-
PDF
- Split View
-
Views
-
Cite
Cite
Nathan R Schwab, Natalie E Young, Davidson U Nzenwata, Evelyn Toh, Joseph A Mikulin, Timothy J Wilson, David E Nelson, Mitchell F Balish, Characterization of Virulence-Associated Traits in Mycoplasma penetrans Strains Acting as Likely Etiological Agents of Idiopathic Nongonococcal Urethritis, The Journal of Infectious Diseases, Volume 227, Issue 9, 1 May 2023, Pages 1050–1058, https://doi.org/10.1093/infdis/jiac505
- Share Icon Share
Abstract
Mycoplasma penetrans is an emerging pathogen with a reduced genome. This bacterium has only previously been cultured from individuals with chronic immunodeficiencies. Here we report the characteristics of 4 M. penetrans isolates from the urine of immunocompetent males with nongonococcal urethritis, in comparison with strain HF-2 from an immunocompromised patient. Several features exhibited distinct differences between these isolates and HF-2. Unlike HF-2, all 4 were resistant to azithromycin. They exhibited greater sialic acid-dependent binding to erythrocytes, gliding motility speed, and H2O2 production than HF-2. All new isolates produced thinner capsules than HF-2. Invasiveness varied, with some isolates being more invasive than HF-2 and some less invasive. Cytotoxicity to HeLa cells was similar to HF-2, and all strains could clear extracellular traps produced by innate immune cells. We conclude that subtle differences among M. penetrans strains may be critical for this organism to establish an infection in an otherwise healthy individual.
Mycoplasma penetrans is a cell wall-lacking bacterium that has been detected in and isolated from people with AIDS and from an individual with primary antiphospholipid syndrome [1, 2]. Whereas it is primarily found in the urogenital tract, M. penetrans has also been isolated from the blood and respiratory tract [2]. M. penetrans can invade host cells and reside within the cytoplasm, a phenomenon that has been observed in vivo and in vitro [3]. Host cell invasion is dependent on actin polymerization within the host cell with the attachment organelle facilitating penetration [3, 4]. The frequency of M. penetrans in individuals with clinical AIDS is high, with up to 40% showing immunoreactivity to M. penetrans surface proteins [5]. M. penetrans has the capacity to infect both men and women [6, 7]. The outcome of a prolonged infection is not yet understood, but it is clear that M. penetrans can disseminate and thrive in multiple tissues [2, 6].
Since its discovery, M. penetrans has been regarded primarily as an opportunist of the immunocompromised. However, recent work has challenged that categorization. In a metagenomic study the presence of M. penetrans was reported in up to 25% of immunocompetent men presenting idiopathic nongonococcal urethritis (NGU), with M. penetrans being the only pathogen found in 6.8% of participants [7], strongly suggesting that M. penetrans has the potential to cause disease in men, either in collaboration with other bacteria or as the primary pathogen. Although in that study M. penetrans was more frequent in men who have sex with men [7], it has also been detected in men who have sex with women (manuscript in preparation). Although other mycoplasma species, such as Mycoplasma hominis and Mycoplasma genitalium, have been described as causing a variety of complications in NGU [8–11], the consequence of an M. penetrans infection in women or a prolonged infection in men is unknown.
Potential virulence factors of M. penetrans include its invasiveness and the production of cytotoxic molecules, including H2O2 and cytopathic proteins [12–14], among which is a homolog of the community-acquired respiratory distress syndrome (CARDS) toxin of Mycoplasma pneumoniae that causes vacuolation of host cells [15]. Antibodies from individuals infected with M. penetrans react to this protein, confirming its production in vivo [15]. Some bacteria can utilize a polysaccharide capsule to prevent phagocytosis and antigen recognition [16]; although M. penetrans produces a capsule with strain-dependent thickness [17], its function remains unknown. Additional characteristics of M. penetrans that might contribute to virulence are motility, phase-variable surface proteins, and a cytotoxic membrane-bound nuclease [14, 18, 19].
