-
PDF
- Split View
-
Views
-
Cite
Cite
Valentina Gracchi, Sophie M. van den Belt, Leanne K. Küpers, Eva Corpeleijn, Dick de Zeeuw, Hiddo J.L. Heerspink, Prevalence and distribution of (micro)albuminuria in toddlers, Nephrology Dialysis Transplantation, Volume 31, Issue 10, October 2016, Pages 1686–1692, https://doi.org/10.1093/ndt/gfv407
- Share Icon Share
Abstract
Microalbuminuria is common in the general adult population, with a prevalence of ∼7%, and is an independent indicator of renal and cardiovascular risks. Whether albuminuria is acquired during life (as a result of hypertension/diabetes) or is congenital and already present at birth is unknown. We studied the prevalence of microalbuminuria in toddlers and compared the distribution of albuminuria with that of the general adult population. In addition, we looked for possible associations between microalbuminuria and antenatal, postnatal and maternal factors.
The urinary albumin concentration (UAC) was measured in 1352 children and the urinary albumin:creatinine ratio (UACR) in 1288 children from the Groningen Expert Center for Kids with Obesity (GECKO) Drenthe cohort (age range 20–40 months). Albuminuria distribution was compared with the albuminuria distribution in 40 854 participants of the general adult cohort of the Prevention of Renal and Vascular End stage Disease (PREVEND) study. Associations between albuminuria (expressed as UAC and UACR) and antenatal, postnatal and maternal factors were tested with linear regression analysis.
The median UAC in the GECKO study was 2.3 mg/L (5th–95th percentiles: 2.1–25.5) and in the PREVEND study it was 6.0 mg/L (2.3–28.6) (P distribution comparison 0.053). The prevalence of UAC ≥ 20 mg/L was 6.9% in the GECKO study and 7.8% in the PREVEND study (P = 0.195). The prevalence of UACR ≥ 30 mg/g in the GECKO study was 23.4%. UAC and UACR were lower in boys. UAC was not associated with other determinants, but UACR was associated with age and gestational diabetes.
The distribution of UAC and the prevalence of UAC > 20 mg/L in toddlers and in the young general adult population are comparable. These findings suggest that microalbuminuria is a congenital condition that may predispose to a higher cardiovascular risk later in life.
INTRODUCTION
A mild to moderate increase of urinary albumin has a high prevalence in patients with diabetes mellitus, hypertension and chronic kidney disease [1, 2]. This so-called microalbuminuria appears to be a risk marker for renal and cardiovascular diseases later in life [3, 4].
It has been discussed whether these increased albuminuria levels are a consequence of renal disease or if albuminuria itself is an independent risk factor for renal and/or cardiovascular disease. Interesting in this context is the finding that apparently ‘healthy’ individuals without renal disease, diabetes or hypertension also show a high prevalence of microalbuminuria, varying from 5.5 to 6.6%. Microalbuminuria is also a predictor for future cardiovascular events [5–8]. These findings appear to support the suggestion that microalbuminuria is an independent risk factor—and could maybe even be a cause—of renal and cardiovascular diseases.
The putative mechanism of such a causal relationship between excessive albumin leak and renal/cardiovascular disease could be an endothelial dysfunction, such as glycocalyx loss [9]. This dysfunction ‘allows’ albumin to escape the vascular space and induces subendothelial vascular low-grade inflammation, with the ultimate consequence of widespread organ damage [10]. The cause of this endothelial dysfunction—and thus of the microalbuminuria—is unknown, but it might be congenital, meaning that different individuals are born with a large variability in vascular function and therefore varying constitutional urine albumin excretions. Indeed, increased albumin excretion is observed in newborns and toddlers in small studies measuring laboratory reference values [11]. This observation is supported by experimental studies demonstrating a large variability in endothelial function in rats at birth. These data also show that the rats with the poorest endothelial function were the most susceptible to renal damage [12]. This could imply that some individuals have already at birth high levels of albuminuria, as a result of inborn impaired endothelial function, which in the long term leads to a higher renal and cardiovascular risk [13].
The aim of the current study was to describe the distribution of albuminuria and the prevalence of microalbuminuria in very young children. Furthermore, we intended to identify antenatal, postnatal and maternal factors that could explain differences between children. For this purpose, we investigated a large cohort of toddlers of the general Dutch population [Groningen Expert Center for Kids with Obesity; (GECKO)]. These data were compared with the data from the general adult Dutch population of the Prevention of Renal and Vascular End stage Disease (PREVEND) cohort.
