-
PDF
- Split View
-
Views
-
Cite
Cite
Wendy N. Jefferson, Dominique M. Chevalier, Jazma Y. Phelps, Amy M. Cantor, Elizabeth Padilla-Banks, Retha R. Newbold, Trevor K. Archer, H. Karimi Kinyamu, Carmen J. Williams, Persistently Altered Epigenetic Marks in the Mouse Uterus After Neonatal Estrogen Exposure, Molecular Endocrinology, Volume 27, Issue 10, 1 October 2013, Pages 1666–1677, https://doi.org/10.1210/me.2013-1211
- Share Icon Share
Neonatal exposure to diethylstilbestrol (DES) causes permanent alterations in female reproductive tract gene expression, infertility, and uterine cancer in mice. To determine whether epigenetic mechanisms could explain these phenotypes, we first tested whether DES altered uterine expression of chromatin-modifying proteins. DES treatment significantly reduced expression of methylcytosine dioxygenase TET oncogene family, member 1 (TET1) on postnatal day 5; this decrease was correlated with a subtle decrease in DNA 5-hydroxymethylcytosine in adults. There were also significant reductions in histone methyltransferase enhancer of zeste homolog 2 (EZH2), histone lysine acetyltransferase 2A (KAT2A), and histone deacetylases HDAC1, HDAC2, and HDAC3. Uterine chromatin immunoprecipitation was used to analyze the locus-specific association of modified histones with 2 genes, lactoferrin (Ltf) and sine oculis homeobox 1 (Six1), which are permanently upregulated in adults after neonatal DES treatment. Three histone modifications associated with active transcription, histone H3 lysine 9 acetylation (H3K9ac), H3 lysine 4 trimethylation (H3K4me3), and H4 lysine 5 acetylation (H4K5ac) were enriched at specific Ltf promoter regions after DES treatment, but this enrichment was not maintained in adults. H3K9ac, H4K5ac, and H3K4me3 were enriched at Six1 exon 1 immediately after neonatal DES treatment. As adults, DES-treated mice had greater differences in H4K5ac and H3K4me3 occupancy at Six1 exon 1 and new differences in these histone marks at an upstream region. These findings indicate that neonatal DES exposure temporarily alters expression of multiple chromatin-modifying proteins and persistently alters epigenetic marks in the adult uterus at the Six1 locus, suggesting a mechanism for developmental exposures leading to altered reproductive function and increased cancer risk.
Endocrine-disrupting chemicals (EDCs) are defined by their abilities to interfere with normal hormonal or homeostatic physiological functions (reviewed in Refs. 1–3). In a classic example of the developmental origins of health and disease, manifestations of EDC exposures are often observed at times remote from the actual exposure (4). In particular, exposures to EDCs during periods of organ development frequently have significant effects that are not evident until adulthood and that differ from the effects observed after an adult exposure to the same EDC (5). One important example in humans of this latency of EDC effects is provided by the increased incidence of reproductive dysfunction and vaginal cancer in adult women exposed to diethylstilbestrol (DES) while they were fetuses (6).
The occurrence of latent EDC effects strongly suggests that permanent epigenetic alterations occur in response to EDC exposure during critical periods of differentiation and are directly responsible for phenotypic changes later in life. Epigenetic changes could increase susceptibility to a second-hit exposure that leads to an altered phenotypic outcome. Almost all mice exposed neonatally to DES develop uterine cancer by 12 to 18 months of age; this effect is estrogen receptor α-dependent and is also dependent on the second hit of estrogen exposure that occurs during puberty (7, 8). The occurrence of uterine cancer may be explained by the observation that neonatal DES exposure permanently alters the expression patterns of many uterine genes (9–13), and these altered genes could promote oncogenesis when additional estrogen exposure provides a permissive environment.
Epigenetic information is largely provided by components of chromatin including DNA methylation and histone modifications. DNA methylation is an important epigenetic modification involved in regulating gene expression by enhancing or repressing transcriptional activity at specific gene promoters during development and oncogenesis (14, 15). Several genes whose expression is altered after DES treatment have subtle changes in DNA methylation in the promoter or other gene regions (13, 16). In the case of 2 of these altered genes, lactoferrin (Ltf) and FBJ osteosarcoma oncogene (Fos), the methylation patterns remain altered throughout life (17, 18). However, it is not clear how the observed alterations in DNA methylation relate to the altered gene expression, and there is limited information available regarding how DES exposure leads to site-specific alterations in DNA methylation.
Three histone H3 lysine methylation states, H3K9me, H3K27me, and H3K4me, are likely candidates for mediating epigenetic regulation. H3K4me3 close to transcriptional start sites is strongly associated with transcriptionally active genes, whereas H3K9me and H3K27me3 are generally associated with gene repression. H3K27me3 in combination with H3K4me3 may mark genes that are poised for transcriptional activation or repression (19, 20). Histone modifications regulate classic epigenetic signals, including positional variegation and heterochromatin spreading (H3K9me), X-chromosome inactivation (H3K27me), and lineage specification during development (H3K4me and H3K27me) (21–23). Protein complexes that establish and maintain these marks are attractive candidates as epigenetic regulators. For example, the H3K9 methyltransferase euchromatic histone-lysine N-methyltransferase 2 (EHMT2) interacts with DNA methyltransferase I (DNMT1) and localizes to the DNA replication fork, suggesting a role in mitotic inheritance and hence supporting a function for the H3K9me mark in epigenetic regulation (24). These protein complexes mediate epigenetic inheritance of silent and active chromatin states in development and have been implicated in cell proliferation, stem cell fate, and cancer, genomic programs that are primarily regulated by epigenetic mechanisms (23, 25–29).
With this in mind, we set out to explore the molecular mechanisms underlying estrogenic EDC-induced alterations in uterine gene expression. We used the well-characterized mouse model of neonatal DES exposure to determine whether this treatment causes global alterations in expression of proteins with documented roles in modifying the epigenome. In addition, we used uterine tissue chromatin immunoprecipitation (ChIP) methods to test whether neonatal DES exposure persistently alters the site-specific occupancy of modified histones H3 and H4 at specific regions of 2 genes, Ltf and sine oculis-related homeobox 1 (Six1), whose expression is permanently altered in adult mice in response to this treatment.
Materials and Methods
Animals
Animals were handled according to National Institutes of Health National Institute of Environmental Health Sciences guidelines under approved animal care and use protocols. Timed pregnant CD-1 mice were obtained from the in-house breeding colony at National Institute of Environmental Health Sciences, National Institutes of Health; housed in a temperature-controlled environment (21°C–22°C) under a 12-hour light,12-hour dark cycle; and fed an NIH-31 diet. At delivery, pups were randomly standardized to 10 female pups per dam. All female pups were treated by sc injections (0.02 mL) on the day of birth (postnatal day 1 [PND1]) through PND5 with either DES at 1 mg/kg/d dissolved in corn oil or with corn oil alone (controls) as described previously (7, 30). Female pups were killed on PND5 at 4 hours after the last injection or were weaned on PND21 and allowed to age to 8 weeks. At 8 weeks, mice were ovariectomized (ovx) to eliminate confounding effects of variations in estrogen levels during the estrous cycle, and tissues were collected 10 to 14 days later. One set of ovx mice was given 25 μg estradiol (Sigma) in 100 μL corn oil, and tissues were collected 24 hours later. At all time points, uteri were collected, frozen on dry ice, and stored at −80°C until further use.
