-
PDF
- Split View
-
Views
-
Cite
Cite
M. I. Galindo, Y. Bigot, M. D. Sánchez, G. Periquet, L. Pascual, Sequences Homologous to the hobo Transposable Element in E Strains of Drosophila melanogaster, Molecular Biology and Evolution, Volume 18, Issue 8, August 2001, Pages 1532–1539, https://doi.org/10.1093/oxfordjournals.molbev.a003939
- Share Icon Share
Abstract
Hobo is one of the three Drosophila melanogaster transposable elements, together with the P and I elements, that seem to have recently invaded the genome of this species. Surveys of the presence of hobo in strains from different geographical and temporal origins have shown that recently collected strains contain complete and deleted elements with high sequence similarity (H strains), but old strains lack hobo elements (E strains). Besides the canonical hobo sequences, both H and E strains show other poorly known hobo-related sequences. In the present work, we analyze the presence, cytogenetic location, and structure of some of these sequences in E strains of D. melanogaster. By in situ hybridization, we found that euchromatic hobo-related sequences were in fixed positions in all six E strains analyzed: 38C in the 2L arm; 42B and 55A in the 2R arm; 79E and 80B in the 3L arm; and 82C, 84C, and 84D in the 3R arm. Sequence comparison shows that some of the hobo-related sequences from Oregon-R and iso-1 strains are similar to the canonical hobo element, but their analysis reveals that they are substantially diverged and rearranged and cannot code for a functional transposase. Our results suggest that these ubiquitous hobo-homologous sequences are immobile and are distantly related to the modern hobo elements from D. melanogaster.
Introduction
Drosophila melanogaster has been extensively used as a model to study the dynamics of transposable elements and the relationship with their host genomes. Much effort has been devoted to questions such as their putative parasitic origin, the modes of containment of the number of copies, the specificity of insertion, and the possibility of horizontal transfer between species. In the present work, we analyze hobo-related sequences that coexist with their modern relatives, a situation that has previously been described for other transposable elements (Vaury et al. 1990 ; Bucheton et al. 1992 ). The analysis of these types of sequences can help us to understand the evolutionary history of the transposable element families.
Hobo belongs to the hAT family of transposable elements, which is named after its three principal members: hobo of D. melanogaster, Ac of Zea mays, and Tam3 of Anthirrinum majus (Calvi et al. 1991 ). The hAT family also has representatives in other drosophilid and nondrosophilid insects (Warren, Atkinson, and O'Brochta 1994 ) and in Caenorhabditis elegans (Bigot, Augé, and Periquet 1996 ). Full-length, active hobo elements of D. melanogaster are 3 kb long with 12-bp terminal inverted repeats and contain a 1.9-kb open reading frame (ORF) (Streck, MacGaffey, and Beckendorf 1986 ; Calvi et al. 1991 ). Hobo is also similar to P and I elements in that it is responsible for a system of hybrid dysgenesis whose effects include sterility by gonadal dysgenesis, hypermutability, and chromosomal rearrangements (Blackman et al. 1987 ; Yannopoulos et al. 1987 ; Stamatis et al. 1989 ; Lim and Simmons 1994 ).
The patterns of distribution of different hobo-related sequences in the Drosophila genus can give us some insight into its recent past. Daniels, Chovnick, and Peterson (1990) detected hobo-homologous sequences only in the melanogaster and montium subgroups and proposed that the observed distribution would have required at least two independent horizontal transfers of the hobo element from other species. The other species of the melanogaster complex, D. melanogaster, Drosophila simulans, Drosophila mauritiana, and Drosophila sechellia, also contain potentially active, full-sized hobo elements along with species-specific defective elements (Daniels, Chovnick, and Peterson 1990 ; Periquet et al. 1990 ) with high sequence homology (Simmons 1992 ). Surveys of D. melanogaster stocks from different locations and dates of capture (Streck, MacGaffey, and Beckendorf 1986 ; Periquet et al. 1989a, 1989b ) revealed that stocks captured before the 1960s lack canonical hobo elements (E strains), while more recent ones contain complete and defective hobo elements (H strains). Analyses of various D. simulans strains (Boussy and Daniels 1991 ) revealed a similar temporal distribution of H and E strains. In these two species, only H strains show complete and species-specific defective hobo elements, but both E and H strains show hobo-related sequences that yield a different restriction pattern of weaker bands in Southern blot analyses. Pascual and Periquet (1991) suggested that these sequences might be the remnants of a putative relictual hobo element.