MATERIALS AND METHODS
This study was embedded in the GECKO Drenthe study, a population-based prospective birth cohort study of children born between April 2006 and April 2007 in Drenthe, a northern Dutch province. The primary goal of this cohort was to investigate overweight risk factors from birth throughout childhood. Details about this cohort have been previously described and are registered at www.birthcohorts.net [14]. The study was approved by the local Medical Research and Ethics Committee. All mothers signed an informed consent form.
In the recruitment period between April 2006 and April 2007, 4778 eligible children were born. Of them, 2874 parent–child pairs have ever participated actively in the cohort. At birth, the obstetricians, midwives or general practitioners took an umbilical cord blood sample that was processed and stored for future analysis. In addition, placenta weight was measured and data on pregnancy and delivery (i.e. gestational diabetes, gestational hypertension, smoking of the mother, maternal age at delivery) were recorded. The study design included anthropometric measurements (including weight, height and head, waist and hip circumference) performed by specifically trained nurses at municipal health services at the ages of 0.5, 1, 2, 3, 4, 5, 6, 7, 9, 11, 14, 18, 24, 36, 45 and 60 months. Questionnaires about lifestyle and environment (including maternal education level) were filled in by parents in the last trimester of pregnancy and at each health control visit.
The 24-month visit was complemented by a measurement of arterial blood pressure and urine collection. For the present analyses, exclusion was based on the availability of a urine sample with albumin measurement. A urinary albumin measurement was obtained in 1352 children.
Blood pressure measurement
Blood pressure measurement was performed on the left arm in a sitting position, with an automatic device (Dinamap, Johnson & Johnson, New Brunswick, NJ, USA). Blood pressure measurement was repeated if the child remained comfortable during the procedure or if the first measurement was unsuccessful.
Urine collection and measurements
Urine collection in toddlers is known to be cumbersome, and urine cannot be extracted from disposable diapers. The currently available plastic bags for urine collection tend to detach easily from children's skin. We therefore designed and validated a specific protocol for urine collection at the Clinical Pharmacology Department of the University Medical Center Groningen (UMCG) using pantyliners. We chose a pantyliner with a simple cottonwool filling, because pilot tests showed that extracting the urine from these pantyliners was easy and convenient for parents and children. Parents received during the 24-month health control visit a kit with a pantyliner (Kruidvat Femme Care mini®, Kruidvat, The Netherlands), a plastic vial and safety bag (both from Minigrip, Lelystad, The Netherlands), a collection form and a postal plastic envelope for biological materials. Parents were asked to place the pantyliner in the child's diaper and remove it once the child had voided. They were also asked to write down the time of placement and removal of the pantyliner. Parents were instructed to discard pantyliners in case of faecal contamination and to repeat the operation later in time. Pantyliners with properly collected urine were placed by parents in the plastic vial and sent as soon as possible by regular mail to the hospital using a dedicated envelope for biological materials. Parents were also asked to include a collection form stating the collection date, child's study number and possible concurrent diseases. Directly upon arrival at the laboratory, the cotton filling of the pantyliners was extracted and centrifuged at 3600 rpm for 10 min (Mistral 3000, MSE). UAC was measured in the centrifuged urine by nephelometry (Behring Nephelometer Analyzer II, Siemens Medical Solutions), with a threshold of 2.1 mg/L. Values of UAC below the detection limit were assigned the value of 2.1 mg/L. Urinary creatinine concentration (Ucc) was measured by the enzymatic method (Roche Modular), with a threshold of 0.1 mmol/L.
Performance of the method against standard urine collection for albumin measurement was analysed as proposed by Stevens [15], presenting bias, precision and accuracy. Bias was defined as the median difference between standard urine collection and the pantyliner method, precision as the interquartile range of the difference between the standard method and pantyliner method and accuracy as the percentage of estimates of the pantyliner method within 30% of the value of the standard method. Bias of the method was −14.0 mg/L, precision 31.3 mg/L and accuracy 48.1%.
Adult cohort
The PREVEND study protocol has been extensively described elsewhere and can be found at www.prevend.org [5]. In short, in the period between 1997 and 1998, all inhabitants of the city of Groningen (in the northern part of The Netherlands) ages 28–75 years (n = 85421) were invited to answer a short questionnaire regarding demographics and risk factors for renal and cardiovascular diseases and to collect a first morning void urine sample for albumin concentration measurement. A total of 40 854 individuals (48%) gave written informed consent and were included in the PREVEND cohort. The characteristics of the 40 854 PREVEND study participants are shown in Supplementary data, Table S1.
