-
PDF
- Split View
-
Views
-
Cite
Cite
Travis B Nielsen, Jun Yan, Matthew Slarve, Rachel Li, Jason A Junge, Brian M Luna, Ian Wilkinson, Udaya Yerramalla, Brad Spellberg, Development of a Bispecific Antibody Targeting Clinical Isolates of Acinetobacter baumannii, The Journal of Infectious Diseases, Volume 227, Issue 9, 1 May 2023, Pages 1042–1049, https://doi.org/10.1093/infdis/jiac499
- Share Icon Share
Abstract
We previously reported developing 2 anticapsular monoclonal antibodies (mAbs) as a novel therapy for Acinetobacter baumannii infections. We sought to determine whether a bispecific mAb (bsAb) could improve avidity and efficacy while maximizing strain coverage in one molecule.
Humanized mAb 65 was cloned into a single-chain variable fragment and attached to humanized mAb C8, combining their paratopes into a single bsAb (C73). We tested bsAb C73’s strain coverage, binding affinity, ex vivo opsonic activity, and in vivo efficacy compared to each mAb alone and combined.
The bsAb demonstrated strain coverage, binding affinity, opsonization, and in vivo efficacy superior to either original mAb alone or combined.
A humanized bsAb targeting distinct A. baumannii capsule moieties enabled potent and effective coverage of disparate A. baumannii clinical isolates. The bsAb enhances feasibility of development by minimizing the number of components of a promising novel therapeutic for these difficult-to-treat infections.
Acinetobacter baumannii is one of the most common causes of extreme and pan drug-resistant clinical infections [1–3]. For this reason, carbapenem-resistant A. baumannii is one of the highest priority antimicrobial-resistant pathogens identified by the World Health Organization that urgently needs new therapeutic options [4]. Experts have called for novel approaches to treating such infections, including vaccines and immunotherapies, which are expected to reduce antibiotics usage and therefore further emergence of antibiotic resistance [5–7].
The capsule of A. baumannii is one of its principal virulence factors, enabling the organism to escape host defense mechanisms and cause lethal disease [8–14]. Neutralization of capsule diminishes its virulence, and we have shown that that very low doses (eg, <5 μg) of humanized anticapsular monoclonal antibodies (mAbs) protect against A. baumannii bacteremia and pneumonia in lethal infection models [15, 16]. However, a challenge to this approach is the high degree of capsular diversity among A. baumannii strains, resulting in relatively low strain coverage for individual anticapsular mAbs [10, 15, 16]. The potential need to administer numerous, unique mAbs to achieve robust strain coverage represents a barrier to drug development, as individual mAbs would have to be studied separately and combined in multiple arms in both preclinical toxicity studies and clinical trials. We sought to determine if a bispecific mAb (bsAb) could help overcome this obstacle by enabling expanded strain coverage in one molecule while maintaining binding affinity and in vivo efficacy against a broad array of A. baumannii clinical isolates.
METHODS
Construction of the Bispecific mAb
The bispecific IgG-scFv was constructed with mAb C8 as a human IgG1 with a 10 amino acid glycine-serine linker on the C-terminus of the heavy chain linked to a single-chain Fv of mAb 65. Heavy chain and light chain genes were synthesized and cloned into a pUV vector (Absolute Antibody). Transient expression was performed by chemical transfection of suspension HEK293 cells grown in serum-free media. Cells were cultured for 6 days and then harvested by centrifugation. Clarified supernatant was loaded on to a MAbselect Sure Protein A column (GE Healthcare) using an AKTA purifier system and antibody was eluted with citrate buffer at pH 3.0. Eluate was neutralized with 0.5 M Tris pH 9.0 and buffer exchanged into phosphate-buffered saline (PBS) using a PD-10 desalting column (Cytiva). Purified antibody was analyzed for purity by sodium dodecyl sulfate polyacrylamide gel electrophoresis (SDS-PAGE) and concentration was determined by absorbance at 280 nm using a spectrophotometer (Absolute Antibody).
