-
PDF
- Split View
-
Views
-
Cite
Cite
Marta Martínez-Guitián, Juan C Vázquez-Ucha, Laura Álvarez-Fraga, Kelly Conde-Pérez, Juan A Vallejo, Alejandra Perina, Germán Bou, Margarita Poza, Alejandro Beceiro, Global Transcriptomic Analysis During Murine Pneumonia Infection Reveals New Virulence Factors in Acinetobacter baumannii, The Journal of Infectious Diseases, Volume 223, Issue 8, 15 April 2021, Pages 1356–1366, https://doi.org/10.1093/infdis/jiaa522
- Share Icon Share
Abstract
Infections caused by multidrug-resistant pathogens such as Acinetobacter baumannii constitute a major health problem worldwide. In this study we present a global in vivo transcriptomic analysis of A. baumannii isolated from the lungs of mice with pneumonia infection.
Mice were infected with A. baumannii ATCC 17978 and AbH12O-A2 strains and the total bacterial RNA were analyzed by RNA sequencing. Lists of differentially expressed genes were obtained and 14 of them were selected for gene deletion and further analysis.
Transcriptomic analysis revealed a specific gene expression profile in A. baumannii during lung infection with upregulation of genes involved in iron acquisition and host invasion. Mutant strains lacking feoA, mtnN, yfgC, basB, hisF, oatA, and bfnL showed a significant loss of virulence in murine pneumonia. A decrease in biofilm formation, adherence to human epithelial cells, and growth rate was observed in selected mutants.
This study provides an insight into A. baumannii gene expression profile during murine pneumonia infection. Data revealed that 7 in vivo upregulated genes were involved in virulence and could be considered new therapeutic targets.
Acinetobacter baumannii is considered to be one of the most critical priority pathogens [1]. Its high genetic plasticity and ability to survive in multiple environments, in addition to its capacity to produce a wide range of virulence factors, allows this microorganism to generate multiresistant strains and outbreaks [2–6]. Currently, there is an urgent need to search for novel targets for use in designing new therapies to combat infections caused by this pathogen.
Recent advances in genomics have led to an improved understanding of the mechanisms involved in the pathogenesis of A. baumannii [7, 8]. Transcriptomic and proteomic analyses have been performed using in vitro models, revealing different gene expression or proteomic profiles in planktonic and sessile cells associated with A. baumannii biofilms [9, 10]. Similarly, transcriptomic analysis has been used to identify A. baumannii genes involved in iron uptake [11]. In vivo transcriptome studies of bacterial RNA samples extracted directly from infected tissues provide important information about the biological processes that occur during pathogenesis. The A. baumannii transcriptome was determined in samples obtained from mice with bacteremia and potential therapeutic targets were detected [12]. Insertion sequencing has also been used with transposon mutagenesis and next-generation sequencing to identify novel virulence factors involved in the persistence of A. baumannii in the lung [13]. Despite massive research efforts, the pathogenicity of this microorganism remains unclear and more in-depth studies are needed.
In the present study, we analyzed the global transcriptomic response of A. baumannii ATCC 17978 and AbH12O-A2 strains to murine pneumonia infection. This study provided insight into the mechanisms behind the development of infection and allowed us to identify potential novel therapeutic targets.
METHODS
Bacterial Strains and Growth Media
A. baumannii ATCC 17978 reference strain and AbH12O-A2 clinical strain were used. AbH12O-A2 strain is a highly invasive multidrug-resistant clinical isolate that triggered the largest outbreak of A. baumannii known worldwide and has been well characterized by our group [8, 14, 15]. Escherichia coli TG1 was used for cloning procedures. Bacteria were routinely grown at 37°C in Luria-Bertani (LB) medium, supplemented with agar or antibiotic when needed.
Murine Pneumonia Infection Model
An experimental pneumonia model was used to obtain transcriptomes of A. baumannii ATCC 17978 and AbH12O-A2 strains during the course of the infection, as described before [16] using BALB/c mice, previously used for similar approaches. BALB/c mice were selected in this study, which are widely used in experimental murine pneumonia models [17]. Male mice were intratracheally inoculated with approximately 5.5 × 107 colony forming units/mouse of exponentially grown cells of ATCC 17978 and the AbH12O-A2 strains. Mice were euthanized with an overdose of sodium thiopental (Sandoz) 20 hours after inoculation (death 30 hours after inoculation). Bronchoalveolar lavage was immediately performed to yield bacteria suitable for RNA extraction (in vivo samples). RNA expressed from bacteria grown in LB flasks (OD600 = 1.0) at 37°C and 180 rpm were isolated and considered in vitro samples. Three biological replicates were conducted in each condition.
This murine model was also used to compare virulence of the isogenic derivative mutants and the parental strain; groups of 10–12 mice were infected and observed during 140 hours at 8-hour intervals.
All experiments were carried out with the approval of and in accordance with the regulatory guidelines and standards established by the Animal Ethics Committee (Hospital Universitario A Coruña, Spain, project code P2015/82).
Bacterial RNA Purification
Total RNA was extracted from both in vivo and in vitro samples with the RNeasy Mini Kit (Qiagen), treated with DNAse I (Invitrogen), and purified with the RNeasy MinElute Cleanup Kit (Qiagen). The concentration and purity of the samples were determined using a BioDrop μLITE system (Isogen Life Science) and a 2100 Bioanalyzer (Agilent Technologies).
