measurements were performed including a 72-h blood pressure measurement, a 24-h urine collection in metabolism cages and a tail vein blood sample collection (Supplementary data, Fig. S1). Subsequently, rats were randomized to their respective diets for 4 weeks. At the end of this time period, the 24-h urine collection was repeated and rats were sacrificed with collection of blood from the portal vein. Kidneys were either stored in formalin followed by ethanol fixation for histological analysis or snap frozen and stored at −80°C for immunoblot analysis.
K+ diets
Rats were randomly assigned to diets containing 0.03%, 0.1% or 2.5% K+ (ssniff Spezialdiäten GmbH, Soest, Germany). A dietary K+ content of 0.1% was calculated to represent adequate dietary K+ intake in rats (see Supplementary data, Table S1). Dietary K+ contents of 0.03% and 2.5% were chosen to represent low and high K+ intakes, respectively, because these contents provide clear differences from the 0.1% K+ diet but avoid the extreme K+ stress associated with 0% and 5% K+ diets [27]. The 2.5% K+ diets were either provided as KCl or KCitrate. All diets contained 0.2% sodium. The chloride contents were 0.3% for the low KCl, normal KCl and high KCitrate diet, and 2.5% for the high KCl diet.
Blood, urine and intracellular measurements
Before the start of the intervention and at end of study, blood and 24-h urine were collected for analysis of electrolyte and acid–base homeostasis, renin–angiotensin system activity and kidney function (Supplementary data). At end of study, muscle and skin samples were obtained from the abdominal wall for intracellular K+ measurements (Supplementary data). Estimated glomerular filtration rate (eGFR) measurements were performed through a recently validated formula in rats [31].
Kidney immunoblot and histology
The abundances of NCC, phosphorylated NCC (pNCC), full-length alpha–epithelial sodium channel (α-ENaC), cleaved α-ENaC and pendrin in the remnant kidney were analyzed by immunoblot. GAPDH was used as housekeeping protein (Supplementary data, Table S2). Immunohistochemistry on kidney slices fixed in ethanol was performed to identify macrophages with an anti-CD68 antibody and T cells with an anti-CD3 antibody (both from Abcam). Collagen staining was performed as measure of fibrosis using picrosirius red staining (see Supplementary data).
Statistical analysis
Data are expressed as mean and standard error of the mean except for plasma renin, plasma aldosterone and urine albumin, which are expressed as median and interquartile range because these data were not normally distributed. At baseline, differences between sham and 5/6Nx rats were analyzed by independent t-tests. At end of study, analyses were either performed between diets within the same model (i.e. sham or 5/6Nx) by analysis of variance (ANOVA) with Dunnetts post hoc testing (comparison with the 0.1% KCl diet) or between models within the same diet by independent t-tests. Non-normally distributed data were log-transformed prior to testing. Blood pressure trajectories were analyzed by two-way repeated measures ANOVA. The correlations between end of study systolic blood pressure and plasma aldosterone and plasma chloride, and the correlation between plasma aldosterone and picrosirius red staining were assessed by Pearson’s r and multivariable regression analysis. Data were analyzed with GraphPad Prism version 8 (GraphPad Software Inc., La Jolla, CA, USA). A P-value ≤.05 was considered statistically significant.
