-
PDF
- Split View
-
Views
-
Cite
Cite
Richard P. M. A. Crooijmans, Rosilde J. M. Dijkhof, Tineke Veenendaal, Jan J. van der Poel, Robert D. Nicholls, Henk Bovenhuis, Martien A. M. Groenen, The Gene Orders on Human Chromosome 15 and Chicken Chromosome 10 Reveal Multiple Inter- and Intrachromosomal Rearrangements, Molecular Biology and Evolution, Volume 18, Issue 11, November 2001, Pages 2102–2109, https://doi.org/10.1093/oxfordjournals.molbev.a003751
- Share Icon Share
Abstract
Comparative mapping between the human and chicken genomes has revealed a striking conservation of synteny between the genomes of these two species, but the results have been based on low-resolution comparative maps. To address this conserved synteny in much more detail, a high-resolution human-chicken comparative map was constructed from human chromosome 15. Mapping, sequencing, and ordering of specific chicken bacterial artificial chromosomes has improved the comparative map of chromosome 15 (Hsa15) and the homologous regions in chicken with almost 100 new genes and/or expressed sequence tags. A comparison of Hsa15 with chicken identified seven conserved chromosomal segments between the two species. In chicken, these were on chromosome 1 (Gga1; two segments), Gga5 (two segments), and Gga10 (three segments). Although four conserved segments were also observed between Hsa15 and mouse, only one of the underlying rearrangement breakpoints was located at the same position as in chicken, indicating that the rearrangements generating the other three breakpoints occurred after the divergence of the rodent and the primate lineages. A high-resolution comparison of Gga10 with Hsa15 identified 19 conserved blocks, indicating the presence of at least 16 intrachromosomal rearrangement breakpoints in the bird lineage after the separation of birds and mammals. These results improve our knowledge of the evolution and dynamics of the vertebrate genomes and will aid in the clarification of the mechanisms that underlie the differentiation between the vertebrate species.
Introduction
By the early 1990s, comparative gene-mapping projects had led to a comparative physical map for 28 species from 8 mammalian orders (O'Brien and Graves 1991 ). Maps containing reasonably large numbers of mapped genes in mice and humans proved to provide an efficient way to identify disease genes in livestock, such as the muscular hypertrophy gene in cattle (Grobet et al. 1997 ; Kambadur et al. 1997 ; McPherron and Lee 1997 ). Much effort has been made by sequencing and mapping genes or expressed sequence tags (ESTs) in farm animals to improve the comparative maps, but so far the resolution is relatively low (O'Brien et al. 1999 ). While in most cases the more dramatic evolutionary rearrangements can be identified, subtle internal rearrangements often remain uncertain or undetected. In comparison, analyses of significantly more detailed human and mouse maps have indicated that many of the syntenically homologous regions can be interrupted by insertions, transpositions, deletions, inversions, and other types of rearrangements (Carver and Stubbs 1997 ).
Comparison of gene and cytogenetic/molecular cytogenetic maps between different extant species can be used to infer the chromosomal constitution of the last common ancestor between these species. For example, chromosomal reconstruction of the ancestral karyotype of primates suggests that 18–20 human chromosomes have remained unchanged during evolution and that the rest have had but a single exchange each (O'Brien and Stanyon 1999 ). Nevertheless, different exchanges have occurred in the lineages leading to distinct primate families and genera. In contrast, based on the cumulative mapping of 223 genes in the chicken genome, the predicted number of conserved autosomal fragments between chickens and humans is 96, and for the chicken-mouse comparison this number is 152 (Burt et al. 1999 ).
Comparative maps between mammals suggest that the number of chromosomal rearrangements in these species can vary from 1 to 10 per million years (O'Brien et al. 1999 ). The observed number of conserved segments in chicken, in comparison with that in human or mouse, therefore indicates an unexpected low number of rearrangements between the two lineages that led to the development of the avian and mammalian species. These results, however, are based on relatively low density chicken-human and chicken-mouse comparative maps. Therefore, gene mapping to a higher resolution would be expected to identify additional chromosome rearrangements over evolutionary time. Although a small improvement in the chicken-human comparative map has been realized through the mapping of genes in chicken by both cytogenetics (Suzuki et al. 1999 ) and linkage analysis within reference pedigrees (Groenen et al. 2000 ), there has been relatively little further progress.