RNAseq Sequencing and Bioinformatic Analyses of Gene Expression Profiles
Reads from mRNA libraries were obtained using HiScanSQ (Illumina). Reads with an average length of 50 nucleotides were aligned with the complete genome of A. baumannii ATCC 17978 (GenBank numbers, CP000521.1, CP5000522.1, and CP000523.1) and A. baumannii AbH12O-A2 (CP009534.1). The gene expression profiles obtained were compared at the Center for Cooperative Research in Biosciences (CIC bioGUNE, Bilbao, Spain). The sequences were functionally annotated with Sma3s version 2 software [18]. The annotated matrix of gene expression analyses was imported into R version 3.5.2. The R packages ggplot2 version 3.1.0 [19], plotly version 4.8.0 [20], and ggrepel version 0.8.0 [21] were used to plot the results of the analyses. Cluster of orthologous groups (COG) enrichment analysis was performed dividing the percentage of genes up- or downregulated for each category by the percentage of genes in that category across the whole genome [12].
Quantitative RT-PCR
The expression level determination of the target genes was performed as previously described [22]. The oligonucleotides and probes used are detailed in Supplementary Table 1.
Construction of Isogenic Derivative Mutants
All mutant derivative strains were constructed using the suicide vector pMo130 as previously described [9, 22]. Briefly, upstream and downstream regions flanking the selected genes were polymerase chain reaction (PCR)-amplified and cloned into the pMo130 vector. The construction was then used to transform the ATCC 17978 cells by electroporation. Recombinant colonies representing the first crossover event were selected and the second crossover event leading to the gene knockout strain was confirmed by PCR and sequencing. The oligonucleotides used are listed in Supplementary Table 1.
Adhesion to Epithelial Cells
Adherence to A549 human alveolar epithelial cells assays were performed as previously described [16, 22]. Three biological replicates were performed.
Quantitative Determination of Biofilm
Biofilm formation ability was determined following previously established protocols [22]. Three biological replicates were performed.
RESULTS
Global Transcriptomic Changes of A. baumannii During Pneumonia Infection
Different gene expression profiles were obtained from A. baumannii ATCC 17978 and AbH12O-A2 cells grown during lung infection (in vivo) relative to cells grown in flasks (in vitro). Raw RNA sequencing (RNAseq) expression data were deposited in the GEO database under accession code GSE100552. A total of 350 and 628 genes were found differentially expressed in A. baumannii ATCC 17978 and AbH12O-A2, respectively, considering 2 cutoff values, a log2-fold change of more than +1 (upregulated genes) or less than −1 (downregulated genes) and an adjusted P value < .05 (Figure 1). Among them, 224 were identified as upregulated genes and 126 as downregulated genes in ATCC 17978 strain (Figure 1A and Supplementary Table 2). In AbH12O-A2 strain, 347 and 279 genes were found to be upregulated and downregulated, respectively (Figure 1B and Supplementary Table 3). Venn diagrams showed that 77 upregulated genes and 38 downregulated genes were found in both ATCC 17978 and AbH12O-A2 strains (Supplementary Figure 1 and Supplementary Table 4).
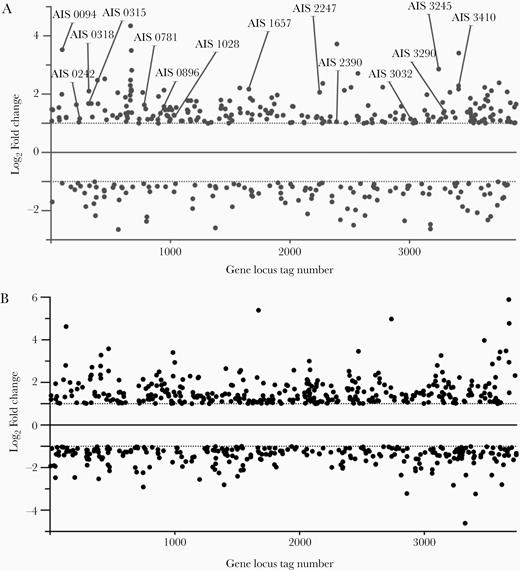
Dispersion plot of genes differentially expressed in (A) ATCC 17978 and (B) AbH12O-A2 strains during lung infection in the murine model. The 14 genes selected for gene deletion in the reference strain ATCC 17978 are indicated.
Illumina results were validated by quantitative reverse transcription PCR (qRT-PCR) on random sample of the genes found to be upregulated during pneumonia infection (Supplementary Figure 2). High Pearson correlation coefficients between RNAseq and qRT-PCR expression data were obtained in both ATCC 17978 and AbH12O-A2 strains (R2 = 0.6794, P = .02 and R2 = 0.8390, P = .004, respectively).
To compare the gene expression pattern between in vitro and in vivo conditions, a functional study was performed. The gene selection for this analysis was based on their up- and downregulation in both strains (Supplementary Table 4). This allowed us to reduce the number of genes to those that were implicitly involved in development of the pneumonia infection in A. baumannii. COG enrichment analysis showed that upregulated genes in both strains were involved in cell division (D), defense mechanisms (V), carbohydrate transport and metabolism (G), and secondary metabolites biosynthesis, transport, and catabolism (Q) (Figure 2). In contrast, genes related to translation (J) and posttranslational modifications (O) were found to be downregulated (Figure 2). Individual COG analysis of each strain showed that, despite only sharing 77 and 35 up- and downregulated genes, their expression patterns during the pneumonia infection were similar (Supplementary Figure 3). These groups of up- and downregulated genes common to both strains were further classified according to their molecular functions, cellular location, and the biological process in which they are involved (Supplementary Figure 4).
