-
PDF
- Split View
-
Views
-
Cite
Cite
Dan Hao, Ling Guo, Qian Wang, Misa Ito, Bin Huang, Chieko Mineo, Philip W Shaul, Xiang-An Li, Relative Adrenal Insufficiency Is a Risk Factor for Pediatric Sepsis: A Proof-of-Concept Study, The Journal of Infectious Diseases, Volume 229, Issue 4, 15 April 2024, Pages 1166–1177, https://doi.org/10.1093/infdis/jiad369
- Share Icon Share
Abstract
Glucocorticoid (GC) therapy had been strongly recommended for pediatric sepsis (grade 1A). However, the recommendation was changed to grade 2C in 2020 due to weak evidence. About 32.8% of patients with pediatric septic develop relative adrenal insufficiency (RAI). But whether GC therapy should be determined by RAI status is controversial. This study utilized 21-day-old SF1CreSRBIfl/fl mice as the first pediatric RAI mouse model to assess the pathogenesis of RAI and evaluate GC therapy. RAI mice exhibited a substantially higher mortality rate in cecal ligation and puncture and cecal slurry–induced sepsis. These mice featured persistent inflammatory responses and were effectively rescued by GC therapy. RNA sequencing analysis revealed persistent inflammatory responses in RAI mice, caused by transcriptional dysregulation of AP-1 and NF-κB, and cytokine-induced secondary inflammatory response. Our findings support a precision medicine approach to guide GC therapy for pediatric patients based on the status of RAI.
Sepsis is caused by a dysregulated host response to infection [1]. It represents a major cause of death in children, with mortality ranging from 4% to as high as 50% [2]. Glucocorticoid (GC) had been considered an effective therapy and strongly recommended for pediatric sepsis (grade 1A) [3]. However, in 2020 the recommendation was changed to grade 2C (weak recommendation with weak evidence) [4]. This change was prompted by the lack of substantial evidence supporting or refuting the beneficial effect of GC therapy in pediatric patients with sepsis. Therefore, it is crucial to determine whether GC therapy is beneficial in pediatric sepsis.
GC is present in circulation at 20 to 200 ng/mL at physiologic conditions [5], and it plays a crucial role in maintaining normal physiologic activities. A lack of GC can be lethal without treatment with physiologic levels of GC, as shown in patients with Addison disease [6] and mice undergoing adrenalectomy [7]. A striking feature of GC biology is its inducible nature. In response to septic stress, GC production is upregulated 5- to 10-fold, a phenomenon known as the adrenal stress response [8]. The function of inducible GC (iGC) is less characterized due to technical difficulties in identifying iGC deficiency in critically ill patients and a lack of an iGC deficiency animal model. The concept of relative adrenal insufficiency (RAI) was introduced in the 2004 guidelines of the Surviving Sepsis Campaign, as supported by a French trial [9]. RAI is characterized by impaired iGC production in response to stress and is diagnosed by a Δ total cortisol <9 µg/dL after adrenocorticotropic hormone (ACTH) stimulation [10]. In 2008, the term RAI was replaced by critical illness–related corticosteroid insufficiency (CIRCI) by a task force of experts in critical care medicine [11]: “The task force coined the term CIRCI to describe the dysfunction of the hypothalamic-pituitary-adrenal axis that occurs during critical illness.” CIRCI includes absolute adrenal insufficiency and RAI, as well as GC resistance. The task force also acknowledged potential technical limitations of using an ACTH test for diagnosing RAI, which is paradoxically used for diagnosing CIRCI. In this study we use RAI, for 3 reasons. First, the flaw associated with an ACTH test is a technical issue that applies equally to RAI and CIRCI. Therefore, it should not be a reason to abandon RAI. Second, we believe that adrenal stress is a host response and that impaired iGC production in response to stress is RAI. Finally, as outlined by the task force, “the mechanisms leading to dysfunction of the HPA axis during critical illness are complex and poorly understood, they likely include decreased production of ACTH and cortisol, as well as dysfunction of their receptors” [11]. A lack of ACTH can lead to an impaired adrenal stress response (iGC), resulting in RAI. In other words, RAI is likely to present in critically ill patients. Thus, rather than abandoning RAI, further efforts should be made to explore its contribution to sepsis and develop a new diagnosis for RAI.
