-
PDF
- Split View
-
Views
-
Cite
Cite
Masato Asai, Yasumasa Iwasaki, Masanori Yoshida, Noriko Mutsuga-Nakayama, Hiroshi Arima, Masafumi Ito, Koji Takano, Yutaka Oiso, Nuclear Factor of Activated T Cells (NFAT) Is Involved in the Depolarization-Induced Activation of Growth Hormone-Releasing Hormone Gene Transcription in Vitro, Molecular Endocrinology, Volume 18, Issue 12, 1 December 2004, Pages 3011–3019, https://doi.org/10.1210/me.2003-0471
- Share Icon Share
Abstract
GHRH plays a pivotal role in the regulation of both synthesis and secretion of GH in the anterior pituitary. In this study, we examined the molecular mechanism of depolarization-induced GHRH gene transcription using the hypothalamus cell line, Gsh+/+, revealing the involvement of the transcription factor called nuclear factor of activated T cells (NFAT). GHRH, NFAT1, NFAT4, and related genes were endogenously expressed in Gsh+/+ cells and the rat arcuate nucleus, where NFAT1 and GHRH were colocalized. Cellular excitation with high potassium potently stimulated endogenous GHRH gene 5′-promoter activity as well as the NFAT-mediated gene transcription, the former being further enhanced by coexpression of NFAT. On the other hand, cyclosporin A (a calcineurin-NFAT inhibitor) or EGTA (a calcium chelator) significantly blocked the depolarization-induced GHRH gene transcription. EMSA and site-directed mutagenesis experiments showed the direct binding of NFAT at five sites of the GHRH promoter, among which the relative importance of three distal sites (−417/−403, −402/−387, −317/−301) was suggested. Finally, elimination of all five sites completely abolished the NFAT-induced GHRH gene up-regulation. Altogether, our results suggest that the transcription factor NFAT is involved in the depolarization-induced transcriptional activation of GHRH gene in the neuronal cells.
GHRH IS EXPRESSED in the arcuate nucleus of the hypothalamus and is assigned a central role in both the synthesis and secretion of GH. In the embryonic brain, the homeobox protein Gsh-1 is known to determine the formation of GHRH neurons, and indeed Gsh-1 homozygous mutants are reported to show impaired development of GHRH-producing cells in the brain, with resultant hypogenesis of the pituitary gland, reduced numbers of somato- and lactotroph cells, and severe dwarfism (1). Furthermore, we have recently shown that Gsh-1 is involved in the tissue-specific expression of GHRH gene in the adult (2).
The molecular mechanism of the acute transcriptional regulation of GHRH gene, however, is not completely clarified. In addition to the GHRH neurons in the arcuate nucleus, GHRH expression is found in a variety of extraneuronal tissues including placenta and testis, and most of the studies regarding GHRH gene expression have been carried out using the human placental cell line JEG-3 that endogenously expresses GHRH (2). However, the regulation of the GHRH gene transcription in neurons and other peripheral organs may be different, in terms of both the transcription factors involved and the promoter regions used (3–5). Thus, further studies using the neuronal cells are needed to clarify the neuron-specific transcriptional regulation of GHRH gene.
In this report, we examined the short-term regulation of GHRH gene transcription with the Gsh+/+ cells derived from the transgenic mouse expressing Gsh-1 (6). We especially focused on the depolarization-induced expression of GHRH gene, because in the neuron cellular excitation usually stimulates both syn-thesis and secretion of the neuropeptides. Using the experimental paradigm, we found the possible role of the calcium-regulated transcription factor nuclear factor of activated T cells (NFATs). Although NFATs form a group of calcium-responsive transcription factors originally found in activated T cells, later studies showed the expression in nonimmune tissues as well, including endocrine organs and the central nervous system (7–10). Our present data suggest strongly that NFAT is involved in the depolarization-induced transcriptional activation of GHRH gene in the neuronal cells.
RESULTS
NFAT and the Related Genes Are Expressed in Gsh+/+ Cells
We first examined the endogenous expression of the NFAT family and related genes in the mouse hypothalamus-derived neuronal cell line Gsh+/+ by RT-PCR. The results showed that, among the NFAT family genes, transcripts of NFAT1b and 4 were amplified (Fig. 1). We also found the expression of the catalytic subunit of calcineurin and the casein kinase I, which phosphorylates NFAT. These results suggest that the NFAT system is functional in Gsh+/+ cells. The transcript of the Gsh-1 homeobox protein gene, which determines the expression of GHRH, was amplified as well.
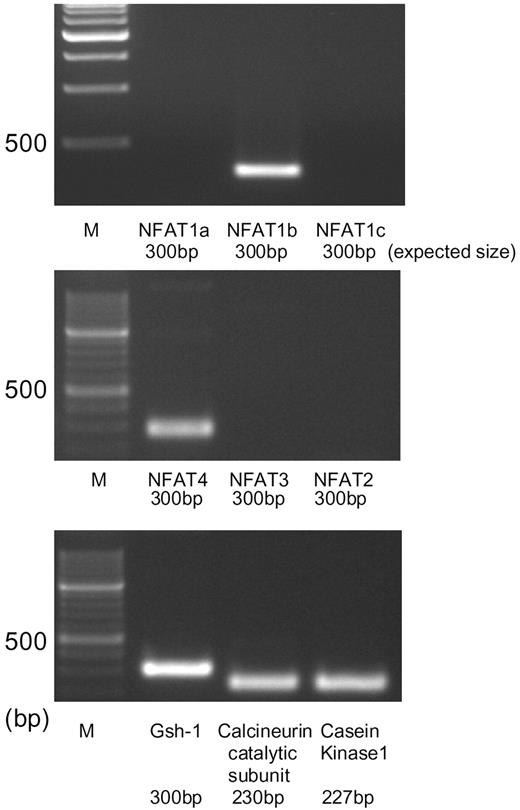
RT-PCR Analysis of the Expression of NFAT and Related Gene Transcripts in Gsh+/+ Cells Total RNA was extracted from the Gsh+/+ cells, and 1 μg was applied for RT reaction, followed by PCR using specific primer sets for each mRNA. The figure shows photographs of the ethidium bromide-stained products using agarose gel electrophoresis. Bands corresponding to NFAT1b, NFAT4, catalytic subunits of calcineurin, casein kinase 1, and Gsh-1 mRNAs were amplified. NFAT1a, NFAT1b, and NFAT1c are splice variants derived from a single gene.