The underdiagnosed nature of M. penetrans is not surprising given the difficulty of detecting, isolating, and culturing mycoplasmas. We were able to isolate and culture M. penetrans from the urine of immunocompetent patients with NGU enrolled in the Idiopathic Urethritis Men's Project ([20]; manuscript in preparation) in whom no other NGU-associated pathogen was detected by metagenomics, and sought to understand the differences between these strains and M. penetrans strain HF-2, whose genome has been sequenced [21]. The question of whether M. penetrans strains isolated from immunocompromised and immunocompetent patients differ in ways that reveal important virulence factors, and therefore might influence therapeutic approaches, was addressed in this study, focusing on antibiotic resistance, production of H2O2, and features associated with interaction with host cells. The results, constituting the first description of M. penetrans acting as a likely etiologic agent of NGU, reveal potentially important information not only about these differences, but also about the likelihood of each of these features to contribute substantially to virulence.
METHODS
Cell Culture
M. penetrans strains HF-2 [2] and U2, U4, U5, and U7, cultured from the urine of patients with NGU in whose urethral swabs M. penetrans was the dominant organism detected by metagenomic analysis (manuscript in preparation), were cultured in SP4 medium [22] at 37°C. Stocks were maintained at −80°C and were broken up with a 25-gauge needle to separate the cells prior to inoculation. All studies were performed using the fourth passage of each isolate.
THP-1 monocytes (American Type Culture Collection [ATCC] TIB-202) were cultured in Roswell Park Memorial Institute (RPMI) 1040 medium (Sigma) with 10% serum at 37°C with 5% CO2. Quantification of cells to be used for each experiment was performed using a hemocytometer. Prior to experimentation, THP-1 cells were centrifuged at 200g for 10 minutes and the pellet was resuspended in fresh RPMI.
HeLa cervical epithelial cells (ATCC CCL-2) were maintained in Dulbecco's modified Eagle's medium (DMEM) (HyClone) at 37°C in 5% CO2. When 70%–80% confluency was reached, the cells were treated using dissociation reagent (Gibco) and subcultured in DMEM. Harvested cells were centrifuged at 2000g for 5 minutes and the supernatant was removed. The cells were then resuspended in fresh DMEM and dispersed using a 25-gauge needle to ensure a uniform cell suspension. The cells were allowed at least 6 hours to recover prior to the start of any infection.
Minimum Inhibitory Concentration
The minimum inhibitory concentration (MIC) of antibiotics was determined by using a previously described method [23]. Briefly, serial 2-fold dilutions of the antibiotic beginning at 512 μg/mL were made in a 96-well plate. Each well was inoculated with 1.0 × 105 cells and the plate was incubated in a humidified incubator for 48 hours at 37°C. Growth was determined by visual inspection of a color change of the pH indicator from red to yellow. Growth controls with no added drug or the greatest amount of ethanol (for azithromycin) were included, in addition to a negative control with no added bacteria for the growth medium and drug of interest. Three separate experiments were performed for each drug.
DNA Sequencing
Late log-phase M. penetrans cells of strains U4 and U7 were pelleted and subjected to pronase digestion followed by incubation with RNase A. The DNA was extracted using phenol and chloroform, followed by an ethanol precipitation and subsequent ethanol wash. The NexteraXT DNA library preparation kit was used to prepare sequencing libraries as per the manufacturer's protocols (Illumina). Sample libraries were multiplexed and sequenced using the Miseq reagent kit version 3 (600-cycle) on an Illumina MiSeq platform.
Hemadsorption
Hemadsorption was assayed as previously described [18, 24, 25]. Bacteria were plated on SP4 agar plates and incubated at 37°C until colonies formed (7–10 days). Sheep red blood cells (SRBCs) in 1:1 Alsever's solution (Colorado Serum Co.) were diluted 1:200 in 0.085% saline and warmed to 37°C. The bacterial colonies were flooded with 1.0 mL of the SRBC suspension and incubated for 30 minutes at 37°C, followed by 3 washes with phosphate-buffered saline (PBS; 145 mM NaCl, 2.83 mM NaH2PO4 H2O, 7.20 mM NaHPO4 7H2O [pH 7.2]). The relative adsorption of the colonies was ranked on a scale of 0–3 based on the degree to which the colonies were covered by SRBCs (3, completely covered; 2, mostly covered; 1, mostly bare; 0, completely bare). Representative images of each colony were captured using the 40 × objective on a Leica DM IRB microscope. Neuraminidase treatment of SRBCs was performed as previously described [26], and hemadsorption assays were also carried out on neuraminidase-treated SRBCs.