RESULTS
Dietary K+ effects on electrolyte and acid–base balance
5/6Nx increased plasma creatinine, albuminuria, blood pressure and heart rate, but had no significant effects on body weight, food intake or activity (Supplementary data, Fig. S2). These data support 5/6Nx as a model for CKD. Plasma creatinine did not differ among 5/6Nx rats which were subsequently randomized to the four different diets (data not shown). After baseline measurements, rats were randomly assigned to a low KCl, normal KCl, high KCl or high KCitrate diet for 4 weeks. The low KCl diet caused hypokalemia in both sham and 5/6Nx rats but this was significantly more severe in 5/6Nx rats (Fig. 1A). The high K+ diets increased plasma K+ in 5/6Nx but not in sham rats. Sham rats developed normochloremic metabolic alkalosis on the low KCl and high KCitrate diets, while 5/6Nx rats on the high KCitrate diet developed hypochloremic metabolic alkalosis (Fig. 1B and C). The remaining 5/6Nx groups all developed hyperchloremic metabolic acidosis. Compared with sham rats, venous plasma pH was lower in the 5/6Nx rats on the low, normal and high KCl diets, but significantly higher on the high KCitrate diet (Fig. 1D). In sham rats, the high KCl diet caused slightly lower plasma renin activity. 5/6Nx suppressed plasma renin, and both high K+ diets suppressed this further (Fig. 1E). Plasma aldosterone increased with high K+ diets, especially in 5/6Nx rats (Fig. 1F). Compared with sham rats, body weight was lower in 5/6Nx rats on the low KCl diet despite higher food intake; body weight was also lower in 5/6Nx rats on the high KCitrate diet (Supplementary data, Table S3A and B). Urine volume was higher in all 5/6Nx rats compared with sham rats, and high K+ diets increased urine volume compared with the normal KCl diet (Supplementary data, Fig. S3).
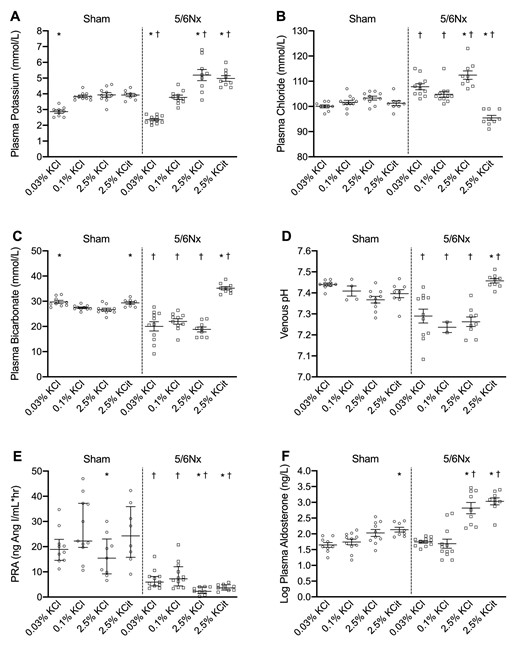
Dietary K+ effects on electrolyte and acid–base balance. Dietary K+ effects on (A) plasma K+, (B) chloride, (C) bicarbonate, (D) venous pH, (E) plasma renin activity (PRA) and (F) aldosterone (n = 8–10/group, except for plasma pH in rats on the 0.1% KCl diet n = 2–4/group). *P ≤ .05 compared with the 0.1% KCl diet within the same model (analyzed by ANOVA). †P ≤ .05 compared with sham rats on the same diet (analyzed by independent t-test).
Dietary K+ effects on urinary excretions
Urine K+ excretion was significantly higher in all high K+ diet groups (Fig. 2A). Urine chloride increased in sham and 5/6Nx rats on the high KCl diet; urinary chloride excretion was also higher in 5/6Nx rats on the low KCl and high KCitrate diets compared with sham rats on the same diets (Fig. 2B). Urine sodium excretion was higher on the high K+ diets especially in 5/6Nx rats (Fig. 2C) despite similar intake (Supplementary data, Table S3B). Plasma K+ correlated significantly with urine sodium excretion (data not shown). Urine ammonium excretion was higher in 5/6Nx rats compared with sham rats, although this was statistically significant only for the low KCl and high KCitrate diets (Fig. 2D). Urine ammonium excretion was suppressed by the high KCitrate diet in sham and 5/6Nx rats.