Statistical analysis
Descriptive characteristics of the study population were reported as mean ± standard deviation or median (25th–75th percentile), as required. The distributions of UAC between the toddler and adult population were compared with the Brown and Forsythe modified Levene's robust statistics test. Since urinary creatinine was not measured in the PREVEND cohort, only comparison of UAC was possible between the two cohorts. The difference in prevalence of increased albuminuria (UAC ≥ 20 mg/L) between the GECKO and PREVEND studies was tested with a χ2 test. Possible associations between antenatal, postnatal and maternal factors and the logarithm of UAC were analysed with univariate linear regression analysis. UAC was log-transformed to account for its skewed distribution. A P-value < 0.05 was considered to be statistically significant. Data were analysed using STATA version 13.1 and SPSS Statistics version 22.
RESULTS
Of the 1352 children included in this study (Figure 1), measurements of Ucc were available in 1288 children. Data for body mass index (BMI) were available in 1017 children, blood pressure measurement in 817 children and placenta weight in 940 children. The characteristics of the study population are shown in Table 1.
. | Overall population (n = 1352) . | UACR population (n = 1288) . |
---|---|---|
Toddler characteristics | ||
Age, months (25th–75th percentile) | 25.8 (24.8–27.1) | 25.8 (24.8–27.1) |
Male, n (%) | 683 (50.5) | 655 (50.9) |
UAC, mg/L (25th–75th percentile) | 2.3 (2.1–7.1) | 2.4 (2.1–7.1) |
Body weight, kg (SD) | 13.2 (1.5) | 13.2 (1.5) |
BMI, kg/m2 (SD) | 16.3 (1.3) | 16.3 (1.3) |
Systolic BP, mmHg (SD) | 101.1 (12.5) | 101.2 (12.6) |
Diastolic BP, mmHg (SD) | 60.5 (11.5) | 60.5 (11.6) |
Pregnancy, birth and maternal characteristics | ||
Gestational age at birth, weeks (SD) | 39.8 (1.6) | 39.8 (1.6) |
Birthweight, g (SD) | 3550.8 (565.9) | 3553 (563) |
Placenta weight, g (SD) | 654.4 (153.8) | 650 (149) |
Maternal age at delivery, years (SD) | 30.9 (4.3) | 30.9 (4.25) |
Gestational diabetes, n (%) | 47 (3.5) | 43 (3.36) |
Gestational hypertension, n (%) | 146 (10.9) | 140 (10.92) |
Smoking during pregnancy, n (%) | 164 (12.3) | 155 (12.20) |
Maternal education level (university), n (%) | 497 (38.0) | 478 (38.24) |
. | Overall population (n = 1352) . | UACR population (n = 1288) . |
---|---|---|
Toddler characteristics | ||
Age, months (25th–75th percentile) | 25.8 (24.8–27.1) | 25.8 (24.8–27.1) |
Male, n (%) | 683 (50.5) | 655 (50.9) |
UAC, mg/L (25th–75th percentile) | 2.3 (2.1–7.1) | 2.4 (2.1–7.1) |
Body weight, kg (SD) | 13.2 (1.5) | 13.2 (1.5) |
BMI, kg/m2 (SD) | 16.3 (1.3) | 16.3 (1.3) |
Systolic BP, mmHg (SD) | 101.1 (12.5) | 101.2 (12.6) |
Diastolic BP, mmHg (SD) | 60.5 (11.5) | 60.5 (11.6) |
Pregnancy, birth and maternal characteristics | ||
Gestational age at birth, weeks (SD) | 39.8 (1.6) | 39.8 (1.6) |
Birthweight, g (SD) | 3550.8 (565.9) | 3553 (563) |
Placenta weight, g (SD) | 654.4 (153.8) | 650 (149) |
Maternal age at delivery, years (SD) | 30.9 (4.3) | 30.9 (4.25) |
Gestational diabetes, n (%) | 47 (3.5) | 43 (3.36) |
Gestational hypertension, n (%) | 146 (10.9) | 140 (10.92) |
Smoking during pregnancy, n (%) | 164 (12.3) | 155 (12.20) |
Maternal education level (university), n (%) | 497 (38.0) | 478 (38.24) |
Values for continuous variables are described as mean ± standard deviation or median (25th–75th percentile), as required; values for categorical variables as number (percentage).
UAC, urinary albumin concentration; UACR, urinary albumin-to-creatinine ratio; BP, blood pressure; BMI, body mass index.
