-
PDF
- Split View
-
Views
-
Cite
Cite
Christian Ott, Felix Mahfoud, Giuseppe Mancia, Krzysztof Narkiewicz, Luis M Ruilope, Martin Fahy, Markus P Schlaich, Michael Böhm, Roland E Schmieder, Renal denervation in patients with versus without chronic kidney disease: results from the Global SYMPLICITY Registry with follow-up data of 3 years, Nephrology Dialysis Transplantation, Volume 37, Issue 2, February 2022, Pages 304–310, https://doi.org/10.1093/ndt/gfab154
- Share Icon Share
Abstract
Activity of the sympathetic nervous system is increased in patients with hypertension and chronic kidney disease (CKD). Here we compare short- and long-term blood pressure (BP)-lowering effects of renal denervation (RDN) between hypertensive patients with or without CKD in the Global SYMPLICITY Registry.
Office and 24-h ambulatory BP (ABP) were assessed at prespecified time points after RDN. The presence of CKD was defined according to the estimated glomerular filtration rate (eGFR) and enrolled patients were stratified based on the presence (n = 475, eGFR <60 mL/min/1.73 m2) or absence (n = 1505, eGFR ≥60mL/min/1.73 m2) of CKD.
Patients with CKD were older (P < 0.001) and were prescribed more antihypertensive medications (P < 0.001). eGFR decline per year was not significantly different between groups after the first year. Office and 24-h ABP were significantly reduced from baseline at all time points after RDN in both groups (all P < 0.001). After adjusting for baseline data, patients without CKD had a greater reduction in office systolic BP (−17.3 ± 28.3 versus −11.7 ± 29.9 mmHg; P = 0.009) but not diastolic BP at 36 months compared with those with CKD. Similar BP and eGFR results were found when the analysis was limited to patients with both baseline and 36-month BP data available. There was no difference in the safety profile of the RDN procedure between groups.
After adjusting for baseline data, 24-h systolic and diastolic ABP reduction were similar in patients with and without CKD after RDN, whereas office systolic but not diastolic BP was reduced less in patients with CKD. We conclude that RDN is an effective antihypertensive treatment option in CKD patients.
KEY LEARNING POINTS
What is already known about this subject?
Sympathetic nervous system activity contributes to hypertension and chronic kidney disease (CKD).
The potential of renal denervation (RDN) to treat uncontrolled hypertension in patients with CKD remains unknown, even though patients with CKD and hypertension may be most suitable for RDN therapy.
The Global SYMPLICITY Registry is the largest data set of RDN-treated patients to date and offers the unique opportunity to address this unsolved question.
What this study adds?
RDN resulted in a significant blood pressure (BP) reduction in short- and long-term follow-up. After adjusting for baseline data, office systolic, but not diastolic, BP reduction was greater in patients without CKD compared with those with CKD and there was no significant difference in 24-h ambulatory systolic and diastolic BP reduction between groups.
After the first year, estimated glomerular filtration rate decline per year was in the expected range and there was no clinically meaningful reduction in both groups.
The safety profile of the RDN procedure was not different between groups.
What impact this may have on practice or policy?
RDN emerged as an effective antihypertensive treatment option in CKD patients.
This retrospective analysis showed no differences in safety outcomes between patients with versus without CKD, supporting the safety of the procedure regardless of CKD.
INTRODUCTION
Hypertension is highly prevalent in patients with chronic kidney disease (CKD) and is considered both a common cause and a major consequence of CKD. More than 20% of people with CKD already have hypertension in the early stages of the disease, increasing to >80% at CKD Stage 4, with poor blood pressure (BP) control among most patients [1, 2].
The prevalence of CKD is expected to increase worldwide, along with the prevalence of secondary hypertension [3]. The mechanism of elevated BP in CKD is multifactorial. Experimental models and human studies have identified elevated sympathetic nervous system activity as a major pathophysiologic contributor to hypertension in CKD [4, 5]. Muscle sympathetic nerve activity in particular, as assessed by microneurography, was shown to be significantly and inversely correlated with estimated glomerular filtration rate (eGFR) in patients with hypertension [6].
Studies in experimental models have shown that surgical ablation can reduce sympathetic overactivity, prevent hypertension and slow the progression of renal disease [7, 8]. While experience with catheter-based renal denervation (RDN) in a clinical setting has increased over the last decade, data in patients with CKD are few and limited to small pilot-type studies [9, 10]. In fact, to date, most RDN studies have restricted enrollment to patients with preserved renal function, usually defined as an eGFR >45 or >60 mL/min/1.73 m2, and with eGFR itself being considered only as a safety parameter [11–13]. Therefore the potential of RDN to treat uncontrolled hypertension in patients with CKD remains unknown, even though patients with CKD and hypertension may be most suitable for RDN therapy and may present at least similar BP reductions as seen in primary hypertension. However, hypertensive patients with CKD have exaggerated vascular remodelling, leading to increased stiffness of large arteries that have been identified to mitigate BP reduction after RDN [14, 15].