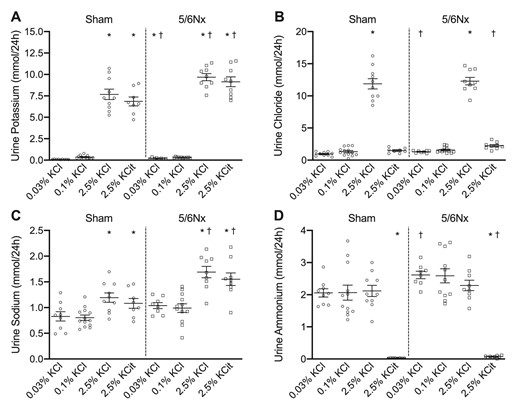
Dietary K+ effects on urine K+, chloride, sodium and ammonium excretion. Dietary K+ effects on urine (A) K+, (B) chloride, (C) sodium and (D) ammonium excretion (n = 8–10/group). *P ≤ .05 compared with the 0.1% KCl diet within the same model (analyzed by ANOVA). †P ≤ .05 compared with sham rats on the same diet (analyzed by independent t-test).
Rats develop intracellular K+ depletion on the low K+ diet
In 5/6Nx rats, the low KCl diet significantly decreased intracellular K+ concentration in muscle, red blood cell and skin compared with the high K+ diets (Fig. 3A–C). There were no significant differences in intracellular K+ concentration in sham rats. Intracellular K+ concentration in muscle and red blood cells was significantly lower in 5/6Nx rats on the low KCl diet compared with sham rats on the low KCl diet (Fig. 3A and B).
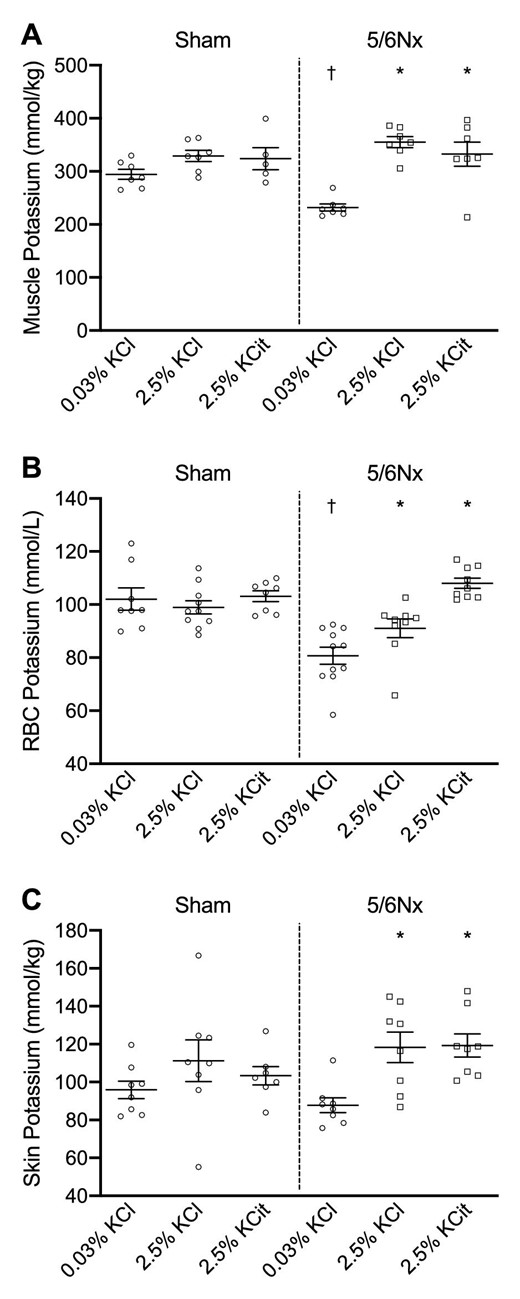
Dietary K+ effects on intracellular K+ concentrations. Dietary K+ effects on intracellular K+ concentrations in (A) muscle, (B) red blood cells (RBC) and (C) skin. *P ≤ .05 compared with the 0.03% KCl diet within the same model (analyzed by ANOVA). †P ≤ .05 compared with sham rats on the same diet (analyzed by independent t-test).