Immunoblots
Cytoplasmic and nuclear protein fractions were isolated from the uterus of individual mice using the NE-PER kit (Thermo Scientific) following the manufacturer's instructions. Nuclear proteins (10 μg) were run on 4% to 12% or 6% Tris-glycine mini gels (Invitrogen) and transferred to polyvinylidene difluoride membrane (Invitrogen). Blots were blocked with 5% milk in Tris-buffered saline containing 0.1% Tween 20 (TBST) and then incubated overnight at 4°C with primary antibodies in 5% milk in TBST. After washes with TBST, the appropriate secondary antibody was applied at a dilution of 1:25 000 in TBST for 1 hour at room temperature. After washing, the blots were incubated with SuperSignal West Femto chemiluminescent substrate (Thermo Scientific) and then exposed to film. Antibodies and dilutions are listed in Table 1.
Purpose . | Antibody . | Source (Catalog No.), Dilution . |
---|---|---|
Immunoblots | Actin | Santa Cruz (sc-47778), 1:500 |
DNMT1 | Upstate (07-688), 1:500 | |
DNMT3A | Abcam (ab23565), 1:200 | |
EHMT2 | Cell Signaling (3306), 1:1000 | |
EZH2 | Active Motif (39933), 1:1000 | |
HDAC1 | Santa Cruz (sc-7872), 1:1000 | |
HDAC2 | Santa Cruz (sc-7899), 1:1000 | |
HDAC3 | Santa Cruz (sc-11417), 1:1000 | |
KAT2A | Santa Cruz (sc-20698), 1:500 | |
Lamin A/C | Santa Cruz (sc-20681), 1:1000 | |
SETDB1b | Upstate (07-378), 1:500 | |
SUZ12 | Upstate (07-379), 1:1000 | |
TET1 | GeneTex (GTX124207), 1:1000 | |
ChIP/histone immunoblotsa | Histone H3K4me3 | Active Motif (39159) |
Histone H3K9me2 | Millipore (07-441) | |
Histone H3K27me3 | Active Motif (39155) | |
Histone H3 acetyl | Millipore (04-928) | |
Histone H4 acetyl | Millipore (06-866) | |
Histone H3K9 acetyl | Millipore (07-352) | |
Histone H4K5 acetyl | Active Motif (39583) | |
Normal rabbit serum | Santa Cruz (sc-2027) | |
Secondary | Peroxidase antimouse | Jackson ImmunoResearch (115-035-174) |
Peroxidase antirabbit | Jackson ImmunoResearch (711-035-152) |
Purpose . | Antibody . | Source (Catalog No.), Dilution . |
---|---|---|
Immunoblots | Actin | Santa Cruz (sc-47778), 1:500 |
DNMT1 | Upstate (07-688), 1:500 | |
DNMT3A | Abcam (ab23565), 1:200 | |
EHMT2 | Cell Signaling (3306), 1:1000 | |
EZH2 | Active Motif (39933), 1:1000 | |
HDAC1 | Santa Cruz (sc-7872), 1:1000 | |
HDAC2 | Santa Cruz (sc-7899), 1:1000 | |
HDAC3 | Santa Cruz (sc-11417), 1:1000 | |
KAT2A | Santa Cruz (sc-20698), 1:500 | |
Lamin A/C | Santa Cruz (sc-20681), 1:1000 | |
SETDB1b | Upstate (07-378), 1:500 | |
SUZ12 | Upstate (07-379), 1:1000 | |
TET1 | GeneTex (GTX124207), 1:1000 | |
ChIP/histone immunoblotsa | Histone H3K4me3 | Active Motif (39159) |
Histone H3K9me2 | Millipore (07-441) | |
Histone H3K27me3 | Active Motif (39155) | |
Histone H3 acetyl | Millipore (04-928) | |
Histone H4 acetyl | Millipore (06-866) | |
Histone H3K9 acetyl | Millipore (07-352) | |
Histone H4K5 acetyl | Active Motif (39583) | |
Normal rabbit serum | Santa Cruz (sc-2027) | |
Secondary | Peroxidase antimouse | Jackson ImmunoResearch (115-035-174) |
Peroxidase antirabbit | Jackson ImmunoResearch (711-035-152) |
All ChIP/histone antibodies were used at 5 μg/sample for ChIP and 1:1000 for immunoblots.
SETDB1, SET domain protein, bifurcated 1.
Purpose . | Antibody . | Source (Catalog No.), Dilution . |
---|---|---|
Immunoblots | Actin | Santa Cruz (sc-47778), 1:500 |
DNMT1 | Upstate (07-688), 1:500 | |
DNMT3A | Abcam (ab23565), 1:200 | |
EHMT2 | Cell Signaling (3306), 1:1000 | |
EZH2 | Active Motif (39933), 1:1000 | |
HDAC1 | Santa Cruz (sc-7872), 1:1000 | |
HDAC2 | Santa Cruz (sc-7899), 1:1000 | |
HDAC3 | Santa Cruz (sc-11417), 1:1000 | |
KAT2A | Santa Cruz (sc-20698), 1:500 | |
Lamin A/C | Santa Cruz (sc-20681), 1:1000 | |
SETDB1b | Upstate (07-378), 1:500 | |
SUZ12 | Upstate (07-379), 1:1000 | |
TET1 | GeneTex (GTX124207), 1:1000 | |
ChIP/histone immunoblotsa | Histone H3K4me3 | Active Motif (39159) |
Histone H3K9me2 | Millipore (07-441) | |
Histone H3K27me3 | Active Motif (39155) | |
Histone H3 acetyl | Millipore (04-928) | |
Histone H4 acetyl | Millipore (06-866) | |
Histone H3K9 acetyl | Millipore (07-352) | |
Histone H4K5 acetyl | Active Motif (39583) | |
Normal rabbit serum | Santa Cruz (sc-2027) | |
Secondary | Peroxidase antimouse | Jackson ImmunoResearch (115-035-174) |
Peroxidase antirabbit | Jackson ImmunoResearch (711-035-152) |
Purpose . | Antibody . | Source (Catalog No.), Dilution . |
---|---|---|
Immunoblots | Actin | Santa Cruz (sc-47778), 1:500 |
DNMT1 | Upstate (07-688), 1:500 | |
DNMT3A | Abcam (ab23565), 1:200 | |
EHMT2 | Cell Signaling (3306), 1:1000 | |
EZH2 | Active Motif (39933), 1:1000 | |
HDAC1 | Santa Cruz (sc-7872), 1:1000 | |
HDAC2 | Santa Cruz (sc-7899), 1:1000 | |
HDAC3 | Santa Cruz (sc-11417), 1:1000 | |
KAT2A | Santa Cruz (sc-20698), 1:500 | |
Lamin A/C | Santa Cruz (sc-20681), 1:1000 | |
SETDB1b | Upstate (07-378), 1:500 | |
SUZ12 | Upstate (07-379), 1:1000 | |
TET1 | GeneTex (GTX124207), 1:1000 | |
ChIP/histone immunoblotsa | Histone H3K4me3 | Active Motif (39159) |
Histone H3K9me2 | Millipore (07-441) | |
Histone H3K27me3 | Active Motif (39155) | |
Histone H3 acetyl | Millipore (04-928) | |
Histone H4 acetyl | Millipore (06-866) | |
Histone H3K9 acetyl | Millipore (07-352) | |
Histone H4K5 acetyl | Active Motif (39583) | |
Normal rabbit serum | Santa Cruz (sc-2027) | |
Secondary | Peroxidase antimouse | Jackson ImmunoResearch (115-035-174) |
Peroxidase antirabbit | Jackson ImmunoResearch (711-035-152) |
All ChIP/histone antibodies were used at 5 μg/sample for ChIP and 1:1000 for immunoblots.
