-
PDF
- Split View
-
Views
-
Cite
Cite
Petter Bjornstad, David Z. Cherney, Janet K. Snell-Bergeon, Laura Pyle, Marian Rewers, Richard J. Johnson, David M. Maahs, Rapid GFR decline is associated with renal hyperfiltration and impaired GFR in adults with Type 1 diabetes, Nephrology Dialysis Transplantation, Volume 30, Issue 10, October 2015, Pages 1706–1711, https://doi.org/10.1093/ndt/gfv121
- Share Icon Share
Abstract
Rapid glomerular filtration rate (GFR) decline (>3 mL/min/1.73 m2) is an increasingly recognized high-risk diabetic nephropathy (DN) phenotype in Type 1 diabetes. Rapid GFR decline is a recognized predictor of impaired GFR (<60 mL/min/1.73 m2). However, the association between rapid GFR decline and renal hyperfiltration is not well described in Type 1 diabetes. We hypothesized that renal hyperfiltration (estimated glomerular filtration rate, eGFR ≥ 120 mL/min/1.73 m2) would predict rapid GFR decline over 6 years and that rapid GFR decline would predict impaired GFR at 6 years in adults with Type 1 diabetes.
GFR was calculated by chronic kidney disease epidemiology (CKD-EPI) creatinine in 646 adults with Type 1 diabetes in the coronary artery calcification in Type 1 diabetes study. Logistic multivariable models were employed to investigate the relationships between renal hyperfiltration and rapid GFR decline, and rapid GFR decline and incident impaired GFR over 6 years.
Renal hyperfiltration predicted greater odds of rapid GFR decline over 6 years [odds ratio (OR): 5.00, 95% confidence interval (CI): 3.03–8.25, P < 0.0001] adjusting for hemoglobin A1c (HbA1c), systolic blood pressure (SBP), low-density lipoprotein cholesterol (LDL-C), sex, duration, log of albumin/creatinine ratio and estimated insulin sensitivity. Furthermore, rapid GFR decline predicted greater odds of incident impaired eGFR (OR: 15.99, 95% CI 2.34–114.37, P = 0.006) in a similarly adjusted model. Sensitivity analyses with GFR calculated by CKD-EPI combined creatinine and cystatin C, and renal hyperfiltration defined as ≥135 mL/min/1.73 m2 yielded similar results.
In adults with Type 1 diabetes, rapid GFR decline over 6 years was associated with baseline renal hyperfiltration and incident GFR impairment. These observations may suggest an intermediate and predictive role of rapid GFR decline in the progression of DN.
INTRODUCTION
Diabetic nephropathy (DN) remains the single most important cause of renal failure in the Western world [1–3], and early detection of risk factors for DN remains a major goal for endocrinologists and nephrologists.
The appearance of microalbuminuria is usually the earliest clinical sign of DN, but the paradigm of early DN has evolved over the past few years based on the demonstration that microalbuminuria does not necessarily imply progressive nephropathy and may in fact regress to normoalbuminuria [4, 5]. Moreover, The Diabetes Control and Complications Trial and Epidemiology of Diabetes Interventions and Complications study (DCCT-EDIC) reported incident glomerular filtration rate (GFR) <60 mL/min/1.73 m2 in 24% of patients without prior microalbuminuria [6]. Therefore, GFR may be the most clinically relevant measure of DN in Type 1 diabetes.
Decades ago, Mogensen suggested that renal hyperfiltration (GFR ≥ 120 mL/min/1.73 m2) might be a risk factor for DN [7, 8], and a number of studies have subsequently affirmed this association, especially in adults with Type 2 diabetes [9]. However, to our knowledge, the linkage between renal hyperfiltration and kidney disease in Type 1 diabetes has been primarily limited to the development of albuminuria [10, 11]. Specifically, since the fall of GFR should be the primary target risk factor, we have introduced the concept of a rapid fall in GFR (defined as annual GFR loss > 3 mL/min/1.73 m2) as an intermediate high-risk phenotype to separate it from issues related to albuminuria or slow changes in GFR that may occur with aging or mild changes in hydration status. Furthermore, the relationships between renal hyperfiltration and rapid GFR decline, and how these early phenotypes of DN relate to incident impaired GFR (<60 mL/min/1.73 m2) remains poorly understood in adults with Type 1 diabetes.