The Global SYMPLICITY Registry (GSR) is the largest data set of RDN-treated patients to date and offers the unique opportunity to address this unsolved question. Herein we report data from the GSR comparing the short- and long-term BP-lowering effects of RDN for uncontrolled hypertension in patients with or without CKD as defined by eGFR >60 or <60 mL/min/1.73 m2, but excluding those on renal replacement therapy. We hypothesize that BP-lowering effects will be comparable in patients with and without CKD. To achieve target BP is of clinical importance and more intensive BP reduction has been linked not only to decelerating the decline of renal function, but also to reduced mortality [16, 17].
MATERIALS AND METHODS
GSR
The rationale and design of the GSR have been published previously [18]. Briefly, the GSR is a prospective, open-label, international, multicentre observational study for assessment of the safety and effectiveness of RDN among real-world patients treated with the Symplicity RDN system (Medtronic, Santa Rosa, CA, USA). Inclusion criteria are age ≥18 years and eligibility for RDN. Enrolled patients were treated based on uncontrolled hypertension and/or other conditions associated with sympathetic nervous system activation.
Patients were enrolled from February 2012 to February 2018 and those with available baseline data on BP and renal function (i.e. eGFR) were analysed over a follow-up period of 3 years. In total, 1980 patients were included and organized into subgroups according to the presence or absence of CKD [with CKD, n = 475 (baseline eGFR <60 mL/min/1.73 m2 but ≥15 mL/min/1.73 m2) versus without CKD, n = 1505 (baseline eGFR ≥60 mL/min/1.73 m2)]. Subgroup definitions were based on the Modification of Diet in Renal Disease formula or the Chronic Kidney Disease Epidemiology Collaboration formula [19]. Patients on renal replacement therapy, either on chronic dialysis or renal transplantation, were excluded, since the number was too small to allow for analysis.
The GSR is conducted according to the Declaration of Helsinki. The local ethics committees of all centres (N = 196 worldwide) approved the clinical protocol and informed consent was obtained from all patients prior to study entry. The GSR is registered at www.clinicaltrials.gov (NCT01534299).
Catheter-based RDN
After confirmation of suitable anatomy by renal angiography, patients underwent catheter-based RDN using the Symplicity Flex system (Medtronic).
Assessments
Three office BP measurements were taken after at least 5 min of rest with 1 min between the readings under standardized practice. In addition, 24-h ambulatory BP (ABP) measurements were performed in compliance with the guidelines of the European Society of Hypertension [20]. Renal function was assessed by eGFR, which was measured at prespecified time points (baseline and 12, 24 and 36 months after RDN). Annual changes in eGFR per year were calculated.
Statistical analyses
Continuous variables are presented as means ± standard deviation (SD) in the text and means ± standard error mean (SEM) in figures. Between-group differences in continuous variables were tested using the Wilcoxon–Mann–Whitney test. Within-group differences in continuous variables from baseline to follow-up were tested using paired t-tests. Categorical variables are presented as counts and percentages and were compared between groups using the chi-squared test. All tests are complete case analyses.
Additional analyses were performed: a multivariable linear regression that adjusted BP changes in both groups for baseline BP (office or ambulatory), age and gender and an analysis restricted to patients with both baseline and 36-month BP data. Two-sided P-values <0.05 were considered statistically significant.
Analyses were performed using SAS version 9.4 or higher (SAS Institute, Cary, NC, USA).
RESULTS
Baseline characteristics
Baseline characteristics for the overall population and for groups with and without CKD are shown in Table 1. Patients with CKD were significantly older (65.3 ± 10.7 versus 59.6 ± 11.7 years; P < 0.0001), were more commonly females (47.8% versus 40.0%; P = 0.003) and received more antihypertensive medications (4.78 ± 1.3 versus 4.48 ± 1.3; P < 0.001) compared with patients without CKD. At baseline, patients with CKD had lower office systolic (162.4 ± 25.7 versus 165.8 ± 24.5 mmHg; P = 0.011) and diastolic BP (83.3 ± 16.7 versus 91.3 ± 16.1 mmHg; P < 0.001) compared with patients without CKD, whereas there was no difference between groups in baseline 24-h ambulatory systolic BP. However, patients with CKD had lower 24-h ambulatory diastolic BP at baseline compared with those without CKD (82.0 ± 13.6 versus 87.8 ± 13.8 mmHg; P < 0.001).