GHRH Gene Expression Is Enhanced Either by High Potassium-Induced Depolarization or by cAMP in Gsh+/+ Cells
We then examined the effect of cellular depolarization- or cAMP-induced GHRH gene expression. We found that treatment of the Gsh+/+ cells with 80 mm potassium chloride (KCl), which induces cellular depolarization, potently enhanced endogenous expression of GHRH mRNA (Fig. 2A). Treatment of the cells with KCl or forskolin, an activator of adenylate cyclase, both significantly increased the transcriptional activity of GHRH gene 5′-promoter (−472/+71) (Fig. 2B). We also examined the effect of high potassium-induced cellular depolarization on intracellular Ca2+ concentration ([Ca2+]i) by using fura2-based [Ca2+]i measurement. In the standard bath solution containing 5 mm potassium, [Ca2+]i was 53 ± 12 nm (n =15). When the bath solution was changed to high potassium solution, [Ca2+]i significantly increased to 178 ± 32 nm (n =15, P < 0.01 by paired t test) (Fig. 2C, upper panel). In the bath solution containing nitrendipine (5 μm) and ω-conotoxin GV1A (1 μm), high potassium did not increase [Ca2+]i (Fig. 2C, lower panel) [49 ± 5 nm to 45 ± 7 nm (n =15), n. s.]. Finally, treatment of the cells with EGTA (5 mm) completely inhibited the depolarization-induced activation of GHRH gene (Fig. 2D). Altogether, our data provide evidence that Gsh+/+ cells express endogenous GHRH gene and are thus recognized as a homologous cell line derived from the hypothalamic GHRH neuron. The data also suggest that GHRH gene transcription is stimulated not only by cAMP/protein kinase A, as was previously shown in JEG3 placental cells, but also by depolarization-induced calcium signaling pathways.
![GHRH Gene Expression Is Enhanced by High Potassium-Induced Depolarization in Gsh+/+ Hypothalamic Cells A, Gsh+/+ cells were treated with KCl (80 mm) for the indicated time intervals. One microgram of RNA extracted from each sample was then reverse-transcribed and amplified using specific primers for the mouse GHRH. Product from glyceraldehyde-3-phosphate dehydrogenase (GAPDH) mRNA was used as an internal control. The figure shows photographs of the ethidium bromide-stained products using agarose gel electrophoresis. B, Gsh+/+ cells were transfected transiently with the rat GHRH (−472/+71)-luciferase plasmid (GHRH-Luc), and then treated with either KCl (80 mm) or forskolin (Fsk; 10 μm) for 5 h. Finally, the cells were lysed and the 5′-promoter activity of GHRH gene was determined by luciferase assay. C, Gsh+/+ cells were treated with high extracellular potassium in bath solution (High K, upper panel) or with the same solution containing nitrendipine (NIT; 5 μm) and ω-conotoxin GV1A (CgTX; 1 μm). Then [Ca2+]i was determined as mentioned in Materials and Methods. D, Gsh+/+ cells were transfected transiently with the GHRH-Luc plasmid. The cells were preincubated with EGTA (5 mm), and then treated with KCl (80 mm) for 5 h. Assay was carried out as mentioned above. *, P < 0.05 vs. control.](https://oup.silverchair-cdn.com/oup/backfile/Content_public/Journal/mend/18/12/10.1210_me.2003-0471/3/m_zmg0120414150002.jpeg?Expires=1750372885&Signature=1HgzXcwB8ZVzCpadAeNdmCmG3C6OJaHQ45pHfjJwkVHonFi0MME4k3gk34xLmbDE1jgZ723I~zmTLvIzMc49moorCVSIqG2aOPGqbkGkYTqT0dFzfPIeXzY7shIx6B1jIxcQ7dioDZb60W8B0rY5oKoZBOL58aHg10tRKjUN2tHmS7BCTOHa06NwG5PX5C02bsjgEDrWPYmA4dgDkiy3pVTDpC3BWDamtOTlHJsVrx3DnAKTGMnVhyQFRG1hFVWkyhEyjA4mAu2nFeQlz8iKI7uTNQGWgcFaWY-FmpryOMsLpBIJZzcA4Jw5jpkpdyyIJwuzYum1KpkYDNM0C0I7-Q__&Key-Pair-Id=APKAIE5G5CRDK6RD3PGA)
GHRH Gene Expression Is Enhanced by High Potassium-Induced Depolarization in Gsh+/+ Hypothalamic Cells A, Gsh+/+ cells were treated with KCl (80 mm) for the indicated time intervals. One microgram of RNA extracted from each sample was then reverse-transcribed and amplified using specific primers for the mouse GHRH. Product from glyceraldehyde-3-phosphate dehydrogenase (GAPDH) mRNA was used as an internal control. The figure shows photographs of the ethidium bromide-stained products using agarose gel electrophoresis. B, Gsh+/+ cells were transfected transiently with the rat GHRH (−472/+71)-luciferase plasmid (GHRH-Luc), and then treated with either KCl (80 mm) or forskolin (Fsk; 10 μm) for 5 h. Finally, the cells were lysed and the 5′-promoter activity of GHRH gene was determined by luciferase assay. C, Gsh+/+ cells were treated with high extracellular potassium in bath solution (High K, upper panel) or with the same solution containing nitrendipine (NIT; 5 μm) and ω-conotoxin GV1A (CgTX; 1 μm). Then [Ca2+]i was determined as mentioned in Materials and Methods. D, Gsh+/+ cells were transfected transiently with the GHRH-Luc plasmid. The cells were preincubated with EGTA (5 mm), and then treated with KCl (80 mm) for 5 h. Assay was carried out as mentioned above. *, P < 0.05 vs. control.