Bacterial Binding by Flow Cytometry
To assess surface binding of antibodies, we used our previously published flow cytometry-based method [15, 16]. In brief, A. baumannii subcultures were passaged for 3 hours to mid-log growth, rinsed 3 times in PBS, and then resuspended in PBS supplemented with 0.1% NaN3 (to prevent contamination in flow cytometer). Bacteria were adjusted to 1 × 108 colony-forming units (CFU)/mL and combined with mAb C8, mAb 65, or bsAb C73 at 10 μg/mL in a microwell plate, gently mixed with a plate vortexer, and incubated for 30 minutes at 37°C. Cells were rinsed, stained by Alexa Fluor 647-conjugated anti-human IgG secondary antibody, and incubated for 30 minutes at 37°C. Cells were rinsed and flow cytometry analysis was conducted on a Accuri C6 plus flow cytometer (BD). Any binding more than 1% above mouse anti-KLH IgG1 isotype control (R & D Systems, catalog No. MAB002) was considered positive.
Confocal Immunofluorescence Microscopy
To detect surface binding of A. baumannii by mAbs, as previously described [15, 16] frozen bacteria were fixed with 4% paraformaldehyde in PBS for 5 minutes, rinsed 3 times with PBS, blocked for 15 minutes in PBS with 1% bovine serum albumin (BSA; Thermo Fisher, catalog No. 23209) and 0.1% Triton X (VWR, catalog No. AAA16046-AE), and then incubated with 10 μg/mL primary antibody (mAb C8, mAb 65, bsAb C73, or isotype control) for 1 hour at 37°C. Bacteria were then rinsed with PBS and blocked in PBS with 10% goat serum (Thermo Fisher, catalog No. 50062Z) for 10 minutes at room temperature. Samples were then incubated with 10 μg/mL Alexa Fluor 647-conjugated secondary antibody (Thermo Fisher, catalog No. A21445) diluted in PBS for 45 minutes at room temperature. The bacteria were then placed in a mounting medium containing propidium iodide (VWR, catalog No. 101098-046) and imaged by confocal microscopy (Leica sp8).
Affinity Binding
To quantify the affinity of mAb binding to the surface of A. baumannii, capsular surface extract was obtained as previously described [10, 15, 17, 18]. In brief, we grew an overnight culture of A. baumannii in tryptic soy broth (TSB), centrifuged it at 4000g for 5 minutes, and extracted capsular polysaccharide with ethylenediaminetetraacetic acid and then phenol.
Biolayer interferometry was conducted by Precision Antibody, Inc using an OctetRED96, per manufacturer's instructions (ForteBio, Inc). mAbs were tested at 30 μg/mL and captured using anti-human Fc capture dip and read biosensors. The probes were dipped into wells with capsule extract at 1:100 to measure association rates. To measure dissociation, the probes were dipped into assay buffer (1× kinetic buffer, PBS pH 7.4 with 0.002% Tween 20% and 0.01% BSA; ForteBio, Inc) and the dissociation rate (off rate) was measured. The KD (This is an equilibrium dissociation constant) was determined using 1:1 local fit analysis and graphically displayed (Fortebio software).
Ex Vivo Macrophage Opsonophagocytosis Assays
To evaluate opsonophagocytosis, we used ex vivo macrophages differentiated from human peripheral blood mononuclear cells (PBMC). We have shown that this quantifies phagocytosis/uptake of bacteria as opposed to surface binding [8]. Fresh PBMCs were acquired from human donors. Human PBMCs were obtained as anonymized samples purchased from a blood bank at the University of California, Los Angeles; human ethics authorization was not required for the use of this resource.
Monocytes were isolated using EasySep human monocyte isolation kit (STEMCELL) then differentiated to macrophages using ImmunoCult-SF Macrophage Medium (STEMCELL) per manufacture’s protocol. Briefly, monocytes were cultured 7 days with ImmunoCult-SF Macrophage Medium supplemented with 50 μg/mL recombinant human macrophage colony-stimulating factor and, 2 days before the assay, 50 ng/mL recombinant human interferon-γ (STEMCELL) was added. A. baumannii was added to the wells in the presence of Hanks’ balanced salt solution (HBSS) and 10% human AB serum (Innovative Research, Inc) with 20 μg/mL mAb or human IgG isotype and incubated for 1 hour at 37°C. For staining, macrophages were rinsed 3 times with HBSS, fixed with 100% methanol, and Hema-3 stained per manufacturer's protocol (Thermo Fisher Scientific).