About 32.8% of pediatric patients with sepsis experience RAI [12]. Unlike the large clinical studies conducted in adult sepsis [9, 13], only a few small observational studies (range, 13–72 patients) have examined adrenal insufficiency in pediatric critical illness [14–18]. Nevertheless, the debate is going on. A lack of understanding about the pathogenesis of RAI presents a significant barrier to resolving this critical issue. To address this gap in knowledge, it is crucial to evaluate the efficacy of GC therapy, ideally in a RAI animal model, to understand the pathophysiology of RAI in pediatric sepsis. Such a study could provide a proof of concept to determine if GC therapy is beneficial or harmful.
Scavenger receptor class B type I (SR-BI) is an HDL receptor (high-density lipoprotein) that is most abundantly expressed in the liver and steroidogenic tissues. It mediates the uptake of cholesterol from HDL for GC synthesis in the adrenal gland [19]. Early studies, including ours, have identified the protective role of SR-BI in sepsis [20, 21]: SR-BI−/− mice displayed impaired iGC production and significantly increased mortality during sepsis [22–24]. Later, we established the adrenal SR-BI–specific knockout mice (SF1CreSRBIfl/fl) as a model of RAI and showed that adult mice with RAI are more susceptible to sepsis induced by cecal ligation and puncture (CLP) [25]. Given the urgent need to determine the effectiveness of GC therapy in pediatric sepsis and the profound differences in host response between adults and children, in this study we used 21-day-old SF1CreSRBIfl/fl mice as the first RAI pediatric model to elucidate the pathogenesis of RAI and evaluate the efficacy of GC therapy. Mice at this age have been weaned and are capable of feeding and drinking independently. They are roughly equivalent to 6 months of human age [26]. We demonstrated that endogenous iGC is a critical protective modulator of pediatric sepsis in mice by controlling the inflammatory response. RAI is a risk factor in pediatric sepsis due to the lack of iGC, and GC therapy effectively rescues mice with RAI. Our study provides a proof of concept supporting the use of a precision medicine approach for sepsis therapy, by selectively applying GC therapy for a subgroup of pediatric patients with RAI.
MATERIALS AND METHODS
Twenty-one-day-old SRBIfl/fl and SF1CreSRBIfl/fl littermates were used in this study. Animal care and experiments were approved by the Institutional Animal Care and Use Committee of the University of Kentucky. See supplementary materials for detailed materials and methods.
RESULTS
Mice With RAI Are Susceptible to Septic Death and Organ Injury
SR-BI is highly expressed in the adrenal gland and provides an essential role in iGC production [19]. We first measured SR-BI expression in the adrenal gland and liver. SRBIfl/fl mice exhibited clear detectable SR-BI expression in the liver and adrenal gland. SF1CreSRBIfl/fl mice showed complete knockout of SR-BI in the adrenal gland while maintaining normal SR-BI expression in the liver (Figure 1A). To test if the SF1CreSRBIfl/fl mouse serves as a model of RAI, an ACTH stimulation test was performed. After 1 hour of ACTH administration, SRBIfl/fl mice featured a significant increase in corticosterone levels. On the contrary, SF1CreSRBIfl/fl mice failed to generate inducible corticosterone in response to ACTH stimulation (Figure 1B), suggesting that it is a useful RAI model. We further performed survival analysis in SF1CreSRBIfl/fl mice to determine their susceptibility to CLP- and cecal slurry–induced septic death (Figure 1C and 1D). Additionally, serum corticosterone levels significantly increased in SRBIfl/fl mice at 4 and 18 hours post-CLP. In contrast, SF1creSRBIfl/fl mice displayed impaired production of inducible corticosterone in response to the CLP challenge (Figure 1E). Hypoglycemia (blood glucose < 70 mg/dL) is associated with increased septic death [27]. SF1CreSRBIfl/fl mice also exhibited significantly lower glucose levels than the control group (Figure 1F). There are no statistical difference in the bacterial load between SRBIfl/fl and SF1CreSRBIfl/fl mice (Supplementary Figure 1).
