-
PDF
- Split View
-
Views
-
Cite
Cite
Fang Fu, Feifei Chen, Ru Li, Yongling Zhang, Min Pan, Dongzhi Li, Can Liao, Prenatal diagnosis of fetal multicystic dysplastic kidney via high-resolution whole-genome array, Nephrology Dialysis Transplantation, Volume 31, Issue 10, October 2016, Pages 1693–1698, https://doi.org/10.1093/ndt/gfv465
- Share Icon Share
Women with fetal multicystic dysplastic kidneys (MCDK) are commonly referred for genetic counseling, for which identification of the correct etiology is a prerequisite.
A total of 72 women with fetal MCDK at Guangzhou Women and Children's Medical Center were examined via invasive prenatal diagnosis from May 2010 to June 2015. Standard karyotyping analysis was provided to all fetuses, and chromosomal microarray with Affymetrix CytoSan HD arrays was offered to cases whose DNA samples were available.
Abnormal karyotypes were detected in 3 of 72 (4.17%) fetuses. Of the 69 (95.8%, 69/72) fetuses with normal karyotypes, 30 (42%, 30/69) underwent chromosome microarray analysis (CMA) testing. The CMA identified pathogenic copy number variations in five fetuses, leading to a pathogenic detection rate of 16.7% (5/30). Well-known microdeletion or microduplication syndromes including renal cysts and diabetes (RCAD) syndrome and Williams–Beuren syndrome (WBS) were identified in three cases. Moreover, four chromosomal imbalanced regions were also identified in our MCDK fetuses: 22q11.1 duplication, 4q35.2 deletion, 22q13.33 duplication and 1p33 duplication. Genes PEX26, ELN, HNF1B, ALG12, FRG1, FRG2 and CYP4A11 were possible candidates for fetal MCDK. The proportions of variants of unknown significance before and after parental analysis were 13.3% (4/30) and 3.3% (1/30), respectively.
In the present study, the frequency of chromosomal abnormalities in MCDK fetuses was 4.17% and all rearrangements were imbalanced aberrations. CMA was able to increase the pathogenic detection rate to 16.7% in MCDK fetuses with normal karyotype. Critical regions for RCAD syndrome, WBS and copy number variants of 22q11.1 duplication, 4q35.2 deletion, 22q13.33 duplication and 1p33 duplication were associated with fetal MCDK. Genes PEX26, ELN, HNF1B, ALG12, FRG1, FRG2 and CYP4A11 were possible candidates for fetal MCDK.
INTRODUCTION
Multicystic dysplastic kidney (MCDK) is a form of renal cystic disease characterized by multiple, non-functioning, non-communicating cysts that replace the normal renal parenchyma resulting from the failed coordination of the metanephros and branching ureteric bud, which leads to renal dysfunction. MCDK has an incidence of 1 in 4300 live births, and 94.1% of all cases are detected using antenatal ultrasound [1]. Up to 39% of patients present with associated anomalies in the contralateral kidney [2]. Bilateral multicystic kidneys have a poor prognosis. Aslam and Watson reported that 41% of patients with unilateral MCDK had renal remnants at 10 years and an impaired glomerular filtration rate (GFR) [3].