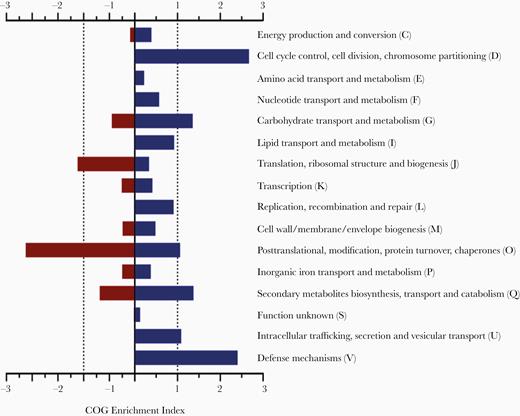
Cluster of orthologous groups (COG) enrichment for differentially regulated genes in Acinetobacter baumannii ATCC 17978 and AbH12O-A2 strains. Positive values refer in vivo upregulated genes when compared with in vitro conditions. Negative values refer to downregulated genes.
Changes in the Expression of Virulence Factors During Pneumonia Infection
A number of genes belonging to the baumanoferrin cluster (bnfA-L, A1S_1647-57), the acinetobactin cluster (A1S_2372-92), and the ferrous iron transport cluster (feoABC, A1S_0242-44) were found to be upregulated in vivo in both strains. Some genes among the fimsbactin siderophore cluster (fbsA-O, A1S_2562-81) were also upregulated in ATCC 17978 but this cluster was not present in the AbH12O-A2 strain. A1S_0452 and A1S_3032 genes, coding for TonB-like proteins, were also found to be overexpressed. Expression levels of the genes are displayed in Supplementary Tables 2, 3, and 4.
Among the genes involved in the lipopolysaccharide and capsule production, only the lpxB (A1S_1668) and the pgm (A1S_0066) genes were overexpressed in vivo. In addition, the capsule repressor BfmR (A1S_0748) was found to be downregulated in both strains; however, it was below the cutoff values in ATCC 17978.
Genes related to the secretion systems T4SS (A1S_0234, A1S_1559, and A1S_0896) and the Ata autotransporter (A1S_1032-33) were also upregulated.
Both strains increased the expression of several efflux pumps genes under in vivo conditions. Among them, only 2 genes (A1S_0007 and A1S_008) were found in both strains.
The ompA (A1S_2840) and omp33-36 (A1S_3297) porin genes were downregulated in ATCC 17978 strain while only ompA was found to be downregulated in AbH12O-A2. The CsuA/B (A1S_2218) major subunit of the type I pili assembly system involved in adhesion to abiotic surfaces was downregulated in ATCC 17978 but no differences were found in AbH12O-A2.
Specific A. baumannii Genes Are Involved in Development of Lung Infection in Mice
The global transcriptomic analysis was followed by an individual bibliographic study of the 224 (ATCC 17978) and 350 (AbH12O-A2) in vivo upregulated genes, allowing us to identify a collection of genes previously related to bacterial pathogenesis. Fourteen genes were selected for gene deletion in ATCC 17978 reference strain and further analysis. The criteria for selection of these genes was their predicted function related to pathogenesis and their upregulation during the pneumonia infection (Figure 1 and Table 1).
Isogenic Derivative Mutants of Acinetobacter baumannii ATCC 17978 Strain, Gene Names and Function of Product, Upregulated Expression During Lung Infection in ATCC 17978, and Previous Studies on the Implication of the Genes in Bacterial Virulence
A. baumannii Mutants . | Gene Description . | Log2 Fold Change (P Value) in ATCC 17978 . | References . |
---|---|---|---|
Δ0242 | feoA, ferrous iron transport protein A | 1.16 (.0023) | [23] |
Δ0781 | mtnN, (MTA/SAH) nucleosidase | 1.63 (9.04 × 10−9) | [24, 25] |
Δ1657 | bnfL, N-acetyltransferase, baumanoferrin biosynthesis protein | 2.17 (5.06 × 10−08) | [11, 26] |
Δ2247 | yfgC (bepA) putative periplasmic metalloprotease | 2.06 (5.52 × 10−05) | [27] |
Δ2390 | basB, acinetobactin biosynthesis protein | 1.05 (.008) | [28, 29] |
Δ3245 | hisF, imidazole glycerol phosphate synthase cyclase subunit | 2.86 (4.14 × 10−18) | [30] |
Δ3410 | oatA, acyltransferase | 2.29 (1.20 × 10−07) | [31, 32] |
Δ0094 | lrp regulon transcriptional regulator | 3.53 (1.10 × 10−17) | [33] |
Δ0315 | DNA repair system (2-oxoglutarate-Fe (II)-oxygenase-like) | 1.68 (.00022) | [34] |
Δ0318 | FusE-MFP/HlyD membrane fusion protein | 2.10 (1.57 × 10−07) | [35] |
Δ0896 | pilU, twitching motility | 1.13 (.00635) | [36] |
Δ1028 | ligB, aromatic ring-opening dioxygenase | 1.29 (.00326) | [37] |
Δ3032 | tonB-like, biological transducer activity | 1.01 (3.63 × 10−05) | [38] |
Δ3290 | esvF2, thiaminase (transcriptional activator TenA) | 1.42 (.00034) | [39, 40] |
A. baumannii Mutants . | Gene Description . | Log2 Fold Change (P Value) in ATCC 17978 . | References . |
---|---|---|---|
Δ0242 | feoA, ferrous iron transport protein A | 1.16 (.0023) | [23] |