. | Overall population (n = 1352) . | UACR population (n = 1288) . |
---|---|---|
Toddler characteristics | ||
Age, months (25th–75th percentile) | 25.8 (24.8–27.1) | 25.8 (24.8–27.1) |
Male, n (%) | 683 (50.5) | 655 (50.9) |
UAC, mg/L (25th–75th percentile) | 2.3 (2.1–7.1) | 2.4 (2.1–7.1) |
Body weight, kg (SD) | 13.2 (1.5) | 13.2 (1.5) |
BMI, kg/m2 (SD) | 16.3 (1.3) | 16.3 (1.3) |
Systolic BP, mmHg (SD) | 101.1 (12.5) | 101.2 (12.6) |
Diastolic BP, mmHg (SD) | 60.5 (11.5) | 60.5 (11.6) |
Pregnancy, birth and maternal characteristics | ||
Gestational age at birth, weeks (SD) | 39.8 (1.6) | 39.8 (1.6) |
Birthweight, g (SD) | 3550.8 (565.9) | 3553 (563) |
Placenta weight, g (SD) | 654.4 (153.8) | 650 (149) |
Maternal age at delivery, years (SD) | 30.9 (4.3) | 30.9 (4.25) |
Gestational diabetes, n (%) | 47 (3.5) | 43 (3.36) |
Gestational hypertension, n (%) | 146 (10.9) | 140 (10.92) |
Smoking during pregnancy, n (%) | 164 (12.3) | 155 (12.20) |
Maternal education level (university), n (%) | 497 (38.0) | 478 (38.24) |
. | Overall population (n = 1352) . | UACR population (n = 1288) . |
---|---|---|
Toddler characteristics | ||
Age, months (25th–75th percentile) | 25.8 (24.8–27.1) | 25.8 (24.8–27.1) |
Male, n (%) | 683 (50.5) | 655 (50.9) |
UAC, mg/L (25th–75th percentile) | 2.3 (2.1–7.1) | 2.4 (2.1–7.1) |
Body weight, kg (SD) | 13.2 (1.5) | 13.2 (1.5) |
BMI, kg/m2 (SD) | 16.3 (1.3) | 16.3 (1.3) |
Systolic BP, mmHg (SD) | 101.1 (12.5) | 101.2 (12.6) |
Diastolic BP, mmHg (SD) | 60.5 (11.5) | 60.5 (11.6) |
Pregnancy, birth and maternal characteristics | ||
Gestational age at birth, weeks (SD) | 39.8 (1.6) | 39.8 (1.6) |
Birthweight, g (SD) | 3550.8 (565.9) | 3553 (563) |
Placenta weight, g (SD) | 654.4 (153.8) | 650 (149) |
Maternal age at delivery, years (SD) | 30.9 (4.3) | 30.9 (4.25) |
Gestational diabetes, n (%) | 47 (3.5) | 43 (3.36) |
Gestational hypertension, n (%) | 146 (10.9) | 140 (10.92) |
Smoking during pregnancy, n (%) | 164 (12.3) | 155 (12.20) |
Maternal education level (university), n (%) | 497 (38.0) | 478 (38.24) |
Values for continuous variables are described as mean ± standard deviation or median (25th–75th percentile), as required; values for categorical variables as number (percentage).
UAC, urinary albumin concentration; UACR, urinary albumin-to-creatinine ratio; BP, blood pressure; BMI, body mass index.
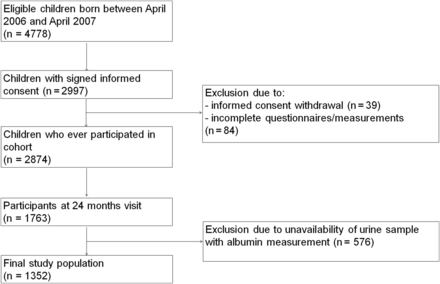
The distribution of UAC in the GECKO cohort is shown in Figure 2A. The median UAC was 2.3 mg/L (25th–75th percentile interval 2.1–7.1 mg/L). The 5th and 95th percentiles were, respectively, 2.1 and 25.5 mg/L. The median UACR was 14.0 mg/g (25th–75th percentile interval 8.0–25.6 mg/g). The 5th and 95th percentiles were, respectively, 4.3 and 89.3 mg/g. The prevalence of elevated albuminuria based on UAC ( ≥20 mg/L) and UACR (≥30 mg/g) was, respectively, 6.9 and 23.4%.
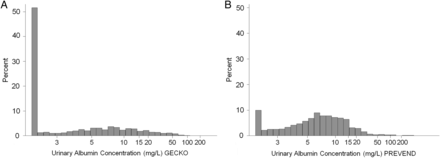
The distribution of UAC in the PREVEND population (n = 40854) is shown in Figure 2B. The median UAC was 6.0 mg/L (25th–75th percentile interval 3.7–9.8 mg/L). The 5th and 95th percentiles were, respectively, 2.3 and 28.6 mg/L. The prevalence of elevated UAC was 7.8%. The distribution of UAC in the GECKO and PREVEND population did not differ significantly (Figure 2; P = 0.053), as did the prevalence of elevated albuminuria (Figure 3; P = 0.195).