Accordingly, in this study, we sought to examine the associations between baseline renal hyperfiltration, rapid GFR decline and incident impaired GFR over 6 years in adults with Type 1 diabetes. We hypothesized that renal hyperfiltration at baseline would be associated with rapid GFR decline over 6 years and that rapid GFR decline would be associated with incident impaired GFR at 6-year follow-up in adults with Type 1 diabetes.
MATERIALS AND METHODS
Cohort and methods
The coronary artery calcification in Type 1 diabetes (CACTI) study enrolled subjects of 19–56 years old, with and without Type 1 diabetes, who were asymptomatic for cardiovascular disease at the baseline visit in 2000–2 and then were reexamined 3 and 6 years later, as previously described [12]. Subjects with the serum creatinine level of >2 mg/dL were excluded at baseline, unless they were participants in the pilot study. Participants (n = 646 at baseline, n = 525 at 6 years) had data to calculate estimated glomerular filtration rate (eGFR) by CKD-EPI creatinine and were eligible for inclusion in the present analyses. The study was approved by the Colorado Multiple Institutional Review Board, and all participants provided informed consent.
Hypertension was defined as current anti-hypertensive therapy or untreated hypertension (BP ≥ 140/90 mmHg) at the time of each study visit. Anti-hypertensive medication use was determined by a medication inventory [12], and use of an ACE inhibitor or an angiotensin receptor blocker was combined for these analyses.
After an overnight fast, blood was collected, centrifuged and separated. Blood glucose was measured using standard enzymatic methods, and high-performance liquid chromatography was used to measure HbA1c (HPLC, BioRad variant). Total plasma cholesterol and triglyceride (TG) levels were measured using standard enzymatic methods, high-density lipoprotein cholesterol was separated using dextran sulfate and LDL-C was calculated using the Friedewald formula. Cystatin C was measured using the commercially available Dade-Behring assay following package insert instructions on a BNII or ProSpec instrument [13]. GFR was determined using the CKD-EPI creatinine equation published by the CKD-EPI Investigators Group [14]. We also calculated eGFR by CKD-EPI combined creatinine and cystatin C as part of a sensitivity analyses. Rapid GFR decline was defined as an annual eGFR loss of >3 mL/min/1.73 m2, and renal hyperfiltration was defined as eGFR ≥ 120 mL/min/1.73 m2. Incident impaired GFR was defined as the development of eGFR < 60 mL/min/1.73 m2 at 6-year follow-up without prior documented impaired GFR.
Estimated insulin sensitivity (eIS) was calculated using an equation developed in a subset of the study cohort who underwent a hyperinsulinemic-euglycemic clamp study to measure insulin sensitivity, as previously described in detail [15]. The model included waist circumference, daily insulin dose per kilogram body weight, TGs and diastolic blood pressure (DBP): exp[4.1075–0.01299 × waist (cm) − 1.05819 × insulin dose (daily units/kg) − 0.00354 × TGs (mg/dL) − 0.00802 × DBP (mm Hg)] [16].
Statistical analysis
Analyses were performed in SAS (version 9.4 for Windows; SAS Institute, Cary, NC, USA). Variables were checked for the distributional assumption of normality using normal plots. For Table 1, we stratified our participants on whether rapid GFR decline was experienced over 6 years or not. Differences between continuous parametric variables were examined with the t-test and dichotomous with the χ2 test.