Characteristics . | All (N = 1980) . | CKD negative (n = 1505) . | CKD positive (n = 475) . | P-value . |
---|---|---|---|---|
Age (years) | 60.9 ± 11.7 | 59.6 ± 11.7 | 65.3 ± 10.7 | <0.001 |
Male, % (n) | 58.1 (1151) | 60.0 (903) | 52.2 (248) | 0.003 |
Antihypertensive medication (n) | 4.55 ± 1.3 | 4.48 ± 1.3 | 4.78 ± 1.3 | <0.001 |
Office BP | ||||
Systolic (mmHg) | 165.0 ± 24.8 | 165.8 ± 24.5 | 162.4 ± 25.7 | 0.011 |
Diastolic (mmHg) | 89.4 ± 16.6 | 91.3 ± 16.1 | 83.3 ± 16.7 | <0.001 |
ABP | ||||
Systolic (mmHg) | 153.7 ± 18.1 | 153.7 ± 17.7 | 153.6 ± 19.0 | 0.916 |
Diastolic (mmHg) | 86.3 ± 14.0 | 87.8 ± 13.8 | 82.0 ± 13.6 | <0.001 |
eGFR (mL/min/1.73 m2) | 77.1 ± 24 | 86.6 ± 19 | 46.9 ± 10 | <0.001 |
Characteristics . | All (N = 1980) . | CKD negative (n = 1505) . | CKD positive (n = 475) . | P-value . |
---|---|---|---|---|
Age (years) | 60.9 ± 11.7 | 59.6 ± 11.7 | 65.3 ± 10.7 | <0.001 |
Male, % (n) | 58.1 (1151) | 60.0 (903) | 52.2 (248) | 0.003 |
Antihypertensive medication (n) | 4.55 ± 1.3 | 4.48 ± 1.3 | 4.78 ± 1.3 | <0.001 |
Office BP | ||||
Systolic (mmHg) | 165.0 ± 24.8 | 165.8 ± 24.5 | 162.4 ± 25.7 | 0.011 |
Diastolic (mmHg) | 89.4 ± 16.6 | 91.3 ± 16.1 | 83.3 ± 16.7 | <0.001 |
ABP | ||||
Systolic (mmHg) | 153.7 ± 18.1 | 153.7 ± 17.7 | 153.6 ± 19.0 | 0.916 |
Diastolic (mmHg) | 86.3 ± 14.0 | 87.8 ± 13.8 | 82.0 ± 13.6 | <0.001 |
eGFR (mL/min/1.73 m2) | 77.1 ± 24 | 86.6 ± 19 | 46.9 ± 10 | <0.001 |
Values are presented as mean ± SD unless stated otherwise.
Characteristics . | All (N = 1980) . | CKD negative (n = 1505) . | CKD positive (n = 475) . | P-value . |
---|---|---|---|---|
Age (years) | 60.9 ± 11.7 | 59.6 ± 11.7 | 65.3 ± 10.7 | <0.001 |
Male, % (n) | 58.1 (1151) | 60.0 (903) | 52.2 (248) | 0.003 |
Antihypertensive medication (n) | 4.55 ± 1.3 | 4.48 ± 1.3 | 4.78 ± 1.3 | <0.001 |
Office BP | ||||
Systolic (mmHg) | 165.0 ± 24.8 | 165.8 ± 24.5 | 162.4 ± 25.7 | 0.011 |
Diastolic (mmHg) | 89.4 ± 16.6 | 91.3 ± 16.1 | 83.3 ± 16.7 | <0.001 |
ABP | ||||
Systolic (mmHg) | 153.7 ± 18.1 | 153.7 ± 17.7 | 153.6 ± 19.0 | 0.916 |
Diastolic (mmHg) | 86.3 ± 14.0 | 87.8 ± 13.8 | 82.0 ± 13.6 | <0.001 |
eGFR (mL/min/1.73 m2) | 77.1 ± 24 | 86.6 ± 19 | 46.9 ± 10 | <0.001 |
Characteristics . | All (N = 1980) . | CKD negative (n = 1505) . | CKD positive (n = 475) . | P-value . |
---|---|---|---|---|
Age (years) | 60.9 ± 11.7 | 59.6 ± 11.7 | 65.3 ± 10.7 | <0.001 |
Male, % (n) | 58.1 (1151) | 60.0 (903) | 52.2 (248) | 0.003 |
Antihypertensive medication (n) | 4.55 ± 1.3 | 4.48 ± 1.3 | 4.78 ± 1.3 | <0.001 |
Office BP | ||||
Systolic (mmHg) | 165.0 ± 24.8 | 165.8 ± 24.5 | 162.4 ± 25.7 | 0.011 |
Diastolic (mmHg) | 89.4 ± 16.6 | 91.3 ± 16.1 | 83.3 ± 16.7 | <0.001 |
ABP | ||||
Systolic (mmHg) | 153.7 ± 18.1 | 153.7 ± 17.7 | 153.6 ± 19.0 | 0.916 |
Diastolic (mmHg) | 86.3 ± 14.0 | 87.8 ± 13.8 | 82.0 ± 13.6 | <0.001 |
eGFR (mL/min/1.73 m2) | 77.1 ± 24 | 86.6 ± 19 | 46.9 ± 10 | <0.001 |
Values are presented as mean ± SD unless stated otherwise.