Mouse Models of Infection
C3HeB/Fe mice (Jackson Labs) between the ages of 7 and 10 weeks were used for all experiments, as we have previously described for A. baumannii infection [13, 14, 19]. For the bloodstream infection model, mice were infected intravenously via the tail vein with designated inocula of A. baumannii isolates. An oropharyngeal model of aspiration pneumonia that recapitulates hospital- or ventilator-associated pneumonia (relevant to intensive care unit populations) was used. In brief, mice were sedated with isoflurane, hung by their maxillary incisors, tongues held to prevent swallowing, and 50 μL bacterial inocula was placed in the trachea to allow inoculation of the bacteria into the lungs by reflexive aspiration [20]. Treatments were administered in equimolar amounts based on bsAb dosing: HUMC1 infections 1.5 μg bsAb C73/mouse; for VA-Ab41 infections 5 μg bsAb C73/mouse; for HUMC9 infections 7 μg bsAb C73/mouse; for LAC-4 infections 7 μg bsAb C73/mouse.
All animal work was conducted following approval by the Institutional Animal Care and Use Committee (IACUC protocol numbers 20208 and 20750) at the University of Southern California, in compliance with the recommendations in the Guide for the Care and Use of Laboratory Animals of the National Institutes of Health.
Bacterial Inoculum Preparation
Bacteria were grown overnight in TSB at 37°C with shaking at 200 rpm. The bacteria were passaged to mid-log growth phase in TSB at 37°C with shaking at 200 rpm for 3 hours. Subcultures were rinsed 3 times with PBS and resuspended in PBS. To reduce variability between inocula, identical aliquots of PBS-suspended subcultures were stored frozen at −80°C, thawed for use when needed, and diluted with PBS to the appropriate concentration, as we have previously described [21]. During all in vivo experiments, the inoculum was delivered prior to treatment.
Statistics
Survival was compared by the nonparametric log-rank test. Bacteria per macrophage was compared with the Wilcoxon rank-sum test for unpaired comparisons. All statistics were run using STATA software version 17. Differences were considered significant if the P value was ≤.05.
RESULTS
Bispecific Antibody Strain Coverage Compared to Individual Monoclonal Antibodies
We compared mAb binding to more than 300 diverse clinical A. baumannii isolates that were acquired from multiple locations across the globe (Table 1 and Supplementary Table 1) [15]. Previously developed mAbs C8 and 65 [15, 16] bound to 27% of strains and 23% of strains, respectively. Of the 83 strains bound by mAb C8, more than half (59%) were bound only by mAb C8 and not by mAb 65. Similarly, of the 70 strains bound by mAb 65, more than half (51%) were bound only by mAb 65 and not by mAb C8. The bispecific antibody (BsAb) C73 bound to 36% of strains overall, including 8 strains to which the individual mAbs bound below the limit of detectability. There were also 14 strains bound minimally by the individual mAbs that were bound below the limit of detectability by bsAb C73.
Heat map of Acinetobacter baumannii strains bound by monoclonal antibody (mAb) C8, mAb 65, and bispecific mAb (BsAb) C73
![]() |
![]() |
The bispecific antibody bound similarly to the combined coverage of mAb C8 and mAb 65. More detailed data may be found in Supplementary Table 1.
Heat map of Acinetobacter baumannii strains bound by monoclonal antibody (mAb) C8, mAb 65, and bispecific mAb (BsAb) C73
![]() |
![]() |
The bispecific antibody bound similarly to the combined coverage of mAb C8 and mAb 65. More detailed data may be found in Supplementary Table 1.
Four hypervirulent and extremely drug-resistant representative A. baumannii strains were isolated for further analysis. Confocal microscopy confirmed diffuse surface binding of bsAb C73 to the surface of HUMC1, VA-Ab41, and HUMC9, but not LAC-4 (Figure 1), in a manner consistent with binding of mAbs C8 and 65, as previously demonstrated [15, 16]. By flow cytometry, HUMC1 was bound strongly by mAb C8 and bsAb C73; VA-Ab41 was bound significantly by mAb 65 and bsAb C73; HUMC9 was bound well by mAb C8, mAb 65, and bsAb C73; and LAC-4 was not bound appreciably by any antibody (Figure 2A and Table 1).