Variables . | Rapid GFR decline (>3 mL/min/1.73 m2) . | P-values . | |
---|---|---|---|
Yes (n = 144) . | No (n = 375) . | ||
Age (years) | 34 ± 9 | 37 ± 9 | <0.0001 |
Duration (years) | 21 ± 8 | 24 ± 9 | 0.003 |
Male (%) | 38 | 46 | 0.31 |
HbA1c (%) | 8.0 ± 1.5 | 7.9 ± 1.2 | 0.52 |
SBP (mm Hg) | 115 ± 15 | 117 ± 13 | 0.31 |
LDL-C (mg/dL) | 95 ± 27 | 101 ± 28 | 0.02 |
eIS | 4.4 ± 1.6 | 4.4 ± 1.6 | 0.93 |
ACRa (mg/g) | 11 (9–15) | 9 (8–10) | 0.08 |
CKD-EPI creatinine | 121 ± 20 | 98 ± 25 | <0.0001 |
Renal hyperfiltration (%) (n = 147) | 54 | 18 | <0.0001 |
Incident eGFR < 60 mL/min/1.73 m2 at 6-year follow-up (%) (n = 15) | 9 | 0.6 | <0.0001 |
Variables . | Rapid GFR decline (>3 mL/min/1.73 m2) . | P-values . | |
---|---|---|---|
Yes (n = 144) . | No (n = 375) . | ||
Age (years) | 34 ± 9 | 37 ± 9 | <0.0001 |
Duration (years) | 21 ± 8 | 24 ± 9 | 0.003 |
Male (%) | 38 | 46 | 0.31 |
HbA1c (%) | 8.0 ± 1.5 | 7.9 ± 1.2 | 0.52 |
SBP (mm Hg) | 115 ± 15 | 117 ± 13 | 0.31 |
LDL-C (mg/dL) | 95 ± 27 | 101 ± 28 | 0.02 |
eIS | 4.4 ± 1.6 | 4.4 ± 1.6 | 0.93 |
ACRa (mg/g) | 11 (9–15) | 9 (8–10) | 0.08 |
CKD-EPI creatinine | 121 ± 20 | 98 ± 25 | <0.0001 |
Renal hyperfiltration (%) (n = 147) | 54 | 18 | <0.0001 |
Incident eGFR < 60 mL/min/1.73 m2 at 6-year follow-up (%) (n = 15) | 9 | 0.6 | <0.0001 |
Data are presented as mean ± standard deviation, unless otherwise specified.
aGeometric means ± 95% confidence interval.
Variables . | Rapid GFR decline (>3 mL/min/1.73 m2) . | P-values . | |
---|---|---|---|
Yes (n = 144) . | No (n = 375) . | ||
Age (years) | 34 ± 9 | 37 ± 9 | <0.0001 |
Duration (years) | 21 ± 8 | 24 ± 9 | 0.003 |
Male (%) | 38 | 46 | 0.31 |
HbA1c (%) | 8.0 ± 1.5 | 7.9 ± 1.2 | 0.52 |
SBP (mm Hg) | 115 ± 15 | 117 ± 13 | 0.31 |
LDL-C (mg/dL) | 95 ± 27 | 101 ± 28 | 0.02 |
eIS | 4.4 ± 1.6 | 4.4 ± 1.6 | 0.93 |
ACRa (mg/g) | 11 (9–15) | 9 (8–10) | 0.08 |
CKD-EPI creatinine | 121 ± 20 | 98 ± 25 | <0.0001 |
Renal hyperfiltration (%) (n = 147) | 54 | 18 | <0.0001 |
Incident eGFR < 60 mL/min/1.73 m2 at 6-year follow-up (%) (n = 15) | 9 | 0.6 | <0.0001 |
Variables . | Rapid GFR decline (>3 mL/min/1.73 m2) . | P-values . | |
---|---|---|---|
Yes (n = 144) . | No (n = 375) . | ||
Age (years) | 34 ± 9 | 37 ± 9 | <0.0001 |
Duration (years) | 21 ± 8 | 24 ± 9 | 0.003 |
Male (%) | 38 | 46 | 0.31 |
HbA1c (%) | 8.0 ± 1.5 | 7.9 ± 1.2 | 0.52 |
SBP (mm Hg) | 115 ± 15 | 117 ± 13 | 0.31 |
LDL-C (mg/dL) | 95 ± 27 | 101 ± 28 | 0.02 |
eIS | 4.4 ± 1.6 | 4.4 ± 1.6 | 0.93 |
ACRa (mg/g) | 11 (9–15) | 9 (8–10) | 0.08 |
CKD-EPI creatinine | 121 ± 20 | 98 ± 25 | <0.0001 |
Renal hyperfiltration (%) (n = 147) | 54 | 18 | <0.0001 |
Incident eGFR < 60 mL/min/1.73 m2 at 6-year follow-up (%) (n = 15) | 9 | 0.6 | <0.0001 |
Data are presented as mean ± standard deviation, unless otherwise specified.