High Potassium-Induced Depolarization Causes Nuclear Translocation of NFAT1
To confirm that high potassium-induced depolarization induces nuclear translocation of NFAT in Gsh+/+ cells, we carried out Western blot analysis using nuclear extract of Gsh+/+ cells treated with KCl. The results showed that the nuclear amount of NFAT1 was increased after depolarization, with maximal effect at 0.5–2 h (Fig. 3A). When the cells were pretreated with cyclosporin A 1 h ahead of KCl treatment, the effect was virtually abolished (Fig. 3B). These data show that NFAT is promptly translocated to the nuclear compartment after cellular excitation (probably by an increase in intracellular calcium), and that cyclosporin A inhibits this nuclear translocation.
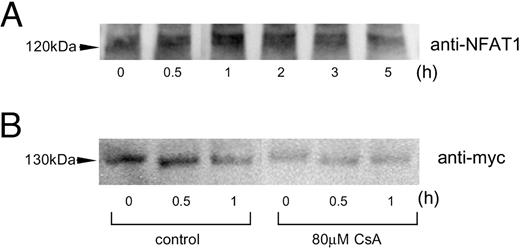
Depolarization-Induced Nuclear Translocation of NFAT1 A, Gsh+/+ cells were treated with 80 mm KCl for the time intervals indicated. Western blot analysis of the nuclear protein was then carried out using anti-NFAT1 antibody. B, Gsh+/+ cells, transfected with 1 μg of human NFAT1c/pcDNA3.1/myc/His, were pretreated with/without cyclosporin A (CsA; 80 μm). The cells were then depolarized with KCl (80 mm) for the defined time intervals, and nuclear protein extracted was applied for Western blot analysis.
NFAT Coexpression Enhances Depolarization-Induced Activation of GHRH Gene
To determine whether NFAT is involved in excitation-induced GHRH gene expression in Gsh+/+ cells, we examine the effect of NFAT coexpression on GHRH 5′-promoter activity under a basal or depolarized condition. When various doses of NFAT1c expression vector were cointroduced with GHRH-Luc plasmid, the GHRH gene 5′-promoter activity was enhanced in a dose-dependent manner (Fig. 4A). This effect was more obvious when the cells were depolarized with KCl treatment. Similar enhancement by NFAT coexpression was obtained when NFAT-responsive reporter plasmid was used (Fig. 4B). These results suggest that NFAT is involved in the basal, and more obviously, excitation-induced activation of the GHRH gene expression.
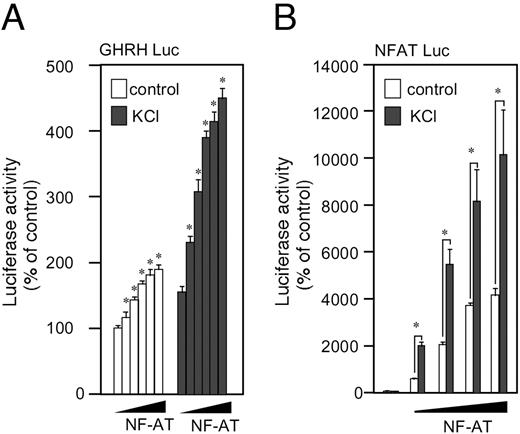
Overexpression of NFAT Enhances the Transcription Activity of GHRH or NFAT-Responsive Genes A, Gsh+/+ cells were transfected transiently with GHRH-Luc and without (empty plasmid), or with increasing doses of human NFAT1c expression vector (2.4, 12, 42, and 60% vs. GHRH-Luc plasmid). The total amount of the expression vector was adjusted to be the same among the groups using the empty plasmid. Sixteen hours later, the cells were treated with KCl (80 mm) for the subsequent 5 h, and then lysed for luciferase assay. B, A similar experiment was carried out using NFAT-responsive luciferase reporter plasmid instead of GHRH-Luc. *, P < 0.05 vs. corresponding control.
Calcineurin/NFAT Inhibitor Cyclocporin A or the Dominant-Negative Form of NFAT Diminishes the Depolarization-Induced Activation of GHRH Gene Transcription
To further confirm the involvement of NFAT in GHRH gene transcription, we examined the effect of calcineurin/NFAT inhibitor cyclosporin A, or the ex-pression of the dominant-negative form of NFAT, in the regulation of GHRH 5′-promoter activity. Treat-ment of the cells with cyclosporin A (20 or 80 μm) did not influence basal GHRH promoter activity (data not shown) but inhibited the depolarization-induced GHRH gene transcription in a dose-dependent manner (Fig. 5A). Furthermore, coexpression of the dominant-negative form of NFAT (AxAxAA) significantly decreased the induction as well (Fig. 5B). These results further support the hypothesis that endogenous NFAT mediates the depolarization-induced rise in the promoter activity of GHRH gene.
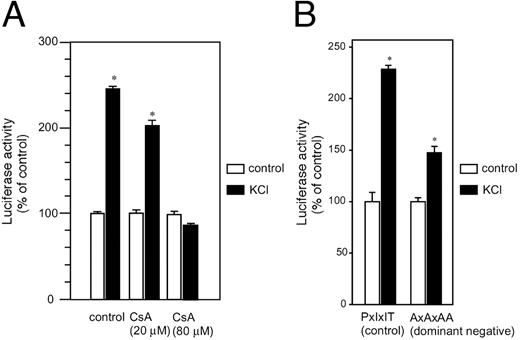
Involvement of Calcium/Calcineurin/NFAT System in Depolarization-Induced Activation of GHRH Gene A, Gsh+/+ cells were transfected transiently with GHRH-Luc, preincubated with/without cyclosporin A (CsA; 20 or 80 μm), and then treated with KCl (80 mm) for 5 h. The cells were lysed and the 5′-promoter activity of GHRH gene was determined by luciferase assay. B, Gsh+/+ cells were transfected with 0.25 μg of GHRH-Luc and 2.5 μg of either the dominant-negative form of NFAT3 expression vector (AxAxAA) or control vector (PxIxIT) (8 ). The cells were then treated with KCl (80 mm) for 5 h, and assays were carried out as mentioned above. *, P < 0.05 vs. control.