Δ0781 | mtnN, (MTA/SAH) nucleosidase | 1.63 (9.04 × 10−9) | [24, 25] |
Δ1657 | bnfL, N-acetyltransferase, baumanoferrin biosynthesis protein | 2.17 (5.06 × 10−08) | [11, 26] |
Δ2247 | yfgC (bepA) putative periplasmic metalloprotease | 2.06 (5.52 × 10−05) | [27] |
Δ2390 | basB, acinetobactin biosynthesis protein | 1.05 (.008) | [28, 29] |
Δ3245 | hisF, imidazole glycerol phosphate synthase cyclase subunit | 2.86 (4.14 × 10−18) | [30] |
Δ3410 | oatA, acyltransferase | 2.29 (1.20 × 10−07) | [31, 32] |
Δ0094 | lrp regulon transcriptional regulator | 3.53 (1.10 × 10−17) | [33] |
Δ0315 | DNA repair system (2-oxoglutarate-Fe (II)-oxygenase-like) | 1.68 (.00022) | [34] |
Δ0318 | FusE-MFP/HlyD membrane fusion protein | 2.10 (1.57 × 10−07) | [35] |
Δ0896 | pilU, twitching motility | 1.13 (.00635) | [36] |
Δ1028 | ligB, aromatic ring-opening dioxygenase | 1.29 (.00326) | [37] |
Δ3032 | tonB-like, biological transducer activity | 1.01 (3.63 × 10−05) | [38] |
Δ3290 | esvF2, thiaminase (transcriptional activator TenA) | 1.42 (.00034) | [39, 40] |
Isogenic Derivative Mutants of Acinetobacter baumannii ATCC 17978 Strain, Gene Names and Function of Product, Upregulated Expression During Lung Infection in ATCC 17978, and Previous Studies on the Implication of the Genes in Bacterial Virulence
A. baumannii Mutants . | Gene Description . | Log2 Fold Change (P Value) in ATCC 17978 . | References . |
---|---|---|---|
Δ0242 | feoA, ferrous iron transport protein A | 1.16 (.0023) | [23] |
Δ0781 | mtnN, (MTA/SAH) nucleosidase | 1.63 (9.04 × 10−9) | [24, 25] |
Δ1657 | bnfL, N-acetyltransferase, baumanoferrin biosynthesis protein | 2.17 (5.06 × 10−08) | [11, 26] |
Δ2247 | yfgC (bepA) putative periplasmic metalloprotease | 2.06 (5.52 × 10−05) | [27] |
Δ2390 | basB, acinetobactin biosynthesis protein | 1.05 (.008) | [28, 29] |
Δ3245 | hisF, imidazole glycerol phosphate synthase cyclase subunit | 2.86 (4.14 × 10−18) | [30] |
Δ3410 | oatA, acyltransferase | 2.29 (1.20 × 10−07) | [31, 32] |
Δ0094 | lrp regulon transcriptional regulator | 3.53 (1.10 × 10−17) | [33] |
Δ0315 | DNA repair system (2-oxoglutarate-Fe (II)-oxygenase-like) | 1.68 (.00022) | [34] |
Δ0318 | FusE-MFP/HlyD membrane fusion protein | 2.10 (1.57 × 10−07) | [35] |
Δ0896 | pilU, twitching motility | 1.13 (.00635) | [36] |
Δ1028 | ligB, aromatic ring-opening dioxygenase | 1.29 (.00326) | [37] |
Δ3032 | tonB-like, biological transducer activity | 1.01 (3.63 × 10−05) | [38] |
Δ3290 | esvF2, thiaminase (transcriptional activator TenA) | 1.42 (.00034) | [39, 40] |
A. baumannii Mutants . | Gene Description . | Log2 Fold Change (P Value) in ATCC 17978 . | References . |
---|---|---|---|
Δ0242 | feoA, ferrous iron transport protein A | 1.16 (.0023) | [23] |
Δ0781 | mtnN, (MTA/SAH) nucleosidase | 1.63 (9.04 × 10−9) | [24, 25] |
Δ1657 | bnfL, N-acetyltransferase, baumanoferrin biosynthesis protein | 2.17 (5.06 × 10−08) | [11, 26] |
Δ2247 | yfgC (bepA) putative periplasmic metalloprotease | 2.06 (5.52 × 10−05) | [27] |
Δ2390 | basB, acinetobactin biosynthesis protein | 1.05 (.008) | [28, 29] |
Δ3245 | hisF, imidazole glycerol phosphate synthase cyclase subunit | 2.86 (4.14 × 10−18) | [30] |
Δ3410 | oatA, acyltransferase | 2.29 (1.20 × 10−07) | [31, 32] |
Δ0094 | lrp regulon transcriptional regulator | 3.53 (1.10 × 10−17) | [33] |
Δ0315 | DNA repair system (2-oxoglutarate-Fe (II)-oxygenase-like) | 1.68 (.00022) | [34] |
Δ0318 | FusE-MFP/HlyD membrane fusion protein | 2.10 (1.57 × 10−07) | [35] |
Δ0896 | pilU, twitching motility | 1.13 (.00635) | [36] |
Δ1028 | ligB, aromatic ring-opening dioxygenase | 1.29 (.00326) | [37] |
Δ3032 | tonB-like, biological transducer activity | 1.01 (3.63 × 10−05) | [38] |
Δ3290 | esvF2, thiaminase (transcriptional activator TenA) | 1.42 (.00034) | [39, 40] |
A pneumonia mice model was performed with the 14 mutant derivative strains. The survival rates of mice infected with the mutant strains Δ0242 (60%), Δ0781 (18%), Δ1657 (20%), Δ2247 (20%), Δ2390 (33%), Δ3245 (75%), and Δ3410 (30%) were significantly higher than those of mice infected with the parental strain (0% survival rate, P < .02) (Figure 3 and Table 2). No significant differences in mice survival were observed in the other isogenic mutant strains (P = .06–.2).