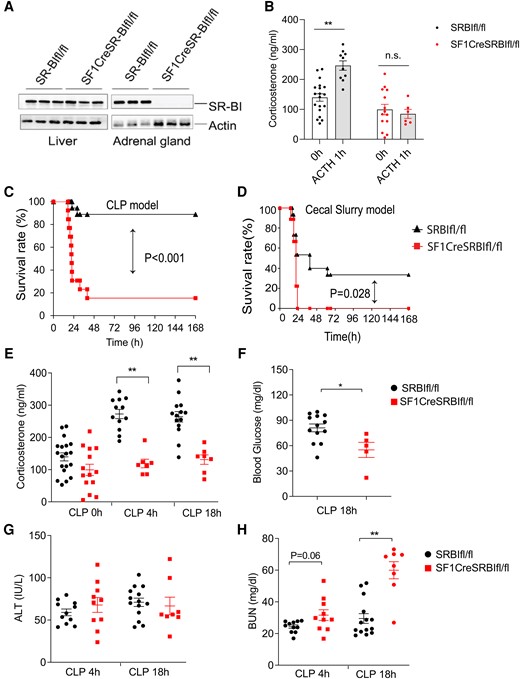
Mice with relative adrenal insufficiency are susceptible to septic death and organ injury. A, Western blot analysis of scavenger receptor class B type I expression in liver and adrenal gland of 21-day-old SRBIfl/fl and SF1CreSRBIfl/fl mice (n = 3). B, Serum corticosterone levels in 21-day-old SRBIfl/fl and SF1CreSRBIfl/fl mice prior and 1 hour post–ACTH stimulation (n = 6–19). C and D, Survival analysis was conducted in 21-day-old SRBIfl/fl and SF1CreSRBIfl/fl mice with CLP (n = 13–18) and cecal slurry–induced sepsis (n = 9–15). Data were analyzed by log-rank test. E, Plasma corticosteroid concentration at 0, 4, and 18 hours post-CLP (n = 7–19). F, Blood glucose concentration at CLP 18 hours (n = 5–13). G and H, ALT and BUN concentration at 4 and 18 hours post-CLP, respectively (n = 8–14). Data are shown as mean ± SEM and were analyzed by Student t test. *P < .05. **P < .01. ALT, alanine aminotransferase; BUN, blood urea nitrogen; CLP, cecal ligation and puncture.
To investigate whether the high septic death rate of SF1CreSRBIfl/fl mice is associated with organ injury, we evaluated liver and kidney injuries at 4 and 18 hours post-CLP by measuring serum levels of alanine aminotransferase and blood urea nitrogen. SF1creSRBIfl/fl mice displayed >2-times higher blood urea nitrogen levels than SRBIfl/fl mice at 18 hours post-CLP (Figure 1H). There was no significant difference in alanine aminotransferase levels between the genotypes of mice (Figure 1G). The bacterial load in blood, liver, spleen, and peritoneal fluid at 4 and 18 hours post-CLP also did not show a statistical difference (Supplementary Figure 1). In summary, the SF1CreSRBIfl/fl mouse is a model of RAI that lacks iGC production in response to septic stress, and mice with RAI have severe kidney injury and increased septic death.
Mice With RAI Feature Persistent Hyperinflammatory Responses in Sepsis
A dysregulated inflammatory response is responsible for the increased severity and mortality of sepsis [28]. We measured 31 plasma cytokines/chemokines in 21-day-old of SRBIfl/fl and SF1CreSRBIfl/fl mice at 0, 4 and 18 hours post-CLP. At baseline, there was no difference in cytokine concentration between the genotypes of mice. Four hours post-CLP, the cytokine levels were dramatically increased in SRBIfl/fl and SF1CreSRBIfl/fl mice. Eighteen hours post-CLP, most cytokines in SRBIfl/fl mice regressed to baseline levels. However, SF1creSRBIfl/fl mice still had markedly high levels of cytokines. Of the 31 cytokines measured, 22 cytokines—such as interleukin 6 (IL-6), tumor necrosis factor-alpha (TNF-α), interferon-gamma (IFN-γ), and interleukin-1 beta (IL-1β)—were significantly higher in SF1creSRBIfl/fl mice than SRBIfl/fl mice at 18 hours post-CLP (Figure 2). These results suggest that the production of iGC is required for controlling inflammatory cytokine production during sepsis.

Mice with relative adrenal insufficiency feature persistent inflammatory responses in sepsis. Serum cytokines in 21-day-old SRBIfl/fl and SF1CreSRBIfl/fl mice were measured by Mouse Cytokine Array/Chemokine Array 31-Plex (MD31) at 0, 4 and 18 hours after cecal ligation and puncture. Data are shown as mean ± SEM. *P < .05 and **P < .01 vs SRBIfl/fl mice by Student t test (n = 7–14).