To obtain a high-density comparative map, regional mapping of sufficient numbers of coding sequences in the species of interest is necessary. The recent construction of arrayed genomic libraries of large insert clones such as bacterial artificial chromosomes (BACs) for many species, including chicken (Crooijmans et al. 2000 ), are powerful tools with which to perform comparative and physical mapping. Large insert clones are used as cytogenetic probes and for direct sequencing and therefore are also useful in identifying orthologous genes to known genes in other species. So far, only eight genes that are located on human chromosome 15 have been mapped in the chicken. Seven of these genes map to chicken chromosome 10, whereas the RYR3 gene maps to chromosome 5 (Jones et al. 1997 ; Smith and Cheng 1998 ; Groenen et al. 1999 ; Morton et al. 1999 ; Crooijmans et al. 2000 ). In this study, we describe the generation of the first detailed comparative map between human chromosome Hsa15, its mouse counterparts on chromosomes Mmu7, Mmu2, and Mmu9, and the homologous regions on the three chicken chromosomes Gga10, Gga5, and Gga1 through the identification and mapping of almost 100 genes in the chicken. These results indicate the occurrence of multiple inter- and intrachromosomal rearrangements during the evolution of these chromosomes between the two species.
Materials and Methods
Chicken Chromosome 10 BAC Clones
The BAC library was screened for all microsatellite markers and genes located on chicken chromosome 10 (former linkage group E29C09W09) by two-dimensional PCR (Crooijmans et al. 2000 ). All the BAC clones from each of the markers were identified, and one BAC clone per marker was selected for both BAC-end sequencing and shotgun sequencing. The BAC-end sequences were used to design specific STS markers for chromosome walking.
Additional BACs were isolated using STS markers developed from chicken sequences present in GenBank. The sequences used were selected based on the preliminary chicken-human comparative map (Morton et al. 1999 ; Groenen et al. 2000 ). Toward this end, human sequences from genes and ESTs known to be located on Hsa15 (www.ncbi.nlm.gov; Homo sapiens genome view build 22 [June 18, 2001]) were used to identify sequences from homologous chicken genes present in GenBank.
BAC-End Sequencing
BAC DNA was isolated with the REAL Prep 96 plasmid kit (Qiagen) or as described by Crooijmans et al. (2000) and dissolved in 32 μl 5 mM Tris-HCl (pH 8.0). PCR Sequencing was performed in 40 μl, consisting of 16 μl of BAC DNA, 8 μl Half Big Dye terminator (Genpak Ltd.), 8 μl Big Dye Terminator Rrmix (Perkin-Elmer), and 8 μl of M13 forward or M13 reverse sequence primer (10 pmol/μl). The amplification reactions were as follows: 5 min at 96°C, followed by 45 cycles of 30 s at 96°C, 20 s at 50°C, and 4 min at 60°C. The amplification product was precipitated with isopropanol and finally dissolved in 3μl 83% deionized formamide and 17% loading buffer (Perkin-Elmer). The sequences were analyzed on a 4.75% Long Ranger Gel (FMC) on an automated ABI377 sequencer (Perkin-Elmer). Electrophoresis was performed for 7 h on 36-cm gels, and the results were analyzed using sequence software (ABI, Perkin-Elmer).
Sample Sequencing of BACs
EcoRI-digested BAC DNA was ligated into the EcoRI site of pTZ18R and transformed to DH5α. Twelve subclones per BAC clone were selected, and plasmid DNA was isolated (Qiaprep 96 miniprep kit; Qiagen). The PCR sequence reaction was performed in 10 μl, with 200–500 ng plasmid DNA, 2 μl Half Big Dye terminator (Genpak Ltd.), 2 μl Big Dye Terminator Rrmix (Perkin-Elmer), and 1 μl of M13 forward or M13 reverse sequence primer (0.8 pmol/μl). PCR was performed according to ABI (Perkin-Elmer), and the excess dye terminator was removed by precipitation with isopropanol. Sequence reactions were analyzed on a 96-well 36-cm 4.75% denaturing Long Ranger Gel (FMC) according to ABI (Perkin-Elmer). All sequences obtained were first analyzed with PREGAP4 of the STADEN software package, and vector, Escherichia coli, and low-quality sequences were removed. The resulting sequences were compared with sequences deposited in the public databases using the network BLAST client software of the NCBI (blastcl3). We inferred homology from DNA-level BLAST expectation values (E) less than 1.0 × 10−7. In the case of observed sequence identity to gene families, the human conserved map position enabled the identification of the orthologous gene.