Women with fetal MCDK are commonly referred to genetic counseling, for which the identification of the correct etiology is a prerequisite. Over the past few years, chromosome microarray analysis (CMA) has been applied in clinical genetics. This technology can detect submicroscopic copy number variations (CNVs), and the literature has shown that it can help to improve the detection rate in 15% of patients with developmental delays and multiple congenital anomalies [4]. CMA is the first-tier clinical diagnostic test for patients with congenital anomalies, mental retardation or both [5]. Recently, a few studies have also established the relevance of CNVs in renal tract disorders. Verbitsky et al. [6] reported a study that enrolled 419 children with chronic kidney disease (CKD), in which the detection rate of a known genomic disorder was 4.5% (19/419). Caruana et al. [7] screened 178 children presenting with a wide spectrum of structural anomalies associated with congenital anomalies of the kidney and urinary tract (CAKUT) using single nucleotide polymorphism microarrays, and identified seven patients (7/178, 3.9%) with pathogenic CNVs. Sanna-Cherchi et al. [8] identified 16.6% (87/522) individuals with kidney malformations who had a pathogenic copy number variant (10.5% with known disorders and 6.1% with rare or novel disorders). The molecular pathogenesis of MCDK is also beginning to be unraveled. Body-Bechou et al. and others have reported that the HNF1B and UPIIIA [9–12] genes are associated with MCDK in humans. However, all the patients reported were postnatal and the detection yield in prenatal cases remains unknown. The current study marks the first systematic assessment of submicroscopic imbalances during the prenatal diagnoses of fetuses with MCDK using whole-genome, high-resolution CMA.
MATERIALS AND METHODS
Patients
From May 2010 to June 2015, 72 pregnant women were consecutively referred to the Prenatal Diagnostic Center at Guangzhou Women and Children's Medical Center for an invasive prenatal diagnostic test because a prenatal ultrasound scan revealed that their fetus had MCDK. Depending on the gestational age (range = 16–35 gestational weeks, median = 26 gestational weeks), fetal samples were obtained using amniocentesis (n = 27), chorionic villus sampling (n = 1) or cord blood sampling (n = 44). A G-banding karyotype analysis was first performed in all 72 samples. Fetuses with normal karyotype results were included in the array test. Because not all pregnant women agreed to participate, finally 30 fetal samples were used for the CMA.

Renal ultrasound scans of Fetus 7 at 25 weeks. This sagittal scan through a multicystic dysplastic kidney reveals multiple cysts.
CMA
CMA was performed using CytoScan HD Array (Affymetrix, Santa Clara, CA, USA) according to the manufacturer's instructions, and the reporting threshold of the CNVs was set at 100 kb with a marker count of ≥50. The CNVs detected were aligned with known CNVs listed in databases such as the database of genomic variants (DGV, http://dgv.tcag.ca/dgv/app/home), DECIPHER (https://decipher.sanger.ac.uk/), OMIM (http://www.omim.org), UCSC (http://genome.ucsc.edu/) and ISCA (https://www.iscaconsortium.org/). According to the literature [13], the CNVs identified using CMA were classified as pathogenic CNVs, VOUS or benign CNVs. To identify possible candidate genes within the altered chromosomal regions, disease-associated analysis and biological analysis were performed.
Quantitative real-time PCR
DNA was used to verify the pathogenic CNVs detected using CMA via real-time fluorescent quantitative PCR techniques based on standard protocols.
Clinical follow-up assessment
Clinical follow-up assessments were scheduled 3 months to 4 years after the birth via telephone and performed via postpartum ultrasound.
RESULTS
From May 2010 to June 2015, 13 880 pregnant women received an invasive prenatal diagnostic test at our center. Exactly 370 of their fetuses (2.7%) had CAKUT, of which 72 fetuses (19.5%) were diagnosed with MCDK using prenatal ultrasound and included in this study.
Sample ID . | Gestational age (in weeks) . | Clinical feature . | Karyotype . | CMA result . | Genes . |
---|---|---|---|---|---|
Fetus 6 | 24 | Bilateral MCDK | 46,XX,der(13)t(1;13)(q21;p12) mat | Dup1q24.2q44 (170271054–249224684); 79 Mb | – |
Fetus 7 | 25 | Right MCDK + VSD | 46,XX,der(21) | Dup22q11.1 (16888899–18916961); 2.03 Mb | PEX26 |
Fetus 70 | 25 | Right MCDK | 48,XY,+2 mar[1]/47,XY,+mar[12]/46, XY [3] | Dup6p22.3 (155568240–15863387); 307 Kb | DTNBP1 |
Sample ID . | Gestational age (in weeks) . | Clinical feature . | Karyotype . | CMA result . | Genes . |
---|---|---|---|---|---|
Fetus 6 | 24 | Bilateral MCDK | 46,XX,der(13)t(1;13)(q21;p12) mat | Dup1q24.2q44 (170271054–249224684); 79 Mb | – |
Fetus 7 | 25 | Right MCDK + VSD | 46,XX,der(21) | Dup22q11.1 (16888899–18916961); 2.03 Mb | PEX26 |
Fetus 70 | 25 | Right MCDK | 48,XY,+2 mar[1]/47,XY,+mar[12]/46, XY [3] | Dup6p22.3 (155568240–15863387); 307 Kb | DTNBP1 |
VSD, ventricular septal defect.