Mutant Strains . | Gene . | Mice Survival During Pneumonia . | Biofilm Formation . | Attachment . | Fitness . | Fitness in Iron-Limiting Conditions . | Increase Antibiotic Susceptibility . |
---|---|---|---|---|---|---|---|
Virulence loss in pneumonia | |||||||
Δ0242 | feoA | ++ | ++ | +++ | − | ++ | − |
Δ0781 | mtnN | + | + | − | − | − | − |
Δ1657 | bnfL, | + | ++ | +++ | − | + | − |
Δ2247 | yfgC | + | − | − | + | − | + |
Δ2390 | basB | + | − | +++ | − | +++ | − |
Δ3245 | hisF | +++ | − | − | − | − | − |
Δ3410 | oatA | + | − | − | − | − | − |
No virulence loss in pneumonia | |||||||
Δ0094 | lrp | − | − | − | − | − | − |
Δ0315 | Oxygenase-like | − | − | ++ | − | − | − |
Δ0318 | fusE-MFP/hylD | − | − | − | − | − | − |
Δ0896 | pilU | − | − | − | − | − | − |
Δ1028 | ligB | − | − | − | − | − | − |
Δ3032 | tonB-like | − | − | ++ | − | − | − |
Δ3290 | esvF2 | − | +++ a | ++ | − | − | − |
Mutant Strains . | Gene . | Mice Survival During Pneumonia . | Biofilm Formation . | Attachment . | Fitness . | Fitness in Iron-Limiting Conditions . | Increase Antibiotic Susceptibility . |
---|---|---|---|---|---|---|---|
Virulence loss in pneumonia | |||||||
Δ0242 | feoA | ++ | ++ | +++ | − | ++ | − |
Δ0781 | mtnN | + | + | − | − | − | − |
Δ1657 | bnfL, | + | ++ | +++ | − | + | − |
Δ2247 | yfgC | + | − | − | + | − | + |
Δ2390 | basB | + | − | +++ | − | +++ | − |
Δ3245 | hisF | +++ | − | − | − | − | − |
Δ3410 | oatA | + | − | − | − | − | − |
No virulence loss in pneumonia | |||||||
Δ0094 | lrp | − | − | − | − | − | − |
Δ0315 | Oxygenase-like | − | − | ++ | − | − | − |
Δ0318 | fusE-MFP/hylD | − | − | − | − | − | − |
Δ0896 | pilU | − | − | − | − | − | − |
Δ1028 | ligB | − | − | − | − | − | − |
Δ3032 | tonB-like | − | − | ++ | − | − | − |
Δ3290 | esvF2 | − | +++ a | ++ | − | − | − |
Differences in bacterial phenotypes observed in the mutant strains compared with the parental ATCC 17978 strain are indicated as follows: +, 20%–49%; ++, 50%–74%; +++, 75%–100%; −, no difference.
Statistical analyses were performed using unpaired student t tests comparing each mutant with the ATCC 17978 wild type strain. Only differences statistically significant (P < .05) are shown.
aMutants improved the phenotype displayed by the parental strain.
Mutant Strains . | Gene . | Mice Survival During Pneumonia . | Biofilm Formation . | Attachment . | Fitness . | Fitness in Iron-Limiting Conditions . | Increase Antibiotic Susceptibility . |
---|---|---|---|---|---|---|---|
Virulence loss in pneumonia | |||||||
Δ0242 | feoA | ++ | ++ | +++ | − | ++ | − |
Δ0781 | mtnN | + | + | − | − | − | − |
Δ1657 | bnfL, | + | ++ | +++ | − | + | − |
Δ2247 | yfgC | + | − | − | + | − | + |
Δ2390 | basB | + | − | +++ | − | +++ | − |
Δ3245 | hisF | +++ | − | − | − | − | − |
Δ3410 | oatA | + | − | − | − | − | − |
No virulence loss in pneumonia | |||||||
Δ0094 | lrp | − | − | − | − | − | − |
Δ0315 | Oxygenase-like | − | − | ++ | − | − | − |
Δ0318 | fusE-MFP/hylD | − | − | − | − | − | − |
Δ0896 | pilU | − | − | − | − | − | − |
Δ1028 | ligB | − | − | − | − | − | − |
Δ3032 | tonB-like | − | − | ++ | − | − | − |
Δ3290 | esvF2 | − | +++ a | ++ | − | − | − |
Mutant Strains . | Gene . | Mice Survival During Pneumonia . | Biofilm Formation . | Attachment . | Fitness . | Fitness in Iron-Limiting Conditions . | Increase Antibiotic Susceptibility . |
---|---|---|---|---|---|---|---|
Virulence loss in pneumonia | |||||||
Δ0242 | feoA | ++ | ++ | +++ | − | ++ | − |
Δ0781 | mtnN | + | + | − | − | − | − |
Δ1657 | bnfL, | + | ++ | +++ | − | + | − |
Δ2247 | yfgC | + | − | − | + | − | + |
Δ2390 | basB | + | − | +++ | − | +++ | − |
Δ3245 | hisF | +++ | − | − | − | − | − |
Δ3410 | oatA | + | − | − | − | − | − |
No virulence loss in pneumonia | |||||||
Δ0094 | lrp | − | − | − | − | − | − |
Δ0315 | Oxygenase-like | − | − | ++ | − | − | − |
Δ0318 | fusE-MFP/hylD | − | − | − | − | − | − |
Δ0896 | pilU | − | − | − | − | − | − |
Δ1028 | ligB | − | − | − | − | − | − |
Δ3032 | tonB-like | − | − | ++ | − | − | − |
Δ3290 | esvF2 | − | +++ a | ++ | − | − | − |
Differences in bacterial phenotypes observed in the mutant strains compared with the parental ATCC 17978 strain are indicated as follows: +, 20%–49%; ++, 50%–74%; +++, 75%–100%; −, no difference.
Statistical analyses were performed using unpaired student t tests comparing each mutant with the ATCC 17978 wild type strain. Only differences statistically significant (P < .05) are shown.
aMutants improved the phenotype displayed by the parental strain.