aGeometric means ± 95% confidence interval.
To examine the relationships between renal hyperfiltration and rapid GFR decline over 6 years, and rapid GFR decline and incident impaired GFR, we employed multivariable logistic regression models. Variables considered for inclusion in the multivariable models were based on a priori criteria: significance in previous work, significant contribution to the model (P-value < 0.1), or confounding between the main variable of interest and the outcome by >10%. Akaike's information criterion was used for model selection. The following variables were considered for inclusion in the models: HbA1c, SBP, LDL-C, eIS, diabetes duration, sex and natural log of baseline albumin/creatinine ratio (Ln ACR) due to positively skewed distribution. Age is part of the CKD-EPI equations and was not adjusted due to collinearity and concern for over-adjustment. We reran the models with eGFR calculated by CKD-EPI combined creatinine and cystatin C, and with renal hyperfiltration defined as ≥135 mL/min/1.73 m2 as sensitivity analyses. A P-value of <0.05 was considered statistically significant.
RESULTS
Participant characteristics stratified by the presence or absence of rapid GFR decline over 6 years are presented in Table 1, and participant characteristics stratified by renal hyperfiltration status at baseline are presented in Supplementary data, Table S1. Participants with rapid GFR decline over 6 years were significantly more likely to have renal hyperfiltration at baseline and impaired GFR at 6-year follow-up compared with participants without rapid GFR decline (Table 1).
Participants with renal hyperfiltration at baseline experienced a greater mean annual GFR loss compared with those without renal hyperfiltration (ΔeGFR: −3.2 versus −0.4 mL/min/1.73 m2/year, P < 0.0001, Supplementary data, Figures S1 and S2). Similarly, participants who developed impaired GFR at 6-year follow-up experienced a greater mean annual GFR loss than those without incident impaired GFR at 6-year follow-up (ΔeGFR: −7.0 versus −1.1 mL/min/1.73 m2, P < 0.0001). In other words, renal hyperfiltration appears to be succeeded by a rapid annual GFR loss, and incident impaired GFR appears to be preceded by a rapid annual GFR loss (Figures 1 and 2 ).
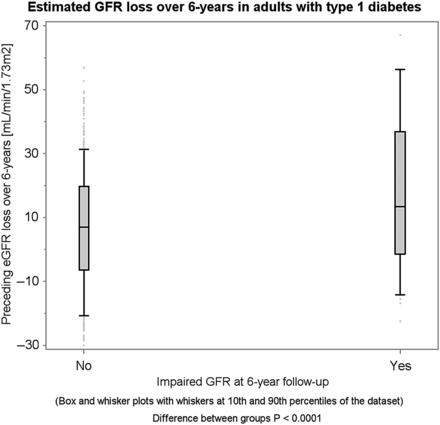
Total eGFR loss over 6 years succeeding renal hyperfiltration at baseline.