A separate analysis examined patients with both baseline and 36-month BP data available. In general, baseline characteristics were similar to the larger cohort with the exception of a more balanced ratio of males and females and no differences in baseline office systolic BP or 24-h ambulatory systolic BP (Supplementary data, Table S1).
Effect of RDN on BP
For the overall population, as well as when stratified by the presence or absence of CKD, office and 24-h ABP were significantly reduced from baseline at all time points (12, 24 and 36 months; all P < 0.001). When adjusted for baseline BP, age and sex there was a numerically greater reduction in office systolic BP among patients without CKD compared with those with CKD, which reached statistical significance at 36 months (−17.3 ± 28.3 versus −11.7 ± 29.9 mmHg; P = 0.009). There was no significant difference in reductions in office diastolic BP between patients with or without CKD at any time point. There was also no difference in 24-h ambulatory systolic or diastolic BP reduction after RDN between groups at any time point, even after adjustment (Table 2).
BP change . | 1 year . | 2 years . | 3 Years . | |||||||||
---|---|---|---|---|---|---|---|---|---|---|---|---|
. | All . | CKD negative . | CKD positive . | P-valuea . | All . | CKD negative . | CKD positive . | P-valuea . | All . | CKD negative . | CKD positive . | P-valuea . |
Δ Office systolic BP (mmHg) | −13.0 ± 26.5 (n = 1441) | −14.4 ± 26.3 (n = 1112) | −8.5 ± 26.6 (n = 329) | 0.056 | −15.2 ± 27.3 (n = 1142) | −16.7 ± 26.9 (n = 879) | −10.2 ± 27.9 (n = 263) | 0.074 | −16.0 ± 28.8 (n = 967) | −17.3 ± 28.3 (n = 752) | −11.7 ± 29.9 (n = 215) | 0.009 |
Δ Ambulatory 24-h systolic BP (mmHg) | −7.6 ± 19.0 (n = 797) | −8.1 ± 19.0 (n = 613) | −6.0 ± 18.9 (n = 184) | 0.317 | −8.5 ± 19.7 (n = 551) | −8.9 ± 19.2 (n = 424) | −7.2 ± 21.2 (n = 127) | 0.405 | −8.7 ± 19.9 (n = 425) | −8.5 ± 20.0 (n = 324) | −9.2 ± 19.5 (n = 101) | 0.690 |
Δ Office diastolic BP (mmHg) | −5.0 ± 15.0 (n = 1440) | −5.7 ± 14.8 (n = 1112) | −2.7 ± 15.5 (n = 328) | 0.585 | −6.1 ± 16.1 (n = 1140) | −6.9 ± 15.9 (n = 877) | −3.3 ± 16.5 (n = 263) | 0.413 | −5.8 ± 16.3 (n = 963) | −6.6 ± 16.2 (n = 750) | −3.0 ± 16.3 (n = 213) | 0.771 |
Δ Ambulatory 24-h diastolic BP (mmHg) | −4.2 ± 11.6, (n = 798) | −4.4 ± 11.9 (n = 614) | −3.5 ± 10.4 (n = 184) | 0.104 | −4.6 ± 11.3 (n = 552) | −4.8 ± 11.4 (n = 425) | −4.0 ± 10.8 (n = 127) | 0.372 | −4.5 ± 12.1 (n = 426) | −4.6 ± 12.4 (n = 325) | −4.2 ± 11.2 (n = 101) | 0.239 |
BP change . | 1 year . | 2 years . | 3 Years . | |||||||||
---|---|---|---|---|---|---|---|---|---|---|---|---|
. | All . | CKD negative . | CKD positive . | P-valuea . | All . | CKD negative . | CKD positive . | P-valuea . | All . | CKD negative . | CKD positive . | P-valuea . |
Δ Office systolic BP (mmHg) | −13.0 ± 26.5 (n = 1441) | −14.4 ± 26.3 (n = 1112) | −8.5 ± 26.6 (n = 329) | 0.056 | −15.2 ± 27.3 (n = 1142) | −16.7 ± 26.9 (n = 879) | −10.2 ± 27.9 (n = 263) | 0.074 | −16.0 ± 28.8 (n = 967) | −17.3 ± 28.3 (n = 752) | −11.7 ± 29.9 (n = 215) | 0.009 |