Fluorescent In Situ Hybridization
Two-color fluorescent in situ hybridization (FISH) was performed according to Trask et al. (1991) . EcoRI-digested BAC DNA was labeled by random priming either with biotin-16-dUTP or with digoxigenin-11-dUTP (Boehringer Mannheim) (Ruyter-Spira et al. 1998 ). BAC clones were isolated from markers known to be located on specific chromosomes (Groenen et al. 2000 ). The BAC clones used as FISH markers to identify the specific chromosome were BAC bw016D10 from marker ADL0038 and BAC bw008K20 from marker MCW0228 for GGA10, BAC bw009B13 from marker ADL0298 and BAC bw037H20 from marker MCW0263 for GGA5, and BAC bw038E08 from marker MCW0107 and BAC bw030P07 from marker MCW0248 for GGA1.
Results and Discussion
Comparative mapping is a powerful tool for utilization of the existing knowledge of species with detailed mapping information, such as humans and mice, in species with less well developed maps. We used this approach for a detailed characterization of chicken chromosome 10 (Gga10) using a bidirectional approach, building the comparative map from Gga10 as well as from the previously identified homologous region in humans (Jones et al. 1997 ). In the chicken, 29 loci have previously been mapped to Gga10, consisting of 20 microsatellite markers, 8 genes, and 1 EST (Groenen et al. 2000 ). In the first step, these markers were used in the two-dimensional PCR screening of our chicken BAC library (Crooijmans et al. 2000 ). The BAC clones isolated were subjected to both end sequencing and sample sequencing. End sequencing enabled the development of sequence tag site (STS) markers for chromosome walking, whereas sample sequencing provided information on the gene content of some of the BACs. Of the eight genes previously mapped to Gga10, seven have a homolog in humans that has been mapped to chromosome 15 (Hsa15) (Groenen et al. 2000 ). The other gene (GNRHR) is located on human chromosome 4 (Groenen et al. 2000 ). Furthermore, another gene known to be located on Hsa15 (RYR3) has been mapped to chicken chromosome 5 (Smith and Cheng 1998 ; Morton et al. 1999 ). Therefore, as a second step in our approach, genes known to be located on Hsa15 (www.ncbi.nlm.gov, Homo sapiens genome view build 22) were selected and used to identify homologous chicken genes whose sequences were present in the public nucleotide databases. Sequences of 36 chicken genes were selected from the database, and primers were designed to screen the chicken BAC library. For 33 genes (table 1 ), we were able to isolate at least one BAC clone; if not already mapped to a Gga10-contig, these clones were subsequently used in two-color FISH to map the chromosome locations of the genes in the chicken (indicated in bold in fig. 1 ). The precise map locations of the genes mapped to chromosome 10 by FISH are not known because of the small size of this microchromosome and the absence of a clear banding pattern. However, based on the map locations in humans and mice and based on the known map locations of other genes mapped on Gga10, we can predict the map locations for some of these genes on this chicken chromosome. For example, the CKMT1 gene is mapped by FISH to Gga10 and has a Hsa15 map location of 38.3–28.3 Mb. A sequence derived from a BAC of marker ADL0112 identified the P53BP1 gene that is mapped in humans to almost the same position as CKMT1. Therefore, we expect the CKMT1 gene to be located in the chicken close to marker ADL0112, although linkage needs to be confirmed.
The BAC clones that were mapped to Gga10 by FISH were also used as starting points for chromosome walking. The approach outlined above has thus far resulted in the development of more than 385 STS markers and the isolation of more than 750 different BAC clones, corresponding with chromosome coverage of more than 65%–70%. The number of BAC clones per marker varied from 1 to 12, with an average of 4.9 BACs per marker, which is in good agreement with the estimated genome coverage of the library of 5.5× (Crooijmans et al. 2000 ).