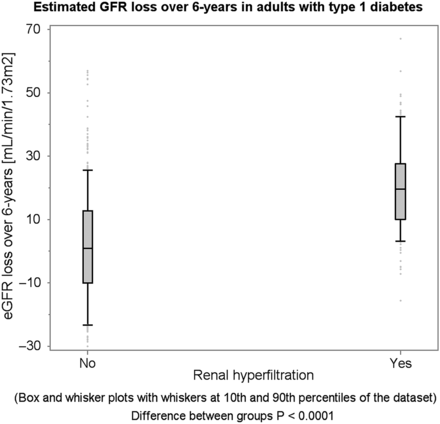
The presence of renal hyperfiltration at baseline was associated with significantly greater odds of rapid GFR decline over 6 years compared with the absence of renal hyperfiltration at baseline (OR: 5.0, 95% CI 3.2–7.8, P < 0.0001) after adjusting for sex and duration. Rapid GFR decline over 6 years was also associated with greater odds of incident impaired GFR at 6-year follow-up (OR 16.5, 95% CI 3.6–75.1, P = 0.0003) after adjusting for sex and duration. The associations between renal hyperfiltration and rapid GFR decline, and between rapid GFR decline and impaired GFR remained significant after adjusting for HbA1c, SBP, LDL-C, sex, duration, natural Ln ACR and eIS (Table 2). As part of sensitivity analyses, we reran models with eGFR calculated by CKD-EPI cystatin C and CKD-EPI combined creatinine and cystatin C equation, and renal hyperfiltration defined as eGFR ≥ 135 mL/min/1.73 m2, and obtained similar associations between renal hyperfiltration and rapid GFR decline, and rapid GFR decline and incident impaired GFR (Supplementary data, Table S2).
Multivariable models predicting rapid GFR decline and incident renal impairment over 6 years
Exposure variables . | Outcome variables . | |
---|---|---|
Rapid GFR decline (>3 mL/min/year) (N = 144) . | Incident impaired GFR (<60 mL/min/1.73 m2) (N = 15) . | |
OR (95% CI) . | OR (95% CI) . | |
HbA1c (per 1%) | 1.07 (0.88–1.29) P = 0.51 | 1.83 (1.09–3.06) P = 0.02 |
SBP (per 1 mm Hg) | 1.00 (0.98–1.02) P = 0.99 | 1.05 (0.99–1.11) P = 0.13 |
LDL-C (per 1 mg/dL) | 0.99 (0.99–1.00) P = 0.15 | 1.01 (0.99, 1.04) P = 0.35 |
Duration (per year) | 0.99 (0.96–1.02) P = 0.45 | 0.96 (0.86–1.08) P = 0.49 |
eIS (per unit) | 1.02 (0.88–1.20) P = 0.83 | 1.40 (0.82–2.40) P = 0.22 |
Ln ACR (per unit) | 1.23 (1.04–1.44) P = 0.01 | 3.04 (1.85–4.99) P < 0.0001 |
Renal hyperfiltration (N = 147) | 5.00 (3.03–8.25) P < 0.0001 | |
Rapid GFR decline (N = 144) | 15.99 (2.34–114.37) P = 0.006 |
Exposure variables . | Outcome variables . | |
---|---|---|
Rapid GFR decline (>3 mL/min/year) (N = 144) . | Incident impaired GFR (<60 mL/min/1.73 m2) (N = 15) . | |
OR (95% CI) . | OR (95% CI) . | |
HbA1c (per 1%) | 1.07 (0.88–1.29) P = 0.51 | 1.83 (1.09–3.06) P = 0.02 |
SBP (per 1 mm Hg) | 1.00 (0.98–1.02) P = 0.99 | 1.05 (0.99–1.11) P = 0.13 |
LDL-C (per 1 mg/dL) | 0.99 (0.99–1.00) P = 0.15 | 1.01 (0.99, 1.04) P = 0.35 |
Duration (per year) | 0.99 (0.96–1.02) P = 0.45 | 0.96 (0.86–1.08) P = 0.49 |
eIS (per unit) | 1.02 (0.88–1.20) P = 0.83 | 1.40 (0.82–2.40) P = 0.22 |
Ln ACR (per unit) | 1.23 (1.04–1.44) P = 0.01 | 3.04 (1.85–4.99) P < 0.0001 |
Renal hyperfiltration (N = 147) | 5.00 (3.03–8.25) P < 0.0001 | |
Rapid GFR decline (N = 144) | 15.99 (2.34–114.37) P = 0.006 |
Data are presented as OR and 95% CI. OR represents the increase in odds of rapid GFR decline and impaired GFR for every unit increase in the independent continuous variable (e.g. HbA1c) or the presence of an independent categorical variable (e.g. renal hyperfiltration or rapid GFR decline).