Sample ID . | Gestational age (in weeks) . | Clinical feature . | Karyotype . | CMA result . | Genes . |
---|---|---|---|---|---|
Fetus 6 | 24 | Bilateral MCDK | 46,XX,der(13)t(1;13)(q21;p12) mat | Dup1q24.2q44 (170271054–249224684); 79 Mb | – |
Fetus 7 | 25 | Right MCDK + VSD | 46,XX,der(21) | Dup22q11.1 (16888899–18916961); 2.03 Mb | PEX26 |
Fetus 70 | 25 | Right MCDK | 48,XY,+2 mar[1]/47,XY,+mar[12]/46, XY [3] | Dup6p22.3 (155568240–15863387); 307 Kb | DTNBP1 |
Sample ID . | Gestational age (in weeks) . | Clinical feature . | Karyotype . | CMA result . | Genes . |
---|---|---|---|---|---|
Fetus 6 | 24 | Bilateral MCDK | 46,XX,der(13)t(1;13)(q21;p12) mat | Dup1q24.2q44 (170271054–249224684); 79 Mb | – |
Fetus 7 | 25 | Right MCDK + VSD | 46,XX,der(21) | Dup22q11.1 (16888899–18916961); 2.03 Mb | PEX26 |
Fetus 70 | 25 | Right MCDK | 48,XY,+2 mar[1]/47,XY,+mar[12]/46, XY [3] | Dup6p22.3 (155568240–15863387); 307 Kb | DTNBP1 |
VSD, ventricular septal defect.
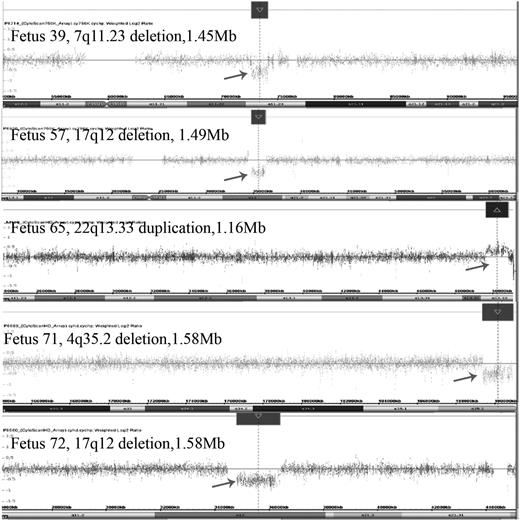
The pathogenic CMA results of our five MCDK fetuses. Fetus 39: CMA revealed a 1.45-Mb deletion spanning genomic position 72701098–74154209 (hg19) at the chromosome 7q11.23 region; Fetus 57: CMA showed a 1.49-Mb deletion spanning genomic position 34822465–36316144 (hg19) at the chromosome 17q12 region; Fetus 65: CMA demonstrated a 1.16-Mb duplication spanning genomic position 49654561–50817492 (hg19) at the chromosome 17q12 region; Fetus 71: CMA detected a 1.57-Mb deletion at the 4q35.2 region (chromosome position: 189380220–19095747, hg19); Fetus 72: CMA revealed a 1.58-Mb deletion at the 17q12 region (chromosome position: 34822465–36404555, hg19).