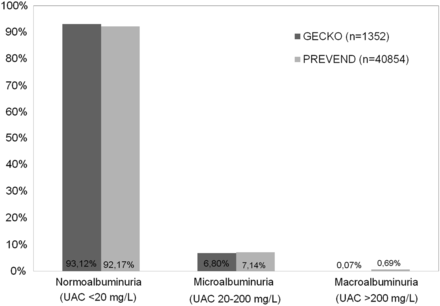
Prevalence of different levels of urinary albumin concentration (UAC) in the general toddler population (GECKO) when compared with the general adult population (PREVEND) in the northern Dutch provinces.
The prevalence of elevated albuminuria in the PREVEND study depended on age (the older, the more microalbuminuria). Therefore, we plotted the percentage of increased albuminuria of the GECKO study combined with the PREVEND study according to age (Figure 4). Although there is a large age gap between the two cohorts, it seems that the GECKO data are similar to those of the young adult population of PREVEND. The prevalence of increased albuminuria in the PREVEND population <50 years of age was 5.8 versus 6.9% in GECKO (P = 0.09).
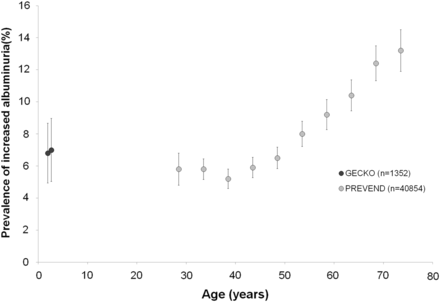
Prevalence of increased albuminuria (UAC ≥ 20 mg/L) in different age ranges in the general population of the Dutch northern provinces (data from the GECKO and PREVEND cohorts).
We tested the hypothesis of possible associations between UAC or UACR in the GECKO cohort and antenatal, postnatal and maternal factors. UAC and UACR were negatively associated with male gender (P < 0.001). No association was found between UAC and other antenatal, postnatal or maternal factors. UACR was associated with age and gestational diabetes (Table 2).
. | UAC . | UACR . | ||
---|---|---|---|---|
Coefficient (95% CI) . | P-value . | Coefficient (95% CI) . | P-value . | |
Toddler characteristics | ||||
Age, months | −0.02 (−0.04–0.01) | 0.119 | −0.04 (−0.07–0.01) | 0.003 |
Male gender | −0.20 (0.11–0.30) | <0.001 | −0.13 (0.03–0.24) | 0.010 |
BMI, kg/m2 | 0.03 (−0.01–0.07) | 0.158 | 0.04 (−0.00–0.01) | 0.063 |
Systolic BP, mmHg | 0.00 (0.00–0.01) | 0.687 | 0.00 (−0.01–0.01) | 0.914 |
Diastolic BP, mmHg | 0.00 (−0.01–0.01) | 0.926 | −0.00 (0.01–0.00) | 0.585 |
Pregnancy, birth and maternal characteristics | ||||
Gestational age at birth, weeks | −0.00 (−0.03–0.03) | 0.903 | −0.01 (−0.04–0.02) | 0.586 |
Birthweight, g | 0.00 (−0.00–0.00) | 0.994 | 0.00 (−0.00–0.00) | 0.203 |
Placenta weight, g | −0.00 (−0.00–0.00) | 0.488 | 0.00 (−0.00–0.00) | 0.948 |
Maternal age at delivery, years | −0.00 (−0.01–0.01) | 0.615 | −0.00 (−0.01–0.00) | 0.899 |
Gestational diabetes | 0.18 (−0.08–0.44) | 0.165 | 0.34 (0.06–0.63) | 0.019 |
Gestational hypertension | −0.05 (−0.20–0.10) | 0.532 | −0.11 (−0.27–0.06) | 0.202 |
Smoking during pregnancy (yes versus no) | −0.11 (−0.26–0.03) | 0.123 | 0.02 (−0.14–0.18) | 0.821 |
Maternal university (yes versus no) | −0.02 (−0.12–0.08) | 0.727 | −0.03 (−0.14–0.08) | 0.612 |
. | UAC . | UACR . | ||
---|---|---|---|---|
Coefficient (95% CI) . | P-value . | Coefficient (95% CI) . | P-value . | |
Toddler characteristics | ||||
Age, months | −0.02 (−0.04–0.01) | 0.119 | −0.04 (−0.07–0.01) | 0.003 |
Male gender | −0.20 (0.11–0.30) | <0.001 | −0.13 (0.03–0.24) | 0.010 |
BMI, kg/m2 | 0.03 (−0.01–0.07) | 0.158 | 0.04 (−0.00–0.01) | 0.063 |
Systolic BP, mmHg | 0.00 (0.00–0.01) | 0.687 | 0.00 (−0.01–0.01) | 0.914 |
Diastolic BP, mmHg | 0.00 (−0.01–0.01) | 0.926 | −0.00 (0.01–0.00) | 0.585 |
Pregnancy, birth and maternal characteristics | ||||
Gestational age at birth, weeks | −0.00 (−0.03–0.03) | 0.903 | −0.01 (−0.04–0.02) | 0.586 |
Birthweight, g | 0.00 (−0.00–0.00) | 0.994 | 0.00 (−0.00–0.00) | 0.203 |