Multivariable models predicting rapid GFR decline and incident renal impairment over 6 years
Exposure variables . | Outcome variables . | |
---|---|---|
Rapid GFR decline (>3 mL/min/year) (N = 144) . | Incident impaired GFR (<60 mL/min/1.73 m2) (N = 15) . | |
OR (95% CI) . | OR (95% CI) . | |
HbA1c (per 1%) | 1.07 (0.88–1.29) P = 0.51 | 1.83 (1.09–3.06) P = 0.02 |
SBP (per 1 mm Hg) | 1.00 (0.98–1.02) P = 0.99 | 1.05 (0.99–1.11) P = 0.13 |
LDL-C (per 1 mg/dL) | 0.99 (0.99–1.00) P = 0.15 | 1.01 (0.99, 1.04) P = 0.35 |
Duration (per year) | 0.99 (0.96–1.02) P = 0.45 | 0.96 (0.86–1.08) P = 0.49 |
eIS (per unit) | 1.02 (0.88–1.20) P = 0.83 | 1.40 (0.82–2.40) P = 0.22 |
Ln ACR (per unit) | 1.23 (1.04–1.44) P = 0.01 | 3.04 (1.85–4.99) P < 0.0001 |
Renal hyperfiltration (N = 147) | 5.00 (3.03–8.25) P < 0.0001 | |
Rapid GFR decline (N = 144) | 15.99 (2.34–114.37) P = 0.006 |
Exposure variables . | Outcome variables . | |
---|---|---|
Rapid GFR decline (>3 mL/min/year) (N = 144) . | Incident impaired GFR (<60 mL/min/1.73 m2) (N = 15) . | |
OR (95% CI) . | OR (95% CI) . | |
HbA1c (per 1%) | 1.07 (0.88–1.29) P = 0.51 | 1.83 (1.09–3.06) P = 0.02 |
SBP (per 1 mm Hg) | 1.00 (0.98–1.02) P = 0.99 | 1.05 (0.99–1.11) P = 0.13 |
LDL-C (per 1 mg/dL) | 0.99 (0.99–1.00) P = 0.15 | 1.01 (0.99, 1.04) P = 0.35 |
Duration (per year) | 0.99 (0.96–1.02) P = 0.45 | 0.96 (0.86–1.08) P = 0.49 |
eIS (per unit) | 1.02 (0.88–1.20) P = 0.83 | 1.40 (0.82–2.40) P = 0.22 |
Ln ACR (per unit) | 1.23 (1.04–1.44) P = 0.01 | 3.04 (1.85–4.99) P < 0.0001 |
Renal hyperfiltration (N = 147) | 5.00 (3.03–8.25) P < 0.0001 | |
Rapid GFR decline (N = 144) | 15.99 (2.34–114.37) P = 0.006 |
Data are presented as OR and 95% CI. OR represents the increase in odds of rapid GFR decline and impaired GFR for every unit increase in the independent continuous variable (e.g. HbA1c) or the presence of an independent categorical variable (e.g. renal hyperfiltration or rapid GFR decline).
DISCUSSION
Renal hyperfiltration at baseline predicted greater odds of rapid GFR decline over 6 years, and rapid GFR decline was associated with incident impaired GFR at 6-year follow-up in adults with Type 1 diabetes, independent of other important covariates. These findings support the role of rapid GFR decline as an intermediate phenotype in the progression of DN in adults with Type 1 diabetes.