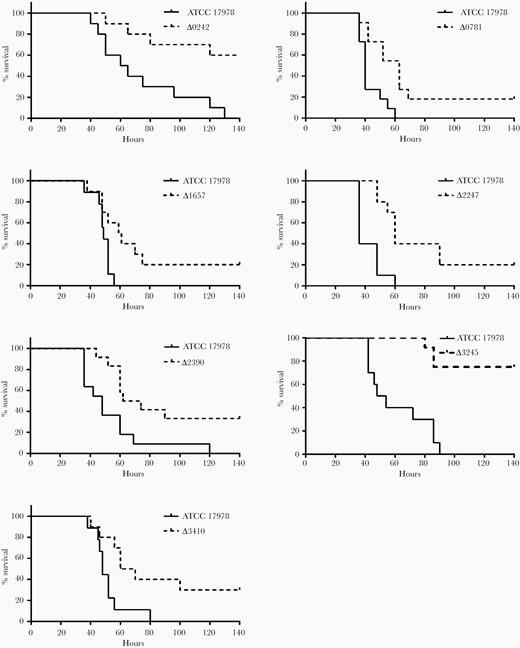
Pneumonia infection in mice. Survival of BALB/c mice after pneumonia infection with Acinetobacter baumannii ATCC 17978 and the isogenic mutant derivative strains Δ0781 (n = 11, P = .0021), Δ0242 (n = 10, P = .0027), Δ1657 (n = 10, P = .0159), Δ2247 (n = 10, P = .0007), Δ2390 (n = 11, P = .007), Δ3245 (n = 11, P < .0001), and Δ3410 (n = 10, P = .013). The log-rank (Mantel-Cox) test was used for statistical analysis.
Effect of Gene Deletion on Bacterial Growth
Growth curves were performed, and the growth rate and replication ability were evaluated. Among all mutants, only the Δ2247 strain showed a lower growth rate, with a longer mean generation time (29 minutes, µ = 0.023) than the parental strain (24 minutes, μ = 0.027), resulting in a significant reduction in fitness (P = .004) (Figure 4A and Table 2). No diminished bacterial growth was observed in any of the other mutants tested (Supplementary Figure 5).
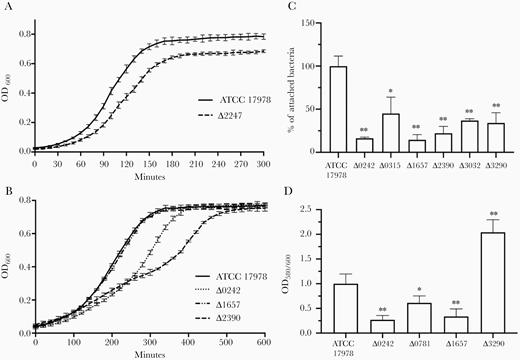
Growth curves of (A) Acinetobacter baumannii ATCC 17978 strain and the isogenic mutant derivative strain ∆2247 in Mueller Hinton II medium (nonlimited iron conditions); and (B) ATCC 17978 strain and the isogenic mutant derivative strains ∆0242, ∆1657, and ∆2390 in Mueller Hinton II medium supplemented with 0.2 mM iron chelator 2,2′-bipyridyl (limited iron conditions). C, Quantification of bacterial adhesion to A549 human alveolar epithelial cells by A. baumannii ATCC 17978 strain and the isogenic mutant derivative strains Δ0242, Δ0315, Δ1657, Δ2390, Δ3032, and Δ3290. D, Quantification of biofilm formation by A. baumannii ATCC 17978 strain and the isogenic mutant derivative strains Δ0242, Δ0781, Δ1657, and Δ3290. Experiments were performed with 3 biological replicates and bars represent SD. Unpaired student t test was used for statistical analysis. * P < .05; ** P < .01.
As feoA (A1S_0242), basB (A1S_2390), and bnfL (A1S_1657) genes encode proteins that are involved in iron uptake, the growth rates of Δ0242, Δ2390, and Δ1657 strains were determined in iron-deficient conditions. Both Δ0242 and Δ2390 showed a significant lower growth rate with a longer mean generation time (52 minutes, μ = 0.013 and 45 minutes, μ = 0.015, respectively) when compared to the parental strain (38 minutes, μ = 0.018), resulting in a significant reduction in fitness (P > .0001 and P = .002, respectively). No significant differences were observed between the Δ1657 strain (40 minutes, μ = 0.017) and the parental strain (Figure 4B and Table 2).
Effect of Gene Deletion on Attachment to Eukaryotic Cells and Biofilm Formation
The possible involvement of these genes in the ability of A. baumannii ATCC 17978 to adhere to biotic and abiotic surfaces was investigated. Deletion of feoA (A1S_0242), an oxygenase-like (A1S_0315), bnfL (A1S_1657), basB (A1S_2390), a tonB-like (A1S_3032), and esvF2 (A1S_3290) genes resulted in a significant reduction in the ability of ATCC 17978 to adhere to A549 human alveolar epithelial cells (Figure 4C and Table 2). No differences in adherence were observed when the other genes were deleted (Supplementary Figure 6A).
Strains Δ0242, Δ0781, and Δ1657 displayed significantly lower ability to form biofilm than the parental strain, while strain Δ3290 showed a higher ability to form biofilm (Figure 4D and Table 2). Deletion of the other selected genes did not lead to any significant differences in biofilm production (Supplementary Figure 6B).
Antimicrobial Susceptibility Assays of Derivative Mutants
The role of genes in antibiotic resistance was also assessed with the aim of finding potential new antimicrobial targets. No differences in antibiotic minimum inhibitory concentrations were observed for the mutant strains in the microdilution assays, except for the Δ2247 strain, where susceptibility to the cephalosporins cefoxitin, ceftazidime, cefotaxime, and cefepime was 4-fold higher than in the parental strain. However, penicillins and carbapenems retained their activity (Table 3). Non-β-lactam antibiotics also retained their activity against the Δ2247 mutant.