Placenta weight, g | −0.00 (−0.00–0.00) | 0.488 | 0.00 (−0.00–0.00) | 0.948 |
Maternal age at delivery, years | −0.00 (−0.01–0.01) | 0.615 | −0.00 (−0.01–0.00) | 0.899 |
Gestational diabetes | 0.18 (−0.08–0.44) | 0.165 | 0.34 (0.06–0.63) | 0.019 |
Gestational hypertension | −0.05 (−0.20–0.10) | 0.532 | −0.11 (−0.27–0.06) | 0.202 |
Smoking during pregnancy (yes versus no) | −0.11 (−0.26–0.03) | 0.123 | 0.02 (−0.14–0.18) | 0.821 |
Maternal university (yes versus no) | −0.02 (−0.12–0.08) | 0.727 | −0.03 (−0.14–0.08) | 0.612 |
Univariate linear regression on logUAC and logUACR.
UAC, urinary albumin concentration; UACR, urinary albumin-to-creatinine ratio; CI, confidence interval; BP, blood pressure; BMI, body mass index.
. | UAC . | UACR . | ||
---|---|---|---|---|
Coefficient (95% CI) . | P-value . | Coefficient (95% CI) . | P-value . | |
Toddler characteristics | ||||
Age, months | −0.02 (−0.04–0.01) | 0.119 | −0.04 (−0.07–0.01) | 0.003 |
Male gender | −0.20 (0.11–0.30) | <0.001 | −0.13 (0.03–0.24) | 0.010 |
BMI, kg/m2 | 0.03 (−0.01–0.07) | 0.158 | 0.04 (−0.00–0.01) | 0.063 |
Systolic BP, mmHg | 0.00 (0.00–0.01) | 0.687 | 0.00 (−0.01–0.01) | 0.914 |
Diastolic BP, mmHg | 0.00 (−0.01–0.01) | 0.926 | −0.00 (0.01–0.00) | 0.585 |
Pregnancy, birth and maternal characteristics | ||||
Gestational age at birth, weeks | −0.00 (−0.03–0.03) | 0.903 | −0.01 (−0.04–0.02) | 0.586 |
Birthweight, g | 0.00 (−0.00–0.00) | 0.994 | 0.00 (−0.00–0.00) | 0.203 |
Placenta weight, g | −0.00 (−0.00–0.00) | 0.488 | 0.00 (−0.00–0.00) | 0.948 |
Maternal age at delivery, years | −0.00 (−0.01–0.01) | 0.615 | −0.00 (−0.01–0.00) | 0.899 |
Gestational diabetes | 0.18 (−0.08–0.44) | 0.165 | 0.34 (0.06–0.63) | 0.019 |
Gestational hypertension | −0.05 (−0.20–0.10) | 0.532 | −0.11 (−0.27–0.06) | 0.202 |
Smoking during pregnancy (yes versus no) | −0.11 (−0.26–0.03) | 0.123 | 0.02 (−0.14–0.18) | 0.821 |
Maternal university (yes versus no) | −0.02 (−0.12–0.08) | 0.727 | −0.03 (−0.14–0.08) | 0.612 |
. | UAC . | UACR . | ||
---|---|---|---|---|
Coefficient (95% CI) . | P-value . | Coefficient (95% CI) . | P-value . | |
Toddler characteristics | ||||
Age, months | −0.02 (−0.04–0.01) | 0.119 | −0.04 (−0.07–0.01) | 0.003 |
Male gender | −0.20 (0.11–0.30) | <0.001 | −0.13 (0.03–0.24) | 0.010 |
BMI, kg/m2 | 0.03 (−0.01–0.07) | 0.158 | 0.04 (−0.00–0.01) | 0.063 |
Systolic BP, mmHg | 0.00 (0.00–0.01) | 0.687 | 0.00 (−0.01–0.01) | 0.914 |
Diastolic BP, mmHg | 0.00 (−0.01–0.01) | 0.926 | −0.00 (0.01–0.00) | 0.585 |
Pregnancy, birth and maternal characteristics | ||||
Gestational age at birth, weeks | −0.00 (−0.03–0.03) | 0.903 | −0.01 (−0.04–0.02) | 0.586 |
Birthweight, g | 0.00 (−0.00–0.00) | 0.994 | 0.00 (−0.00–0.00) | 0.203 |
Placenta weight, g | −0.00 (−0.00–0.00) | 0.488 | 0.00 (−0.00–0.00) | 0.948 |
Maternal age at delivery, years | −0.00 (−0.01–0.01) | 0.615 | −0.00 (−0.01–0.00) | 0.899 |
Gestational diabetes | 0.18 (−0.08–0.44) | 0.165 | 0.34 (0.06–0.63) | 0.019 |
Gestational hypertension | −0.05 (−0.20–0.10) | 0.532 | −0.11 (−0.27–0.06) | 0.202 |
Smoking during pregnancy (yes versus no) | −0.11 (−0.26–0.03) | 0.123 | 0.02 (−0.14–0.18) | 0.821 |
Maternal university (yes versus no) | −0.02 (−0.12–0.08) | 0.727 | −0.03 (−0.14–0.08) | 0.612 |
Univariate linear regression on logUAC and logUACR.