NFAT Binds to the 5′-Promoter Region of GHRH Gene
The 5′-flanking region of the rat GHRH gene contains the putative binding sites for NFAT (core motif: GGAAA or TTTCC) (Fig. 6). To directly map the NFAT binding site(s) in the promoter, we carried out an EMSA. The result showed that NFAT could bind to all of the five putative NFAT binding sites (sites 1 to 5), and that excessive cold probe containing the consensus NFAT binding sequence significantly competed for the binding at all five sites (Fig. 7). The cold probe did not influence the nonspecific bands, indicating the specificity of NFAT binding.
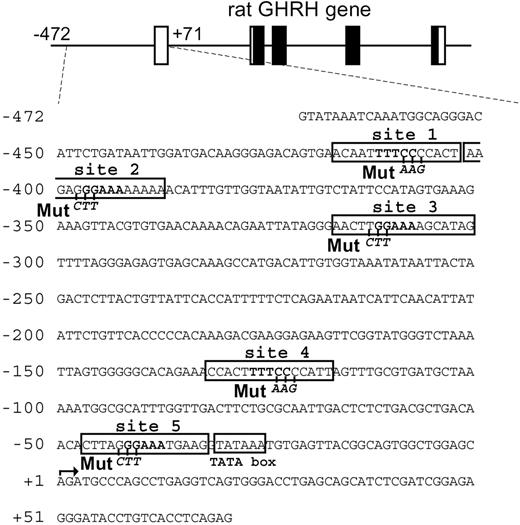
Nucleotide Sequence of Rat GHRH 5′-Promoter Used in this Study Putative NFAT binding sites containing the core motif (GGAAA or TTTCC, boxed) are designated as sites 1 to 5. “Mut” shows the location of site-directed mutagenesis used in the subsequent studies.
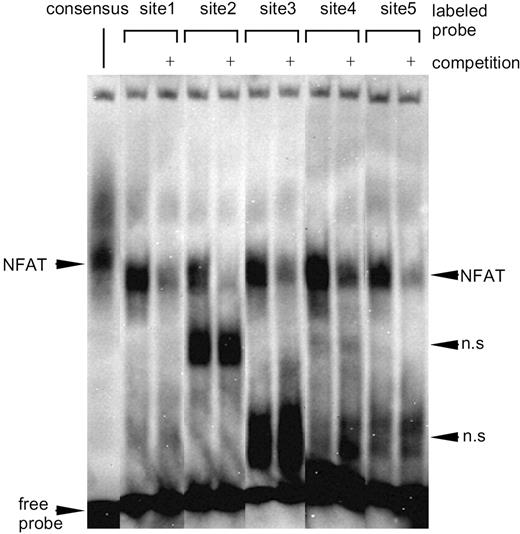
EMSA of the NFAT Binding in the Rat GHRH Promoter A probe containing the consensus NFAT-binding sequence located in the rat insulin I gene and the probes corresponding to sites1 to 5 were biotin-labeled and incubated with the whole cell lysate extracted from the NFAT-overexpressed Gsh+/+ cells, followed by electrophoresis (see Materials and Methods). Competition experiments were carried out using 500-fold molar excess of the unlabeled probe.
Elimination of the NFAT Binding Sites Abolishes NFAT-Induced GHRH Gene Transcription
We tried to determine which of the NFAT binding sites is functional in the transcriptional regulation of GHRH gene. Nucleotide sequences of each binding site (sites 1 to 5) were isolated and located immediately upstream of thymidine kinase minimal promoter-luciferase construct (pTAL-Luc), and examined for the effect of NFAT overexpression. We found that only site 1 and site 3 responded to the induction by NFAT (Fig. 8A). We also carried out experiments using mutated promoter constructs in which one site, multiple sites in combination, or all of the NFAT binding sites were destroyed. The results showed that mutation of either site 1, 2, or 3 significantly attenuated NFAT-mediated GHRH induction (Fig. 8B), and elimination of all the sites completely abolished the responsiveness to NFAT. This suggests the relative importance of sites 1 to 3, with a minimal but significant role of sites 4 and 5 in NFAT-induced transcriptional activation of GHRH gene.
![Relative Importance of Five NFAT Responsive Elements A, Plasmids with minimal thymidine kinase promoter (pTAL-Luc) containing the nucleotide sequence of each binding site (sites 1–5) were expressed without or with NFAT expression vector, and the ratio of the promoter activity [NFAT(+)/NFAT(−)] was determined. B, Basal promoter activity and the induction by NFAT were examined using wild-type (WT) or mutated GHRH gene 5′-promoter constructs in which one, multiple in combination, or all of the NFAT binding sites were destroyed. *, P < 0.05 vs. basal promoter activity.](https://oup.silverchair-cdn.com/oup/backfile/Content_public/Journal/mend/18/12/10.1210_me.2003-0471/3/m_zmg0120414150008.jpeg?Expires=1750372885&Signature=4ESAWNPBss1fKm4K4ZubD3ZIoeoPb8ortRe0UZyJ346l3ueTMzLcG-B-BjiiNiT6AKJ8IFVs-qZ07O7oa9mJsQQKldUSwoUCGydLzqut4lfbd90sSQJrjLPo~5SYZHbUS3ybjxAyGsF7JsaFHoTyE~LYNX0Kqoc5DvjBCwu1YIOuKeYvqJJSGVsVVxyZSVARHH1sInC6x7NgXvwmwAaxwzivVxagyflvJtGZrJRS3kjAeuMJOVGLVqK95GEv0y5kQkFSoyh-Hgdb-a7fj4jaCsZbEn28qqzBtMwQXIwmh2LkRqbRbqUmu1xh1BEG3VfnH-8akK6MG1ckBmSmGrH1Og__&Key-Pair-Id=APKAIE5G5CRDK6RD3PGA)
Relative Importance of Five NFAT Responsive Elements A, Plasmids with minimal thymidine kinase promoter (pTAL-Luc) containing the nucleotide sequence of each binding site (sites 1–5) were expressed without or with NFAT expression vector, and the ratio of the promoter activity [NFAT(+)/NFAT(−)] was determined. B, Basal promoter activity and the induction by NFAT were examined using wild-type (WT) or mutated GHRH gene 5′-promoter constructs in which one, multiple in combination, or all of the NFAT binding sites were destroyed. *, P < 0.05 vs. basal promoter activity.