Confocal microscopy shows binding of bispecific monoclonal antibody (BsAb C73) to the surface of Acinetobacter baumannii. A. baumannii strains HUMC1, VA-Ab41, HUMC9, and LAC-4 were grown to log phase and incubated with primary antibody (isotype control or bsAb C73), followed by secondary antibody (Alexa Fluor 647-conjugated anti-human IgG heavy and light chain) to assess antibody binding (green) by confocal microscopy. Bacteria were counter-stained with propidium iodide (red).
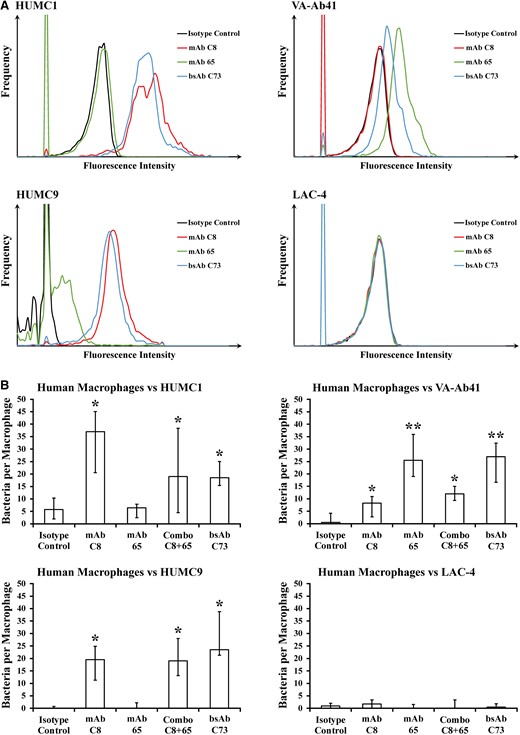
Bispecific monoclonal antibody (BsAb) C73 opsonizes Acinetobacter baumannii for macrophage uptake. A, By flow cytometry, monoclonal antibody (mAb) C8 and bsAb C73 were both able to bind HUMC1; mAb 65 and bsAb C73 were both able to bind VA-Ab41; mAb C8, mAb 65, and bsAb C73 were all able to bind HUMC9; and the antibodies were unable to bind LAC-4. B, Primary human macrophage cells were unable to appreciably uptake extremely drug-resistant hypervirulent A. baumannii strains in the absence of mAb. Addition of mAb C8, mAb 65, mAbs C8 and 65, or bsAb C73 helped opsonize bacteria and facilitate macrophage uptake, whereas the isotype control did not. A. baumannii strains opsonized by mAb C8, mAb 65, or both were also opsonized by bsAb C73. *P ≤ .05 versus isotype control group; **P ≤ .05 versus isotype control group, mAb C8 group, and Combo C8 + 65 group by the Wilcoxon rank-sum test for unpaired comparisons.
BsAb C73 Binds More Avidly Than mAb C8 and mAb 65
We used biolayer interferometry (ie, octet binding) to quantify mAb binding to surface extracts of 3 key bacterial target strains. mAb C8 had more specific binding to HUMC1 extract and mAb 65 and bsAb C73 had high binding to HUMC1 and VA-Ab41 extracts (Table 2). All 3 antibodies failed to bind to LAC-4 extract, which was the negative control.
Binding Affinity of Antibodies to Surface Extracts of Individual Acinetobacter baumannii Strains
A. baumannii Strain . | Binding Affinity, nm . | ||
---|---|---|---|
mAb C8 . | bsAb C73 . | mAb 65 . | |
HUMC1 | 0.77 | 0.77 | 0.51 |
VA-Ab41 | 0.39 | 0.70 | 0.81 |
A. baumannii Strain . | Binding Affinity, nm . | ||
---|---|---|---|
mAb C8 . | bsAb C73 . | mAb 65 . | |
HUMC1 | 0.77 | 0.77 | 0.51 |
VA-Ab41 | 0.39 | 0.70 | 0.81 |
Binding affinity was quantified by nanometer (nm) shift in octet binding signal compared to A. baumannii negative control strain, LAC-4. Higher nanometer shift indicates greater binding affinity.