UAC, urinary albumin concentration; UACR, urinary albumin-to-creatinine ratio; CI, confidence interval; BP, blood pressure; BMI, body mass index.
DISCUSSION
This study provides unique information about the natural distribution of albuminuria in the general toddler population. The most interesting finding is the wide range of albuminuria in young children. This shows how in toddlers, just as in the general adult population of the same geographic region, there is an important variability in albumin excretion. Moreover, when comparing the prevalence of UAC ≥ 20 mg/L in the young adult and toddler cohorts, these are strikingly similar, increasing in time as individuals get older. To our knowledge, this is the first time that urine albumin levels have been studied on an epidemiological scale in toddlers.
What is the meaning of these increased levels of urinary albumin already in such young individuals? And what is the meaning of the striking similarity of the prevalence of microalbuminuria in the toddler and adult population? If increased levels of albumin in the urine are a consequence of renal damage, these children must have been damaged just after birth or in utero. This could fit with the so-called Barker hypothesis in which ‘external’ influences determine the intra-uterine development of the foetus, causing diseases later in life [16]. We selected a number of variables that, according to the Barker hypothesis, could have had a relationship with microalbuminuria. Some associations have been previously reported [17, 18]. In our study, we could not find an association with factors such as birthweight or placenta weight, as we would have expected on the basis of the Barker hypothesis. The only association found was between UACR and gestational diabetes and between UACR and age. It could be that albuminuria at the paediatric age is mostly affected by genetic factors and predisposition and not by (or less by) environmental or pregnancy factors. This does not exclude that these factors could have a stronger influence on renal outcome later in life. Nevertheless, the lack of associations between albuminuria and birthweight, gestational age, impaired placental growth, gestational hypertension and smoking during pregnancy does not support the Barker hypothesis as a mechanism for the development of increased albuminuria in toddlers.
An alternative hypothesis would be that children are born with a constitutive different albuminuria excretion (natural variation). It is well possible that children are born with a variety of vascular function (endothelial function) and that this is reflected by a varying degree of albumin leakage in all vessels including the kidney [13]. When urinary albumin concentration is plotted against age in the adult population, it becomes clear that urinary albumin concentration varies between individuals at all ages (Figure 5) [5, 19]. Intriguingly, when the individual variation of albuminuria in toddlers and children is compared with the adult population [11], a strikingly similar pattern is observed with nearly similar median and 95th percentiles of the urinary albumin concentration from young age to adolescence. These findings suggest that an individual is born with a certain albuminuria level that remains (relatively) constant for that individual over time and may represent a vascular state that is associated with a risk for organ damage. These findings make the alternative hypothesis that high urinary albumin excretion in toddlers is an expression of vasculature development early in life and increased albuminuria diminishes during development less likely. Longitudinal studies with multiple albuminuria measurements during life are obviously necessary to confirm or refute this hypothesis.
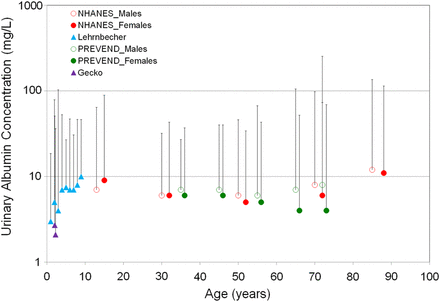
Individual variation of urinary albumin concentration is relatively constant with ageing. Median and 97.5th percentile of the UAC distribution according to different age categories in two adult general population cohorts (PREVEND and NHANES), a child cohort (Lehrnbecher) and a toddler cohort (GECKO). *95th percentile of UAC in NHANES was plotted.