NFAT Is Expressed in the Rat GHRH Neurons of the Arcuate Nucleus in Vivo
Finally, we tried to confirm the in vivo expression of NFATs in GHRH neuron of the hypothalamus. RT-PCR analysis using RNA extract from the punched-out brain tissue of the rat arcuate nucleus clearly showed local expression of NFAT1, NFAT4, and calcineurin, as well as GHRH and Gsh-1 (Fig. 9). NFAT3 was not examined because of the lack of sequence data in GenBank. Further analysis using immunohistochemistry technique revealed that GHRH and at least NFAT1 are colocalized in the arcuate nucleus of the rat hypothalamus (Fig. 10). These data suggest the presence of the calcineurin/NFAT system in the GHRH neurons in vivo.
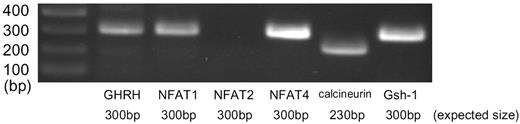
Expression of NFAT Families in the Arcuate Nucleus of the Rat Hypothalamus Rat brain was coronal-sectioned at the position 4.2-mm caudal from bregma, and arcuate nuclei were punched out by an 18-gauge fine needle. One microgram of total RNA extracted from the sample was reverse-transcribed and then analyzed by RT-PCR using specific primers. The figure shows photographs of the ethidium bromide-stained products using agarose gel electrophoresis.
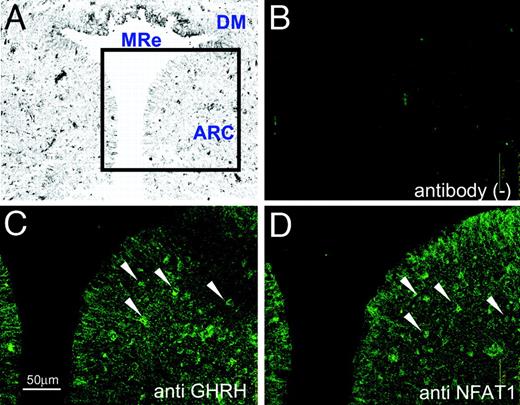
Colocalization of NFAT1 and GHRH in the Arcuate Nucleus of the Hypothalamus Analyzed by Immunofluorescence Photomicrographs A–D, Rat brain was coronal-sectioned at the position 4.2-mm caudal from bregma, and the arcuate nucleus was stained with anti-GHRH antibody (C), anti-NFAT1 antibody (D), or without antibody (B). Sections B–D are serial in order and 8 μm in thickness. The arrows indicate both the GHRH- and NFAT1-positive neurons. A weakly magnified gray-scale image (A) is referenced to show the location. MRe, Mammillary recess third ventricle; DM, dorsomedial hypothalamic nucleus; ARC, arcuate nucleus. Scale bar, 50 μm.
DISCUSSION
Previously, we reported the molecular mechanism underlying the tissue-specific expression of GHRH gene (2). In this study, we focused on the neuron-specific and depolarization-induced regulation of the GHRH gene using homologous neuronal cell line, and obtained data indicating the involvement of calcium-regulated transcription factor NFAT. Although initially cloned from immune cells, NFAT has been shown to play an important role in nonimmune tissues, and our findings suggest the link between neuronal excitation with increased intracellular calcium and GHRH gene expression.
We adopted in this study the Gsh+/+ cell line derived from the hypothalamus of transgenic mice expressing Gsh-1 (6). GHRH is expressed not only in the arcuate nucleus of the central nervous system but also in some peripheral organs, and the short-term transcriptional regulation may be different among the tissues expressed. Indeed, the alternative promoter region of GHRH gene is used in the placental tissues (4). In this sense, Gsh+/+ is an appropriate host cell line for studying the neuron-specific transcriptional regulation of GHRH gene in the hypothalamus.
The transcription factor NFAT protein family is originally found in the activated T cells in the immune system (7, 8). They consist of at least five subtypes (NFAT1 to 5) and are known to be involved in the calcium-sensitive transcriptional regulation in not only immune but also nonimmune tissues including the central nervous system (9, 11). After the rise in intracellular calcium, activated calcineurin elicits dephosphorylation of NFAT proteins, which allows them to translocate into the nucleus and function as transcription factors. In neuronal cells, cellular depolarization triggers the rise in intracellular calcium followed by the release and synthesis of a variety of neuropeptides. In this study, we used a high potassium-induced cellular depolarization paradigm, because high potassium concentration in the extracellular solution increases [Ca2+]i dependently on L-type and N-type voltage-gated calcium channels, followed by calcium influx through these channels in Gsh+/+ cells. Our data indicate that the system employed is effective in increasing [Ca2+]i. Because neuronal excitation is known to stimulate both secretion and gene expression of neuropeptides, the results obtained strongly suggest that NFAT is one of the candidate transcription factors linking neuronal excitation and gene expression. Indeed, our data show that some of the NFAT subtypes and related genes are expressed in Gsh+/+ cells as well as in hypothalamic GHRH neurons, and KCl-induced cellular depolarization potently stimulates NFAT-specific gene transcription.