Many of the hobo-homologous sequences in E strains seem to be heterochromatic and not polytenized in salivary gland chromosomes (Di Franco et al. 1997 ). In the present work, we identified euchromatic sequences that were similar to hobo. This allowed us to determine their location along the chromosomal arms and to assess their relationship to the canonical hobo element by the analysis of their sequence and structural integrity.
Materials and Methods
Strains of Drosophila melanogaster
For the analysis of the putative ancestral hobo-related sequences, six E strains were used. Three of these strains have been previously described and used as E strains in hobo mobility assays: Hikone and Oregon-K are described in Galindo et al. (1995) , and Berlin-K is described in Yannopoulos et al. (1994). ry506 was established from a Canton-S stock (Gelbart et al. 1974 ), ry1 was isolated by Bridges (described in Glassman and Mitchell 1959 ), and st1 was isolated by Richards (1918) . These last three stocks were obtained from the Bloomington Stock Center (stock numbers 225, 584, and 605, respectively).
The hobo-homologous sequences studied here originate from European Drosophila Genome Project (EDGP) genomic clones from the Oregon-R strain (Siden-Kiamos et al. 1990 ) and from the published sequences of the Berkeley Drosophila Genome Project (BDGP) from the iso-1 strain (Kimmerly et al. 1996 ).
Plasmids
pHFL1 (Blackman et al. 1989 ) contains the full-size autonomous hobo element HFL1 plus 0.5 kb of flanking genomic DNA from cytogenetic locus 94E cloned in pBluescript KS. It was used as a probe for in situ hybridization and to detect hobo-homologous sequences in cosmids containing DNA from cytogenetic region 38C.
In Situ Hybridization
Standard techniques were employed in all of the hybridizations, but it must be noted that for the detection of sequences of small size and/or reduced homology, extra care must be taken to reach a balance between good sensitivity and acceptable background. The detailed protocol can be obtained from us. Salivary glands from late third-instar larvae were dissected in 100 mM NaCl and fixed for a few seconds in 45% acetic acid and then for between 1 and 3 min in acetic acid : lactic acid : water (3:1:2). The chromosomes were spread, squashed, and dehydrated in ethanol. The pretreatment, hybridization, and detection procedures are based on the protocol described by de Frutos, Kimura, and Peterson (1990) with the following modifications. The plasmid pHFL1 was nonradioactively labeled with the digoxigenin-based system of Boehringer-Mannheim by random priming. For each slide to be hybridized, 20 ng of digoxigenin-labeled probe in 20 μl of hybridization solution was used, and the hybridization was carried out overnight at 37°C. The subsequent low-stringency washes were carried out in 4× SSC at 35°C, instead of the usual 42°C.
For each strain, five larvae were analyzed, and we ensured that at least five nuclei on each slide contained all of the bands described. The cytogenetic loci of the stainings were determined with the help of the photographic maps of Lefevre (as reproduced in Lindsley and Zimm 1992 ) and Heino, Saura, and Sorsa (1994) .
Cloning and Sequencing
Cosmids from cytogenetic region 38C, obtained from the EDGP, were double-digested with EcoRI and HindIII restriction enzymes and subcloned into pBluescript SK+. Putative hobo-homologous fragments were detected by screening with pHFL1 (described above) as a probe. Positive clones were sequenced and compared with the sequence of hobo element HFL1 (Blackman et al. 1989 ).