Binding Affinity of Antibodies to Surface Extracts of Individual Acinetobacter baumannii Strains
A. baumannii Strain . | Binding Affinity, nm . | ||
---|---|---|---|
mAb C8 . | bsAb C73 . | mAb 65 . | |
HUMC1 | 0.77 | 0.77 | 0.51 |
VA-Ab41 | 0.39 | 0.70 | 0.81 |
A. baumannii Strain . | Binding Affinity, nm . | ||
---|---|---|---|
mAb C8 . | bsAb C73 . | mAb 65 . | |
HUMC1 | 0.77 | 0.77 | 0.51 |
VA-Ab41 | 0.39 | 0.70 | 0.81 |
Binding affinity was quantified by nanometer (nm) shift in octet binding signal compared to A. baumannii negative control strain, LAC-4. Higher nanometer shift indicates greater binding affinity.
Ex Vivo Opsonophagocytosis Assay
HUMC1, VA-Ab41, HUMC9, and LAC-4 are extremely drug-resistant A. baumannii strains, which cause lethal bacteremia in mice at a relatively low inoculum (ie, 2–8 × 107 CFU) [13]. Virulence is driven by escape of innate effector clearance, primarily phagocytosis, and cytokine production mediated by macrophages and neutrophils [12, 13, 22]. Hence, all 4 strains are resistant to phagocytosis without antibody opsonization.
We tested the ability of each antibody to opsonize these bacterial strains for human macrophage uptake ex vivo, which is a marker host capacity for opsonophagocytosis [13, 15, 22]. Concordant with the flow results of strain binding, mAb C8 statistically significantly improved macrophage uptake of HUMC1 and HUMC9 but not the other 2 strains (Figure 2B). mAb 65 significantly improved macrophage uptake of HUMC1 but not VA-Ab41 or HUMC9, despite not binding well to HUMC1 and binding well to HUMC9 by flow cytometry (Figure 2A and 2B). By contrast, bsAb C73 statistically significantly improved macrophage uptake of HUMC1, VA-Ab41, and HUMC9. The antibodies were unable to improve macrophage uptake of the negative control strain LAC-4.
In Vivo Efficacy of the Bispecific Antibody
We tested the efficacy of each antibody in our lethal bacteremia model in mice using the same 4 extremely drug-resistant strains of A. baumannii. Survival with antibody treatment (Figure 3) reflected opsonophagocytosis trends (Figure 2B). mAb C8 protected mice against HUMC1 and somewhat against HUMC9, but not VA-Ab41 or LAC-4 (Figure 3). mAb 65 protected mice against VA-Ab41, minimally against HUMC1, but not against HUMC9 or LAC-4. bsAb C73 provided protection against HUMC1, VA-Ab41, and HUMC9, but not the negative control LAC-4. bsAb C73 provided better or similar protection compared to the combination of mAb C8 and mAb 65 in the 3 strains HUMC1, VA-Ab41, and HUMC9.