CMA was applied in the case of 30 fetuses. (i) Five CNVs (5/30, 16.7%) in 30 fetuses were classified as pathogenic, overlapping with either the DECIPHER entry or the known syndrome (Table 2). Of the 30 fetuses, three cases of microdeletion and microduplication syndromes were detected: renal cyst and diabetes syndrome (RCAD; Fetuses 57 and 72) and Williams–Beuren syndrome (WBS; Fetus 39). Moreover, four chromosomal imbalanced regions were also identified in our MCDK fetuses: 22q11.1, 4q35.2, 22q13.33 and 1p33. The genes PEX26, ELN, HNF1B, ALG12, FRG1, FRG2 and CYP4A11 were possible candidate genes for fetal MCDK. (ii) Benign CNVs were detected in 24 fetuses (24/30, 80%). The proportions of VOUS were 13.3% (4/30) and 3.3% (1/30) before and after parental analysis, respectively. (iii) The pathogenic CNV detection rates in the isolated MCDK group were 15% (3/20) [1/11 in unilateral MCDK and 2/9 in bilateral MCDK], while in the group with MCDK plus extra renal abnormalities it was 20% (2/10). However, the sample size was too small to make the assertion whether there was a difference between the two groups.
Case no. . | Clinical feature . | Copy number . | Cytoband . | Chromosome physical location (hg19) . | Size (kb) . | Decipher patient/known syndrome . | OMIM morbid gene . |
---|---|---|---|---|---|---|---|
Fetus 39 | MCDK plus FGR | Loss | 7q11.23 | 72701098–74154209 | 1450 | WBS | ELN |
Fetus 57 | Bilateral MCDK plus polyhydramnios | Loss | 17q12 | 34822465–36316144 | 1490 | RCAD | HNF1B |
Fetus 65 | MCDK plus spina bifida and ventriculomegaly | Gain | 22q13.33 | 49654561– 50817492 | 1163 | 283243 | ALG12 |
Fetus 71 | Bilateral MCDK without amniotic fluid | Loss | 4q35.2 | 189380220–190957473 | 1577 | 268249 | FRG1, FRG2 |
Fetus 72 | Right MCDK | Loss | 17q12 | 34822465–36404555 | 1580 | RCAD | HNF1B |
Fetus 36 | Left MCDK | Gain | 1p33 | 47365097–47620957 | 256 | VOUS | CYP4A11 |
Case no. . | Clinical feature . | Copy number . | Cytoband . | Chromosome physical location (hg19) . | Size (kb) . | Decipher patient/known syndrome . | OMIM morbid gene . |
---|---|---|---|---|---|---|---|
Fetus 39 | MCDK plus FGR | Loss | 7q11.23 | 72701098–74154209 | 1450 | WBS | ELN |
Fetus 57 | Bilateral MCDK plus polyhydramnios | Loss | 17q12 | 34822465–36316144 | 1490 | RCAD | HNF1B |
Fetus 65 | MCDK plus spina bifida and ventriculomegaly | Gain | 22q13.33 | 49654561– 50817492 | 1163 | 283243 | ALG12 |
Fetus 71 | Bilateral MCDK without amniotic fluid | Loss | 4q35.2 | 189380220–190957473 | 1577 | 268249 | FRG1, FRG2 |
Fetus 72 | Right MCDK | Loss | 17q12 | 34822465–36404555 | 1580 | RCAD | HNF1B |
Fetus 36 | Left MCDK | Gain | 1p33 | 47365097–47620957 | 256 | VOUS | CYP4A11 |
WBS, Williams–Beuren syndrome; RCAD, renal cysts and diabetes; FGR, fetal growth restriction; VOUS, variants of unknown significance; FRG1, facioscapulohumeral muscular dystrophy (FSHD) region gene-1; FRG2, FSHD region gene-2.