SETDB1, SET domain protein, bifurcated 1.
Histone extraction and immunoblotting
Histones were isolated from the uterus on PND5 (2 uteri per sample) using the histone purification kit (Active Motif) following the manufacturer's instructions. Isolated histones (2 μg) were resolved on 16% Tris-glycine mini gels (Invitrogen) and transferred to polyvinylidene difluoride membrane (Invitrogen) (n = 4 samples per group). Immunoblots were performed as described above. Total histones were detected using GelCode blue stain reagent (Thermo Scientific) on duplicate gels to document equal protein loading. Antibodies and dilutions are listed in Table 1.
Real-time RT-PCR
Total RNA was isolated from the uterus of individual mice (PND5 or ovx adult) using the RNeasy kit and ribonuclease-free deoxyribonuclease set (QIAGEN) following the manufacturer's instructions. Total RNA (1 μg) was reverse transcribed using the first-strand synthesis system (Invitrogen) following the manufacturer's instructions. Real-time RT-PCR was performed using 2 ng cDNA, forward and reverse primers (5 pmol each), and SYBR Green PCR master mix (Applied Biosystems) in a 25-μL reaction volume. Amplification was carried out using an ABI Prism 7900 sequence detection system and analysis software (Applied Biosystems). Primers were designed using Primer3 (31) and are listed in Table 2. Amplification was carried out as follows: 50°C for 2 minutes, 95°C for 10 minutes, and then 40 cycles of 95°C for 15 seconds and 60°C for 30 seconds. Expression levels were calculated using the equation 2−(Ct[gene]−Ct[Ppia] × 10 000 (32), where Ct is cycle threshold, and were normalized to Ppia (cyclophilin A).
Gene . | PCR Primer Name . | Chromosome Location . | Distance From Transcription Start Site . |
---|---|---|---|
Ltf | Distal | 9:111 016 273 | −3019 |
CpG | 9:111 018 750 | −542 | |
ERE | 9:111 018 929 | −363 | |
Six1 | Distal - 1 | 12:73 058 616 | −12 750 |
GC-rich upstream-1 | 12:73 052 443 | −6597 | |
GC-rich upstream-2 | 12:73 051 946 | −6080 | |
NonGC-rich upstream-1 | 12:73 041 597 | −4269 | |
Exon1-intron1–1 | 12:73 047 050 | +129 | |
Exon1-intron1–2 | 12:73 046 207 | +972 | |
Exon1-intron1–3 | 12:73 045 409 | +1770 | |
Exon1-intron1–4 | 12:73 045 275 | +1904 | |
Exon1-intron1–5 | 12:73 044 932 | +2247 | |
Exon1-intron1–6 | 12:73 044 576 | +2603 | |
Exon1-intron1–7 | 12:73 044 292 | +2887 |
Gene . | PCR Primer Name . | Chromosome Location . | Distance From Transcription Start Site . |
---|---|---|---|
Ltf | Distal | 9:111 016 273 | −3019 |
CpG | 9:111 018 750 | −542 | |
ERE | 9:111 018 929 | −363 | |
Six1 | Distal - 1 | 12:73 058 616 | −12 750 |
GC-rich upstream-1 | 12:73 052 443 | −6597 | |
GC-rich upstream-2 | 12:73 051 946 | −6080 | |
NonGC-rich upstream-1 | 12:73 041 597 | −4269 | |
Exon1-intron1–1 | 12:73 047 050 | +129 | |
Exon1-intron1–2 | 12:73 046 207 | +972 | |
Exon1-intron1–3 | 12:73 045 409 | +1770 | |
Exon1-intron1–4 | 12:73 045 275 | +1904 | |
Exon1-intron1–5 | 12:73 044 932 | +2247 | |
Exon1-intron1–6 | 12:73 044 576 | +2603 | |
Exon1-intron1–7 | 12:73 044 292 | +2887 |
Gene . | PCR Primer Name . | Chromosome Location . | Distance From Transcription Start Site . |
---|---|---|---|
Ltf | Distal | 9:111 016 273 | −3019 |
CpG | 9:111 018 750 | −542 | |
ERE | 9:111 018 929 | −363 | |
Six1 | Distal - 1 | 12:73 058 616 | −12 750 |
GC-rich upstream-1 | 12:73 052 443 | −6597 | |
GC-rich upstream-2 | 12:73 051 946 | −6080 | |
NonGC-rich upstream-1 | 12:73 041 597 | −4269 | |
Exon1-intron1–1 | 12:73 047 050 | +129 | |
Exon1-intron1–2 | 12:73 046 207 | +972 | |
Exon1-intron1–3 | 12:73 045 409 | +1770 | |
Exon1-intron1–4 | 12:73 045 275 | +1904 | |
Exon1-intron1–5 | 12:73 044 932 | +2247 | |
Exon1-intron1–6 | 12:73 044 576 | +2603 | |
Exon1-intron1–7 | 12:73 044 292 | +2887 |
Gene . | PCR Primer Name . | Chromosome Location . | Distance From Transcription Start Site . |
---|---|---|---|
Ltf | Distal | 9:111 016 273 | −3019 |
CpG | 9:111 018 750 | −542 | |
ERE | 9:111 018 929 | −363 | |
Six1 | Distal - 1 | 12:73 058 616 | −12 750 |
GC-rich upstream-1 | 12:73 052 443 | −6597 | |
GC-rich upstream-2 | 12:73 051 946 | −6080 | |
NonGC-rich upstream-1 | 12:73 041 597 | −4269 | |
Exon1-intron1–1 | 12:73 047 050 | +129 | |
Exon1-intron1–2 | 12:73 046 207 | +972 | |
Exon1-intron1–3 | 12:73 045 409 | +1770 | |
Exon1-intron1–4 | 12:73 045 275 | +1904 | |
Exon1-intron1–5 | 12:73 044 932 | +2247 | |
Exon1-intron1–6 | 12:73 044 576 | +2603 | |
Exon1-intron1–7 | 12:73 044 292 | +2887 |
Immunohistochemistry
Uterine tissues were collected from adult control and DES-treated intact adult mice (n = 4 per group), fixed in cold 10% neutral buffered formalin overnight, and then changed to cold 70% ethanol. Tissues were embedded in paraffin and sectioned at 5 to 6 μm. Formalin-fixed paraffin-embedded tissues were deparaffinized and rehydrated through graded alcohols. Endogenous peroxidase activity was quenched with 3% H2O2 followed by heat-induced antigen retrieval in citrate buffer (pH 6.0). Nonspecific binding was blocked with the avidin/biotin blocking kit (Vector Laboratories) and 10% normal donkey serum (Jackson Immunoresearch). Sections were incubated with the sine oculis-related homeobox 1 (SIX1) antibody (HPA001893; Sigma-Aldrich) or ChromPure rabbit IgG control serum (Jackson Immunoresearch) as a negative control at 1:500 for 1 hour at room temperature. Sections were then incubated with biotinylated donkey antirabbit IgG (Jackson Immunoresearch) at a 1:500 dilution. The antigen-antibody complex was detected using the Vectastain Elite ABC R.T.U. label (Vector Laboratories) and 3,3-diaminobenzidine (Dako). Slides were then counterstained with hematoxylin, dehydrated, and coverslipped.