Cell Motility
Gliding motility analysis was performed as previously described [18]. Cells were inoculated in SP4 with 3% gelatin and incubated at 37°C for 4 hours. Images of the cells were captured using the 100 × objective lens on a Leica DM IRB microscope at 1.0-second intervals. Speed was calculated based on the distance traveled continuously by individual cells. Cells that collided or stopped were excluded.
Capsule Measurement
Capsule thickness was determined by ruthenium red staining of samples visualized by transmission electron microscopy (TEM), as previously described [17]. Briefly, M. penetrans cells were grown to late log phase in 75-cm2 culture flasks. The cells were fixed at room temperature (0.075% ruthenium red, 3.0% glutaraldehyde, in 0.05 M sodium cacodylate), washed twice in PBS, and incubated in a buffer with osmium tetroxide (0.05 M sodium cacodylate, 0.075% ruthenium red, 3.0% OsO4) for an additional 2 hours. The flasks were washed 3 times, scraped, and centrifuged at 17 400g for 20 minutes. The pellets were then serially dehydrated in acetone and embedded in Spurr's resin [27]. Sections of embedded samples were imaged on a JEM-1200EX II TEM. Measurements of extracellular capsule were made using ImagePro 7.1 software using the scale bar on the micrograph to ensure consistency.
Cytotoxicity
Approximately 2 × 104 HeLa cells were seeded in a 96-well plate (Fisher Scientific) and allowed to recover in 100 µL DMEM. The cells were then infected with M. penetrans strains at a multiplicity of infection (MOI) of 100 for the times indicated in the “Results” section. A lactate dehydrogenase (LDH)-Glo (Promega) cytotoxicity kit was then used according to the manufacturer's instructions. Briefly, at each time point, the medium was evaluated for LDH activity in white 96-well plates (Costar) using a luminescence assay. Luminescence was measured on an iD5 Spectramax. This procedure was performed in triplicate.
H2O2 Production
H2O2 produced from glycerol metabolism was detected using previously described methods [28, 29]. Briefly, M. penetrans strains were grown to late log phase in 175-cm2 culture flasks with 50 mL of SP4. The cells from each flask were scraped and centrifuged at 20 000g for 20 minutes at 4°C, followed by 3 washes with cold HEPES buffer (140 mM NaCl, 7 mM MgCl2, and 67 mM HEPES [pH 7.3]) and subsequent centrifugations using the same parameters. The final cell suspension in HEPES buffer (see above) was normalized to an optical density at 550 nm (OD550) of 1.0, and 1.0-mL aliquots were dispensed into microcentrifuge tubes, followed by a 1-hour incubation at 37°C. Sterile glycerol was added to each tube to a final concentration of 100 µM, followed by an additional 2-hour incubation at 37°C. The amount of H2O2 produced was measured using colorimetric test strips (Quantofix) according to the manufacturer’s instructions. This procedure was performed in triplicate, with each replicate representing a separate experiment.
Host Cell Invasion
Approximately 2.0 × 105 HeLa cells were seeded in a 24-well plate (Fisher Scientific) and suspended in 1.0 mL of DMEM. After recovery, the cells were infected with M. penetrans strains at an MOI of 10 and incubated for the appropriate amount of time (see “Results” section). To measure the number of cells that had invaded versus those that had not, one set of host cells was treated with DMEM containing Triton X-100 (0.01%) and gentamicin (400 μg/mL) for 2 hours prior to dissociation and plating, whereas another was not treated. Serial dilutions were made in SP4 broth, and the samples were plated on SP4 agar plates and incubated at 37°C for 14 days. Colony forming units were quantified and the percent recovered was calculated.