DN continues to cause major morbidity and mortality in Type 1 diabetes [1, 17]. Treatment exists to prevent or slow decline in renal function, including intensive glucose [18, 19] and blood pressure control [13, 14]; however, improved diagnostic methods are needed to better identify who is at risk to lose renal function at an early stage [20]. It is now clear that albuminuria is not a sensitive marker of early kidney injury in Type 1 diabetes [21]. There are strong data supporting GFR as a more clinically relevant measure of early DN in patients with Type 1 diabetes. GFR declines with age, and an annual loss of 1 mL/min/1.73 m2 is described in studies of normal aging [22]. Rapid GFR decline, defined as an annual GFR loss of >3 mL/min/1.73 m2, represents a magnitude of change three times the rate of what is expected in normal physiology and corresponded to 25% of the cohort of the Cardiovascular Health Study with the largest decline in GFR [23, 24]. The cutoff is important to distinguish it from slow changes in GFR associated with aging, and beyond the range of noise expected when estimating GFR [23]. Rapid GFR decline has been shown to predict end-stage renal disease and cardiovascular outcomes [2, 25].
Renal hyperfiltration, another preclinical phenotype of DN, is also associated with early cardiovascular abnormalities [26, 27]. The mechanisms responsible for renal hyperfiltration in diabetes are not completely understood but are likely due to a combination of glomerular hemodynamic, vasoactive, tubular, growth promoting and metabolic factors that together increase intraglomerular pressure, leading to structural changes including mesangial expansion and glomerular basement membrane thickening [28, 29]. Previous studies have for the most part supported a role for hyperfiltration in the initiation and progression of albuminuria in Type 1 diabetes [10]. Similarly, in patients with Type 2 diabetes and GFR measured by iohexol clearance, hyperfiltration was associated with an increased risk of proteinuria and GFR decline over a 6-year period [9]. To our knowledge, the report herein is the first to demonstrate a strong relationship between renal hyperfiltration and rapid GFR decline, defined as an annual GFR loss of >3 mL/min/1.73 m2, independent of ACR and other important covariates in adults with Type 1 diabetes.
GFR is hypothesized to fall progressively after renal hyperfiltration and may lead, in the long run, to end-stage renal disease [9]. Early rapid GFR decline has also been shown to precede the onset of microalbuminuria in Type 1 diabetes [30]. The mechanisms that initiate renal GFR decline in Type 1 diabetes remain controversial but likely include both renal arteriolar and glomerular functional and structural effects due to hyperglycemia, hypertension, dyslipidemia and smoking. Also uncertain is whether those initial mechanisms remain operative as renal function decline progresses through advanced CKD stages. It is possible that early rapid GFR decline (in the range of normal renal function) is related to functional changes and that the late rapid GFR decline (CKD Stages 3–5) is due to morphological injuries. However, data from the Joslin Kidney Study demonstrated similar rates of GFR loss during early and late phases of rapid GFR decline [30], which may suggest similar underlying mechanisms.
In this study, we similarly demonstrated in the Type 1 diabetes cohort that renal hyperfiltration at baseline predicted rapid GFR decline over 6 years and that rapid GFR decline in turn was strongly associated with incident impaired GFR, suggesting a possible role for rapid GFR decline as an intermediate phenotype between renal hyperfiltration and incident impaired GFR in adults with Type 1 diabetes. Beyond known effects of ACE inhibition on hyperfiltration [31], data in animals and in patients with Type 1 diabetes suggest that sodium–glucose co-transporter 2 (SGLT-2) inhibitors may reverse renal hyperfiltration by counteracting tubuloglomerular feedback pathways [32, 33]. Whether reversal of renal hyperfiltration by SGLT-2 inhibitors can prevent rapid GFR decline and incident impaired GFR remains unclear but warrants further study [33].