Chromatin immunoprecipitation-PCR
Uteri were pooled together (4–6 per sample for PND5 and 2 uterine horns per sample for ovx adults) and pulverized on dry ice. Proteins were fixed to chromatin with 0.5% formaldehyde in 1 mL PBS for 5 minutes at room temperature and quenched by addition of 0.125M glycine. Fixative was removed, and the samples were rinsed twice with cold PBS containing protease and phosphatase inhibitor cocktail (Sigma). Samples were homogenized in 50mM Tris, 1% Nonidet P-40, 0.25% deoxycholic acid, 1mM EDTA, and inhibitors as indicated above and centrifuged at 12 000 rpm for 5 minutes at 4°C. Samples were resuspended in lysis buffer (1% sodium dodecyl sulfate, 50mM Tris [pH 8.1], and 10mM EDTA) and incubated for 15 minutes on ice. Samples were then sonicated using a Bioruptor (Diagenode) at the highest setting for 5.5 minutes (30 seconds on and 30 seconds off), and the chromatin fraction cleared by centrifugation at 12 000 rpm for 10 minutes at 4°C. Samples were diluted 10-fold with homogenization-immunoprecipitation buffer (HIP buffer; 50mM Tris, 150mM NaCl, and 1% Triton X-100). The chromatin was precleared by incubation with HIP buffer containing 1 mg/mL protein A-Sepharose (PAS) (GE Healthcare) and 1 mg/mL salmon sperm DNA (Stratagene) for 30 minutes at 4°C followed by centrifugation at 1000 rpm for 2 minutes. The supernatant was transferred to fresh microfuge tubes, the appropriate antibody was added, and the samples were incubated on a rotating platform for 2 hours at room temperature (antibodies used for ChIP are detailed in Table 1). Normal rabbit serum was used as a negative control for each experiment, and a small aliquot (10%) of the supernatant was used as input for each sample. Immunocomplexes were captured by adding PAS followed by incubation on a rotating platform for 1 hour at 4°C. The PAS was collected by centrifugation at 1000 rpm for 2 minutes and then washed 3 times for 3 minutes each with HIP buffer. Chromatin immunocomplexes were eluted using 200 μL elution buffer (1% SDS and 0.1M NaHCO3). Captured DNA and input DNA were reverse cross-linked by adding 5M NaCl to a final concentration of 0.25M and incubating overnight at 65°C. Residual proteins were digested by incubation in 250 μg/mL proteinase K, 0.025mM EDTA, and 100mM Tris (pH 8.0) for 3 hours at 45°C for 3 hours. Captured DNA and input DNA were isolated using the QIAquick PCR purification kit (QIAGEN). Real-time RT-PCR was performed using primers designed to amplify regions of the Ltf and Six1 genes (primers listed in Table 2). Mouse DNA was used to generate a standard curve for each set of primers.
Global DNA methylation and hydroxymethylation ELISAs
DNA was isolated from PND5 (2 uteri per sample) or ovx adults (2 uterine horns per sample) using the DNeasy blood and tissue kit (QIAGEN) following the manufacturer's instructions; RNA was removed using ribonuclease A (Sigma). The level of 5′-methyl-2′-deoxycytidine (5mC) was measured using the global DNA methylation ELISA (Cell Biolabs) following the manufacturer's instructions. Briefly, 2.5 μg DNA was denatured, completely digested to single nucleosides, and then added to the ELISA plate in duplicate. A standard curve was generated from 5mC standards ranging from 0nM to 10 000nM; sample concentrations were calculated after absorbance measurements at 450 nm. Readings were then converted to nanograms of 5mC per microgram sample. The level of 5-hydroxymethylcytosine (5hmC) was measured using the global hydroxymethylation ELISA kit (Cell Biolabs) following the manufacturer's instructions. Briefly, 50 to 200 ng DNA was added to the ELISA plate in duplicate. A standard curve was generated from 5hmC standards ranging from 0 to 80 ng; sample concentrations were calculated after absorbance measurements at 450 nm.
Statistical analysis
Immunoblot signals were quantified relative to the signal in the loading control blot using ImageJ software (33). Data were analyzed using GraphPad Prism version 5.0c (GraphPad Software, Inc). Unpaired t tests were used to compare control and DES-treated samples for immunoblot signals, real-time RT-PCR data, and ChIP data.
Results
DES induces global alterations in DNA 5hmC levels
To test whether DES caused global alterations in uterine expression of proteins that regulate DNA methylation, mRNA expression levels of DNA methyltransferases (Dnmt1, Dnmt3a, and Dnmt3b), methylcytosine dioxygenases (Tet1, Tet2, and Tet3), and thymine-DNA glycosylase (Tdg) were determined in control and DES-treated mice. On PND5, Dnmt1 and Dnmt3a expression was significantly reduced and Dnmt3b expression was not different (Figure 1A). Tet1 expression was dramatically reduced, whereas Tet2 and Tdg expression levels were reduced to a lesser extent (Figure 1B). At the protein level, there was no change in DNMT1 or DNMT3A, suggesting that these proteins are relatively stable. There was ∼60% reduction in TET oncogene family, member 1 (TET1) protein (P < .05) after DES treatment as compared with controls, consistent with the observed decrease in mRNA (Figure 1, B and D). In ovx DES-treated adults, TET1 was reduced by ∼30% but was not statistically different from controls (Figure 1E). These overall alterations in expression of regulators of DNA methylation suggested that global alterations in DNA methylation resulted from DES treatment. DNA cytosine methylation was assessed in uteri of PND5 and adult ovx females using ELISAs to quantify total 5mC and 5hmC. Both 5mC and 5hmC levels increased significantly between PND5 and adult time points regardless of treatment group (Figure 1, F and G). DES treatment did not alter 5mC or 5hmC levels on PND5, but there was a small but significant decrease in 5hmC in neonatal DES-treated adults as compared with controls (Figure 1G). This decrease in 5hmC is consistent with the observed reductions in TET1 protein.