Macrophage Extracellular Trap Clearing
Extracellular traps (ETs) were produced by THP-1 cells as previously described [30]. Briefly, 2.0 × 105 THP-1 cells were inoculated in a 24-well plate (Fisher Scientific) and differentiated in RPMI with 10% serum and 100 nM phorbol myristate acetate (PMA) for 24 hours. The medium was then removed, and the unstimulated control cells were submerged in PMA-free RPMI with 10% serum, whereas the stimulated cells were submerged in RPMI with 0.5% serum and 200 nM PMA for 48 hours. M. penetrans cells were introduced to the stimulated THP-1 cells for 6 hours at an MOI of 100. SYTOX green (Invitrogen) was used (100 nM) to fluorescently label DNA, which was imaged using a Leica DM IRB microscope.
Statistics
For hemadsorption analysis, a 1-way analysis of variance with Tukey post hoc test, run on Graphpad Prism 6.07, was applied to evaluate statistical significance. For other analyses, statistical significance was determined by a Student t test. The resulting P value is reported in each figure.
RESULTS
Antibiotic Resistance
To provide an in vitro comparison of antibiotic resistance with representatives of commonly prescribed classes of antibiotics, we performed MIC assays using azithromycin, the previous standard of care for NGU [31], tetracycline, moxifloxacin, or doxycycline, the current standard of care. All 4 of the patients from whom the isolates originated had failed azithromycin therapy but responded to moxifloxacin (manuscript in preparation). All 4 strains showed complete resistance to azithromycin, unlike strain HF-2 (Table 1), congruent with preliminary sequencing data showing the A2059G mutation in the 23S rDNA gene for these isolates (not shown). There were smaller differences in sensitivity to the other drugs among the strains and compared to HF-2 (Table 1), none of which would suggest clinical resistance.
The Minimum Inhibitory Concentration (μg/mL) of Various Drugs Used on Mycoplasma penetrans Strains
Strain . | Azithromycin . | Moxifloxacin . | Doxycycline . | Tetracycline . |
---|---|---|---|---|
U2 | >512 | 32–64 | 32–64 | 64–256 |
U4 | >512 | 128–256 | 256 | 512 |
U5 | >512 | 64 | 256 | 512 |
U7 | >512 | 64 | 128–256 | 256 |
HF-2 | 0.8–1.6 | 64 | 256–512 | >512 |
Strain . | Azithromycin . | Moxifloxacin . | Doxycycline . | Tetracycline . |
---|---|---|---|---|
U2 | >512 | 32–64 | 32–64 | 64–256 |
U4 | >512 | 128–256 | 256 | 512 |
U5 | >512 | 64 | 256 | 512 |
U7 | >512 | 64 | 128–256 | 256 |
HF-2 | 0.8–1.6 | 64 | 256–512 | >512 |
The Minimum Inhibitory Concentration (μg/mL) of Various Drugs Used on Mycoplasma penetrans Strains
Strain . | Azithromycin . | Moxifloxacin . | Doxycycline . | Tetracycline . |
---|---|---|---|---|
U2 | >512 | 32–64 | 32–64 | 64–256 |
U4 | >512 | 128–256 | 256 | 512 |
U5 | >512 | 64 | 256 | 512 |
U7 | >512 | 64 | 128–256 | 256 |
HF-2 | 0.8–1.6 | 64 | 256–512 | >512 |
Strain . | Azithromycin . | Moxifloxacin . | Doxycycline . | Tetracycline . |
---|---|---|---|---|
U2 | >512 | 32–64 | 32–64 | 64–256 |
U4 | >512 | 128–256 | 256 | 512 |
U5 | >512 | 64 | 256 | 512 |
U7 | >512 | 64 | 128–256 | 256 |
HF-2 | 0.8–1.6 | 64 | 256–512 | >512 |
Adherence and Gliding Motility
Given the reduced metabolic capability of mycoplasmas, close association with the host cell is crucial for acquisition of nutrients. Colonies of 3 of the newly isolated M. penetrans strains bound SRBCs more efficiently than strain HF-2 in hemadsorption assays (Figure 1A and Supplementary Figure 1), suggesting greater ability to adhere to host cells; for strain U2, there was increased binding but the P value was .0624. Sialic acid is a common binding target for mycoplasmas [32]. To determine the role of sialic acid in hemadsorption, we digested the SRBCs with neuraminidase to remove sialic acid from their surfaces, which sharply reduced adsorption of the M. penetrans strains to the SRBCs (Figure 1B and Supplementary Figure 1). It is noteworthy that although HF-2 binding was largely dependent upon sialic acid, neuraminidase treatment inhibited binding of the newly isolated strains to a greater extent (Figure 1B). Taken together these results suggest that in comparison to strain HF-2, the 4 new M. penetrans isolates bind more tightly to host cell sialic acid and/or are less reliant on other ligands.