Seventy different BAC clones derived from Gga10 were selected and used for sample sequencing. All sequences obtained by BAC-end sequencing and sample sequencing were compared with sequences in the nucleotide database (BLAST). In most cases, the observed homology (E values >10−7) to human genes, in combination with the human map location, enabled the unambiguous identification of the orthologous gene. Often, several different exons of the same gene were identified. For example, six subclones from BAC bw046J07 (MCW0194) identified seven different exons of the gene MLSN1. Six different exons of the gene IQGAP1 were identified with six subclones from BAC bw098O14 (ADL0112). More difficulties occur when homology is detected with several genes belonging to a gene family. This occurred after shotgun sequencing of a BAC clone derived from marker ABR0012 mapped to Gga10. Gene identity was found with transducin-like enhancer protein family; TLE1 (83% identity), TLE2 (77%), TLE3 (88%), and TLE4 (83%). TLE1 and TLE4 are located on Hsa9, TLE2 is located on Hsa19, and TLE3 is located on Hsa15 (15q22; 67.7–67.7 Mb). According to the gene identity and human chromosome location of the TLE gene family, we assume TLE3 is located on Gga10.
Blocks with similar gene orders in chickens and humans were identified in several contigs, such as the contig of the mapped marker ADL0038. The gene/EST order, identified with the sequences of chicken BAC clones from this block, was FLJ20086 (53.9–53.9), TCF12 (54.2–54.6), and ALDH1A2 (55.2–55.3), with the sequence notation in megabases in parentheses. A similar gene order in chickens and humans was also identified with sequence homology within one BAC clone. For example, with BAC clone bw094I14, within the chicken contig of MCW0035, the two genes BTBD1 and HOMER-2B were identified, which are both mapped in humans to Hsa15 (81.6–81.7 and 81.4–81.4 Mb).
The sequence results, together with the genes mapped by FISH, revealed sequence identity to almost 120 human, mouse, rat, and chicken genes and ESTs. The homologs of several chicken genes and ESTs, such as the epsilon adaptin gene (ADTE), have not been mapped in humans yet. The chicken ADTE gene, found after sample sequencing of a BAC clone of marker MCW0357 (CYP19), belongs to the adaptin family. Of this family, beta 1, delta, and gamma are mapped to human chromosomes 22, 19, and 16, respectively. Since human CYP19 maps to Hsa15 (fig. 1 ), the human ADTE gene is predicted to be on Hsa15. Besides homology found with genes predominantly mapped to Hsa15, sequence homology was occasionally observed with human genes that mapped to other human chromosomes (fig. 1 ). For example, the HK1 gene maps to Hsa10, the GNRHR gene maps to Hsa4, and the ENC1 gene maps to Hsa5. These observed homologies either may indicate the presence of gene families of which one member has not yet been mapped to Hsa15 or might indicate the presence of small regions of homology to other human chromosomes. However, in the latter case, one would expect that more genes from those regions would have been identified in the current study. The former hypothesis is further strengthened by the fact that for the chicken genes that have related human genes located on different human chromosomes, on the same chicken BAC clone a gene has been identified whose ortholog does map to Hsa15 (i.e., the HK1 and RAB11A genes on BAC bw017J21, and the GNRHR and PUNC genes on BAC bw091J11, respectively).