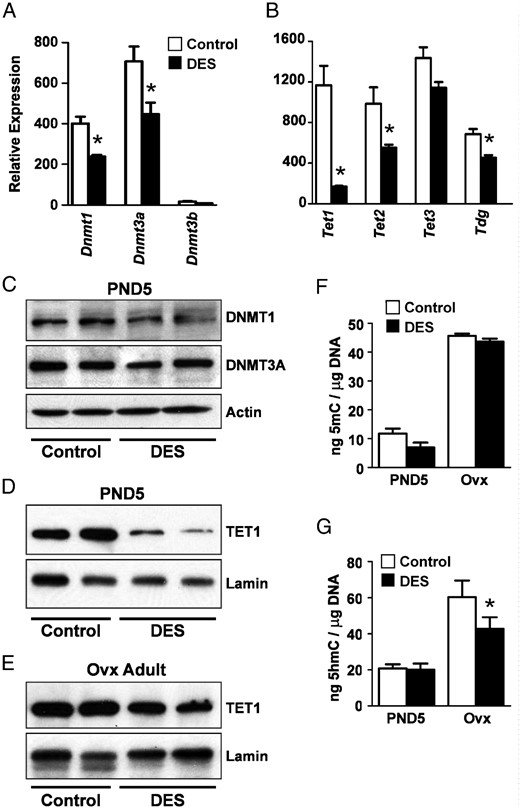
DES-induced alterations in uterine DNA methylation regulatory enzymes and DNA methylation. A, Relative mRNA expression of DNA methyltransferases on PND5. B, Relative mRNA expression of TET methylcytosine dioxygenases and thymine deglycosylase on PND5. C, Immunoblots of DNMT1 and DNMT3A on PND5. Actin was used as a loading control. In this and all subsequent immunoblots, each lane contains protein extract from a single animal, and n = 2–4 animals per group on each blot; protein from at least 4 animals was run in all cases. Representative blots are shown. D and E, Immunoblots of TET1 in PND5 (D) or adult ovx (E) uteri. Lamin was used as a loading control. F, Total DNA 5-methylcytosine. G, Total DNA 5hmC. For all graphs, mean ± SE is plotted; n = 4–8 per group. *, P < .05 compared with control at same time point.
DES-induced global alterations in expression of histone-modifying proteins
To determine whether there were DES-induced changes in expression of genes that regulate H3 lysine methylation, real-time RT-PCR was performed. The genes tested were categorized according to the lysine residue for which there is evidence that they have the strongest activity, although some have weak activity to methylate other lysines. On PND5 after DES treatment, there were significant reductions in mRNAs encoding modifiers of H3K9 methylation, including Setdb1 (Eset), Suv39h1, and Ehmt1 (Glp), whereas Ehmt2 (G9a) mRNA was not different from controls (Figure 2A). H3K27 methylation is regulated by polycomb repressive complex components, including suppressor of zeste 12 (SUZ12), enhancer of zeste homolog 2 (EZH2), and embryonic ectoderm development protein (EED) (22, 26). Of these, only Ezh2 mRNA was reduced significantly (Figure 2B). Finally, of genes encoding modifiers of H3K4 methylation, only Mll4 (Kmt2b) mRNA was reduced significantly (Figure 2C). At the protein level, DES treatment caused ∼45% reduction in EZH2 (P < .05) (Figure 2D), but there was no significant difference in EHMT2, SET domain protein, bifurcated 1 (SETDB1) or SUZ12 (data not shown). Reductions in mRNA that did not result in decreases in protein expression may be explained in part by the relative stability of the proteins. Despite the DES-induced reduction in EZH2 protein, there was no difference in total H3K27me3, and there was also no difference in H3K9me2 or H3K4me3 detected by immunoblotting of isolated histone proteins (Figure 2E), indicating that global alterations in these histone H3 lysine marks could not explain the observed gene expression changes.
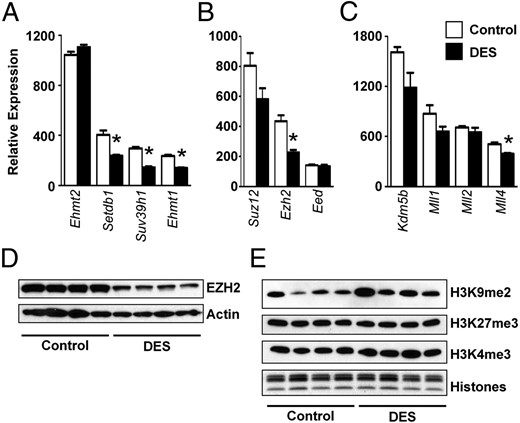
DES-induced alterations in histone methyltransferases but not methylated histones on PND5. A, Relative mRNA expression of histone H3 methyltransferases on PND5. A–C, Genes encoding methyltransferases with major reported activity on H3K9 (A), H3K27 (B), and H3K4 (C). Mean ± SE is plotted; n = 4 per group. *, P < .05 compared with control. D, Immunoblot of EZH2. Actin was used as a loading control. E, Immunoblots of H3K9me2, H3K27me3, and H4K4me3. In this and all subsequent histone immunoblots, each lane contains 2 μg histones isolated from 2 uteri per sample, and n = 4 samples per group on each blot. In the bottom panel, total histones were stained on a duplicate gel to document equal protein loading.
We next determined whether DES treatment altered expression of modifiers of histone acetylation. DES treatment had mixed effects on expression of histone acetyltransferases, causing reduced expression of Myst2 (Kat7) and Kat2a (Gcn5), increased expression of Kat2b (p300/CBP-associated factor), and no change in Kat5 (Tip60) expression (Figure 3A). Similar to the mRNA expression, there was ∼60% reduction in lysine acetyltransferase 2A (KAT2A) protein (P < .05) in DES-treated uteri compared with controls (Figure 3B). Of the histone deacetylases (HDACs), Hdac1 and Hdac3 mRNA expression levels were not different between control and DES-treated groups, but Hdac2 was decreased after DES treatment (Figure 4A). However, the protein levels of all 3 HDACs were decreased more than 65% (P < .05) in DES-treated uteri compared with controls at PND5, suggesting differential posttranscriptional regulation of these proteins after DES treatment (Figure 4B). These decreases appeared to be a temporary response to DES treatment because protein levels of HDAC1, HDAC2, and HDAC3 in uteri of adult ovx mice were much closer to control levels (Figure 4C). However, despite the alterations in expression of histone acetylation modifiers, there was no significant difference in the level of total acetylated histone H3 or H4 (data not shown) or in H4K5ac (Figure 4D). H3K9ac was decreased ∼33% in DES-treated uteri as compared with controls (P < .05), which is an effect opposite that expected from decreases in HDACs. Taken together, these findings indicate that although changes in expression of histone-modifying proteins do occur in response to DES treatment, these changes alone do not result in detectable global alterations in histone states that might explain the observed permanent alterations in gene expression.
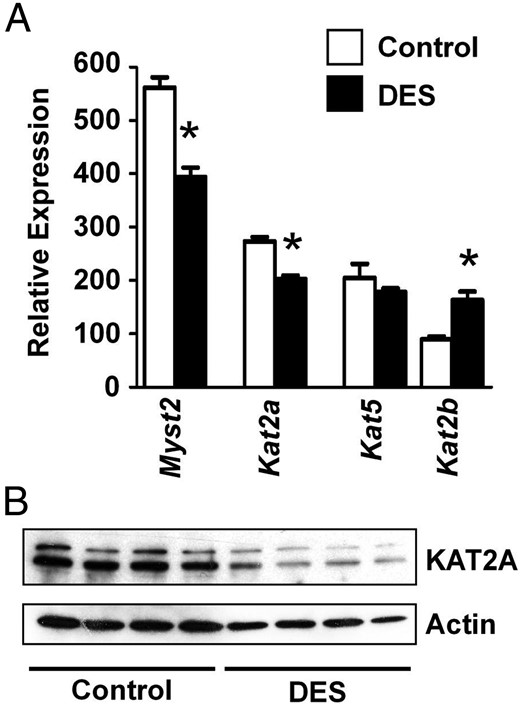
DES-induced alterations in histone-acetylating enzymes on PND5. A, Relative mRNA expression of histone acetyltransferases on PND5. Mean ± SE is plotted; n = 4 per group. *, P < .05 compared with control. B, Immunoblot of KAT2A in PND5 uteri. Actin was used as a loading control.