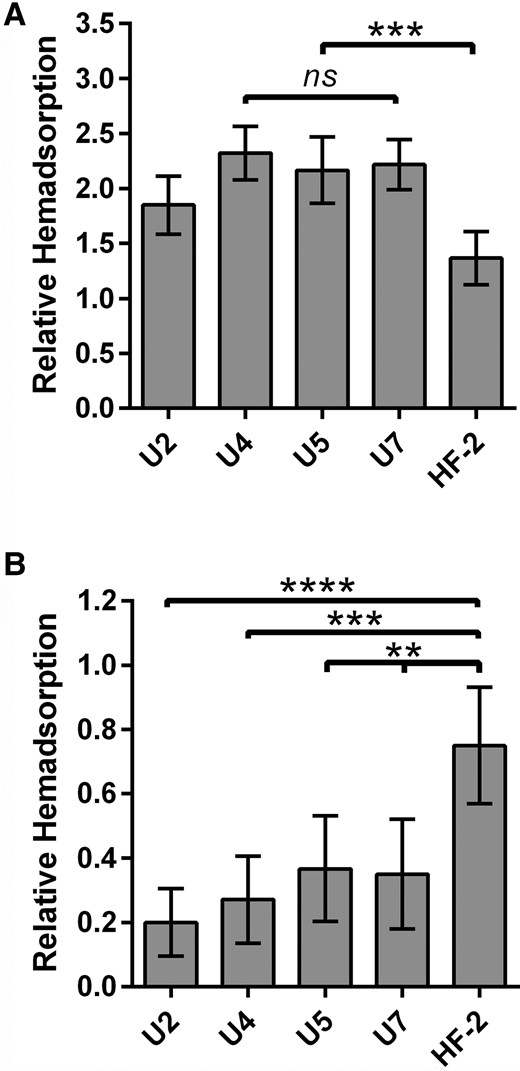
Relative hemadsorption by Mycoplasma penetrans isolates. Semiquantitative measure of the relative adsorption of M. penetrans isolates to untreated (A) or neuraminidase-treated (B) sheep red blood cells as described in “Methods”. For each strain, n = 60. Statistical comparison to HF-2: ns, not significant; **P < .01; ***P < .001, ****P < .0001. Error bars, 95% confidence interval.
Gliding motility is generally a feature of polarized mycoplasma species like M. penetrans. Like strains HF-2 and GTU-54-6A1 [18], all 4 new isolates exhibited gliding motility (Table 2). Although the relationship between gliding speed and virulence is poorly understood, among the newly isolated strains, both the mean gliding speed and ranges of speeds achieved by individual cells were highly variable (Table 2) and different from both strains HF-2 and GTU-54-6A1 [18].
Gliding Speed and Capsule Thickness of Mycoplasma penetrans Clinical Isolates
Strain . | Speed, nm/s, (SD) . | Capsule Thickness, nm, (SD) . |
---|---|---|
U2 | 290 (337)a | 16.7 (4.60)a |
U4 | 677 (684)a | 15.5 (4.29)a |
U5 | 412 (542)a | 14.8 (3.62)a |
U7 | 429 (512)a | 13.7 (2.62)a |
Gliding Speed and Capsule Thickness of Mycoplasma penetrans Clinical Isolates
Strain . | Speed, nm/s, (SD) . | Capsule Thickness, nm, (SD) . |
---|---|---|
U2 | 290 (337)a | 16.7 (4.60)a |
U4 | 677 (684)a | 15.5 (4.29)a |
U5 | 412 (542)a | 14.8 (3.62)a |
U7 | 429 (512)a | 13.7 (2.62)a |
The ability to form a capsule is critical for some organisms to prevent phagocytosis or mask antigens from the immune system [33]. Capsule thickness of M. penetrans differs among strains [17]. We measured the thickness of the capsules formed by the newly isolated strains to compare with previously described measurements [17] of strains HF-2 (25–30 nm) and GTU-54-6A1 (11–13 nm) (Table 2). At 14–17 nm, the capsule thicknesses of the new isolates were closer to strain GTU-54-6A1, which was also isolated from the urogenital tract, unlike strain HF-2.