Δ Ambulatory 24-h systolic BP (mmHg) | −7.6 ± 19.0 (n = 797) | −8.1 ± 19.0 (n = 613) | −6.0 ± 18.9 (n = 184) | 0.317 | −8.5 ± 19.7 (n = 551) | −8.9 ± 19.2 (n = 424) | −7.2 ± 21.2 (n = 127) | 0.405 | −8.7 ± 19.9 (n = 425) | −8.5 ± 20.0 (n = 324) | −9.2 ± 19.5 (n = 101) | 0.690 |
Δ Office diastolic BP (mmHg) | −5.0 ± 15.0 (n = 1440) | −5.7 ± 14.8 (n = 1112) | −2.7 ± 15.5 (n = 328) | 0.585 | −6.1 ± 16.1 (n = 1140) | −6.9 ± 15.9 (n = 877) | −3.3 ± 16.5 (n = 263) | 0.413 | −5.8 ± 16.3 (n = 963) | −6.6 ± 16.2 (n = 750) | −3.0 ± 16.3 (n = 213) | 0.771 |
Δ Ambulatory 24-h diastolic BP (mmHg) | −4.2 ± 11.6, (n = 798) | −4.4 ± 11.9 (n = 614) | −3.5 ± 10.4 (n = 184) | 0.104 | −4.6 ± 11.3 (n = 552) | −4.8 ± 11.4 (n = 425) | −4.0 ± 10.8 (n = 127) | 0.372 | −4.5 ± 12.1 (n = 426) | −4.6 ± 12.4 (n = 325) | −4.2 ± 11.2 (n = 101) | 0.239 |
No CKD versus CKD, adjusted for baseline BP (office or 24-h ambulatory), age, gender. Values are presented as mean ± SD.
BP change . | 1 year . | 2 years . | 3 Years . | |||||||||
---|---|---|---|---|---|---|---|---|---|---|---|---|
. | All . | CKD negative . | CKD positive . | P-valuea . | All . | CKD negative . | CKD positive . | P-valuea . | All . | CKD negative . | CKD positive . | P-valuea . |
Δ Office systolic BP (mmHg) | −13.0 ± 26.5 (n = 1441) | −14.4 ± 26.3 (n = 1112) | −8.5 ± 26.6 (n = 329) | 0.056 | −15.2 ± 27.3 (n = 1142) | −16.7 ± 26.9 (n = 879) | −10.2 ± 27.9 (n = 263) | 0.074 | −16.0 ± 28.8 (n = 967) | −17.3 ± 28.3 (n = 752) | −11.7 ± 29.9 (n = 215) | 0.009 |
Δ Ambulatory 24-h systolic BP (mmHg) | −7.6 ± 19.0 (n = 797) | −8.1 ± 19.0 (n = 613) | −6.0 ± 18.9 (n = 184) | 0.317 | −8.5 ± 19.7 (n = 551) | −8.9 ± 19.2 (n = 424) | −7.2 ± 21.2 (n = 127) | 0.405 | −8.7 ± 19.9 (n = 425) | −8.5 ± 20.0 (n = 324) | −9.2 ± 19.5 (n = 101) | 0.690 |
Δ Office diastolic BP (mmHg) | −5.0 ± 15.0 (n = 1440) | −5.7 ± 14.8 (n = 1112) | −2.7 ± 15.5 (n = 328) | 0.585 | −6.1 ± 16.1 (n = 1140) | −6.9 ± 15.9 (n = 877) | −3.3 ± 16.5 (n = 263) | 0.413 | −5.8 ± 16.3 (n = 963) | −6.6 ± 16.2 (n = 750) | −3.0 ± 16.3 (n = 213) | 0.771 |
Δ Ambulatory 24-h diastolic BP (mmHg) | −4.2 ± 11.6, (n = 798) | −4.4 ± 11.9 (n = 614) | −3.5 ± 10.4 (n = 184) | 0.104 | −4.6 ± 11.3 (n = 552) | −4.8 ± 11.4 (n = 425) | −4.0 ± 10.8 (n = 127) | 0.372 | −4.5 ± 12.1 (n = 426) | −4.6 ± 12.4 (n = 325) | −4.2 ± 11.2 (n = 101) | 0.239 |
BP change . | 1 year . | 2 years . | 3 Years . | |||||||||
---|---|---|---|---|---|---|---|---|---|---|---|---|
. | All . | CKD negative . | CKD positive . | P-valuea . | All . | CKD negative . | CKD positive . | P-valuea . | All . | CKD negative . | CKD positive . | P-valuea . |
Δ Office systolic BP (mmHg) | −13.0 ± 26.5 (n = 1441) | −14.4 ± 26.3 (n = 1112) | −8.5 ± 26.6 (n = 329) | 0.056 | −15.2 ± 27.3 (n = 1142) | −16.7 ± 26.9 (n = 879) | −10.2 ± 27.9 (n = 263) | 0.074 | −16.0 ± 28.8 (n = 967) | −17.3 ± 28.3 (n = 752) | −11.7 ± 29.9 (n = 215) | 0.009 |