5/6Nx but not sham rats develop hypertension on high K+ diets
In sham rats, blood pressure was unaffected by K+ intake (Fig. 4A and B). In 5/6Nx rats, the high KCl diet significantly increased systolic and diastolic blood pressure (Fig. 4C and D). The high KCitrate diet also significantly increased systolic and diastolic blood pressure, but this was attenuated compared with the high KCl diet. In 5/6Nx rats, end of study systolic blood pressure showed significant positive correlations with plasma aldosterone (r = 0.52, P = .003) and plasma chloride (r = 0.45, P = .01) (Fig. 4E and F), which together predicted 60% of the variability in blood pressure between groups. Blood pressures remained constant in 5/6Nx rats on the low and normal KCl diets. Plasma K+ correlated significantly with systolic blood pressure (data not shown).
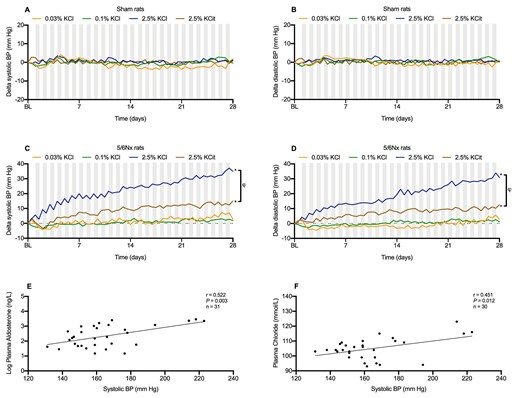
Dietary K+ effects on telemetric blood pressure. Dietary K+ effects on systolic and diastolic blood pressure in (A, B) sham rats and (C, D) 5/6Nx rats (n = 8–10/group). Correlations between plasma aldosterone, plasma chloride and final systolic blood pressure in 5/6Nx rats (E, F). *P ≤ .05 compared with the 0.1% KCl diet within the same model (analyzed by ANOVA). †P ≤ .05 between high KCl and high K-Citrate diets (analyzed by two-way repeated measures ANOVA). Nighttime blood pressures are shown in gray columns, daytime blood pressure in white columns.
NCC, ENaC and pendrin
In 5/6Nx rats, the low KCl diet increased pNCC, while the high KCl diet decreased pNCC (Fig. 5A and B), as reported previously for normal rats and mice [27, 32–36]. In group comparison, however, these changes did not reach statistical significance, likely because the high KCitrate diet caused a ∼5-fold increase in pNCC. The abundance of full-length and cleaved α-ENaC increased significantly with both high K+ diets. Pendrin abundance increased significantly only on the high KCitrate diet. Plasma chloride but not plasma K+ showed a significant negative correlation with pNCC abundance (Fig. 5C). Because low intracellular chloride phosphorylates NCC [37], we hypothesized that the hypochloremic metabolic alkalosis secondary to high KCitrate obscured the correlation between plasma K+ and pNCC. To address this, we repeated the correlation analysis without the KCitrate group and were able to demonstrate a significant negative correlation between plasma K+ and pNCC abundance (Fig. 5C), similar to what has been reported previously [33].
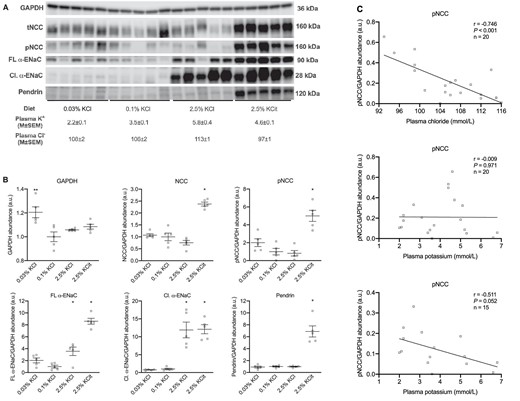
Dietary K+ effects on NCC, ENaC, and pendrin in 5/6Nx rats. Immunoblots and corresponding densitometry (n = 5/group, A and B). Pearson correlations between kidney phosphorylated NCC with plasma Cl− and plasma K+ (C). The correlation between pNCC and plasma K+ was analyzed with and without the high KCitrate group. *P ≤ .05 compared with the 0.1% KCl diet (analyzed by ANOVA).