Host Cell Invasion
The ability to invade host cells is one of the hallmark features of M. penetrans [1]. We tested whether there was a difference in the efficiency of HeLa cell invasion among the newly isolated strains of M. penetrans and HF-2. The results indicated a wide range of invasive behavior (Figure 2). Strain U7 exhibited comparatively poor invasion at both 16 and 32 hours postinfection, with ≤1% invasion, whereas 15% and 30% of strain U5 cells invaded host cells at the respective time points. HF-2, U2, and U4 were intermediate. The ability of each strain to invade was not commensurate with hemadsorption ability (Figure 1) and was also unrelated to gliding speed (Table 2).
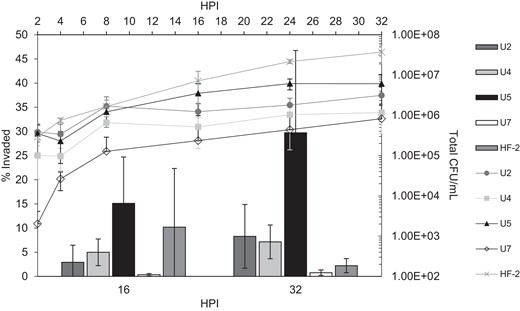
Assay for HeLa cell invasion by Mycoplasma penetrans isolates. The bar graph shows the percentage of the total population of bacteria that had invaded host cells by 16 and 32 hours postinfection (HPI), measured as described in “Methods”. The line graph (secondary axes) shows the total colony forming units (CFUs) available at the given time point. Three biological replicates were performed for each strain at each time point. Error bars, SD.
Cytotoxicity
Little difference in toxicity to HeLa cells was observed among isolates (Figure 3). Several pathogenic mycoplasmas rely on the production of toxic molecules to help establish an infection, among which are H2O2, which is produced mainly from metabolism of glycerol and glycerol-containing membrane lipids [34, 35]. To evaluate the role of H2O2 in cytotoxicity, we measured its production from glycerol across isolates. Under assay conditions, H2O2 was detected in each of the newly isolated strains of M. penetrans, but not strain HF-2. Production by strains U2 and U7 exceeded that of M. pneumoniae strain M129 (Figure 4). The differential capacity to produce H2O2 in the face of little difference in cytotoxicity suggests the possibility that H2O2 production is not a significant virulence factor for M. penetrans, emphasizing the importance of other virulence factors.
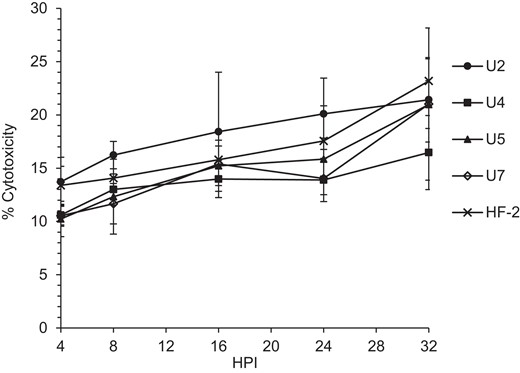
Cytotoxicity of Mycoplasma penetrans isolates to HeLa cells. After infection of HeLa cells as described in “Methods”, a lactate dehydrogenase-based cytotoxicity assay was used to evaluate cytotoxicity. There was no statistically significant difference compared to strain HF-2 at any time point. For each strain, there were 3 technical replicates of 10 biological replicates at each time point. Error bars, SD. Abbreviation: HPI, hours postinfection.