Sequence Search and Analysis
Sequences homologous to the hobo element were searched in the BDGP database of Drosophila genomic sequences (Kimmerly et al. 1996 ; BDGP, personal communication) through the BDGP server (http://www.fruitfly.org) with the BLAST search engine (Altschul et al. 1990 ). The cytogenetic locations of all of the clones selected for further analysis had been already determined (Hartl et al. 1994 ; BDGP, personal communication). The sequence alignments of hobo-homologous sequences to hobo element HFL1 were performed with the LALIGN local alignment tool (Huang and Miller 1991 ).
Results
Cytogenetic Locations of the hobo-Homologous Sequences in Polytene Chromosomes
The hobo-homologous sequences that appear in all of the strains of D. melanogaster can be distinguished from the canonical sequences in Southern blot analyses by two features. First, hobo elements have two XhoI restriction sites close to either end, and a digestion with this enzyme yields intense bands corresponding to the accumulation of the internal fragments of elements with the same structure. Complete 3-kb hobo elements produce a 2.6-kb band, and each particular class of defective element yields a characteristic band of smaller size. In contrast, hobo-homologous sequences produce numerous faint high-molecular-weight bands. Second, hobo-homologous sequences require much longer exposure times. Such hobo-homologous sequences have already been described in the Hikone strain (Galindo et al. 1995 ). Five further E strains (Oregon-K, Berlin-K, ry506, ry1, and st1) were tested for this study. All of them showed the expected high-molecular-weight hobo-homologous sequences in Southern blot analyses (data not shown). Our first goal was to determine if there were hobo-homologous sequences in the euchromatin or if they were restricted to the heterochromatin. In situ hybridization to polytene chromosomes of all six E strains was carried out with a probe containing a complete hobo element.
In all of the strains analyzed by in situ hybridization, the same nine cytogenetic loci were stained. These loci were 38C in the 2L arm; 42B and 55A in the 2R arm; 79E and 80B in the 3L arm; and 82C, 84C, 84D, and 94E in the 3R arm. Examples of these sites in the Hikone strain are shown in figure 1 . Of these loci, 94E might correspond to the genomic DNA present in the probe, and therefore only the other eight loci would contain sequences homologous to hobo. The sites had the same relative intensities in all six strains. The weakest ones did not appear in all of the nuclei analyzed, and when they did, they were difficult to detect. These were the 82C site (in a decondensed chromatin area, which usually appears as a line of dots), 84D, and the sites in 79E and 80B (extremely weak and only detectable in chromosomes with poor squashing and therefore bad morphology). However, in any case, all of the sites described always appeared in all five individuals analyzed for each strain. The 84C locus was of intermediate intensity, while the sites in 38C, 42B, and 55A were always easily detectable as strong bands. The site in 94E, corresponding to the ∼500 bp flanking genomic DNA present in the probe, usually appeared as a faint band, but it was always visible in all of the nuclei. Our results indicate that some of the hobo-homologous sequences may be present in euchromatic locations and that these are conserved in the strains under analysis.
Identification of hobo-Homologous Sequences
Our next objective was to verify that these sites corresponded to hobo-homologous sequences, rather than being an effect of nonspecific hybridization in the low-stringency conditions employed, and if so, to study the structural integrity and similarity to the canonical hobo element. We considered two possible sources for the sequences. First, we tried to detect hobo sequences in one of the candidate cytogenetic loci revealed by in situ hybridization. The locus 38C is particularly interesting because it is one of the sites that shows the strongest signal in the present experiments and was detected in normal in situ hybridization conditions in previous experiments with hobo in different strains (Ladevèze et al. 1994 ; Yannopoulos, Zabalou, and Alahiotis 1994 ). In order to detect possible sequences that may hybridize to hobo, genomic cosmid clones of the OregonR strain from the EDGP that mapped to 38C were searched through the flybase server (http://flybase.bio.indiana.edu). Only one out of eight available clones that map to 38C1-10, cosmid 28H10, hybridized to the probe pHFL1. The cosmid was double-digested with EcoRI and HindIII, and the resulting fragments were subcloned and again hybridized to pHFL1. Two of these fragments yielded sequences with similarity to hobo. The portions of these sequences that showed such similarity were used for the subsequent alignments to the HFL1 sequence and will be referred to as h38C-1 and h38C-2.