The human chromosome 15-chicken comparative map consists of 91 genes and ESTs and is shown in figure 1 . The genes that are located in humans on chromosome 15 are located in chickens in seven regions of conserved synteny on three different chromosomes, Gga1, Gga5, and Gga10. The majority of these genes, however, are mapped in chicken to chromosome 10. In mice, four conserved chromosome segments are also observed, in the order Mmu7, Mmu2, Mmu9, and Mmu7. In an attempt to reconstruct a common ancestor of humans, mice, and chickens, three time nodules in evolution have been described (Burt et al. 1999 ). The first time nodule is 300 MYA, when birds diverged from mammals; the second is 100 MYA, when the mouse diverged; and the third is 65 MYA, when the common ancestor of the primates lived. The reconstructed ancestor of all primates has a chromosome 6 consisting of a rather unchanged human chromosome 15 and chromosome 14 (O'Brien and Stanyon 1999 ). This reconstructed chromosome is based on the comparison of chromosome paints of primates in which the gene order of the conserved segments was not considered. The comparison of Hsa15 with chicken and of Hsa15 with mouse chromosomes indicates the occurrence of six and three interchromosomal rearrangements (interCRs) during evolution between each pair of species (fig. 2 ). Only one of these rearrangements appears to be at the same location in the chicken and the mouse; if so, this would indicate that this translocation occurred in the lineage leading to humans after the mouse and human lineages diverged, between 100 and 65 MYA. The other five interchromosomal rearrangements involving segments on Gga1-Gga10 (twice) and Gga5-Gga10 (three times) probably occurred before the human and mouse lineages diverged, either in a predecessor of mammals or in the chicken lineage. In the mouse, the other two interCRs between the segments on Mmu2-Mmu9 and Mmu9-Mmu7 probably occurred within the mouse lineage during evolution starting 100 MYA.
A more detailed comparison of the conserved chromosome segments between chicken chromosome 10 and human chromosome 15 reveals a much more scattered picture (fig. 2 ), as predicted by Burt et al. (1999) . In total, 78 homologous genes and ESTs have been mapped to both chromosomes of these two species. The estimated size and order of the conserved gene blocks in both species as shown in figure 2 is based on a combination of genetic mapping, chromosome walking results, FISH results, and sequencing. The exact gene order is known only when a complete BAC contig and/or sequence is available for both species. We can identify at least 19 blocks, which is the minimum number of conserved segments between Gga10 and Hsa15. The number of genes per block varies from 1 (blocks 2 and 10) to 7 (block 3). An example of this scattered picture is clearly demonstrated from the sequencing results of a chicken BAC clone identified with marker MCW0228. Gene identity with sequences of this BAC clone was found with the family of the S-cyclophilin-like genes, of which the cyclophillin B (PPIB) gene, located on human chromosome 15 (62.3–62.3 Mb), shows the highest homology (data not shown). In addition to this gene, significant gene identity was found with sequences from the same BAC clone for five other human genes (B2M, RPS17, SNX1, CPEB1, and AP3B2), one mouse gene (Ckgl), and one rat gene (Casein kinase 1 gamma 1 like gene). The map location of the four human genes is scattered over Hsa15 (39.5–83.2 Mb). An example of the genes detected in the contig of microsatellite marker MCW0194 is given in figure 3 . In total, 101 BAC clones are identified by chromosome walking, representing approximately 2.5 Mb of chicken Gga10 sequence. The number of genes/ESTs detected within this contig is 11, where 9 have an ortholog mapped to Hsa15. The gene order from DUT to GALK2 is similar in chickens and humans, while between GALK2 and USP8 in humans the gene CYP19 (46.6–46.7 Mb) is mapped. In chickens, however, CYP19 is not mapped within the MCW0194 contig, but is mapped within another contig on Gga10. The MLSN1 gene within the MCW0194 contig is mapped in humans at a position between 22.6–22.7 Mb, which is more than 20 Mb apart from the genes from DUT to GALK2.
By comparing the chromosome block order of GGA10 (fig. 2 , segment order in chicken in brackets) with human chromosome Hsa15, at least 16 intrachromosomal rearrangements can be identified that have taken place during evolution. Identification of more genes on Gga10 will subsequently indicate more rearrangements. Assuming that the most likely intrachromosomal recombination events are inversions, the gene order belonging to the common ancestor of humans and birds can only be designed when the gene orders are known in both species. Therefore, a complete chicken BAC contig, or, more precisely, the complete chicken sequence, is inevitable. Also taking into account the gene mapping information of zebrafish (Danio rerio; Dre) (Barbazuk et al. 2000 ) for evolution studies, several genes homologous to genes mapped to Hsa15 have been identified. These genes are mapped in zebrafish to linkage groups 2, 4, 7, 18, and 25, and syntenic blocks (based on at most two mapped genes, such as is in case of linkage group 18 with the genes CYP19 and MEF2A) can be identified between all four organisms. Also, for zebrafish, more information is needed for the construction of the ancestral chromosomes.