Minimal Inhibitory Concentration to β-Lactams of Parental Acinetobacter baumannii ATCC 17978 and its Derivative Mutant Strain Δ2247
β-lactam . | Minimal Inhibitory Concentration, mg/L . | |
---|---|---|
. | ATCC 17978 . | Δ2247 . |
Ampicillin | 16 | 16 |
Piperacillin/tazobactam | 8 | 8 |
Cefoxitin | 128 | 32 |
Ceftazidime | 4 | 1 |
Cefotaxime | 8 | 2 |
Cefepime | 2 | 0.5 |
Imipenem | 0.25 | 0.25 |
Meropenem | 0.25 | 0.25 |
β-lactam . | Minimal Inhibitory Concentration, mg/L . | |
---|---|---|
. | ATCC 17978 . | Δ2247 . |
Ampicillin | 16 | 16 |
Piperacillin/tazobactam | 8 | 8 |
Cefoxitin | 128 | 32 |
Ceftazidime | 4 | 1 |
Cefotaxime | 8 | 2 |
Cefepime | 2 | 0.5 |
Imipenem | 0.25 | 0.25 |
Meropenem | 0.25 | 0.25 |
Minimal Inhibitory Concentration to β-Lactams of Parental Acinetobacter baumannii ATCC 17978 and its Derivative Mutant Strain Δ2247
β-lactam . | Minimal Inhibitory Concentration, mg/L . | |
---|---|---|
. | ATCC 17978 . | Δ2247 . |
Ampicillin | 16 | 16 |
Piperacillin/tazobactam | 8 | 8 |
Cefoxitin | 128 | 32 |
Ceftazidime | 4 | 1 |
Cefotaxime | 8 | 2 |
Cefepime | 2 | 0.5 |
Imipenem | 0.25 | 0.25 |
Meropenem | 0.25 | 0.25 |
β-lactam . | Minimal Inhibitory Concentration, mg/L . | |
---|---|---|
. | ATCC 17978 . | Δ2247 . |
Ampicillin | 16 | 16 |
Piperacillin/tazobactam | 8 | 8 |
Cefoxitin | 128 | 32 |
Ceftazidime | 4 | 1 |
Cefotaxime | 8 | 2 |
Cefepime | 2 | 0.5 |
Imipenem | 0.25 | 0.25 |
Meropenem | 0.25 | 0.25 |
DISCUSSION
In this study, we characterized the complete transcriptome of A. baumannii ATCC 17978 and the clinical AbH12O-A2 strains during a pneumonia infection. Gene expression in A. baumannii isolated from infected lungs displayed a specific profile, with many genes up- or downregulated relative to in vitro growth conditions. A deeper study of these genes revealed that during the pneumonia infection A. baumannii upregulates different virulence factors involved in iron acquisition, host invasion, and antibiotic resistance. Recently, Zeng et al analyzed the transcriptome changes in lung cells in response to A. baumannii lethal pneumonia, which also showed a specific transcriptional profile enriched in inflammation pathways [41].
Different iron acquisition systems have been previously found to be highly upregulated in A. baumannii during bacteremia infection [12]. The level of expression and the number of upregulated genes were higher, thus iron could be more limited during bacteremia compared to pneumonia infection.
In the current study, 3 upregulated genes belonging to different iron uptake systems were selected for gene deletion and their role in virulence was studied showing a loss of adhesion ability, a lower growth rate in the absence of iron, and a decrease in virulence in a mice pneumonia infection model.
The A1S_0242 gene (feoA) belongs to the feoABC system, which has been proposed to be the major ferrous iron transport system in prokaryotes [23]. In a previous study, the role of this gene in iron uptake and virulence in A. baumannii was evaluated [16].
The A1S_1657 gene (bfnL), belonging to the baumanoferrin cluster, encodes an N-acetyltransferase that is involved in ferric siderophore biosynthesis [11]. This cluster contains 12 genes (bfnA-L) that have a high homology with genes encoding the acinetoferrin siderophore present in Acinetobacter haemolyticus [26]. The bfnL gene is probably involved in recognition of the siderophore; however, this role may be fulfilled by other siderophore receptors [11].
The A1S_2390 gene (basB) encodes a protein that is involved in the biosynthesis of acinetobactin. Acinetobactin has previously been described as the most important A. baumannii siderophore necessary for establishment of bacterial infection and cell damage [28]. The role of genes implicated in synthesis and uptake of this siderophore in the virulence of A. baumannii has been demonstrated [28]. BasB takes part in the final step of acinetobactin biosynthesis and is involved in obtaining preacinetobactin from N-hydroxyhistamine and aminoacyl-BasB.
The TonB-ExbBD inner membrane complex transfers the energy necessary for the iron uptake to outer membrane transporters [38]. Deletion of A1S_3032 gene, coding for a TonB-like protein, did not have any effect in the pneumonia infection. This could be due to the presence of other tonB genes that can carry out the same function or this gene could be involved in other TonB-dependent processes.
Lipopolysaccharide and capsule are important virulence factors for A. baumannii as they protect the bacteria against the host immune response [42]. During both bacteremia and pneumonia infection genes related to lipopolysaccharide and capsule production were upregulated [12]. The BfmR regulator was downregulated in vivo, which is expected considering that it has been described as a capsule repressor [42].
Genes belonging to secretion systems were also found to be overexpressed under in vivo conditions, showing the same profile as A. baumannii grown in human serum [43]. Interestedly, the deletion of A1S_0318 (T1SS) and A1S_0896 (T4SS) did not have any effect in the virulence during the pneumonia infection.