Case no. . | Clinical feature . | Copy number . | Cytoband . | Chromosome physical location (hg19) . | Size (kb) . | Decipher patient/known syndrome . | OMIM morbid gene . |
---|---|---|---|---|---|---|---|
Fetus 39 | MCDK plus FGR | Loss | 7q11.23 | 72701098–74154209 | 1450 | WBS | ELN |
Fetus 57 | Bilateral MCDK plus polyhydramnios | Loss | 17q12 | 34822465–36316144 | 1490 | RCAD | HNF1B |
Fetus 65 | MCDK plus spina bifida and ventriculomegaly | Gain | 22q13.33 | 49654561– 50817492 | 1163 | 283243 | ALG12 |
Fetus 71 | Bilateral MCDK without amniotic fluid | Loss | 4q35.2 | 189380220–190957473 | 1577 | 268249 | FRG1, FRG2 |
Fetus 72 | Right MCDK | Loss | 17q12 | 34822465–36404555 | 1580 | RCAD | HNF1B |
Fetus 36 | Left MCDK | Gain | 1p33 | 47365097–47620957 | 256 | VOUS | CYP4A11 |
Case no. . | Clinical feature . | Copy number . | Cytoband . | Chromosome physical location (hg19) . | Size (kb) . | Decipher patient/known syndrome . | OMIM morbid gene . |
---|---|---|---|---|---|---|---|
Fetus 39 | MCDK plus FGR | Loss | 7q11.23 | 72701098–74154209 | 1450 | WBS | ELN |
Fetus 57 | Bilateral MCDK plus polyhydramnios | Loss | 17q12 | 34822465–36316144 | 1490 | RCAD | HNF1B |
Fetus 65 | MCDK plus spina bifida and ventriculomegaly | Gain | 22q13.33 | 49654561– 50817492 | 1163 | 283243 | ALG12 |
Fetus 71 | Bilateral MCDK without amniotic fluid | Loss | 4q35.2 | 189380220–190957473 | 1577 | 268249 | FRG1, FRG2 |
Fetus 72 | Right MCDK | Loss | 17q12 | 34822465–36404555 | 1580 | RCAD | HNF1B |
Fetus 36 | Left MCDK | Gain | 1p33 | 47365097–47620957 | 256 | VOUS | CYP4A11 |
WBS, Williams–Beuren syndrome; RCAD, renal cysts and diabetes; FGR, fetal growth restriction; VOUS, variants of unknown significance; FRG1, facioscapulohumeral muscular dystrophy (FSHD) region gene-1; FRG2, FSHD region gene-2.
The clinical follow-up assessments after prenatal diagnosis of the fetuses with MCDK in this study are summarized in Table 3.
Clinical follow-up assessment of fetuses with MCDK after prenatal diagnosis
. | Karyotype analysis (N = 72) . | CMA (N = 30) . | |||
---|---|---|---|---|---|
Normal (n = 69) . | Abnormal (n = 3) . | Benign CNVs (n = 24) . | VOUS (n = 1) . | Pathogenic CNVs (n = 5) . | |
Labor | 39 (56.5%) | – | 10 (41.7%) | 1 (100%) | – |
TOP | 20 (29%) | 3 (100%) | 10 (41.7%) | – | 5 (100%) |
Premature | 2 (2.9%) | – | 1 (4.16%) | – | – |
Birth with other defects | 2 (2.9%) | – | 1 (4.16%) | – | – |
Loss to follow-up | 6 (8.7%) | – | 2 (8.33%) | – | – |
. | Karyotype analysis (N = 72) . | CMA (N = 30) . | |||
---|---|---|---|---|---|
Normal (n = 69) . | Abnormal (n = 3) . | Benign CNVs (n = 24) . | VOUS (n = 1) . | Pathogenic CNVs (n = 5) . | |
Labor | 39 (56.5%) | – | 10 (41.7%) | 1 (100%) | – |
TOP | 20 (29%) | 3 (100%) | 10 (41.7%) | – | 5 (100%) |
Premature | 2 (2.9%) | – | 1 (4.16%) | – | – |
Birth with other defects | 2 (2.9%) | – | 1 (4.16%) | – | – |
Loss to follow-up | 6 (8.7%) | – | 2 (8.33%) | – | – |
TOP, termination of pregnancy.