There are important limitations to the present study worth mentioning, including the observational design and the absence of a direct measurement of GFR. A direct measure of GFR would have been too cumbersome for use in a large-scale clinical study like CACTI, and improved methods to detect early kidney changes are needed [34]. We employed the state-of-the-art CKD-EPI equations [14], recently used by studies such as the DCCT–EDIC [19], but it is established that eGFR at higher levels is associated with greater variability, which could lead to misclassification of hyperfiltration and normofiltration, and bias our study to not find an effect. There is no generally accepted definition of renal hyperfiltration in Type 1 diabetes, but it is generally defined as between 120 and 150 mL/min/1.73 m2. Our 6-year longitudinal data are unable to demonstrate a one-way progression from renal hyperfiltration to impaired GFR with only three participants with renal hyperfiltration at baseline experiencing incident impaired GFR at 6-year follow-up.
The challenge in carrying out studies to establish a relationship between renal hyperfiltration rapid GFR decline and impaired GFR is the long duration between renal hyperfiltration and impaired GFR, which could span decades. In the current analysis, we have shown important temporal relationships between renal hyperfiltration and rapid GFR decline, and rapid GFR decline and impaired GFR, suggesting a sequential progression from renal hyperfiltration to impaired GFR via rapid GFR decline. Our hypothesis generating data suggest that future research should examine the relationships between renal hyperfiltration, rapid GFR decline and impaired GFR in adults with Type 1 diabetes over a longer time period to establish the sequence of progression.
Understanding the mechanisms underlying the progression from renal hyperfiltration to rapid GFR decline will help in stratifying patients according to risk of impaired GFR and direct development of therapies to prevent or delay rapid GFR loss. Longitudinal GFR data are needed to examine whether prevention of rapid GFR loss at an early stage can prevent the development of impaired GFR and end-stage renal disease in Type 1 diabetes.
In summary, we report a strong association between rapid GFR decline over 6 years and incident impaired GFR in adults with Type 1 diabetes. Moreover, baseline hyperfiltration was associated with rapid GFR decline. These epidemiologic observations support the role of rapid GFR decline as an important high-risk phenotype of DN in adults with Type 1 diabetes. Further studies are needed to elucidate the pathophysiologic mechanisms underlying the associations between renal hyperfiltration, rapid GFR decline and incident impaired GFR, and whether halting rapid GFR decline will prevent progression of DN.
AUTHOR CONTRIBUTIONS
P.B. researched, wrote, contributed to discussion and reviewed/edited the manuscript; D.Z.C. researched, contributed to the discussion and reviewed/edited the manuscript; L.P. researched, performed the statistical analyses, contributed to the discussion and reviewed/edited the manuscript; J.K.S.B. researched, contributed to the discussion and reviewed/edited the manuscript; M.R. designed the CACTI study, researched, contributed to the discussion and reviewed/edited the manuscript; R.J.J. researched, contributed to the discussion and reviewed/edited the manuscript; D.M.M. researched, contributed to discussion and reviewed/edited the manuscript.
CONFLICT OF INTEREST STATEMENT
P.B. and L.P. are the guarantors of this work and, as such, had full access to all the data in the study and take responsibility for the integrity of the data and the accuracy of the data analysis.
ACKNOWLEDGEMENTS
Support for this study was provided by NHLBI grant R01 HL61753, HL79611, DERC Clinical Investigation Core P30 DK57516 and JDRF grant 17-2013-313. The study was performed at the Adult CTRC at UCD support by NIH-M01-RR00051, at the Barbara Davis Center for Childhood Diabetes and at Colorado Heart Imaging Center in Denver, CO. J.K.S.-B. was supported by an American Diabetes Association Career Development Award (7-13-CD-10). D.Z.I.C. was supported by a Kidney Foundation of Canada Scholarship and a Canadian Diabetes Association-KRESCENT Program Joint New Investigator Award. P.B., D.C., L.P., J.K.S.-B., M.R. and D.M.M. have no conflict of interest to disclose.
REFERENCES
Comments