Mice With RAI Feature Dysregulated Inflammatory Signaling
The unbiased transcriptomic studies can reveal endotypes of disease based on coordinated gene expression patterns. We conducted RNA sequencing (RNA-seq) analysis 6 hours post-CLP to identify genome-wide expression profiling. As shown in Figure 3A, 658 and 435 distinctive genes were detected in SRBIfl/fl and SF1CreSRBIfl/fl mice, respectively. When compared with SRBIfl/fl mice, 841 upregulated and 829 downregulated differentially expressed genes (DEGs) were identified in SF1CreSRBIfl/fl mice (Figure 3B). In line with the increases in plasma cytokines (Figure 2), the expression of many cytokine genes was significantly upregulated in SF1CreSRBIfl/fl mice (Figure 3C). We next conducted enrichment analysis using ingenuity pathway analysis (IPA). IPA predicted sepsis as a major disease in SF1CreSRBIfl/fl mice (Figure 3D), as mainly promoted by inflammatory-related genes (Figure 3D and 3E), including biomarker cytokine genes associated with increased risk of septic death, such as IL-6, TNF-α, and IL-1β [29].
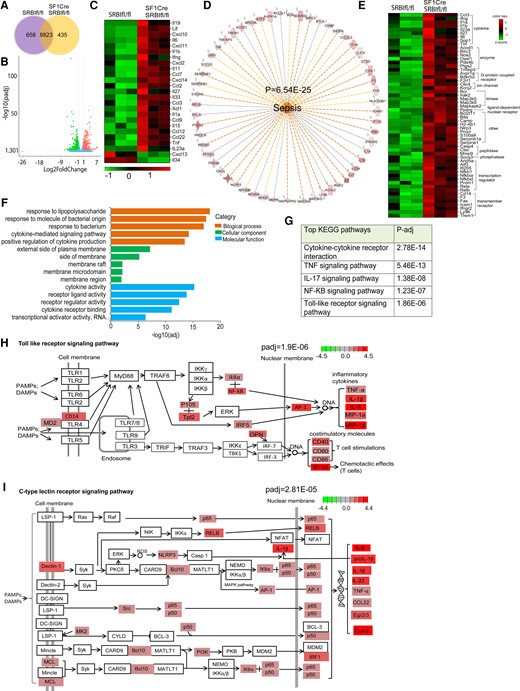
Mice with relative adrenal insufficiency feature dysregulated inflammatory signaling. Liver RNA-seq analysis was performed in 21-day-old SRBIfl/fl and SF1CreSRBIfl/fl mice at 6 hours post-CLP (25G, half; n = 3). A, The distribution of overlapping genes and unique DEGs. B, Significantly altered genes (n = 1670) detected from the RNA-seq data set. DEGs are red and green, identifying 841 upregulated and 829 downregulated genes, respectively. C, Differential cytokine gene expression in SF1CreSRBIfl/fl over SRBIfl/fl mice (adjusted P < .05 and |log2 fold change| > 1). D, Biological functional analysis of ingenuity pathway analysis predicts activation of sepsis. E, Gene lists represent the DEGs that contribute to increased severity of sepsis. Top enriched pathways for upregulated DEGs: F, Gene Ontology; G, KEGG. Modified signaling pathways from KEGG: H, Toll-like receptor; I, C-type lectin receptor. DEG, differentially expressed gene; KEGG, Kyoto Encyclopedia of Genes and Genomes; RNA-seq, RNA sequencing.
During sepsis, activation of immune responses relies on the expression of pattern recognition receptors, which detect distinct conserved microbial structures, called pathogen-associated molecular patterns. Using Gene Ontology enrichment analysis, we found that the top enriched signaling pathways were related to infection responses, such as “response to lipopolysaccharide,” “response to molecule of bacterial origin,” “response to bacterium,” and “cytokine-related signaling pathways” (Figure 3F). We then performed pathway analysis per the Kyoto Encyclopedia of Genes and Genomes. This analysis identified the enriched pattern recognition receptor signaling pathways in mice with RAI, such as the activated Toll-like receptor (TLR) and C-type lectin receptor signaling pathways (Figure 3H and 3I). In addition, “cytokine-cytokine receptor interaction,” “TNF singling pathway,” “IL-17 signaling pathway,” “NF-κB signaling pathway,” and “TLRs signaling pathway” were ranked among the top significantly influenced pathways (Figure 3G). These results indicate the hyperinflammatory response in SF1CreSRBIfl/fl mice that contributes to the development of sepsis. In summary, the RNA-seq analysis revealed that mice with RAI feature persistent hyperinflammatory responses associated with severe sepsis.