To find relevant sequences corresponding to loci different from 38C, we searched the BDGP database of genomic sequences (http://www.fruitfly.org) with the BLAST search engine using the sequence of hobo element HFL1 (GenBank accession number M69216) as a query against the genomic clones sequenced by the Drosophila genome projects. Significantly similar matches were obtained to some clones mapped to autosomes 2 and 3 (described in table 1 ). High scores were obtained for sequences from clones mapped to loci coincident or close to the ones detected by us by in situ hybridization, namely, 38C, 55AB, and 84B. Other homologous sequences belonged to clones from 31A and 36AC. The portions of these sequences that were homologous to hobo and were used in the alignments were named according to their locations, as noted in table 1 . The sequence derived from clone DS07993 will not be discussed because it is identical to the one from DS02528 and also from 36AC (see table 1 ). Other sequences were also discarded because they represented modern deleted elements present in the iso-1 strain (BDGP, personal communication). In fact, they showed an atypically high similarity to HFL1 (99%) compared with the rest of the sequences and had a structure typical of defective hobo elements, with both ends intact and a large deletion between positions 905 and 2448. The left breakpoint was only 1 bp away from the one in the Th element. The high similarity and presence of both XhoI sites would yield a clear band in Southern blot.
All of the sequences were aligned to hobo element HFL1. The best results were obtained by the local alignment method LALIGN, as the analyzed sequences contained numerous gaps, insertions, and duplications relative to the canonical hobo sequence. For the sake of clarity, we will only refer to nucleotide positions of hobo element HFL1 when we describe the structure and degree of similarity of the analyzed sequences. In figure 2 , graphical representations of the similarities based on the local alignments are shown, but these alignments are available from us on request.
Sequences in 38C
The 38C locus yielded the longest stretches of sequence similarity to the hobo element. In addition to h38C-1 and h38C-2 characterized by us from the genomic clones of Oregon-R, we found the P1-clone-derived sequence h38C-3 from the iso-1 bank. All three sequences share a number of features (figs. 2A and B ). h38C-1 shows similarity to positions 30–316 of HFL1, with an insertion between positions homologous to 192–193. h38C-3 is also similar to the 5′ region of HFL1 from position 93 to position 316 and with a similar insertion. Both h38C-1 and h38C-3 lack one of the L repeats present in this region of the hobo element. The homology to hobo is then interrupted in both cases by a fragment of 0.4 kb homologous to the 5′ end of transposable element 1360, and then the homology to hobo is resumed from position 330. A closer inspection of the right limit between 1360- and hobo-homologous regions reveals that there is a stretch in h38C-1 and h38C-3 that is similar to both adjacent transposon sequences, suggesting that recombination between a similar sequence present in both hobo and 1360 might have originated this chimera. In figure 3A, the corresponding region in h38C-3 is illustrated.
In the region 3′ of this 1360-homologous region, h38C-1 is homologous to hobo positions 330–1818, with a gap between positions 1650 and 1755. h38C-2 is 98.6% similar to h38C-1 in a 1,825-bp overlap that includes the 1360- and right hobo-homologous fragments up to their right ends (demarcated by a HindIII site), but the sequence 5′ of h38C-2 is not similar to either h38C-1 or hobo. h38C-3 is also similar to hobo, from position 330 to position 2354, but the structure of this 3′ region is different from that of h38C-1. First, there is a direct repeat that in hobo is present as a single sequence from position 1283 to position 1349. Then, regions homologous to hobo in positions 1490–1649 and 1799–1957 are missing from h38C-3. This second region contains the hobo S repeats. There is a further region similar to hobo positions 2362–2580.