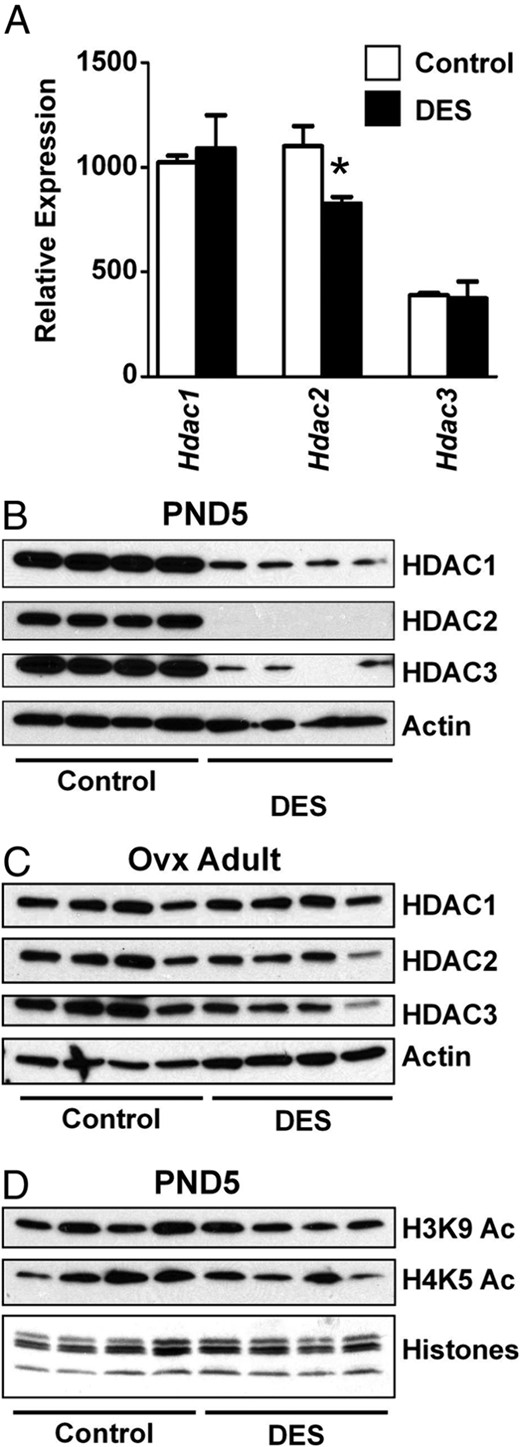
DES-induced alterations in HDACs but not acetylated histones. A, Relative mRNA expression of HDACs on PND5. Mean ± SE is plotted; n = 4 per group. *, P < .05 compared with control. B and C, Immunoblots of HDAC1, HDAC2, and HDAC3 in PND5 (B) or adult ovx (C) uteri. Actin was used as a loading control. D, Immunoblots of modified histones H3K9ac and H4K5ac. Total histone proteins were stained on a duplicate gel to ensure equal loading.
Permanent dysregulation of Ltf and Six1 expression in the uterus after neonatal DES treatment
The expression of 2 estrogen-responsive genes, Ltf and Six1, is modified in adults after neonatal DES exposure (34, 35). Consistent with previous reports, Ltf was highly upregulated in the uterus on PND5 after neonatal DES treatment and although the expression level was much lower than during the time of treatment, Ltf was still significantly upregulated in neonatal DES-treated ovx adult females (Figure 5A). Ltf expression remained estrogen-responsive in both control and neonatal DES-treated ovx females, with about a 4-fold increase over their untreated counterparts (Figure 5A). Like Ltf, Six1 was upregulated in the uterus after neonatal DES treatment on PND5, and it remained upregulated in ovx adult females (Figure 5B). In contrast to Ltf, Six1 was not estrogen-responsive at all in adult controls; however, neonatal DES treatment caused estrogen responsiveness to persist in adult ovx mice (Figure 5B). At the protein level, SIX1 was not detected in endometrium of adult controls but was highly expressed in uterine epithelial cells of mice treated neonatally with DES (Figure 5C). These findings indicate that both Ltf and Six1 are persistently dysregulated in response to neonatal DES treatment and that Six1 abnormally retains estrogen responsiveness in adults.
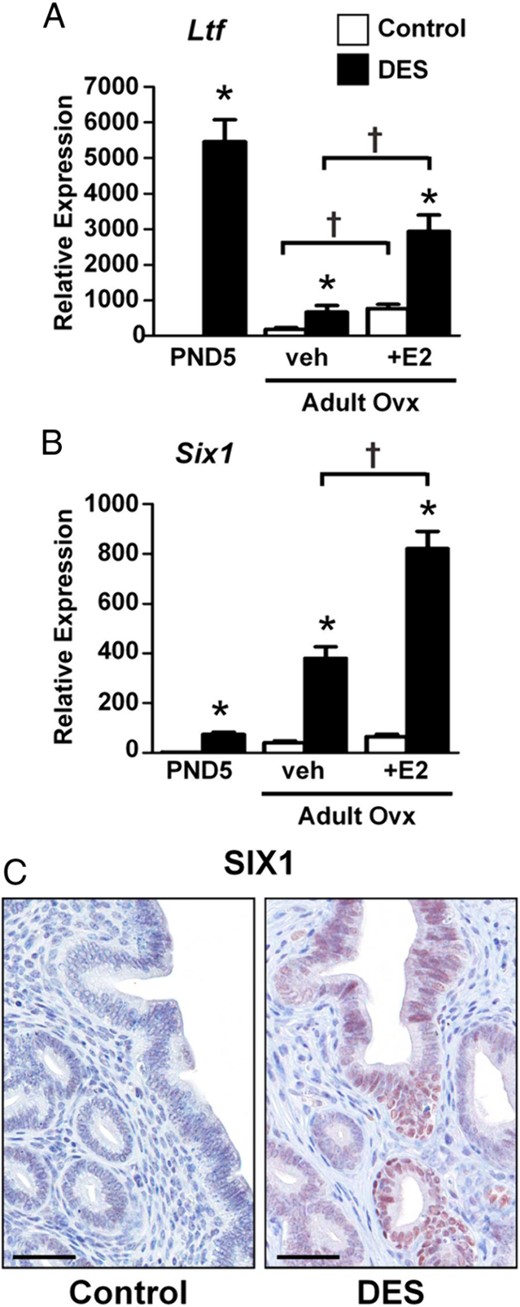
Permanent overexpression of Ltf and Six1 after neonatal DES treatment. A, Relative mRNA expression of the indicated gene in uteri from PND5, adult ovx vehicle (veh)-treated, or adult ovx plus estradiol (E2)-treated mice. Mean ± SE is plotted; n = 4 per group. *, P < .05 compared with control; †, P < .05 compared with the group indicated by the associated line. C, Immunohistochemical localization of SIX1 in the adult mouse uterus. Scale bars, 50 μm.