We speculated the involvement of NFATs in the neuronal excitation-stimulated short-term regulation of GHRH gene expression because five putative NFAT binding sites were recognized in its 5′-promoter region. Our EMSA data clearly showed that NFAT binds to all sites of the GHRH gene promoter, and expression studies indicated the relative importance of three distal sites. Furthermore, elimination of all five NFAT binding sites in the GHRH promoter completely abolished NFAT-induced transcriptional activation, suggesting that multiple binding sites function in a cooperative fashion.
Animal models of NFAT deficiency using gene targeting techniques showed that knockout of some subtypes of NFAT caused embryonic lethality (12, 13), and the other cases that survived had no dwarf phenotype (14), unlike the Gsh-1 knockout mice showing severe somatic growth defect (1). Whereas the Gsh-1 is the key transcription factor for both the development of GHRH neuron and the tissue-specific regulation of GHRH gene, NFAT seems to be involved in the acute regulation of GHRH gene transcription. In the latter case, some other signaling pathways, as mentioned above, are supposed to compensate for the calcium-mediated regulation in knockout mice. Furthermore, because NFATs consist of large family proteins, functional redundancy may explain the obscure impact of gene knockout.
From the clinical standpoint, it is also important to define whether administration of calcineurin/NFAT inhibitors such as cyclosporin A and FK506 cause impaired GH secretion in children. Again, because the function of NFAT can be replaced by another signaling system, we assume that the use of these immunosuppressants does not influence somatic growth. Interestingly, cyclosporin A is shown to have a positive effect on GH secretion at the pituitary level (15, 16), which might also cancel the possible negative effect of the reagent for the hypothalamus.
Finally, GHRH/GH axis is one of the antistress systems in the living organism, requiring an immediate response of the neuropeptide release/synthesis to stress such as hypoglycemia. Because the NFAT signaling system does not require de novo protein synthesis, calcium-induced dephosphorylation of cytosolic NFAT by calcineurin can immediately cause nuclear translocation and transcriptional induction of NFAT-dependent genes including GHRH. In this sense, NFAT signaling appears to be suitable to the calcium-regulated gene expression in the central nervous system.
MATERIALS AND METHODS
Cell Culture and Transfection
The neuronal cell line Gsh+/+ derived from the mouse hypothalamus (6) was grown in DMEM (Invitrogen Life Technologies, Carlsbad, CA) supplemented with 10% FBS, 100 U/ml penicillin, and 100 μg/ml streptomycin. Cells were maintained at 37 C in a humid atmosphere of 5% CO2. In each experiment, cells were plated in 12-well plates with approximately 80% confluency and were transfected transiently using FuGENE6 reagent (Roche, Indianapolis, IN). If needed, RSV-β-galactosidase expression vector was cotransfected as an internal control. In depolarization studies, 745.5 mg KCl or 584.4 mg NaCl were dissolved in 10 ml DMEM, and 130 μl were added to each well. After transfection and incubation, cells were harvested with lysis buffer containing 1% Triton X-100 and centrifuged, and the supernatant was used for luciferase assay.
Reporter Assays
Luciferase assay was performed as previously described (17). Briefly, 100 μl of each supernatant were added to 400 μl of reaction buffer, and the reactions were started by the infection of 200 μl of luciferin solution containing 0.2 mmd-luciferin (Sigma, St. Louis, MO). Light output was measured for 20 sec at room temperature using a luminometer (Lumat LB9501, EG&G Berthold, Munich, Germany). β-Galactosidase assay was carried out when equivalence of transfection efficacy needed to be evaluated using Galacto Light Plus Kit (Tropix, Inc., Bedford, MA) based on the manufacturer’s instructions.
RT-PCR
Total RNA was isolated from the Gsh+/+ cells using Sepazol RNA I Super reagent (Nacalai Tesque, Kyoto, Japan), or from the arcuate nucleus of the rat brain. In the latter case, whole brain was removed from 8-wk-old male Sprague-Dawley rats (Charles River, Wilmington, MA), frozen, and sliced into 1-mm-thick sections. Then total RNA was isolated from the punched-out samples. For RT-PCR, 1 μg of the total RNA was applied using SuperScript II First-Strand Synthesis System (Invitrogen Life Technologies) according to the manufacturer’s protocol. The cDNA sample obtained was used as templates for the subsequent PCR. Primer sets for RT-PCR analysis were designed so that they could span an intron to minimize the problems associated with genomic DNA contamination. The primers used are shown as follows:
Mouse NFAT1 common forward, 5′-TACTCACCCACCA-ACCAGCA-3′; mouse NFAT1a reverse, 5′-CCAGCTTTCTGAAGTGGCTG-3′; mouse NFAT1b reverse, 5′-AAGGTGTGTGTCTATCAACT-3′;
Mouse NFAT 1c reverse, 5′-CTTCCTGATGATTTCATT-AA-3′; mouse NFAT2 forward, 5′-ACATCATATATGAGCCCATC-3′; reverse, 5′-GGTGGAGACAGTCTTCCCCG-3′; mouse NFAT3 forward, 5′-GTGGCGTCGACTCGATCAGC-3′; reverse, 5′-ACTCCCTCCCCACACATCTG-3′;
Mouse NFAT4 forward, 5′-TAATATGTCAGCGAGTATTG-3′; reverse, 5′-GATCCAGTCACAATCATTTC-3′; mouse Gsh-1 forward, 5′-ACTGCATCTCTGTGGACAGC-3′;
Reverse, 5′-AGAGCGAAGAGCACTTGCAG-3′; mouse calcineurin catalytic subunit forward, 5′-CAGGGTGGTGAAA-GCCGTTC-3′; reverse, 5′-GGATGTCCCCACAAACTGTG-3′; mouse casein kinase 1 forward, 5′-GGAACATGCTCAAATTCGGTGC-3′; reverse, 5′-TCTGGGAGAAGTATTGCCAG-3′; mouseGHRH forward, 5′-CCACAACATCACAGAGTCCC-3′;
Reverse, 5′-TCTCCAGGGTCATCTGCTTG-3′; rat GHRH forward, 5′-CCACAACTGCACAGTGTCCC-3′; reverse, 5′-CCAGGGCCATCTGCTTGTCC-3′; rat NFAT1 forward, 5′-CACCCAATGCTGGCCGAGTC-3′; reverse, 5′-CTGCTGGCTGGCCGAGGAGG-3′; rat NFAT2 forward, 5′-TTCTGACTTCCCACCCGAGG-3′; reverse, 5′-CCTCCTGGACCGTTCCTACC-3′; rat NFAT4 forward, 5′-GACTTTGCTCCCAGAGAACA-3′; reverse, 5′-GATCCAGTCAACACTAGCTC-3′; rat calcineurin forward, 5′-CAGGGTGGTGAAAGCCGTTC-3′; reverse, 5′-GGATGTCCCCGCAAACTGTG-3′; rat Gsh-1 forward, 5′-ACTGCATCTCCGTGGACAGC-3′; reverse, 5′-AGAGCGAAGAGCACTTGCAG-3′.