Sequences in Other Loci
Two sequences corresponding to 55A appeared (fig. 2C and D ). h55A-1 shows homology to five different fragments of hobo, including the 5′ end up to position 130, but a considerable portion of the transposon is absent. In this case, the border between the third and the fourth hobo-homologous regions also indicates a possible recombinational event (fig. 3B ). h55A-2 is a small sequence that shows similarity only to the first 128 bp of the 5′ end of hobo, in a fragment similar to the 5′-end-homologous zone of h55A.
h84B (fig. 2E ) is weakly similar to the 5′ end of hobo (from nucleotide 5 to nucleotide 240), and then there are two fragments that are >80% similar to the same region of HFL1, which produces a direct duplication of the region homologous to positions 459–898.
There are two other sequences with short stretches of similarity. h36B (fig. 2F ) shows similarity to three regions of hobo: the 5′ end (positions 2–395), a short region from position 2294 to position 2426 with an insertion between positions 2359 and 2360, and the 3′ end from 2796. h31A (fig. 2G ) has some similarity to two short fragments of HFL1, again possibly brought together by recombination (fig. 3C ).
Discussion
Our analyses of hobo-homologous sequences present in E strains of D. melanogaster revealed that at least some of them are localized in the euchromatic portion of the genome. This allowed us to accurately determine their cytogenetic locations in different strains and to obtain sequences in order to investigate their relationship with the modern hobo element.
Structure of hobo-Homologous Sequences
The analyses of hobo-related sequences in Oregon-R and iso-1 revealed that they show differences in nucleotide sequence when compared with the canonical hobo element and when compared with each other. The longest portions show a similarity typically ranging from 80% to 88%. None of the sequences can code for anything substantially homologous to the hobo transposase, with the only possible exception being the sequences in 38C. In these sequences, the homology to hobo in the main central portion starts at position 330. This is in a codon that corresponds to the second of two methionines at the beginning of the hobo ORF, but 300 bp downstream of this codon in h38C-1 and h38C-2, and 240 bp downstream of it in h38C-3, we found the first frameshift mutation followed by many others.
Although the homologies to the hobo element are fragmented in different stretches of the compared sequences, they always keep the same orientation. The presence of adjacent direct repeats that in the canonical hobo element are represented as single sequences, and the presence of deletions flanked by partially homologous sequences suggests that recombinational events must have played a role in the origin of these sequences. For the 1360-hobo chimeras in 38C, the high homology found in the overlapping regions leaves little room to doubt that these elements have been brought together by recombination of partially homologous sequences.
These hobo-homologous sequences are clearly different from the deleted hobo elements that are common in wild D. melanogaster populations, such as the Th element. The typical defective elements present in all of the melanogaster complex species differ from the complete hobo element in that they have a single large deletion that removes the central part of the sequence but does not affect the element ends (for a review, see Periquet et al. 1994 ). In contrast, the hobo-homologous sequences characterized by us have suffered more complex rearrangements.
A further question posed by the presence of these putative ancient sequences, which will have to be addressed, is that of the possible interaction with the mobile hobo sequences. If the conservation of stretches of sequence is high enough, they may titrate and/or interfere with the proteins that hobo requires for its transposition, either the transposase or host-encoded products, a process known as homology-dependent gene silencing. Such events have been observed in I elements after the introduction of an artificial defective I element (Jensen et al. 1995 ) or even a small fragment of this transposon in a transgene with its translation impaired by the introduction of stop codons in all three reading frames (Jensen, Gassama, and Heidmann 1999 ).