Δ Ambulatory 24-h systolic BP (mmHg) | −7.6 ± 19.0 (n = 797) | −8.1 ± 19.0 (n = 613) | −6.0 ± 18.9 (n = 184) | 0.317 | −8.5 ± 19.7 (n = 551) | −8.9 ± 19.2 (n = 424) | −7.2 ± 21.2 (n = 127) | 0.405 | −8.7 ± 19.9 (n = 425) | −8.5 ± 20.0 (n = 324) | −9.2 ± 19.5 (n = 101) | 0.690 |
Δ Office diastolic BP (mmHg) | −5.0 ± 15.0 (n = 1440) | −5.7 ± 14.8 (n = 1112) | −2.7 ± 15.5 (n = 328) | 0.585 | −6.1 ± 16.1 (n = 1140) | −6.9 ± 15.9 (n = 877) | −3.3 ± 16.5 (n = 263) | 0.413 | −5.8 ± 16.3 (n = 963) | −6.6 ± 16.2 (n = 750) | −3.0 ± 16.3 (n = 213) | 0.771 |
Δ Ambulatory 24-h diastolic BP (mmHg) | −4.2 ± 11.6, (n = 798) | −4.4 ± 11.9 (n = 614) | −3.5 ± 10.4 (n = 184) | 0.104 | −4.6 ± 11.3 (n = 552) | −4.8 ± 11.4 (n = 425) | −4.0 ± 10.8 (n = 127) | 0.372 | −4.5 ± 12.1 (n = 426) | −4.6 ± 12.4 (n = 325) | −4.2 ± 11.2 (n = 101) | 0.239 |
No CKD versus CKD, adjusted for baseline BP (office or 24-h ambulatory), age, gender. Values are presented as mean ± SD.
Results were similar when the analysis was limited to patients with both baseline and 36-month BP data available. Patients without CKD had greater reductions in office systolic BP at 12 and 36 months compared with those with CKD. There were no differences in reductions in office diastolic BP at any time point between patients with or without CKD and no differences in 24-h ambulatory systolic or diastolic BP reductions between groups at any time point, even after adjustment (Supplementary data, Table S2).
Renal function
Patients without CKD had a steeper decline in eGFR between baseline and 1 year compared with those with CKD (−5.81 ± 16 versus −0.44 ± 12 mL/min/1.73 m2; P < 0.001). After the first year, eGFR decline/year was not significantly different between groups (from 1 to 2 years: −0.51 ± 14 without CKD versus −1.85 ± 11 mL/min/1.73 m2 with CKD, P = 0.135; from 2 to 3 years: −1.56 ± 15 without CKD versus −1.64 ± 9.2 mL/min/1.73 m2 with CKD, P = 0.703).
Results were similar when the analysis was limited to patients with both baseline and 36-month BP data available (from baseline to 1 year: −6.28 ± 16 without CKD versus −0.79 ± 13 mL/min/1.73 m2 with CKD, P = 0.0003; from 1 to 2 years: 0.3 ± 14 without CKD versus −1.9 ± 12 mL/min/1.73 m2 with CKD, P = 0.074; from 2 to 3 years: −2.1 ± 14 without CKD versus −1.7 ± 9.4 mL/min/1.73 m2 without CKD, P = 0.467).
Safety
Safety outcomes are reported as percentages and shown in Figure 1. Patients with CKD had higher rates of cardiovascular death at 36 months (5.3% versus 2.4%; P = 0.007) compared with those without CKD, although the rate of non-cardiovascular death (2.1% versus 1.5%; P = 0.445) was similar between groups. In accordance, major adverse events and stroke, but not myocardial infarction, were documented more often in patients with CKD compared with those without. Serum creatinine elevation (>50%) and new-onset end-stage renal disease were more pronounced in patients with CKD compared with those without. There was no difference in new artery stenosis (>70% diameter), renal artery reintervention or other vascular complications between groups.