Plasmid Construction
The 5′-flanking region of the rat GHRH gene (−472/+71; +1 designates the transcription start site), generously provided by Dr. Kelly E. Mayo (Northwestern University, Evanston, IL), was fused with the luciferase reporter gene in the pA3/Luc plasmid (GHRH-Luc). The hNFAT expression vector (pME human NFAT1c) and the empty vector (pME 18S) were kindly given to us by Dr. K. Maruyama (University of Tokyo, Tokyo, Japan). NFAT-responsive reporter plasmid (pNFAT-Luc, PathDetect NFAT Cis-Reporting System) was purchased from Stratagene (La Jolla, CA). Plasmids using thymidine kinase minimal promoter were constructed using pTAL vectors (Mercury Pathway Profiling System, CLONTECH, Palo Alto, CA), and the isolated DNA sequences were inserted between the KpnI/BglII sites of the plasmid. The nucleotide sequences of the sense oligonucleotides are as follows:
Site 1, 5′-CAGACAGTGAACAATTTTCCCCACTAAGAGA-3′; site 2, 5′-CCCACTAAGAGGGAAAAAAAAACATTTGTTA-3′; site 3, 5′-CCTTGGAAAAGCATAGTTTTAGGGAGAGTGA-3′; site 4, 5′-CCAGAAACCACTTTTCCCCATTAGTTTGCGA-3′; site 5, 5′-CCGCTGACAACACTTAGGGAAATGAAGGTAA-3′.
Site-Directed Mutagenesis
Plasmids containing mutated GHRH 5′-promoter were constructed using the QuikChange Multi Site-Directed Mutagenesis Kit (Stratagene) and 5′-phosphorylated primers (shown below; bold letters denote the substituted nucleotides) according to the manufacturer’s instructions. Because site 1 and site 2 are closely located, a different primer was used to mutate both sites 1 and 2 (site 1/2). The primers used are shown as follows:
Site 1-mut, 5′-pGGAGACAGTGAACAATTTTAAGCACTAAGAGGGAAAAAAAAAC-3′; site 2-mut, 5′-pCAATTTTCCCCACTAAGACTTAAAAAAAAACATTTGTTGG-3′; site 1/2-mut, 5′-pCAATTTTAAGCACTAAGACTTAAAAAAAAACATTTGTTGG-3′; site 3-mut, 5′-pCAGAATTATAGGGAACTCTTAAAAGCATAGTTTTAGGG-3′; site 4-mut, 5′-pGCACAGAAACCACTTTTAAGCATTAGTTTGCGTGATGC-3′; site 5-mut, 5′-pGACGCTGACAACACTTACTTAAATGAAGGTATAAATG-3′.
EMSA
Gsh+/+ cells were plated in 10 cm-diameter plates and transfected with pME-hNFAT1c, and then whole cell extracts were obtained using CelLytic-M Mammalian Cell Lysis/Extraction reagent (Sigma). Biotin-labeled probes containing the rat GHRH promoter fragments were synthesized as shown below and annealed. EMSA was carried out using LightShift Chemiluminescent EMSA Kit (Pierce, Rockford, IL). Binding reactions were loaded onto an 8 × 8 × 0.1-cm 6% polyacrylamide gel in 0.5× TBE buffer and electrophoresed at 100 V at 4 C for 2 h. Biotin-labeled double-stranded DNAs were transferred to positive charge nylon membrane (Hybond-N, Amersham Pharmacia Biotech, Buckinghamshire, UK) using a capillary method. Biotin-labeled DNA was integrated with streptavidin-horseradish peroxidase conjugate. Finally, chemiluminescence was detected using Light Capture imaging apparatus (AE-6961, ATTO, Tokyo, Japan). The sense probes used are shown as follows: consensus sense, 5′-ATGAGGTGGAAAATGCTCAG-3′; site 1 sense, 5′-AGACAGTGAACAATTTTCCCCACTAAGAG-3′; site 2 sense, 5′-CCACTAAGAGGGAAAAAAAAACATTTGTT-3′; site 3 sense, 5′-CTTGGAAAAGCATAGTTTTAGGGAGAGTG-3′; site 4 sense, 5′-CAGAAACCACTTTTCCCCATTAGTTTGCG-3′; site 5 sense, 5′-CGCTGACAACACTTAGGGAAATGAAGGTA-3′.