Cytogenetic Locations of the hobo-Homologous Sequences
Most of the inactive transposable elements are located in the heterochromatin (for a review, see Dimitri and Junakovic 1999 ). Fluorescent in situ hybridization to mitotic chromosomes (Carmena and González 1995 ; Pimpinelli et al. 1995 ) showed that in the mitotic heterochromatin there is an absolutely conserved distribution pattern of different transposable elements (copia, mdg1, mdg3, gypsy, Doc, blood, F, G, I, and FB). On the other hand, P and hobo show polymorphisms in their heterochromatic locations. Di Franco et al. (1997) also found that heterochromatic copies of hobo-homologous sequences (referred to as non-hobos) give heterogeneous patterns in Southern blots with genomic DNA from different E stocks. However, this heterogeneity does not necessarily imply mobility. Two of our sequences, h38C-1 and h38C-2, are clearly different copies present in the same region in Oregon-R. We cannot tell if h38C-3 is a third member yet to be identified in Oregon-R or a different version of this sequence characteristic of the iso-1 strain. If the second possibility were true, it could produce a different band pattern in Southern blot despite the fact that it occupies the same locus in both strains.
Our results reveal that hobo-homologous sequences are not restricted to the pericentric heterochromatin. In the six strains analyzed, the same cytogenetic loci were stained, each with identical relative intensities, suggesting that the sequences detected are in fixed positions in the D. melanogaster genome. For the strongest sites detected by in situ hybridization, candidate sequences have been found in 38C, 55A, and 84B. The small size and low homology of the sequences from these loci can justify the absence of hybridization signals in 31A and 36B.
The low similarity to hobo elements may explain why these sequences have not previously been detected in in situ hybridization experiments. In fact, only by using low-stringency conditions were we able to detect them consistently in every strain. In any case, the strongest among these sites have been sporadically mentioned in the literature. 38C can be considered a very strong candidate for a preferential site of insertion for hobo based on experiments by Yannopoulos, Zabalou, and Alahiotis (1994) and Ladevèze et al. (1994, 1998). 55A has been described as a hot spot for hobo (Smith et al. 1993 ), and 42B has been described as a hot spot for several elements (Biémont 1993 ). In light of our results, it is not possible to discard the possibility that the first two are not sites of preferential occupation by the hobo element but, instead, sites that are commonly detected because they contain a hobo-homologous sequence.
Origin of the hobo-Homologous Sequences
The scenario described here for hobo-homologous sequences, divergence in sequence and fixed positions in particular sites among strains of distant geographical and temporal origins, suggests that they have remained immobile for a long period, probably since the speciation of D. melanogaster.Boussy and Daniels (1991) proposed that the distribution of hobo sequences in D. melanogaster and its sibling species can only be explained with two different introductions of the hobo element, one before the divergence of the melanogaster and montium subgroups, and the second later in the ancestor of the melanogaster complex. The sequences described here may correspond to the remnants of the hobo elements from one of these invasions. If this were true, the more ancient hobo or hobo-related element would have lost its mobility and become a moderately repetitive sequence in fixed positions of the genome.
Interestingly, a similar situation has been described for the I element of D. melanogaster. Inactive elements homologous to the I element have been found in both inducer (with mobile I elements) and reactive (without mobile I elements) strains of D. melanogaster (Bucheton et al. 1992 ). Four I-homologous sequences found in a reactive strain showed numerous rearrangements with respect to the canonical I element, similar to the ones described in the present work (Vaury et al. 1990 ). Therefore, inactivation and rearrangement of transposable-element sequences could be a common process in the Drosophila genome. An insight into the dynamics of this process can be obtained from the analyses of other transposable-element sequences putatively more recently inactivated. Immobile copies have been described with a structure similar to that of typical defective elements, like the P elements found in Drosophila species of the obscura group (Paricio et al. 1991 ; Miller et al. 1992 ), or with a structure similar to that of complete elements, like a fixed mariner element found in D. sechellia (Capy et al. 1991 ). It is then possible that fixed copies are initially structurally similar to typical mobile elements but then start to recombine with other sequences from the middle repetitive fraction of the genome, as our results suggest. A more extensive catalog of hobo-homologous sequences and other mobile element families, including heterochromatic copies, will hopefully help to elucidate their origin and their possible functional or structural roles.
Manolo Gouy, Reviewing Editor
Present address: School of Biological Sciences, University of Sussex, Brighton, England.