Different kidney injury patterns on low vs high K+ diets
In 5/6Nx but not in sham rats, the low KCl diet increased plasma creatinine and urea, and decreased eGFR compared with the 0.1% KCl diet (Fig. 6A–C). The low KCl diet also caused more severe albuminuria in 5/6Nx rats (Fig. 6D). 5/6Nx rats had higher kidney weight than sham rats as reported previously [38]. The low KCl diet increased kidney weight significantly both in sham and 5/6Nx rats (Fig. 6E), although this effect was stronger in the 5/6Nx rats. Collagen deposition in the remnant kidney of the 5/6Nx rats was greater than in sham rats (Fig. 7A), and the high KCitrate diet increased this further (Fig. 7B). 5/6Nx rats had a higher number of T cells per mm2 in their remnant kidneys than the sham rats and the low KCl diet increased this further in both sham and 5/6Nx rats (Fig. 7A and B). There was more macrophage infiltration in 5/6Nx rats than in sham rats, but this was only statistically significant on the low KCl diet (Fig. 7A and B). Plasma aldosterone concentration and Sirius red staining correlated strongly (r = 0.53, P < .001) (Fig. 7B).
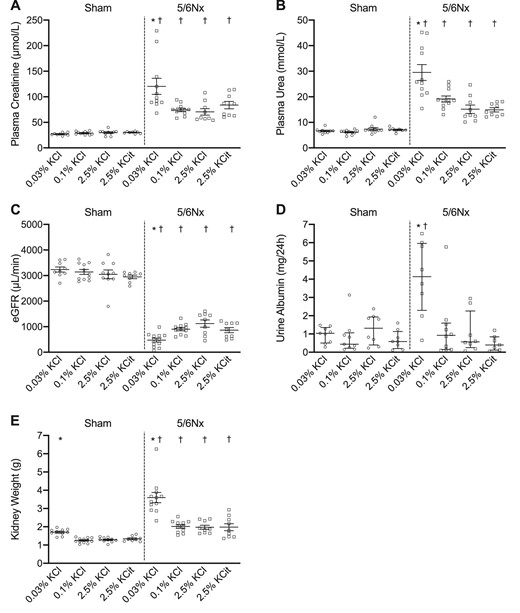
Dietary K+ effects on kidney function. Dietary K+ effects on (A) plasma creatinine, (B) plasma urea, (C) eGFR, (D) urine albumin and (E) kidney weight (n = 8–11/group). *P ≤ .05 compared with the 0.1% KCl diet within the same model (analyzed by ANOVA). †P ≤ .05 compared with sham rats on the same diet (analyzed by independent t-test).
Dietary K+ effects on collagen deposition, T-cell infiltration and macrophage infiltration. Representative immunohistochemic kidney images (A) and quantification of picrosirius red staining, CD3+ cells and CD68+ cells and the correlation between plasma aldosterone and picrosirius red staining (B). *P ≤ .05 compared with the 0.1% KCl diet within the same model (analyzed by ANOVA). †P ≤ .05 compared with sham rats on the same diet (analyzed by independent t-test).
DISCUSSION
In this study, we tested the hypothesis that CKD aggravates the detrimental effects of low and high K+ intake using the 5/6Nx model. Our study shows that 5/6Nx rats are more susceptible to negative effects of a low and high K+ diet than normal rats. The injury patterns, however, differ and are characterized by inflammatory injury with kidney function decline on the low K+ diet and hypertensive injury with preserved kidney function on the high K+ diet (Fig. 8). We also show that the hypertensive effect of the high KCl diet is attenuated with the high KCitrate diet despite higher pNCC abundance. Our data add several novel insights into K+ homeostasis in the setting of CKD with relevance for the current debate on dietary K+ in people with CKD [39].
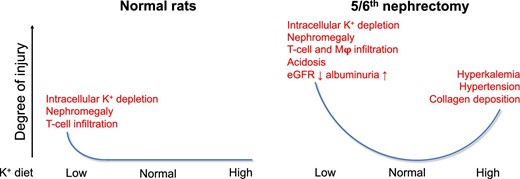
Detrimental effects of low and high dietary K+ in normal and 5/6Nx rats. The figure shows the degree of injury and the types of injuries observed on the low, normal and high K+ diets used in this study. We found that the 5/6Nx model of CKD aggravated the negative effects of both a low and high K+ diet.