We found several efflux pumps to be upregulated during pneumonia infection. These results agreed with Jacobs et al showing that, during growth in serum, A. baumannii overexpressed 22 efflux pumps allowing the bacteria to tolerate antibiotic challenges [43]. Previous studies also demonstrated the role of these transporters in the protection of bacteria against the host immune response [44].
Bacteria communicate through the production of diffusible signal molecules, termed autoinducers during quorum sensing (QS). The expression of virulence factors in pathogenic bacteria is one of the processes controlled by QS [24]. The A1S_0781 gene (mtnN) codes for a methylthioadenosine/S-adenosylhomocysteine nucleosidase, which is directly involved in the biosynthesis of autoinducers such as AI-1 and AI-2 [25]. MtnN inhibition may block the production of both autoinducers, thereby disrupting QS [24]. In particular, deletion of mtnN resulted in diminished growth and reduced motility in Vibrio cholera [45].
Deletion of A1S_2247, A1S_3410, and A1S_3245, genes with different metabolic functions in A. baumannii, also led to a reduction in virulence in the murine pneumonia model. The A1S_2247 gene (yfgC, renamed bepA) codes for a putative periplasmic metalloprotease. This protein has been suggested to be responsible for maintenance of outer membrane integrity in E. coli, both by promoting assembly of outer membrane proteins (OMPs) and by proteolytically eliminating OMPs when their correct assembly is compromised [29]. Previous studies indicated that inactivation of BepA led to higher susceptibility to several antimicrobials in E. coli [46]. Interestingly, in the present study, deletion of this gene also increased susceptibility to all cephalosporins tested, while maintaining the susceptibility to penicillins, carbapenems, and other antimicrobial families. The involvement of this gene in β-lactam resistance may be related to access difficulties of the chromosomal β-lactamase to the β-lactams antimicrobials or to compromised barrier functions of the outer membrane [29]. Apart from the loss of virulence in the pneumonia model, the Δ2247 mutant showed a slight loss of fitness. Inhibition of this gene would thus have a double objective to combat both virulence and resistance to cephalosporins in these bacteria.
The A1S_3245 gene (hisF) is implicated in purine and histidine biosynthesis. This gene catalyzes production of 5′-(5-aminoimidazole-4-carboxamide) ribonucleotide [47], which can inhibit the lipopolysaccharide-induced production of proinflammatory cytokines, thus regulating the inflammation process [48]. The strain lacking this gene displayed much lower virulence, probably due to the involvement of the gene in the host immune response in vivo, preventing appropriate interleukin-6 secretion and leukocyte recruitment [49].
The A1S_3410 gene is thought to code for an acyltransferase, and it is possibly involved in the O-acetylation of the cell wall polymer peptidoglycan. The peptidoglycan is the target of lytic enzymes (eg, lysozyme), which are produced by eukaryotic hosts as the first line of defense against pathogens. The O-acetylation of peptidoglycan leads to resistance to lysozyme [31]. Sequence alignment searches suggested that the protein encoded by the A1S_3410 gene presents homology with the O-acetyltransferase OatA of Klebsiella pneumoniae (94% of identity) [32].
Other mutant strains lacking genes were constructed from the ATCC 17978 strain but did not decrease the virulence in murine pneumonia. In vitro assays revealed that the Δ3290 mutant increased biofilm production relative to the parental strain while decreasing its adhesion efficacy to epithelial alveolar cells. A1S_3290 encodes EsvF2, which is a transcriptional activator of TenA. TenA of Staphylococcus aureus is a thiaminase with a role in thiamine (vitamin B1) degradation. In biofilm-bound prokaryotic cells, Thermotoga maritima exhibits increased transcription of the genes involved in thiamine production [39]. Thus, inactivation of A1S_3290 may cause an excess of intracellular thiamine and an increase in biofilm production.
The present study described the whole transcriptome of A. baumannii during a pneumonia infection and demonstrated in vivo the key role of selected genes in the ability of A. baumannii to cause infection. This important advance will potentially provide valuable information that may help drug development in future studies.
Supplementary Data
Supplementary materials are available at The Journal of Infectious Diseases online. Consisting of data provided by the authors to benefit the reader, the posted materials are not copyedited and are the sole responsibility of the authors, so questions or comments should be addressed to the corresponding author.
Notes
Acknowledgment. We thank M. I. Voskuil (Department of Immunology and Microbiology, University of Colorado Medical School, CO) for providing pMo130.
Financial support. This work was supported by Instituto de Salud Carlos III (PI15/00860 and PI18/00501 to G. B. and PI14/00059 and PI17/01482 to A. B. and M. P.); Axencia Galega de Innovacion- Consellería de Economía, Emprego e Industria (IN607A 2016/22 to G. B); Spanish Network for Research in Infectious Diseases and European Development Regional Fund (REIPI RD16/0016/006 and FI18/00315 to J. C. V.-U); M. M.-G. was supported by the Spanish Society of Clinical Microbiology and Infectious Diseases (Clara Roy grant); and K. C.-P. was supported by the Spanish Association Against Cancer.
Potential conflicts of interest. All authors: No reported conflicts of interest. All authors have submitted the ICMJE Form for Disclosure of Potential Conflicts of Interest. Conflicts that the editors consider relevant to the content of the manuscript have been disclosed.
Presented in part: American Society for Microbiology Microbe, 20–23 June 2019, San Francisco, CA; and 29th European Congress of Clinical Microbiology and Infectious Diseases, 13–16 April 2019, Amsterdam, the Netherlands.
References
Author notes
M. M.-G., J. C. V.-U., and L. A.-F. contributed equally.
M. P. and A. B. contributed equally.