Clinical follow-up assessment of fetuses with MCDK after prenatal diagnosis
. | Karyotype analysis (N = 72) . | CMA (N = 30) . | |||
---|---|---|---|---|---|
Normal (n = 69) . | Abnormal (n = 3) . | Benign CNVs (n = 24) . | VOUS (n = 1) . | Pathogenic CNVs (n = 5) . | |
Labor | 39 (56.5%) | – | 10 (41.7%) | 1 (100%) | – |
TOP | 20 (29%) | 3 (100%) | 10 (41.7%) | – | 5 (100%) |
Premature | 2 (2.9%) | – | 1 (4.16%) | – | – |
Birth with other defects | 2 (2.9%) | – | 1 (4.16%) | – | – |
Loss to follow-up | 6 (8.7%) | – | 2 (8.33%) | – | – |
. | Karyotype analysis (N = 72) . | CMA (N = 30) . | |||
---|---|---|---|---|---|
Normal (n = 69) . | Abnormal (n = 3) . | Benign CNVs (n = 24) . | VOUS (n = 1) . | Pathogenic CNVs (n = 5) . | |
Labor | 39 (56.5%) | – | 10 (41.7%) | 1 (100%) | – |
TOP | 20 (29%) | 3 (100%) | 10 (41.7%) | – | 5 (100%) |
Premature | 2 (2.9%) | – | 1 (4.16%) | – | – |
Birth with other defects | 2 (2.9%) | – | 1 (4.16%) | – | – |
Loss to follow-up | 6 (8.7%) | – | 2 (8.33%) | – | – |
TOP, termination of pregnancy.
DISCUSSION
Applying CMA for fetuses with abnormal karyotypes and MCDK
In the past, many researchers established relationships between chromosomal abnormalities and the etiology of certain fetal renal defects [14–16]. In 2005, Staebler et al. demonstrated that isolated MCKD showed a low risk of chromosomal abnormality (0%, 0/9) [17]. In 2012, Hsu et al. studied 14 cases of MDCK, and found that only one fetus presented with balanced translocation [t(12,13)(p13:q21.2)] [14]. Our study drew the same conclusion: we performed a karyotype analysis of 72 fetuses with MCDK, and identified only three fetuses (4.17%) with derivative chromosome abnormities (46,XX,der[1;13][q21;p12]mat [Fetus 6], 46,XX,der[21][Fetus 7] and 48,XY,+2mar[1]/47,XY,+mar[12]/46,XY [3] [Fetus 70]). Fetuses 7 and 70 were also tested using CMA. The results revealed a 407-kb microduplication at the 6p22.3 region and a 2.03-Mb microduplication at the 22q11.1 region. According to the DECIPHER database, the 22q11.1 microduplication produces pathogenic CNVs with phenotypes of growth retardation, congenital heart disease and renal malformations. Thirteen OMIM genes are contained in this region, including the PEX26 gene. The PEX26 gene is the most highly expressed in kidney tissue and mutation in PEX26 can cause peroxisome biogenesis disorder 7A (Zellweger syndrome; OMIM 614872). Symptoms of these disorders include an enlarged liver, neurological abnormalities, characteristic facial features and renal cysts [18]. Thus, the PEX26 gene might be responsible for the observed phenotype in Fetus 7.
No reports have shown the same aberrations related to MCDK, and no specific syndrome or triploid has been detected using a karyotype analysis. In contrast, all three of these unbalanced aberrations in our study can be detected using CMA. CMA likely not only determines the source, size and nature of the fragments found via the G-banding karyotype analysis but also detects the microdeletion/microduplication not recognized by this analysis. It should be considered whether to replace karyotype analysis with CMA as the first-tier clinical diagnostic test for MCDK.