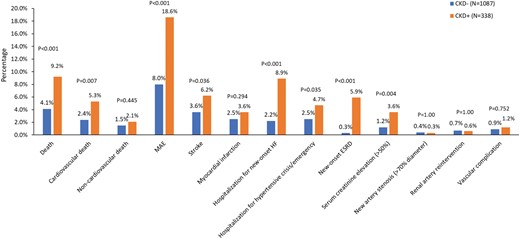
Safety events at the 3-year follow-up. Data are in percentages. MAE, major adverse event (defined as any of the following events: death, new-onset end-stage renal disease, significant embolic event resulting in end-organ damage, renal artery reintervention due to perforation or dissection, vascular complications, hospitalization for hypertensive crisis/hypertensive emergency or new renal artery stenosis >70%); HF, heart failure; ESRD, end-stage renal disease.
DISCUSSION
To our knowledge, this retrospective GSR analysis has provided the largest set of data on the efficacy and safety of RDN in patients with versus without CKD. Findings showed that RDN was followed by significant reductions in office and 24-h ABP sustained up to 3 years regardless of the presence or absence of CKD. However, there were greater reductions in office systolic, but not diastolic, BP in patients without CKD compared with those with CKD, while reductions in 24-h ABP were similar between groups. In addition, the analyses were adjusted for baseline office and 24-h ABP, thus eliminating any confounding from differences in initial BP values for patients with or without CKD [21]. The results of this large retrospective GSR analysis are consistent with prospective, albeit small and preliminary, studies of patients with CKD and contribute to the body of evidence showing that RDN can lead to a clinically meaningful reduction in office and ABP in this patient population, including those with end-stage renal disease [9, 10, 22, 23].
Patients in registries often do not complete the full follow-up schedule, whether due to dropout or death. To address this limitation, an additional analysis was performed that included only patients who had evaluable BP data at baseline and 36 months. In doing so, available data were reduced to nearly half (office BP data cut to 48.8%, 24-h ABP data cut to 46.4%). The results of this analysis showed the same pattern of reduced office systolic, but not diastolic, BP in both groups with no differences in 24-h ABP reduction between groups. This shows that the BP-lowering effects of RDN treatment are consistent among the analysis populations, from an all-comer group that includes patients who died or were lost to follow-up before the final visit to a more strictly defined group limited to patients with both baseline and 36-month BP data available.
One unique and critical feature of the retrospective GSR analysis was the separate assessment of diastolic BP, which has been shown to have an independent effect on cardiovascular outcomes in patients with hypertension. The analysis showed that all patients had significant reductions in office diastolic BP between baseline and 3 years, but that BP reductions were similar for patients without CKD versus those with CKD. This is significant because, while the control of systolic BP has always been well established as a means to manage cardiovascular outcomes, the control of diastolic BP has only recently come into focus. A retrospective study of outcomes from the large ONTARGET and TRANSCEND trials of antihypertensive medications showed that patients who had diastolic BP outside of the target range were at higher risk of cardiovascular events, including stroke, myocardial infarction and hospitalization for heart failure [24]. These findings were supported by a separate study of 1.3 million patients from a large integrated health system that showed diastolic BP was independently associated with increased risk of adverse cardiovascular outcomes, including myocardial infarction, ischaemic stroke and haemorrhagic stroke [25]. The role of diastolic BP in patient outcomes should be further explored and future studies on the treatment or mechanisms of hypertension should include full assessments of both systolic and diastolic BP.
The proper assessment and management of hypertension should be based on out-of-office BP measurement, including ABP monitoring. This is especially true for patients with CKD, who have different ABP patterns compared with those without CKD and BP components that progressively deteriorate over the course of disease [26, 27]. The importance of using ABP is highlighted by data from the Spanish ABPM registry, which showed that one of three hypertensive CKD patients were misclassified when assessed with office BP alone [28]. In addition, a prospective study of 436 patients with CKD Stages 2–4 and a median follow-up of 4.2 years showed that ABP is a better tool for predicting cardiovascular and renal risk compared with office BP [29]. It must be kept in mind that BP control is of crucial importance in preventing both CKD progression and associated cardiovascular complications, but in patients with CKD it might be more difficult to control BP values. In the Masterplan trial, there were 788 patients with CKD under nephrologist care and more than a third still had treatment-resistant hypertension even with a multifactorial intervention. The presence of apparent treatment-resistant hypertension was related to an increased risk of cardiovascular events and renal dysfunction [30]. In a study of 1116 antihypertensive drug–naïve patients with CKD, linear regression analyses identified age as a main factor associated with differences in clinical and ABP [31]. Notably, our results were adjusted for age, ruling this out as a major confounding effect.