![Bispecific monoclonal antibody (bsAb) C73 rescues C3HeB/Fe mice with Acinetobacter baumannii bacteremia. Mice were infected intravenously (IV) via the tail vein with hypervirulent extremely drug-resistant A. baumannii and treated with antibody (isotype control, monoclonal antibody [mAb] C8, mAb 65, Combo C8 + 65, or bsAb C73) at equimolar amounts. A, Mice were infected with HUMC1 and treated with 1.5 μg bsAb C73 or molar equivalent of another antibody. All survived when treated with bsAb C73 or mAb C8, most survived when treated with Combo C8 + 65, few survived when treated with mAb 65, and none survived when treated with isotype control. B, Mice were infected with VA-Ab41 and treated with 5 μg bsAb C73 or molar equivalent of another antibody. All survived when treated with bsAb C73, most survived when treated with Combo C8 + 65 or mAb 65, few survived when treated with isotype control, and none survived when treated with mAb C8. C, Mice were infected with HUMC9 and treated with 7 μg bsAb C73 or molar equivalent of another antibody. Half survived when treated with bsAb C73, one-third survived when treated with Combo C8 + 65 or mAb C8, and none survived when treated with mAb 65 or isotype control. D, Mice were infected with LAC-4 and treated with 7 μg bsAb C73 or molar equivalent of another antibody. No mice survived, regardless of what treatment they received. n = 4–10 mice/group/experiment over 2–3 experiments; *P ≤ .05 versus isotype control group by the nonparametric log-rank test.](https://oup.silverchair-cdn.com/oup/backfile/Content_public/Journal/jid/227/9/10.1093_infdis_jiac499/2/m_jiac499f3.jpeg?Expires=1750338541&Signature=2dSOAOQ892rt0RA258mw~ik2lcDStDpJEJuIil428Bfz76FP8K-3HwF1O8rY5Sf~jiqlWLGetwwyJIVgJY8jm7NdJaBG0z5YEapS7OZdLt6t5ndyecsS0PZPQKg9AJoQDUvzEi2hMTC9DMMBb1WKXV8Yd9tERKjb9t-3tBheARzJsFLPp14fwsbouDLqScH-VhceLIB8lxyGxDJlyD163G8YdYenRis6e8Y9oStsqpkRekBl1aLft2I0dUxzubDWEq9uovAAZx7xNN8KMHyLvoISu9A-6gIGL2p29cgM-TeY88OKSfQtvtMvcrNWGOE~IGyBI97F~D456mooTnjAEg__&Key-Pair-Id=APKAIE5G5CRDK6RD3PGA)
Bispecific monoclonal antibody (bsAb) C73 rescues C3HeB/Fe mice with Acinetobacter baumannii bacteremia. Mice were infected intravenously (IV) via the tail vein with hypervirulent extremely drug-resistant A. baumannii and treated with antibody (isotype control, monoclonal antibody [mAb] C8, mAb 65, Combo C8 + 65, or bsAb C73) at equimolar amounts. A, Mice were infected with HUMC1 and treated with 1.5 μg bsAb C73 or molar equivalent of another antibody. All survived when treated with bsAb C73 or mAb C8, most survived when treated with Combo C8 + 65, few survived when treated with mAb 65, and none survived when treated with isotype control. B, Mice were infected with VA-Ab41 and treated with 5 μg bsAb C73 or molar equivalent of another antibody. All survived when treated with bsAb C73, most survived when treated with Combo C8 + 65 or mAb 65, few survived when treated with isotype control, and none survived when treated with mAb C8. C, Mice were infected with HUMC9 and treated with 7 μg bsAb C73 or molar equivalent of another antibody. Half survived when treated with bsAb C73, one-third survived when treated with Combo C8 + 65 or mAb C8, and none survived when treated with mAb 65 or isotype control. D, Mice were infected with LAC-4 and treated with 7 μg bsAb C73 or molar equivalent of another antibody. No mice survived, regardless of what treatment they received. n = 4–10 mice/group/experiment over 2–3 experiments; *P ≤ .05 versus isotype control group by the nonparametric log-rank test.
In the setting of subtherapeutic dosing, bsAb C73 worked synergistically with the antibiotic colistin to rescue mice from otherwise lethal bacteremia (Figure 4A). Whereas neither group of mice receiving either monotherapy survived, the entire group receiving combined therapy (BsAb C73 plus colistin) survived. Even when mice received delayed treatment by 1 hour, bsAb C73 still provided full protection (Figure 4B). Finally, mice that were infected in an oropharyngeal aspiration model that emulates aspiration pneumonia were better able to survive infection when treated with bsAb C73 compared with mice treated with isotype control antibody (Figure 4C).