Applying CMA for fetuses with normal karyotypes and MCDK
We performed a CMA of 30 fetuses with MCDK and normal karyotypes using a high-resolution, whole-genome array. This method successfully identified five fetuses with pathogenic CNVs, increasing the detection rate of submicroscopic imbalances by an additional 16.7% (5/30). Caruana et al. [7] screened 178 postnatal patients presenting with CAKUT; however, a total of 10 cases were classified into the MCDK group, and only one case was found to be associated with pathogenic CNVs, leading to a pathogenic detection rate of 10% (1/10). The different detection frequency between two studies may be attributed to the difference in sample size and platform. This demonstrates that CMA is a useful tool in the prenatal diagnosis of fetal MCDK because it provides a higher diagnostic yield.
An ultrasound scan showed MCDK in the left kidney of Fetus 39 in addition to fetal growth restriction (FGR), although the fetal karyotype was normal (46,XY). CMA revealed a de novo 1.45-Mb deletion at the 7q11.23 region (chromosome position: 72701098–74154209, UCSC hg19) that overlapped with the critical region for WBS (OMIM 194050). WBS is a multisystem disease characterized by cardiovascular anomalies [19], mirror neuron system disorder [20, 21], metabolic abnormalities [22] and intrauterine growth retardation. When WBS occurs in the genitourinary system, the major clinical manifestations include renal cystic dysplasia, bladder diverticulum and recurrent urinary tract infections after birth [23, 24]. In this study, Fetus 39 had an overlapping region with WBS, and the clinical manifestations of MCDK in the left kidney plus FGR. The 7q11.23 deletion contains 23 OMIM genes, including the ELN (OMIM 130160) gene, which encodes elastin. An ELN mutation can result in subendothelial cell proliferation and the reorganization of smooth muscle; furthermore, it can cause the development of arterial thickening and markedly increase the risk of obstructive vascular disease [25]. Pober et al. [26] reported that renal artery stenosis was sought in nine patients presenting with WBS who underwent abdominal angiography during cardiac catheterization. Unilateral or bilateral mild renal artery narrowing was found in four persons and normal renal arteries in the remaining five. Faury et al. [27] reported that mice with haploinsufficiency for elastin were stably hypertensive from birth. They discussed the mechanism by which decreased elastin in vessel walls leads to hypertension. Thus, ELN gene mutation is likely to regulate different stages of the development of renal arteries and it might play a key role in the development of MCDK.
The prenatal ultrasound showed bilateral MCDK and polyhydramnios (amniotic fluid index = 290 mm) in Fetus 57 and enlarged kidneys (left kidney measuring 26 × 15 × 14 mm, right kidney measuring 26 × 14 × 14 mm) with MCDK in the right kidney of Fetus 72. The fetal karyotypes were 46,XY and 46,XX, respectively. The CMA revealed a de novo 1.49-Mb deletion at 17q12 (chromosome position: 34822465–36316144, UCSC hg19) in Fetus 57 and a 1.58-Mb deletion at a similar region (chromosome position: 34822465–36404555, UCSC hg19) in Fetus 72. According to the DECIPHER database, both deletions overlap with chromosome 17q12 deletion syndrome (OMIM 614527), an autosomal-dominant genetic disease with clinical phenotypic features that include RCAD (OMIM 137920) [28], neonatal cholestasis [29], autism spectrum disorder and schizophrenia [30, 31]. The gene responsible for RCAD syndrome is HNF1B (OMIM 189907). In a study of patients with fetal hyperechogenic or cystic kidneys, Decramer et al. identified a 17q12 deletion in 18 of 62 patients [32]. Verdeguer et al. found that the conditional knockout of HNF1B expression in young mice led to the development of polycystic kidneys. These authors concluded that HNF1B is required to rapidly reactivate the crucial target genes that proliferated cells towards tubule elongation [33]. Taking together our Fetuses 72 and 46 it seems most likely that the phenotype is due to the deletion of the HNF1B gene.