Most adult studies use a creatinine-corrected urinary albumin concentration as the optimal representation for describing the amount of albumin leakage [7, 20]. The use of creatinine is meant to correct albumin for urine concentration and dilution differences, and indeed works well in adults [21]. A striking finding of our study is that, according to the UACR cut-off points used in the adult population (i.e. UACR ≥ 30 mg/g), 23.4% of the toddlers were found to have high urinary albumin. This extremely high prevalence might be driven by the low urinary creatinine values in toddlers, which reflects the lower muscle mass when compared with older children and adults. Although UACR is a useful measurement also in toddlers, we believe that the cut-off points used for adults are not appropriate for young children and we suggest that the 95th percentile should be used. In the study by Sanchez-Bayle et al. [22], a similar median UACR was found in children <6 years of age, but our cohort shows a wider distribution. We speculate that this might be related to a different genetic background of the populations or to differences in environmental factors.
A certainly interesting finding in our cohort is the gender difference in albuminuria, both if expressed as UAC or UACR. Male gender had a statistically significant negative association with albuminuria, meaning that female toddlers have a higher urinary albumin concentration when compared with males. In studies on general adult populations, a higher male prevalence of albuminuria has mostly been found. In paediatric studies, data are non-consistent [22, 23]. Nevertheless, a higher prevalence of high urinary albuminuria has been found also in the National Health and Nutrition Examination Survey (NHANES) [19]. Albuminuria in this survey was expressed as UACR, and the children of this survey were older (6–19 years), so the differences were interpreted as differences in muscle mass. This explanation cannot be applied to our cohort, as Ucc did not differ per gender. We cannot exclude that this finding in our cohort is only due to chance and this needs to be confirmed or refuted in repeated studies.
Probably because of difficulties in urine collection in toddlers and young children, most previous paediatric studies have investigated urinary albumin in school-aged children or adolescents [22–25]. Data concerning albuminuria in toddlers are limited to small numbers of individuals [11]. To our knowledge, this is the first study investigating albuminuria in a large toddler cohort. Many of the previous studies on albuminuria in children report daily urinary albumin excretion, UACR or UAC in spot urine, but not microalbuminuria prevalence. Unfortunately, this hampers direct comparison with our data. In addition, to our knowledge, this is the first study that directly compared the prevalence of microalbuminuria in a toddler population with the adult population of the same geographical region.
Our study has several limitations. The UAC measurement was done on a single sample. Thus we have no information on whether the albuminuria values in these children are constant in time or could be influenced by concurrent problems such as viral infections or timing of the sampling (morning versus afternoon), and we do not know if these values are representative of the daily urinary albumin excretion. However, we made the comparison with an adult population also using single urine samples to determine the prevalence of microalbuminuria. Second, the urine collection method used has a rather poor recovery. It is possible that the albumin is retained in the cotton wool of the pantyliners in some individuals, leading to albumin concentrations below the detection limit. This bias, however, does not have a big impact on our conclusion since it only leads to an underestimation of the UAC measurements in the toddlers.
In conclusion, urinary albumin excretion in toddlers is very similar to that in young adults, including the prevalence of microalbuminuria. This finding has important implications for the current pathophysiological view on albuminuria as a cause or consequence of renal and cardiovascular disease. Our data suggest that albumin excretion may represent an inborn constitutive characteristic, determining the fate of the individual in later life.
FUNDING
The GECKO Drenthe birth cohort was funded by an unrestricted grant of Hutchison Whampoa Ltd, Hong Kong and received support from the UMCG.
SUPPLEMENTARY MATERIAL
Supplementary data are available online at http://ndt.oxfordjournals.org.
CONFLICT OF INTEREST STATEMENT
D.d.Z. is a consultant for and receives honoraria (to employer) from AbbVie, Astellas, ChemoCentryx, Eli Lilly, Janssen, Fresenius and Merck Darmstadt. H.J.L.H. received a research grant from AstraZeneca (payment to employer) and is a consultant for and receives honoraria (to employer) from AbbVie, Astellas, Janssen, Reata Pharmaceuticals, Vitae and ZS-Pharma.
ACKNOWLEDGEMENTS
We thank all parents and children for participation in the GECKO Drenthe cohort, Prof. S.J.L. Bakker for his support in designing urine collections and Dr Kröpelin for statistical advice and support.
REFERENCES
Author notes
Both authors contributed equally to the manuscript.
Comments