Based on the rates of chromosomal change observed in mammals (Andersson et al. 1996 ; O'Brien, Weinberg, and Lyons 1997 ), one can calculate that the number of conserved segments between chickens and humans would be on the order of 100–600. Based on the comparative mapping data of 223 genes, Burt et al. (1999) suggested that the number of conserved segments between chickens and humans would be in the lower part of this range. However, our data on the detailed comparison between human chromosome 15 and that of the chicken indicates that this was probably an underestimate and that the number of conserved segments is at least 600 (19 conserved segments between Gga10 and Hsa15, with a size of Gga10 of approximately 3%–4% of the chicken genome).
In this paper, we clearly demonstrate the importance of high gene densities in comparative mapping in identification of both inter- and intrachromosomal rearrangements. The development of complete physical maps either as BAC contigs or as the complete sequence will further aid in the detailed reconstruction of rearrangements during evolution which resulted in the chromosomes in the different species as we know them today. A detailed comparative map as described in this paper will be of much value in the identification and further characterization of candidate genes in quantitative trait loci studies in the chicken as well as in the analysis of complex traits in humans and other vertebrate species.
Supplementary Material
All data are stored in our database Chickace, an object-based database that is based on the AceDB database software, and will be made available through our web site at http://www.zod.wau.nl/vf.
Mark Ragan, Reviewing Editor
Present address: Center for Neurobiology and Behavior, Department of Psychiatry, University of Pennsylvania.
Keywords: genes chicken human rearrangements FISH BACs
Address for correspondence and reprints: Richard P. M. A. Crooijmans, Wageningen Institute of Animal Sciences, Animal Breeding and Genetics Group, Wageningen Agricultural University, Postbox 338, 6700 AH Wageningen, The Netherlands. [email protected] .
Table 1 PCR Primers of 33 Chicken Genes Orthologous to HSA15
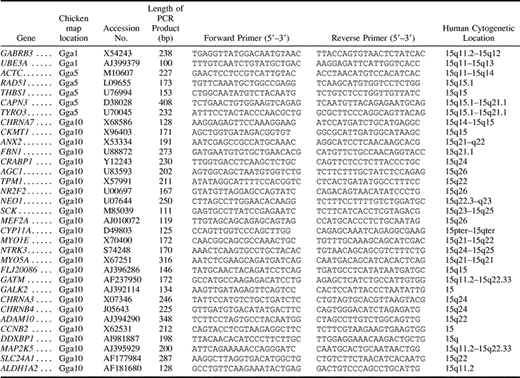
Table 1 PCR Primers of 33 Chicken Genes Orthologous to HSA15
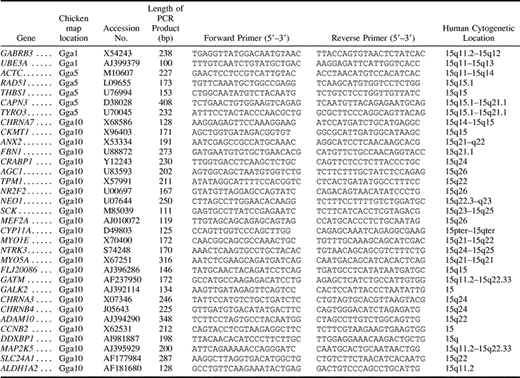
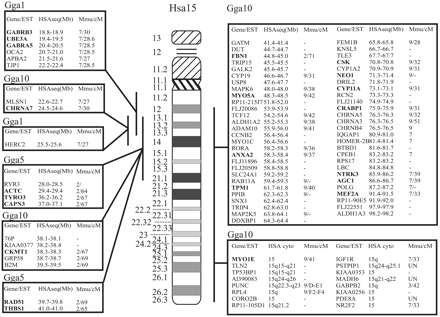
Fig. 1.—Homologs of genes and expressed sequence tags (ESTs) mapped to Homo sapiens chromosome 15 (Hsa15) have been mapped in the chicken (Gallus gallus; Gga) to three different chromosomes. The homologous chicken genes are ordered by the human gene sequence positions. When known, the chromosome location in the mouse is given as well. The genes indicated in bold have been mapped in the chicken by two-color fluorescent in situ hybridization (FISH). The others have been mapped by genetic linkage or have been identified by sequence analysis of specific chicken BAC clones. * The OCA2 gene was mapped by FISH by M. A. Ohta, R. P. M. A. Crooijmans, T. A. Gray, R. J. Smyth, J. J. Bitgood, M. A. M. Groenen, and R. D. Nicholls (unpublished data)