Mice With RAI Have a Dysregulated GR Signaling Pathway in Which NF-κB and AP-1 Serve as the Major Upstream Transcriptional Regulators
To understand the mechanisms of why mice with RAI exhibit a hyperinflammatory response, we investigated GC/Glucocorticoid receptor (GR) signaling pathway changes. Figure 4A shows DEGs in the GR signaling pathway, including cytokines and transcriptional regulators (Figure 4B and 4C). To identify factors driving the DEGs in RAI mice, we performed upstream regulator analysis using IPA, which predicts potential upstream regulators. Of interest, this analysis identified that Nuclear factor kappa B (NF-κB) and Activator Protein-1 (AP-1) served as major upstream transcriptional regulators to regulate gene expression (Figure 4D). For example, activation of RELA/NF-κB and FOS/AP-1 increased transcriptional expression of many genes (Supplementary Figures 2 and 3), such as inflammatory cytokines (IL-6, IL-1β, TNF-α), chemokines (CCL2, CXCL2, CXL3), and other transcriptional factors in the AP-1 and NF-κB families (FOS, JUN, NFKB1, RELB) that contribute to the hyperinflammatory response. We also investigated the gene expression of other subunits in the NF-κB and AP-1 families, all of which were significantly upregulated in mice with RAI (Figure 4E). In addition, upregulation of other genes in the GR signaling pathway, such as transmembrane receptors (eg, VCAM-1, ICAM-1), enzymes (eg, Nos2), and kinase (eg, JAK-2), promoted the hyperinflammatory responses in RAI mice. In particular, tsc22d3 (alias GILZ) is an important GR-responsive gene that mediated the GC anti-inflammatory effect, and its expression was significantly downregulated in mice with RAI, which may contribute to the downregulated anti-inflammatory potency in RAI mice. In conclusion, the RNA-seq analysis revealed that dysregulation of the GR signaling pathway contributes to the hyperinflammatory response in RAI mice, in which NF-κB and AP-1 serve as the major upstream transcriptional regulators.
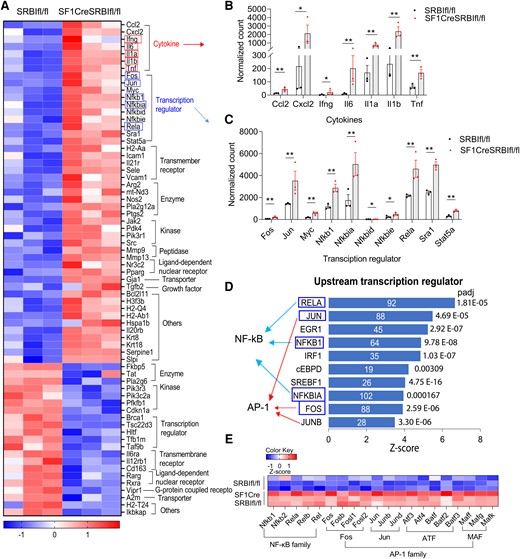
Mice with relative adrenal insufficiency have a dysregulated GR signaling pathway in which NF-κB and AP-1 serve as the major upstream transcriptional regulators. Liver RNA sequencing analysis was performed in 21-day-old SRBIfl/fl and SF1creSRBIfl/fl mice at 6 hours after cecal ligation and puncture. A, DEGs in GR signaling pathway. B and C, Normalized count of DEGs of cytokines and transcriptional regulators in the GR signaling pathway. Data are shown as mean ± SEM.*P < .05. **P < .01. D, Upstream regulator analysis of ingenuity pathway analysis identifies upstream transcriptional regulators in SF1CreSRBIfl/fl mice. Blue rectangle highlights DEGs in GR signaling pathway. E, DEGs in subunits of NF-κB and AP-1 families. DEG, differentially expressed gene.