Fetus 65 presented with the clinical phenotypes of spina bifida, ventriculomegaly and left MCDK. CMA revealed a 1.16-Mb gain in 22q13.33 (chromosome position: 49654561–50817492, UCSC hg19), which overlapped the duplicated region in DECIPHER patient 283243. The 22q13.33 duplication contained 15 genes, among which the ALG12 (OMIM 607144) gene was related to the congenital disorder of glycosylation, type Ig (OMIM 607143), which can cause serious, sometimes fatal, malfunction of several different organ systems (especially the nervous system, muscles, intestines and urogenital system). Thus, the ALG12 gene might be responsible for the observed phenotype in Fetus 65. It is worth noting that DECIPHER patient 283243 demonstrated moderate intrauterine growth retardation and Tetralogy of Fallot, not clinical features of renal disease, which might be explained by the incomplete penetrance of the ALG12 gene.
The major feature of Fetus 71 was bilateral MCDK without amniotic fluid. CMA was used to detect a 1.57-Mb loss at 4q35.2 (chromosome position: 189380220–19095747, UCSC hg19) containing two OMIM genes: FRG1 (OMIM 601278) and FRG2 (OMIM 609032). The fragment overlapped with several pathogenic fragments reported in the DECIPHER database, with patient phenotypes of intellectual disability, microcephaly, delayed speech and language development, delayed gross motor development and low-set ears. No renal abnormalities have previously been reported. According to the OMIM database, the 4q35 genes FRG1 and FRG2, or FSHD region gene-1 and FSHD region gene-2, are inappropriately overexpressed in patients with facioscapulohumeral muscular dystrophy (FSHD, OMIM 158900). Therefore, we require more evidence that the 4q35.2 loss was the genetic cause of MCDK in this fetus.
Fetus 36 had MCDK in the left kidney. The CMA results revealed a 256-kb microduplication at 1p33 (chromosome 47365097–47620957) containing two pathogenic genes: CYP4X1 (OMIM 614999) and CYP4A11 (OMIM 601310). The fragment was a variant of unknown significance because it was not detected in the DGV or DECIPHER databases. A CYP4A11 mutation can cause essential hypertension. Nakagawa et al. created mice lacking Cyp4a10 (an ortholog of human CYP4A11), and they concluded that arachidonate monooxygenases can alter renal sodium reabsorption and blood pressure and might play a direct role in the gating activity of the kidney epithelial sodium channel [34]. Although highly related to the kidneys, this gene has not been reported in fetuses, and its effect on the fetus after birth is unknown. Hence, additional experiments are needed to confirm the relationship between CYP4A11 and MCDK.
Clinical follow-up assessment
Comparing the fetal prognoses of the 72 karyotype analyses with the 30 CMA analyses, we found that, if CMA was performed in all cases with normal karyotypes, then 15 (20 − 5 = 15) fetuses were identified as having negative CMA results. This result might help to increase confidence in continued pregnancy, especially for women carrying fetuses with unilateral MCDK.
CONCLUSIONS
The karyotype analysis in this study revealed that 4.17% of fetuses with MCDK had chromosome abnormalities. However, CMA might increase the detection rate of submicroscopic imbalances by an additional 16.7%. Moreover, all three of these unbalanced mutations were detected using CMA, suggesting that CMA can replace karyotype analysis and be used as the first-tier clinical diagnostic test for fetal MCDK. By applying CMA, we identified two syndromes known to be associated with MCDK (RCAD syndrome and WBS) and four new chromosomal regions possibly associated with MCDK (22q11.1 duplication, 22q13.33 duplication, 4q35.2 deletion and 1p33 duplication).
CONFLICT OF INTEREST
The authors declare that they have no competing financial interests.
ACKNOWLEDGEMENTS
This study was funded by the National Natural Science Foundation of China (81501267); the Department of Science and Technology of Guangdong Province (2013B022000005; 2014B020213001); and the Bureau of Science and Technology of Guangzhou Municipal (2014Y2-00059; 201300000086).
REFERENCES
Author notes
These authors contributed equally to this work.
Comments