Our study shows that rats with CKD are less capable of conserving K+ and therefore develop more severe hypokalemia on a low K+ diet. Because K+ is mostly an intracellular cation, we determined concentrations of K+ in red blood cells, muscle and skin. Intracellular K+ concentrations were lower in the rats with CKD on the low K+ diet than in sham rats, indicating total body K+ depletion. Total body K+ depletion has been reported previously in humans with CKD and is associated with increased mortality [40]. While hypokalemia in the normal rats on the low K+ diet was accompanied by the expected metabolic alkalosis, the rats with CKD on the low K+ diet developed hyperchloremic metabolic acidosis with higher urine ammonium excretion. Further impairment of kidney function in the rats with CKD on the low K+ diet may explain this metabolic acidosis. The high K+ diets resulted in higher plasma K+ levels in the rats with CKD although few animals developed overt hyperkalemia. In people with CKD, the relationship between dietary K+ intake and plasma K+ is weak [41], while this relationship is stronger for K+ supplements [15]. This difference is likely explained by a lower bioavailability of K+ in foods than in supplements [42]. Because the bioavailability of K+ in rodent chow is also likely to be near-maximal, this may explain the diet-induced rise in plasma K+. The high KCitrate diet caused severe hypochloremic alkalosis and pendrin upregulation in the rats with CKD. Metabolic alkalosis likely developed because the excretion of the dietary alkali load was impaired by the low GFR and hypochloremia [43]. In turn, hypochloremia was likely the result of the Cl−-deficient diet and possibly higher urinary chloride excretion secondary to an expanded extracellular fluid volume [44].
The development of hypertension secondary to extreme high K+ diets in rodents has now been reported by several groups [25, 27, 28, 36], and was also clear in our 5/6Nx rats on a less extreme but still unphysiologically high 2.5% K+ diet. This suggests that increasing dietary K+ intake may only decrease blood pressure when it is used to normalize a low K+ diet. This is in agreement with a previous meta-analysis which demonstrated a U-shaped relationship between dietary K+ intake and blood pressure [45]. There are several possible reasons why a high K+ diet increases blood pressure, including higher plasma aldosterone, hyperchloremia and metabolic acidosis [46]. Higher ENaC abundance is compatible with an aldosterone effect, although ENaC can also be activated directly by extracellular K+ [47, 48]. Little et al. recently showed that hypertension on a high K+ diet can be reversed by amiloride further supporting a role for ENaC [25]. An interesting finding was that the attenuated hypertension on the high KCitrate diet occurred with higher pNCC and with similar plasma aldosterone and ENaC levels. The stimulatory effect of KCitrate on pNCC has been reported previously [32]. We propose that hypochloremia explained higher pNCC assuming that intracellular chloride concentrations were also lower, which are a recognized stimulant for NCC [49]. The greater rise in blood pressure with KCl suggests a role for dietary chloride and, indeed, we found a significant correlation between plasma chloride and blood pressure. Previous studies demonstrated an effect of dietary chloride on blood pressure. In uninephrectomized rats treated with desoxycorticosterone, a high sodium chloride but not sodium bicarbonate diet increased blood pressure [50]. In 25 patients with essential hypertension, K+ citrate supplementation decreased blood pressure to a greater extent than K+ chloride [51]. Chloride can induce renal vasoconstriction, which could potentially result in higher blood pressure [52]. Another interesting observation is the high urinary sodium excretion in the high dietary K+ groups. This has been shown previously [25, 53] and, although speculative, may imply that the animals are not in steady state or that part of the K+ salts are excreted as sodium salts.