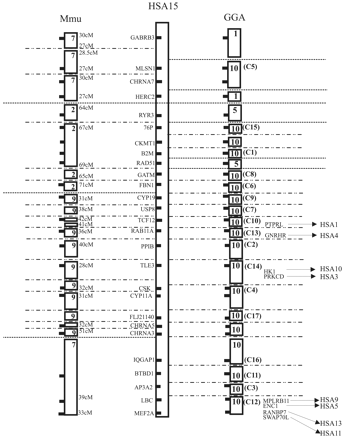
Fig. 2.—Comparative map of human chromosome 15 (Homo sapiens; Hsa) to chicken (Gallus gallus; Gga) and mouse (Mus musculus; Mmu). For every chromosome block, at least one gene is indicated. The chromosome block order for Gga10, if known, is given in brackets. Positions of interchromosomal rearrangement (interCR; indicated with dotted lines) and intrachromosomal rearrangement (intraCR; indicated with long dash-dot-dot lines) breakpoints are indicated. Map positions in the mouse are given in cM according to the mouse genome informatics of the Jackson Laboratory (http://www.informatics.jax.org)
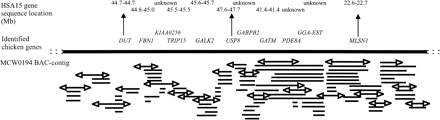
Fig. 3.—The BAC contig around microsatellite marker MCW0194, including the identified chicken genes. This contig spans around 2.5 Mb of chicken sequence. Gene locations in humans are given in Mb according to the Homo sapiens genome viewer BUILD 22 (www.ncbi.nlm.gov). BAC clones are not on scale; arrows at the end of a BAC clone indicate the development of an STS marker that was used for chromosome walking
We thank Dr. N. Bumstead for providing the cDNA sequences of markers COM0101 and RPL1B. This work was supported by a grant from the Dutch ministry of economic affairs (project number BTS97089).
References
Andersson L., A. Archibald, M. Ashburner, et al. (55 co-authors)
Barbazuk B. W., I. Korf, C. Kadavi, J. Heyen, S. Tate, E. Wun, J. A. Bedell, J. D. McPherson, S. L. Johnson,
Burt D. W., C. Bruley, I. C. Dunn, et al. (13 co-authors)
Carver E. A., A. L. Stubbs,
Crooijmans R. P. M. A., J. Vrebalov, R. J. M. Dijkhof, J. J. van der Poel, M. A. M. Groenen,
Grobet L., L. J. Martin, D. Poncelet, et al. (13 co-authors)
Groenen M. A. M., R. P. M. A. Crooijmans, R. J. M. Dijkhof, R. Acar, J. J. van der Poel,
Groenen M. A. M., H. H. Cheng, N. Bumstead, et al. (15 co-authors)
Jones C. T., D. R. Morrice, I. R. Paton, D. W. Burt,
Kambadur R., M. Sharma, T. P. Smith, J. J. Bass,
McPherron A. C., S. J. Lee,
Morton C. C., S. L. Christian, T. A. Donlon, et al. (27 co-authors)
O'Brien S. J., J. A. M. Graves,
O'Brien S. J., M. Menotti-Raymond, W. J. Murphy, W. G. Nash, J. Wienberg, R. Stanyon, N. G. Copeland, N. A. Jenkins, J. E. Womack, J. A. M. Graves,
O'Brien S. J., J. Wienberg, L. A. Lyons,
Ruyter-Spira C. P., A. J. C. de Groot, J. J. van der Poel, J. Herbergs, J. Masabanda, R. Fries, M. A. M. Groenen,
Smith E. J., H. H. Cheng,
Suzuki T., T. Kurosali, K. Shimada, et al. (15 co-authors)