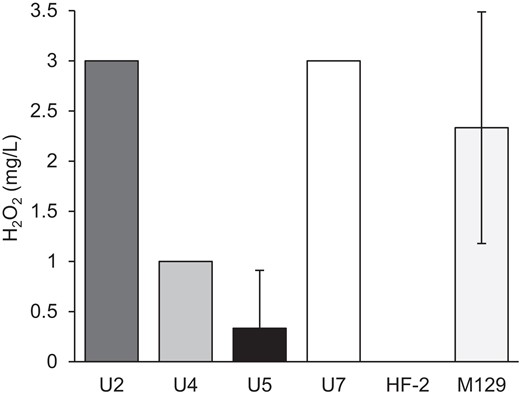
H2O2 production by Mycoplasma penetrans isolates. Production of H2O2 upon incubation with glycerol was measured as described in “Methods” for each strain of M. penetrans and compared to Mycoplasma pneumoniae M129. For each strain there were 3 replicates. Error bars, SD.
Macrophage Extracellular Traps
Mycoplasmas have a variety of mechanisms to evade the host immune system, including deployment of phase-variable immunodominant surface proteins to avoid the adaptive immune response, and nuclease activity to escape from ETs from the innate immune response. M. penetrans has a large family of highly phase-variable surface proteins [19, 36]. However, whether these bacteria can escape ETs has yet to be described. Like many mycoplasmas, M. penetrans has a membrane-bound nuclease that presumably aids in the acquisition of nucleotides [37, 38]. We used THP-1 cells as a proxy for ETs produced by immune cells as previously described [30]. Each of the M. penetrans strains demonstrated the ability to clear the ETs produced by the THP-1 cells (Figure 5), albeit with differing efficiency. Remnants of ETs were occasionally visualized in strains U4 and U7 (Figure 5, white arrows), suggesting that these strains may degrade the ETs more slowly than the others.
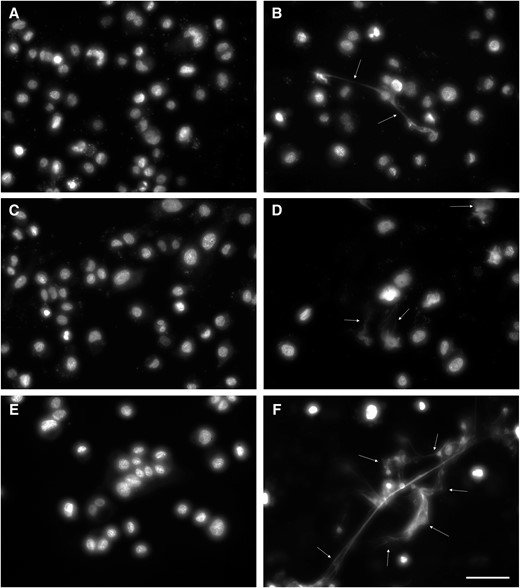
Degradation of extracellular traps produced by THP-1 cells. THP-1 cells were stimulated to produce extracellular traps and then incubated with Mycoplasma penetrans isolates as described in “Methods” and imaged using SYTOX green DNA stain. Strains U2, U4, U5, and U7 (A, B, C, and D, respectively) all showed the ability to clear the DNA released by the THP-1 cells, though strains U4 and U7 infrequently left some intact. Unstimulated (E) and stimulated (F) THP-1 cells are shown as controls. White arrows, extracellular traps; scale bar, 50 μm.
DISCUSSION
Reports of M. penetrans as an infectious agent in individuals not known to be immunocompromised are uncommon. To our knowledge, this is the first report describing isolates from human immunodeficiency virus (HIV)-negative men with NGU and no other NGU-associated pathogen detected (manuscript in preparation). To understand what characteristics may promote the ability of these organisms to cause NGU in immunocompetent patients, we sought to characterize them in comparison to strain HF-2, an isolate from a patient with an immune disorder, and whose genome sequence is published [2, 21]. We undertook a series of experiments to elucidate specific characteristics associated with virulence, aiming to rule out those that were not different in HF-2.