Inflammatory Cytokines Induce Secondary Inflammatory Responses in Mice With RAI
There were marked increases in inflammatory cytokines in circulation (Figure 2), which may further augment the inflammatory response [30]. Indeed, the Kyoto Encyclopedia of Genes and Genomes pathway analysis showed the upregulation of cytokine-cytokine interaction as the top enriched signaling pathway (Figure 3G). Additionally, the IPA revealed that TNF, IFN-γ, IL-1β, IL-1α, IL-6, and other cytokines acted as upstream regulators to mediate expression of many inflammatory genes (Figure 5A). The IPA revealed that TNF-α, IL-6, or IL-1α binds to its receptor, which upregulates an array of genes involved in various biological processes and functions, such as leukocyte recruitment (eg, CCL2, CXCL1, CXCL2), intracellular signaling (SOCS3, TRAF1, NFKBIA), transcriptional regulation (eg, FOS, JUN, JUNB), and other cytokine genes (IL-1β, IL-6, LIF). Notably, they are primarily controlled by transcriptional regulation of AP-1, NF-κB, and CREB (Figure 5B–D). The IPA also showed that upstream cytokine regulators interact with representative inflammatory signaling pathways (eg, iNOS, HMGB1) and immune cell responses (eg, stimulation of lymphocytes and leukocytes, adhesion of mononuclear leukocytes), which contribute to the hyperinflammatory response in mice with RAI (Figure 5E). To the best of our knowledge, this is the first evidence showing that cytokines induce secondary inflammatory signaling that contributes to the hyperinflammatory response in pediatric sepsis animals.
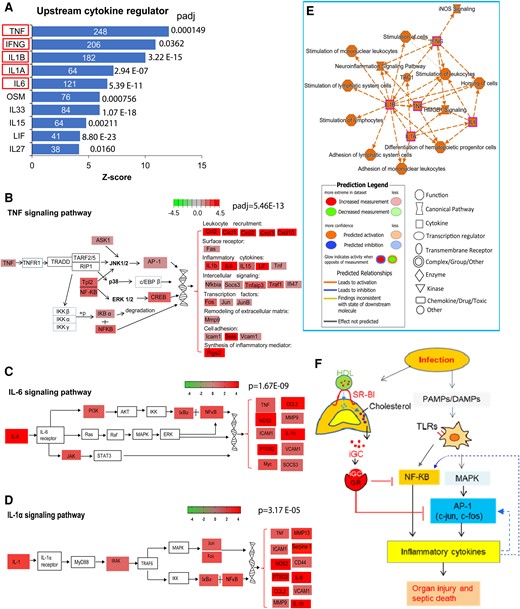
Inflammatory cytokines induce secondary inflammatory responses in mice with relative adrenal insufficiency. Liver RNA sequencing analysis was performed in 21-day-old SRBIfl/fl and SF1CreSRBIfl/fl mice at 6 hours after cecal ligation and puncture. A, Upstream regulator analysis of IPA identifies the upstream cytokine regulators in SF1CreSRBIfl/fl mice. Red rectangle highlights DEGs involved in GR signaling pathway. B, Modified TNF signaling pathway from KEGG. C and D, Modified IL-6 and IL-1α signaling pathways from IPA. E, A subset of the most significant entities’ interaction with legend for the IPA network. F, The mechanisms of how inducible glucocorticoid functions in pediatric sepsis. DEG, differentially expressed gene; IL, interleukin; IPA, ingenuity pathway analysis; KEGG, Kyoto Encyclopedia of Genes and Genomes; TNF, tumor necrosis factor.
In summary, the RNA-seq analysis revealed that, upon infection, pathogen-associated molecular patterns and DAMPs are recognized by TLRs. This recognition activates signaling pathways, including NF-κB and MAPK, which is followed by MAPK-induced activation of AP-1 transcription factor. AP-1 and NF-κB serve as transcriptional regulators to upregulate inflammatory cytokine genes. As a host response, the adrenal gland produces iGC in response to infection. iGC binding to GR suppresses gene expression of inflammatory cytokines by inhibiting multiple transcriptional regulators, such as AP-1 and NF-κB, and it suppresses the cytokine-induced secondary inflammatory response, which protects against organ injury and septic death. These protective effects are lost in RAI mice due to the lack of iGC (Figure 5F).