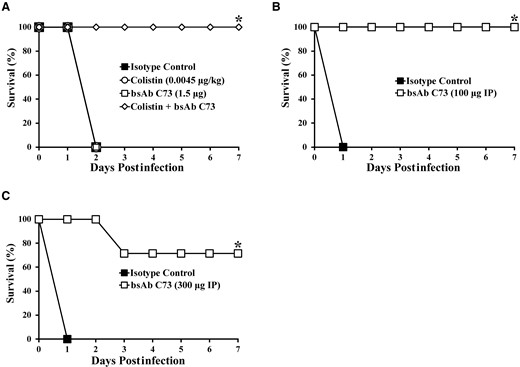
Bispecific monoclonal antibody (bsAb) C73 works synergistically with colistin, in the setting of delayed treatment, and against pneumonia. A, Combined with colistin (0.0045 mg/kg), a low dose of bsAb C73 (1.5 μg) was able to rescue all C3HeB/Fe mice from lethal bacteremia that both monotherapies failed to treat. B, Delayed intraperitoneal (IP) treatment with bsAb C73 (100 μg) was also capable of rescuing all mice from lethal bacteremia. C, bsAb C73 (300 μg) rescued most mice in an otherwise lethal aspiration pneumonia infection model. n = 5 mice/group/experiment over 2 experiments; *P ≤ .05 versus isotype control group by nonparametric log-rank test.
DISCUSSION
Given its high frequency of antibiotic resistance, A. baumannii is a priority target for new therapeutic development [11]. Its high rate of emerging antibiotic resistance and the relative paucity of new antibiotics in the pipeline targeting A. baumannii make the organism a prime candidate for novel immunotherapies [4]. We have identified and fully humanized 2 mAbs that bind to A. baumannii capsule, opsonize the organism for phagocytosis, and are highly protective against lethal infection in vivo [15, 16]. This protection is conferred by immunomodulation as much as opsonophagocytosis [23]. Combining the complementary determining regions of these 2 monoclonal antibodies into a bispecific antibody combined their clinical efficacy, thereby broadening the strain coverage available in one antibody. Minimizing the number of molecules is critical to enabling technology development, as regulatory agencies may require each individual component of a mixture of components to be studied in separate arms, in addition to the combined mixture, during preclinical toxicity studies and clinical trials.
We found that the resultant bispecific antibody binds to a broader array of strains than either mAb alone, with no loss of binding avidity, enabling a single bispecific antibody to replace both monoclonal antibodies. The bispecific antibody was also capable of better enhancing opsonophagocytosis than either mAb alone. Moreover, the bispecific antibody was synergistic in combination with an antibiotic and in the setting of delayed therapy. Finally, the bispecific mAb provided considerable protection in a model of lethal aspiration pneumonia.
In summary, a bispecific humanized antibody is now available that binds 36% of >300 diverse clinical A. baumannii isolates from around the world and provides a high degree of protection against bacteremia and pneumonia in mice. The results of our strain binding analyses suggest that additional antibodies will be needed to provide adequate clinical strain coverage for empiric use. However, the binding affinity and in vivo efficacy retained by the bsAb suggests that one additional bsAb may be adequate to enable a clinically deployable therapeutic.
Supplementary Data
Supplementary materials are available at The Journal of Infectious Diseases online. Consisting of data provided by the authors to benefit the reader, the posted materials are not copyedited and are the sole responsibility of the authors, so questions or comments should be addressed to the corresponding author.
Notes
Financial support. This work was supported by the National Institute of Allergy and Infectious Diseases at the National Institutes of Health (grant numbers R01 AI130060, R01 AI117211, R21 AI127954, and R42 AI106375 to B. S.; R01AI139052 to B. L.; and 5P30 AI028697 to University of California Los Angeles, Virology Core Laboratory).
References
Author notes
Potential conflicts of interest. T. B. N. and B. S. are inventors on patents related to monoclonal antibodies C8 and 65. T. B. N., J. Y., B. M. L., and B. S. are inventors on a patent related to bispecific monoclonal antibody C73. T. B. N. and B. S. own equity in BioAIM, which is developing the antibodies. U. S. C. has an ownership interest in BioAIM and is entitled to a share of royalties based on a licensing agreement with BioAIM. All other authors report no potential conflicts.
All authors have submitted the ICMJE Form for Disclosure of Potential Conflicts of Interest. Conflicts that the editors consider relevant to the content of the manuscript have been disclosed.