Keywords: hobo, Drosophila transposable elements ancestral hobo elements polytene chromosomes
Address for correspondence and reprints: Luis Pascual, Departament de Genètica, Universitat de València, ℅ Dr. Moliner, 50. 46100 Burjassot, Valencia, Spain. [email protected].
Table 1 Genomic Sequences from BDGP with Significant Homology to HFL1
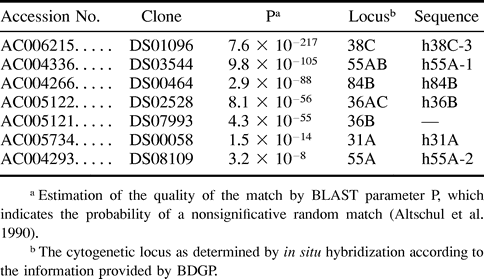
Table 1 Genomic Sequences from BDGP with Significant Homology to HFL1
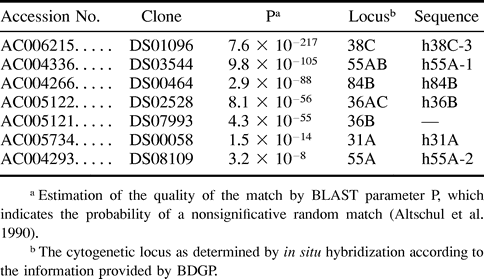
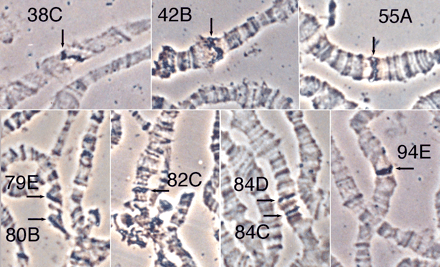
Fig. 1.—In situ hybridization to polytene chromosomes of the Hikone strain with the pHFL1 probe. In each panel, hybridization signals are marked with arrows, and the corresponding loci are indicated
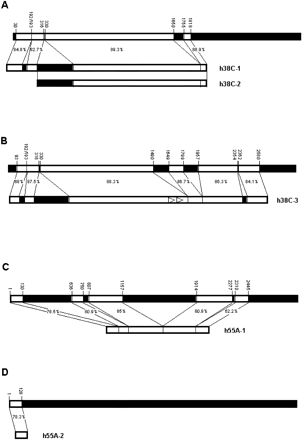
Fig. 2.—Comparison of the hobo-homologous sequences to the hobo element HFL1. Coordinates of HFL1 are indicated above the diagram. White boxes indicate the stretches that are similar between both sequences compared, and black boxes indicate lack of similarity. Empty arrowheads represent direct repeats present in hobo-homologous sequences
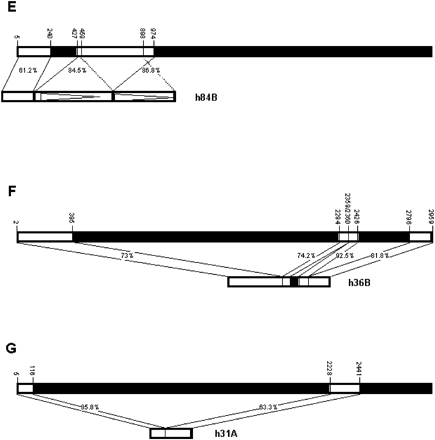
Fig. 2 (Continued)
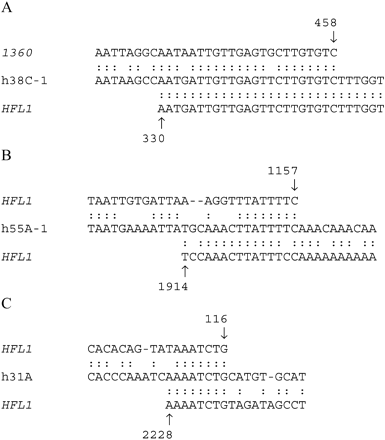
Fig. 3.—Putative sites of recombination in the hobo-homologous sequences. The hobo-homologous sequences in the center are aligned to the sequences flanking the candidate recombination breakpoints as indicated in the text. See also figure 2
This research was financed by projects PB96-0803 and HF-031 of Ministerio de Educacion y Ciencia and project GR00-32 of Conselleria de Cultura i Educació de la G. Valenciana. We thank the Drosophila genome projects for making sequences and clones freely available to the scientific community.