Three characteristics that center on the attachment organelle are adherence, motility, and host cell invasion. The new isolates had greater adherence than HF-2, but not greater invasiveness (Figure 1 and Figure 2). In fact, U7 was scarcely invasive, indicating that adherence and invasion may be at least partially independent, and that host cell invasion is not dependent solely upon a sialylated ligand. Although the contribution of gliding speed to virulence is unknown, the new isolates glided far faster than HF-2 [18] (Table 2). Capsule thickness, which may be related to adherence and/or immune evasion, was different from HF-2 but more similar to a strain from the urogenital tract of a person with HIV (Table 2), suggesting a potential connection between this feature and site of infection but not necessarily the ability to cause NGU.
Production of toxic molecules, including H2O2, H2S, and CARDS toxin, is well documented in M. pneumoniae and can impact virulence [35, 39, 40]. We tested the ability of each of the M. penetrans isolates to produce H2O2 from added glycerol and found that each isolate generated more H2O2 than HF-2, with strains U2 and U7 both producing amounts comparable to M. pneumoniae strain M129 (Figure 4). In M. pneumoniae the production of H2S relies on cysteine [40]. In M. penetrans the production of H2S is undescribed; however, there is evidence that hemolysis is enhanced in the presence of cysteine [13]. Additionally, although M. penetrans has a CARDS toxin-like protein, it remains unknown whether its production varies across strains. In M. penetrans, an invasive bacterium, how these toxins are deployed in vivo is uncertain. Interestingly, whereas the newly isolated strains showed greater H2O2 production with glycerol as a substrate (Figure 4), differences in the overall cytotoxicity were mainly statistically insignificant (Figure 3). These data suggest that each strain might present independent strategies for cytotoxicity, which could include modulation of the levels of toxic molecules, as well as features that remain to be tested, including immune evasion.
Membrane-bound and/or secreted nucleases are an extremely important feature of mycoplasmas, breaking down exogenous DNA to acquire nucleotides [30]. This process presents an effective way to escape from ETs produced by the innate immune system and promote the survival of the bacteria [30, 41, 42]. Each strain of M. penetrans demonstrated the ability to degrade ETs in our experiments, although there was a suggestion that strains U4 and U7 may be somewhat less efficient. M. penetrans has a membrane-bound nuclease, P40 [38], which is a candidate for involvement in this process.
In other mycoplasmas that infect humans, including M. pneumoniae and Mycoplasma genitalium, resistance to macrolides is increasing [43, 44]. Preliminary genome sequence data from the isolates, in accordance with treatment outcomes (data not shown) predict resistance to azithromycin, concordant with our MIC data (Table 1), and distinct from strain HF-2.
In this study we were able to document some differences between the newly isolated strains of M. penetrans and strain HF-2; however, we lack a model for understanding the contributions of these differences to infection and disease. The importance of gliding speed and capsule thickness is unknown, and although it is tempting to speculate that they could play a role in virulence, given the dramatic differences compared to HF-2, the new isolates are very similar in both areas to strain GTU-54-6A1 [18]. Additionally, we were able to demonstrate the ability to escape ETs produced by cells of the innate immune system; however, strain HF-2 was not different in this regard (Supplementary Figure 2). Other immune evasion strategies were not identified in this study. Both the CARDS-like toxin and the use of sialylated compounds as primary binding receptors on the host cells are subjects of extreme interest to further understand how these organisms can establish themselves in their hosts.
Supplementary Data
Supplementary materials are available at The Journal of Infectious Diseases online. Consisting of data provided by the authors to benefit the reader, the posted materials are not copyedited and are the sole responsibility of the authors, so questions or comments should be addressed to the corresponding author.
Notes
Acknowledgments. We thank the Miami University Center for Bioinformatics and Functional Genomics for instrumentation and support as well as M. Duley (Miami University) and the Miami University Center for Advanced Microscopy and Imaging for help with and use of the electron microscope.
Financial support. This work was supported by the National Institute of Allergy and Infectious Disease of the National Institutes of Health (grant number RO1AI116706 to D. E. N.).
References
Author notes
Presented in part: 23rd Congress of the International Organization for Mycoplasmology, November 2021, online.
Potential conflicts of interest. All authors: No reported conflicts of interest. All authors have submitted the ICMJE Form for Disclosure of Potential Conflicts of Interest. Conflicts that the editors consider relevant to the content of the manuscript have been disclosed.