GC Supplementation Improves Survival in Mice With RAI
We tested the effect of GC supplementation on the survival of mice with RAI. Administration of a single dose of hydrocortisone significantly increased the survival rate of SF1CreSRBIfl/fl mice from 15.4% to 41.7% (Figure 6C); however, considering that the duration of action of hydrocortisone is about 8 to 12 hours, a single dose of hydrocortisone may not maintain its protective effect during sepsis. Then, we applied multiple dosages (5 dosages/5 days) of hydrocortisone treatment, resulting in an increased survival rate of SF1CreSRBIfl/fl mice to 71.4% (Figure 6D). In contrast, we observed no significant improvement in the survival rate of sepsis in SRBIfl/fl mice (Figure 6A and 6B). Taken together, these results suggest that GC therapy benefits mice with RAI.
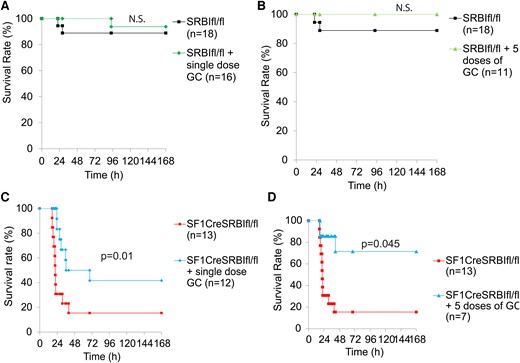
GC supplementation improves survival in mice with RAI. A and C, SRBIfl/fl and SF1CreSRBIfl/fl mice were injected with or without a single dose of hydrocortisone (5 mg/kg intraperitoneally) immediately after CLP treatment. B and D, SRBIfl/fl and SF1CreSRBIfl/fl mice received 5 consecutive daily doses of hydrocortisone (5 mg/kg/d) or not. In this case, the first dose was administered at 2 hours post-CLP, followed by daily doses for 5 days. Mice were monitored for 7 days for the survival rate. Data are expressed as the percentage of mice surviving at the indicated time points and analyzed by log-rank test. n = 7–18. CLP, cecal ligation and puncture; GC, glucocorticoid.
DISCUSSION
GC therapy had been strongly recommended for pediatric sepsis at grade 1A, but the recommendation was changed to grade 2C in 2020 due to insufficient evidence supporting or refuting its use. Thus, it is critical to provide mechanistic evidence to determine if GC therapy is beneficial in pediatric sepsis. In this proof-of-concept study, we used 21-day-old SF1CreSRBIfl/fl mice as a pediatric RAI model to elucidate the pathogenesis of RAI and evaluate the efficacy of GC therapy in CLP- and cecal slurry–induced sepsis. We demonstrated that (1) mice with RAI are susceptible to sepsis and feature hyperinflammatory responses; (2) RAI leads to hyperinflammatory responses, mainly due to transcriptional dysregulation of AP-1 and NF-κB, and a cytokine-induced secondary inflammatory response; and (3) GC therapy benefits mice with RAI.
RAI Is a Risk Factor and an Endotype in Pediatric Sepsis
In this study, we found that pediatric mice with RAI are susceptible to septic death and that supplementation of GC effectively rescues the mice. These results indicate that RAI is a risk factor in pediatric sepsis. We further found that septic mice with RAI displayed a persistent hyperinflammatory response and that targeting the hyperinflammatory response by a low-stress dose of GC rescued the mice. It is worth noting that extensive clinical trials targeting individual inflammatory cytokines have had limited effects [31–34]. One possible cause of the failure is that the inflammatory response is systemic and many inflammatory cytokines/chemokines contribute to sepsis. Targeting individual cytokines may have a limited effect. In this study, we showed that a panel of inflammatory cytokines, including IL-1β, IL-6, and TNF-α, is significantly increased, which supports this notion. Another plausible explanation is that patients who are septic are heterogenous in the status of an inflammatory response—not all have a persistent hyperinflammatory response. Targeting all patients who are septic without considering their inflammatory status may not benefit patients without a hyperinflammatory response. In this study, we found that mice without RAI have a well-controlled inflammatory response. On the contrary, mice with RAI developed a persistent hyperinflammatory response and that GC treatment rescued them.