A previously published analysis of the GSR has reported first insights into renal function up to 3 years after treatment with RDN for all patients and places the current retrospective data into context [32]. While the retrospective analysis showed that patients without CKD had greater reductions in eGFR during the first year compared with those with CKD, this is likely due to ‘regression-to-the-mean’ at this single time point. For all other intervals after the first year there were no differences in annual eGFR decline between groups and the overall rate of decline between baseline and 3 years was consistent with what has been reported for patients with CKD and hypertension [32].
Several research groups have even reported that RDN may prevent the further decline of renal function and Kiuchi et al. [33, 34] observed that RDN in patients with CKD Stages 2–4 resulted in a progressive increase in eGFR (30% at 6 months, 39% at 12 months and 42% at 24 months, from 61.9 ± 24 to 88.0 ± 40 mL/min/1.73 m2). However, it is beyond the scope of a registry to derive any conclusion on the efficacy of RDN on the progression of renal function.
RDN can be technically challenging in patients with advanced CKD and the first priority is to ‘do no harm’ during the procedure [22, 23]. In a thorough analysis by Townsend et al. [35], 26 patients with renal artery stenosis or dissection (0.45%) were identified, of whom 24 (0.41%) required renal artery stenting. The primary meta-analysis of all reports indicated a 0.20% pooled annual incidence rate of stent implantation [95% confidence interval (CI) 0.12–0.29% per year]. Thus, overall, the currently reported percentage is in the same range and does not suggest an increased rate of renal artery stenosis. The GSR was designed primarily as a safety study and this retrospective analysis showed no differences in safety outcomes between patients with versus without CKD. This is a critical contribution to the safety profile of RDN, supporting the safety of the procedure regardless of CKD. Nevertheless, data indicate an increased cardiovascular risk in patients with CKD compared with those without.
There are several limitations. The GSR includes a heterogeneous population, since enrollment is based on uncontrolled hypertension and other conditions associated with sympathetic nervous system activation are allowed. Moreover, as is common in registries, patients are lost to follow-up. However, the results of our analysis that was limited to patients with both baseline and 36-month BP data available corroborated our findings, because we thereby could exclude any selection bias by different patient populations at baseline and last follow up, a bias that is found too often in the literature. Typical of an all-comer registry study, there is no comparison to a sham or other control group. In these types of retrospective analyses, control for adherence and/or medication and changes is rather difficult. Findings need to be supported by a rigorously conducted, prospective, double-blind, sham-controlled study.
SUPPLEMENTARY DATA
Supplementary data are available at ndt online.
ACKNOWLEDGEMENTS
The authors thank Marianne Wanten and Robin Hermans for study management, Minglei Liu for statistical support, Jessica Dries-Devlin for editorial support and Vanessa DeBruin, Sandeep Brar and Sidney A. Cohen for study support and expert technical review (all from Medtronic). Zachary Harrelson also provided editorial support.
FUNDING
The GSR is funded by Medtronic.
AUTHORS’ CONTRIBUTIONS
C.O. and R.E.S. analysed the data, wrote the manuscript and contributed to the discussion. F.M., G.M., K.N., L.M.R., M.P.S. and M.B. contributed to the discussion and reviewed the manuscript. M.F. analysed the data. All authors approved the final version of the manuscript.
CONFLICT OF INTEREST STATEMENT
C.O. and L.M.R. declared no conflicts. F.M. reports grants from Deutsche Gesellschaft für Kardiologie and Deutsche Forschungsgemeinschaft (SFB TRR219) and grants and personal fees from Bayer, Boehringer Ingelheim, Medtronic and ReCor Medical, outside the submitted work. G.M. reports personal fees from AstraaZeneca, Boehringer Ingelheim, Daiichi Sankyo, Medtronic, Menarini, Novartis Pharma, Neopharmed Gentili, Recordati, Sandoz, Sanofi and Servier, outside the submitted work. K.N. reports personal fees from Berlin-Chemie/Menarini, Egis, Idorsia, Gedeon Richter, Krka, Medtronic, Merck, Polpharma, Recordati and Servier, outside the submitted work. M.F. is an employee of Medtronic. M.P.S. reports grants and personal fees from Medtronic during the conduct of the study. M.B. reports personal fees from Abbott, Amgen, AstraZeneca, Bayer, Boehringer Ingelheim, Bristol Myers Squibb, Medtronic, Novartis, Servier and Vifor, outside the submitted work. R.E.S. reports grants and personal fees from Medtronic during the conduct of the study and grants and personal fees from Medtronic, outside the submitted work.
DATA AVAILABILITY STATEMENT
Data are available upon request to the Steering Commitee of the Global SYMPLICITY Registry (chairperson: Prof. Dr. M. Böhm).
Comments