One could argue that the injury pattern secondary to the low K+ diet is more detrimental than that observed with the high K+ diet. The low K+ diet caused injury regardless of the presence of CKD and worsened eGFR and albuminuria in the rats with CKD. This raises the possibility that the associations between lower urinary K+ and worse kidney outcomes in observational studies [11] may be explained by a direct nephrotoxic effect of low dietary K+ intake. The pattern of kidney injury that we observed with the low K+ diet is consistent with previous literature, which also reported nephromegaly and decreased creatinine clearance [17–19, 21, 22, 54, 55]. Other common manifestations of hypokalemia-induced nephropathy include macrophage infiltration [17, 19, 22] and fibrosis [17–19]. T-cell infiltration has also been reported previously in the context of hypokalemic injury [56], and the general role of macrophages and T cells in kidney injury and fibrosis is well-characterized [57]. The kidney injury pattern in rats with CKD on the high K+ diets was different from the low K+ diet in the sense that it was characterized by increased collagen deposition without a clear influx of macrophages or T cells. A recent study in two different mouse models of CKD showed that K+-induced fibrosis is dependent on the mineralocorticoid receptor [58]. This suggests a role for the K+-induced rise in plasma aldosterone, which is also supported by the strong correlation between plasma aldosterone and collagen deposition.
The strength of this study is the direct comparison between the effects of low, normal and high K+ diets in rats with normal kidney function and rats with CKD. The high K+ diets had no clear negative effects in the rats with normal kidney function, whereas previous studies did show that mice on a high K+ diet developed hypertension [25, 27, 36]. This may be explained by a species difference or difference in the magnitude of K+ supplementation. We also acknowledge a number of limitations of our study. First, the multi-group comparison forced us to make choices and therefore we tested KCitrate only as high K+ diet and measured the intracellular K+ concentrations only in rats on the low and high K+ diets. Only male rats were used in order to limit the number of animals and the blood pressure variability related to sex hormones. However, given the emerging insights on the importance of sex differences in tubular transport and hypertension, future studies should also include female rats. We tested the diets only in the 5/6Nx model of CKD and it therefore remains to be determined to what degree our results also apply to other experimental CKD models or to human CKD. Finally, we used less extreme K+ diets than in previous studies, but acknowledge that they are still supraphysiological. Indeed, the 2.5% K+ diets for rats in our experimental conditions result in a 25-fold higher potassium intake compared with a regular diet for rats, which is outside the range of physiological variations in humans. Therefore, these results should be interpreted with caution.
In conclusion, CKD increases susceptibility to the negative effects of low and high K+ diets but leads to different injury patterns including inflammation, fibrosis and kidney function decline with the low K+ diet and hyperaldosteronism, hypertension and fibrosis on the high K+ diet. Our results suggest that especially in people with CKD it is important to establish the optimal threshold of dietary K+ intake to avoid the negative effects of a low and high K+ diet.
FUNDING
This study was supported by the Dutch Kidney Foundation (CP16.01)
AUTHORS’ CONTRIBUTIONS
M.G. (conceptualization; data curation; formal analysis; investigation; methodology; visualization; writing—original draft). K.-Y.W. (data curation; formal analysis; writing—review and editing). R.D.W. (data curation; formal analysis; methodology; writing—review and editing). U.M.M.-B. (data curation; investigation; methodology; writing—review and editing). K.L.D. (data curation; formal analysis; methodology; writing—review and editing). J.K. (methodology; supervision; writing—review and editing). C.R. (methodology; supervision; writing—review and editing). L.V. (conceptualization; supervision; writing—review and editing). M.H.d.B. (conceptualization; supervision; writing—review and editing). A.H.J.D. (methodology; supervision; writing—review and editing). E.J.H. (conceptualization; formal analysis; funding acquisition; project administration; supervision; visualization; writing—original draft; writing—review and editing). J.I.R. (conceptualization; funding acquisition; methodology; project administration; supervision; writing—review and editing).
DATA AVAILABILITY STATEMENT
The data underlying this article are available in the article and in its online Supplementary data.
CONFLICT OF INTEREST STATEMENT
The authors declare no conflict of interest.
REFERENCES
Author notes
These authors contributed equally to this work
Comments