Site-specific alterations in associations of modified histones H3 and H4
To determine whether neonatal DES-induced dysregulation of Ltf and Six1 gene expression was a result of chromatin modifications, we performed uterine tissue ChIP to test the association of modified histone proteins at specific regions of Ltf and Six1 (Table 2). For Ltf, we examined modified histone associations at the well-characterized estrogen response element (ERE) in the promoter region and a CpG-rich region near the ERE, both of which are important regulators of Ltf expression (36). Like H3K4me3, acetylated histones H3 at lysine 9 (H3K9ac) and H4 at lysine 5 (H4K5ac) are generally associated with active chromatin. Compared with controls, all 3 of the modified histones were enriched at the ERE and CpG regions of the Ltf promoter on PND5 after DES treatment, whereas there was no difference in occupancy at a distal region ∼3 kb upstream from the transcription start site (Figure 6, left panels). Association of the repressive modification H3K27me3 was low and no different between treatment groups at any region examined (Figure 6, left panels). To determine whether these modified histone associations persisted in adults, ChIP-PCR experiments were performed in control and neonatal DES-treated adult ovx mice. There was no longer a differential association of any of the histone modifications examined at these known regulatory regions of Ltf (Figure 6, right panels), indicating that these specific modifications could not explain the persistent differences in Ltf expression after neonatal DES exposure. Normal rabbit serum was used in all of these experiments as a negative control and always demonstrated less than the lowest percent DNA input observed with the specific antibodies (data not shown).
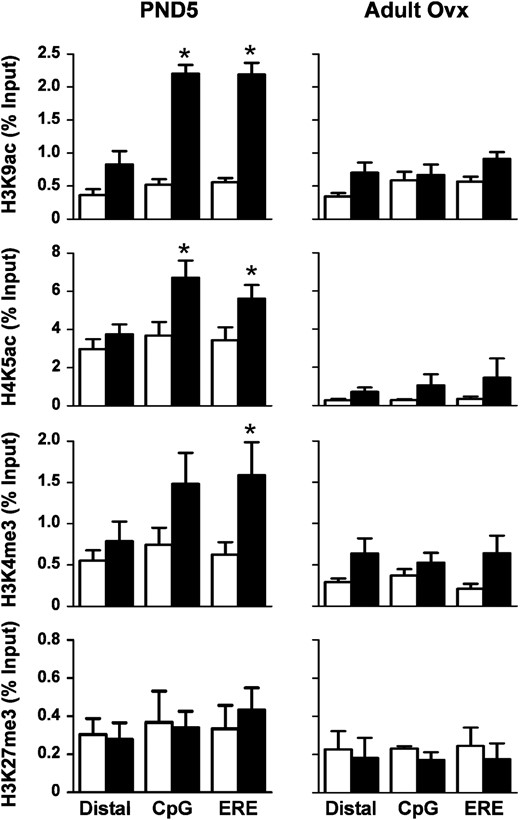
Differential association of modified histones with the Ltf gene region after neonatal DES exposure. ChIP assays were performed on uterine tissue from PND5 (left panels) or adult ovx (right panels) mice using the indicated modified histone antibodies. PCR was performed on a distal region and 2 regions near the Ltf transcription start site (CpG and ERE) (see Table 2 for primer locations). Mean ± SE is plotted; n = 4 per group. White bars represent control; black bars represent DES. *, P < .05 compared with control.
Association of the same modified histones was also determined for the Six1 gene. Six1 regulatory regions are not yet well defined experimentally, so primers were designed broadly across regions that in human cell lines either were or were not regulatory regions based on transcription factor binding data available in the online ENCODE database (37). The 4 Six1 gene regions tested were a distal upstream region, a GC-rich upstream region, a non–GC-rich upstream region, and a region encompassing exon 1 through intron 1 (Table 2). All 3 histone modifications tested that are associated with active transcription, H3K9ac, H4K5ac, and H3K4me3, were enriched at specific loci in the exon 1/intron 1 region of Six1 on PND5 after DES treatment, whereas there was no difference in the other 3 regions examined (Figure 7, left panels). H3K27me3 was significantly associated with all 3 regions close to Six1 but not the distal region; however, this modification was not differentially associated when comparing control and DES-treated groups (Figure 7, left panel).
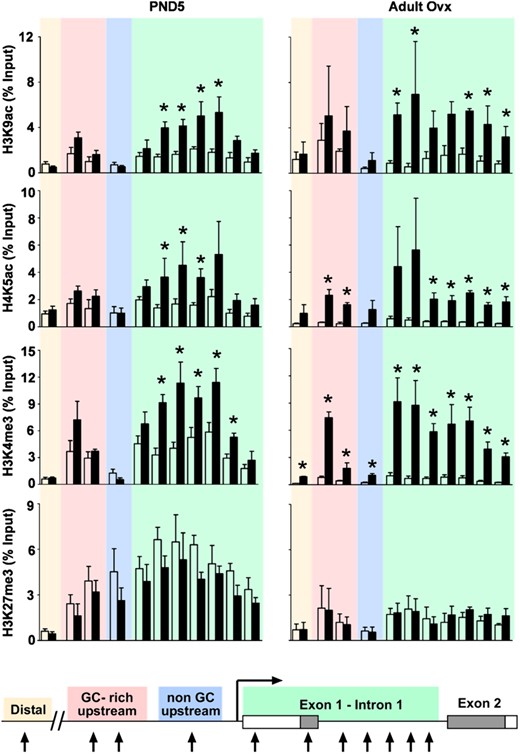
Persistent differential association of modified histones with the Six1 locus after neonatal DES exposure. ChIP assays were performed on uteri from PND5 (left panels) and adult ovx (right panels) mice using the indicated modified histone antibodies. PCR was performed on a distal region (tan), a GC-rich upstream region (pink), a non–GC-rich upstream region (blue), and a region covering exon 1 to intron 1 (green) of the Six1 gene (see Table 2 for primer locations). Mean ± SE is plotted; n = 4 per group. White bars represent control; black bars represent DES. *, P < .05 compared with control. A schematic representation of the Six1 gene indicating the primer locations is shown for reference.
To determine whether these modified histone associations were persistent, ChIP was performed on uteri of control and DES-treated ovx adults. H3K9ac association was similar to that observed on PND5, with enrichment at specific loci in the exon 1/intron 1 region after DES treatment and no differences in the other regions (Figure 7, right panels). H4K5ac and H3K4me3 were differentially associated in the exon 1/intron 1 region similar to PND5, but the GC-rich upstream region also had sites of increased association of both of these activating histone marks after DES treatment (Figure 7, right panel). The H3K4me3 association at this region was similar in amplitude to that observed in the Six1 exon 1/intron 1 region. H3K27me3 associations in the adult uteri were similar to those observed on PND5, with no differences between control and DES-treated samples. In general, the differences observed in adult uteri were of a higher magnitude than on PND5, primarily due to reduced association in the controls. For example, there was an 8- to 12-fold increase in association of H3K4me3 at sites in the exon 1/intron 1 region of DES-treated adult uteri as compared with a 2- to 3-fold increase observed in the PND5 samples. These data suggest that there is consolidation of the associated histone modifications over time and that these activating histone marks could explain the increased Six1 expression in adult uteri.
Discussion
The results presented here indicate that exposure of neonatal mice to DES results in significant alterations in uterine expression of several types of chromatin-modifying proteins. In general, these changes did not correlate with global alterations in histone modifications, indicating that the function of these proteins is tightly regulated in a locus-specific manner. However, the reduction in TET1 protein was correlated with global alterations in DNA 5-hydroxymethylation that could affect gene expression in adults. DES treatment also caused temporary enrichment of specific histone modifications at selected regulatory sites within the Ltf promoter and long-term alterations in the histone modifications associated with Six1. These findings clearly demonstrate that in vivo developmental exposure to estrogenic EDCs can modify the epigenetic landscape of the Six1 locus in the adult uterus.