References
Altschul S. F., W. Gish, W. Miller, E. W. Myers, D. J. Lipman,
Biémont C.,
Bigot Y., C. Augé-Gouillou, G. Periquet,
Blackman R. K., R. Grimaila, M. Macy, D. Koeehler, W. M. Gelbart,
Blackman R. K., M. Macy, D. Koehler, R. Grimaila, W. M. Gelbart,
Boussy I. A., S. B. Daniels,
Bucheton A., C. Vaury, M. C. Chaboissier, P. Abad, A. Pélisson, M. Simonelig,
Calvi B. R., T. H. Hong, S. D. Findley, W. M. Gelbart,
Capy P., K. Maruyama, J. David, D. Hartl,
Carmena M., C. González,
Daniels S. B., A. Chovnick, I. A. Boussy,
de Frutos R., K. Kimura, K. Peterson,
Di Franco C., A. Terrinoni, P. Dimitri, N. Junakovic,
Dimitri P., N. Junakovic,
Galindo M. I., V. Ladevèze, F. Lemeunier, R. Kalmes, G. Periquet, L. Pascual,
Gelbart W. M., M. McCarron, J. Pandey, A. Chovnick,
Glassman E., H. K. Mitchell,
Hartl D. L., D. I. Nurminsky, R. W. Jones, E. R. Lozovskaya,
Heino T. I., A. O. Saura, V. Sorsa,
Huang X. Q., W. A. Miller,
Jensen S., L. Cavarec, M. P. Gassama, T. Heidmann,
Jensen S., M. P. Gassama, T. Heidmann,
Kimmerly W., K. Stultz, S. Lewis, et al. (11 co-authors).
Ladevèze V., M. I. Galindo, N. Chaminade, L. Pascual, G. Periquet, F. Lemeunier,
Ladevèze V., M. I. Galindo, L. Pascual, G. Periquet, F. Lemeunier,
Lim J. L., M. J. Simmons,
Miller W. J., S. Hagemann, E. Reiter, W. Pinsker,
Paricio N., M. Pérez-Alonso, M. J. Martnez-Sebastián, R. de Frutos,
Pascual L., G. Periquet,
Periquet G., M. H. Hamelin, Y. Bigot, K. Hu,
Periquet G., M. H. Hamelin, Y. Bigot, A. Lepissier,
Periquet G., M. H. Hamelin, R. Kalmes, J. Eeken,
Periquet G., F. Lemeunier, Y. Bigot, M. H. Hamelin, C. Bazin, V. Ladevèze, J. Eeken, M. I. Galindo, L. Pascual, I. Boussy,
Pimpinelli S., M. Berloco, L. Fanti, P. Dimitri, S. Bonacorsi, E. Marchetti, R. Caizzi, C. Caggese, M. Gatti,
Richards M. H.,
Siden-Kiamos I., R. D. C. Saunders, L. Spanos, T. Majerus, J. Treanear, C. Savakis, C. Louis, D. Glover, M. Ashburner,
Simmons G. M.,
Smith D., J. Wohlgemouth, B. R. Calvi, I. Franklin, W. M. Gelbart,
Stamatis N., M. Monastirioti, G. Yannopoulos, C. Louis,
Streck R. D., J. E. Macgaffey, S. K. Beckendorf,
Vaury C., P. Abad, A. Pelisson, A. Lenoir, A. Bucheton,
Warren W. D., P. W. Atkinson, D. A. O'Brochta,
Yannopoulos G., N. Stamatis, M. Monastirioti, P. Hartzopoulos, C. Louis,
Yannopoulos G., S. Zabalou, S. N. Alahiotis,