RAI Causes a Hyperinflammatory Response due to Dysregulated Transcriptional Regulation of AP-1 and NF-κB
GC has been known to be a potent immune regulator, with a single dose of cortisol shown to alter the expression of 20% to 30% of genes [35]. Although extensive efforts have been made to understand the roles of GC and these studies have profoundly improved GC biology [36, 37], the mechanism of how iGC functions in sepsis, particularly in pediatric sepsis, remains largely unknown. In this study, we utilized RNA-seq analysis, an unbiased approach, to elucidate the mechanism of how iGC functions in pediatric sepsis. Previous reports have shown that GC regulates the inflammatory response through various signaling pathways, such as suppression of AP-1, NF-κB, STAT3, SMAD3, C/EBPα, GATA3, IRF3 (IFN regulatory factor 3), and T-bet (T-box expressed in T cells). However, these investigations were conducted under nonsepsis circumstances [38, 39]. In contrast, our RNA-seq analysis revealed that in pediatric septic mice, iGC controls inflammatory cytokine production mainly through transcriptional regulators AP-1 and NF-κB. Furthermore, our RNA-seq analysis revealed that iGC controls a cytokine-induced secondary inflammatory response. To the best of our knowledge, this is the first report demonstrating that cytokines induce a secondary inflammatory response in pediatric sepsis. These mechanistic findings explain that targeting an individual cytokine may not be sufficient in controlling the inflammatory response.
Our Study Supports the Use of a Precision Medicine Approach to Guide GC Therapy for Pediatric Patients
The current criterion for the use of GC in patients who are septic is poor response to fluid and vasopressor therapy [40]. This approach is frequently adopted in clinical practice based on empirical observations. However, it does not fully take into account the protective functions of GC in sepsis. In this study, we demonstrated that iGC protects against sepsis by preventing a hyperinflammatory response in 21-day-old septic mice. The sepsis guideline recommends administrating hydrocortisone up to 50 mg/kg in pediatric sepsis [2], which is much higher than the body's natural level. In this study, we opted for a lower dose of hydrocortisone (5 mg/kg) to treat septic mice, with the anticipated plasma GC concentrations reaching endogenous stress levels of approximately 700 ng/mL, as shown in a previous report [41]. The results indicated that GC supplementation rescued septic mice with RAI. Our study provides mechanistic support for a precision medicine approach to guide GC therapy—specifically, selective GC therapy for a subgroup of pediatric patients with sepsis and RAI.
Of note, the current guidelines make no recommendation for diagnosis of RAI for pediatric sepsis. The ongoing SHIPSS clinical trial (Stress Hydrocortisone in Pediatric Septic Shock) tests GC therapy in pediatric patients with sepsis without considering the status of RAI. Our study suggests that checking RAI in pediatric cases of sepsis and applying GC therapy based on the status of RAI may improve the efficacy of GC therapy. A further clinical trial is needed to test this speculation.
Justification of the Sepsis Models
The National Institutes of Health recently issued a notice to point out the limitations of the CLP sepsis model due to potential differences in host response between humans and rodents. iGC production is a hallmark of sepsis in humans, as shown by a 5- to 10-fold increase in cortisol levels in patients with sepsis [8]. A number of previous studies, including ours, showed that mice also have a 5- to 10-fold increase in corticosterone levels upon CLP-induced sepsis [23, 24, 42, 43], which indicates a similar adrenal stress response to sepsis in humans and rodents. These findings suggest that CLP is an appropriate model to elucidate the role of iGC in sepsis. In addition to a CLP model, we used a cecal slurry model to confirm sepsis survival in mice with RAI. Nevertheless, further clinical study is required to validate the role of iGC and the efficacy of GC therapy in pediatric patients with RAI.
Supplementary Data
Supplementary materials are available at The Journal of Infectious Diseases online. Consisting of data provided by the authors to benefit the reader, the posted materials are not copyedited and are the sole responsibility of the authors, so questions or comments should be addressed to the corresponding author.
Notes
Author contributions. X.-A. L. conceptualized and oversaw studies and procured funding. D. H., L. G., Q. W., M. I., and B. H. performed experiments and analyzed or interpreted data. C. M. and P. W. S. generated SR-BI–floxed mice. D. H. and X.-A. L. wrote the manuscript. All authors had final approval of manuscript submission.
Disclaimer. The contents of this article are solely the responsibility of the authors and do not necessarily represent the official views of the National Institutes of Health.
Financial support. This work was supported by the National Institutes of Health (R01GM121796 and 1R35GM141478 to X.-A. L.; R01HL126795 to C. M.; R01HL131597 to P. W. S.).
References
Author notes
Potential conflicts of interest. The authors declare no conflict of interest.
All authors have submitted the ICMJE Form for Disclosure of Potential Conflicts of Interest. Conflicts that the editors consider relevant to the content of the manuscript have been disclosed.