There are several previous reports of alterations in DNA methylation as a result of EDC exposure in mouse models (13, 16, 38–40). Perhaps the best known of these is the case of the dominant agouti allele, Aiapy, whose differential expression is regulated by methylation of a cryptic promoter within a retrotransposon integrated upstream of the first coding exon (41). Prenatal exposure to the EDC bisphenol A decreases methylation of this promoter, resulting in variable expression of the agouti gene in the exposed offspring (38). Similarly, prenatal exposure to bisphenol A results in decreased methylation of the Hoxa10 promoter and intron 1 regions, whereas prenatal exposure to DES results in increased methylation of the intron 1 region (16, 39). However, it is not clear that these alterations in Hoxa10 methylation are responsible for changes in Hoxa10 expression because the 2 EDC exposures have opposite effects on methylation and yet they both result in increased Hoxa10 expression. Instead, it is likely that additional epigenetic modifications also occur and contribute to the final gene expression profiles observed. We observed a significant decrease in TET1 protein on PND5 that was still apparent in adults. Because TET methylcytosine dioxygenases convert 5-methylcytosine to 5hmC (42, 43), a reduction in this protein could contribute to the observed decrease in DNA hydroxymethylation in adults and could impact expression of specific genes.
One additional epigenetic modification that can be influenced by EDC exposure is H3K27 methylation. Rats neonatally exposed to the phytoestrogen genistein have increased phosphorylation of the histone methyltransferase EZH2 and may have a subtle reduction in global H3K27me3 in uterine myometrium (44). Neonatal genistein treatment also alters expression of several genes in uteri of cycling adult rats, but whether or not these alterations are related to changes in H3K27me3 at the affected gene loci was not tested. In the results reported here, neonatal DES exposure resulted in significant reductions in total EZH2 protein levels but did not affect either global H3K27me3 levels or the gene-specific associations of this histone modification that we examined. These findings suggest that alterations in H3K27me3 associations do not explain the DES-induced alterations in gene expression observed in our mouse model, at least at the loci examined. Of interest, there was a significant association of H3K27me3 in the upstream and exon 1/intron 1 regions of Six1 in DES-treated mice on PND5 and to a lesser extent in adult ovx mice, both of which are times when Six1 is highly expressed. This finding conflicts with the general view that H3K27me3 is a repressive chromatin mark. However, it was shown recently in embryonic stem cells and hematopoietic cells that H3K27me3 in promoter regions can be associated with active gene transcription (45). Alternatively, the H3K27me3 associations in our experiments could be a result of cell-type–specific associations in myometrial or endometrial stromal cells that do not express Six1.
Ltf, a traditional estrogen-responsive gene, is regulated by classical estrogen receptor/ERE interactions in the promoter region (36), suggesting that the temporary alterations in histone modifications associated with this locus are a result of changes in chromatin structure near the promoter during DES-induced gene transcription. Because these promoter region histone associations were not maintained in adults, alternative mechanisms must be responsible for permanently altering gene expression. Previous studies have shown subtle decreases in DNA methylation in the Ltf promoter long after the last neonatal DES treatment (34), but it is not known whether this methylation change is responsible for altered Ltf expression. In fact, the reduced promoter methylation is dependent on a second hit of ovarian hormone exposure because DES-treated mice ovx before puberty have the same Ltf promoter methylation pattern as controls. Future experiments will explore alternative epigenetic mechanisms that could explain the observed permanent alterations in Ltf expression.
Very little is known regarding how Six1 is regulated. In control mice, it is expressed in the cervix and vagina but not the uterus (35), suggesting an influence during development by regulators of anterior-posterior patterning such as Hoxa genes. We could not identify a consensus ERE in the 2-kb region upstream from the Six1 transcription start site; however, the increased expression of this gene in response to DES suggests either that there is a regulatory ERE located elsewhere or that a non–ERE-mediated tethering mechanism of transcriptional activation occurs (46, 47). We found that Six1 is aberrantly upregulated by estrogen in neonatal DES-treated adults, suggesting that the estrogen responsiveness is a result of epigenetic modifications induced during neonatal DES treatment. There is evidence for SIX1 autoregulation of its own expression via SIX homeoprotein binding sites during sensory organ development (48, 49), so DES-mediated initiation of inappropriate Six1 expression during neonatal treatment could cause a persistent positive feedback loop in the absence of appropriate inhibitory mechanisms and thereby result in continued Six1 expression. Recruitment of chromatin-modifying protein complexes by SIX1 and other associated transcription factors or simply transcription-associated increases in accessibility of these loci to chromatin modifiers could explain how the observed epigenetic changes are initiated.
Several enhancer elements that regulate Six1 expression in the developing mouse nervous system have been identified (48). One of these enhancers coincides with the GC-rich region ∼6.5 kb upstream from the transcription start site that in this study had persistently altered H4K5ac and H3K4me3 associations. Enhancer regions are typically marked by high H3K4me1 and low H3K4me3 associations; however, increased H3K4me3 association is observed at active enhancers engaged by RNA polymerase II (50, 51). Indeed, in data from several cell lines released by the ENCODE project, the homologous GC-rich region ∼7 kb upstream of the human Six1 transcription start site has classic features of chromatin at active enhancer regions including deoxyribonuclease I hypersensitivity, transcription factor and RNA polymerase II binding, and histone H2A.Z, H3K27ac, and H3K4me3 associations (37). These findings, together with our results, suggest that the GC-rich upstream region may function as a Six1 enhancer in the uterus after neonatal DES exposure.
In summary, we have demonstrated that brief neonatal exposure to an estrogenic EDC persistently alters histone modifications associated with Six1 gene-regulatory regions functioning in the adult uterus. Combined with previous data demonstrating EDC-induced alterations in gene-specific DNA methylation, and our data showing global alterations in DNA hydroxymethylation, these findings provide the mechanistic underpinnings for understanding how EDC exposures during development can lead to functional and pathological changes in somatic tissues of adult animals.
Acknowledgments
We thank Paul Wade and Sylvia Hewitt for critical reading of the manuscript.
This work was supported by the Intramural Research Program of the National Institutes of Health, National Institutes of Environmental Health Sciences, ES102405 (to C.J.W.) and ES071006 (to T.K.A.).
Disclosure Summary: The authors have nothing to disclose.
Abbreviations
- ChIP
chromatin immunoprecipitation
- DES
diethylstilbestrol
- DNMT1
DNA methyltransferase I
- EDC
endocrine-disrupting chemical
- EHMT2
euchromatic histone-lysine N-methyltransferase 2
- ERE
estrogen response element
- EZH2
enhancer of zeste homolog 2
- HDAC
histone deacetylase
- HIP
homogenization-immunoprecipitation
- 5hmC
5-hydroxymethylcytosine
- KAT2A
lysine acetyltransferase 2A
- 5mC
5′-methyl-2′-deoxycytidine
- ovx
ovariectomized
- PAS
protein A-Sepharose
- PND1
postnatal day 1
- SIX1
sine oculis-related homeobox 1
- SUZ12
suppressor of zeste 12
- TBST
Tris-buffered saline containing 0.1% Tween 20
- TET1
TET oncogene family, member 1.