[Ca2+]i Measurement
The details of the method used have been described elsewhere (18, 19). Briefly, Gsh+/+ cells, seeded on 22-mm round cover glasses and placed in 35-mm-diameter culture dishes, were cultured in DMEM containing 10% heat-inactivated FBS, and kept in humidified air containing 5% CO2 at 37 C. Electrophysiological studies and [Ca2+]i measurements were performed on the third day after plating the cells. The standard bath solution was (in mm): 128 NaCl, 5 KCl, 1 MgCl2, 2.5 CaCl2, and 10 HEPES (Na salt, pH 7.4). High potassium solution was made by increasing the potassium concentration of the bath solution to 80 mm and decreasing extracellular sodium isoosmotically. During the experiments, the extracellular solution was continuously perfused by a peristaltic pump. The time needed to change the whole extracellular solution in our peristaltic pump system was about 2 min. Agents were applied by changing the perfusing solution. Cells were loaded with fura2 (Molecular Probes, Eugene, OR) by incubating with 2 μm fura2/AM in Hanks’ balanced salt solution containing 0.1% BSA for 40 min at room temperature. [Ca2+]i measurements were performed on a Nikon Diaphot microscope (Nikon, Tokyo, Japan). Cells were excited at 340 nm and 380 nm alternately at a frequency of 100 Hz with CAM220 (Nihonbunko, Tokyo, Japan). Fluorescent light was collected from a single cell. A band filter was used to monitor the fluorescent emission at 510 nm. The cytosolic free Ca2+ concentration was determined from the equation [Ca2+]i =K(R − Rmin)/(Rmax − R) (1). In this equation, K represents Kd (Fmin/Fmax), where Kd is the dissociation constant of fura2 (130 nm at 25 C), and Fmax/Fmin is the ratio of Ca2+-free and Ca2+-bound fura2 fluorescence at 380 nm. Rmin is the 340/380 fluorescence ratio of Ca2+-free fura 2, and Rmax is the 340/380 ratio of Ca2+-bound fura 2. Calibration was performed by permeabilizing the cell to Ca2+ with 2 μm digitonin. Cells were first permeabilized in Ca2+-free saline [5 mm EGTA, 150 mm KCl, and 10 mm HEPES (pH 7.2)], for determination of Rmin and Fmin, and then in high Ca2+ saline [2.5 mm CaCl2, 150 mm KCl, and 10 mm HEPES (pH 7.4)] for determination of Rmax and Fmax. The [Ca2+]i traces shown in the figures were filtered with a bandwidth of 1 Hz to reduce the noise. Agonists were applied by changing the bath solution with a peristaltic pump. In the [Ca2+]i measurement experiment it took about 30 sec to change the bath solution in this peristaltic pump system.
Western Blotting Analysis
Human NFAT1c cDNA (provided by Dr. K. Maruyama, University of Tokyo) was inserted into pcDNA3.1/myc-His A (Invitrogen Life Technologies) at the EcoRI/SpeI site. The primers used are shown as follows: NFAT1c myc/His forward, 5′-GGTAAGAATTCGCCACCATGAACGCCCCCGAGCGGCA-3′; reverse, 5′-ATATAGCGGCCGCGTCTGATTTCTGGCAGGAG-3′. Gsh+/+ cells were plated in 10 cm-diameter dishes and transfected with/without pME-NFAT1c myc/His. After incubation with 80 mm KCl for the indicated time, the nuclear fraction of the cell extracts was obtained using NE-PER Nuclear and Cytoplasmic Extraction Reagents (Pierce). Proteins (25 μg) were separated on 7.5% SDS-PAGE and transferred onto polyvinylidene difluoride (Immobiline P membrane, Millipore, Bedford, MA) in Tris-glycine buffer containing 20% methanol. The membrane was then blocked in 5% fat-free milk in PBS/0.1% Tween 20. After overnight incubation with monoclonal mouse antibodies to NFAT1 (sc7295, dilution 1:1000; Santa Cruz Biotechnology, Santa Cruz, CA) or monoclonal mouse antibodies to c-Myc (sc-40, dilution 1:1000; Santa Cruz Biotechnology), the blot was incubated with monoclonal goat antibodies to mouse IgG-horseradish peroxidase (sc2031, dilution 1:1000; Santa Cruz Biotechnology). Finally, proteins were visualized using a Light Capture imaging apparatus (ATTO).
Immunohistochemical Staining
Eight-week-old male Sprague-Dawley rats (Charles River) were decapitated between 1000 and 1200 h, corresponding to 4–6 h after lights on. Animal surgery and care were in accordance with the Nagoya University institutional guidelines complying with the National Institutes of Health policy. Immunohistochemistry using representative formaldehyde-fixed, paraffin-embedded tissue blocks was performed after microwave pretreatment. Polyclonal goat antibodies to GHRH (sc-10283, dilution 1:20; Santa Cruz Biotechnology) or polyclonal goat antibodies to NFAT (sc-1151, dilution 1:20; Santa Cruz Biotechnology) was reacted overnight at 4 C. After washing, the samples were incubated for 1 h at 37 C with the biotinylated rabbit antigoat Igs (dilution 1:400; E0466, Dako, Carpinteria, CA) and followed by another washing. The samples were then incubated with streptavidin fluorescein isothiocyanate (1:100; F0422, Dako) for 1 h at 37 C. The sections and cells were examined with a confocal imaging system IX70 (Olympus, Tokyo, Japan). As the excitation source, a krypton-argon ion laser (LG-PS2; Olympus) was used. Fluorescein isothiocyanate was imaged with 488-nm excitation and a 505- to 540-nm bandpass emission filter.
Data Analyses
Samples in each group of the experiments are in triplicate, and data are presented as mean ± se. When statistical analyses were performed, data were compared by one-way ANOVA with Fisher’s protected least significant difference test, and P < 0.05 was considered significant.
Acknowledgments
We are grateful to Drs. Kelly E. Mayo (Northwestern University, Evanston, IL), Roger J. Davis (University of Massachusetts Medical School, Worcester, MA), and Steven S. Potter (University of Cincinnati, Cincinnati, OH) for providing the rat GHRH gene, dominant NFAT expression vectors, and Gsh+/+ cells, respectively. We also thank Dr. Kazuo Maruyama (University of Tokyo, Tokyo, Japan) for providing an NFAT expression vector. Excellent technical assistance by Ms. Machiko Kambayashi and Ms. Tatsuyo Miura is also greatly appreciated.
Abbreviations:
- NFAT,
Nuclear factor of activated T cells;
- pTAL-Luc,
thymidine kinase minimal promoter-luciferase construct.