-
PDF
- Split View
-
Views
-
Cite
Cite
IkRak Jung, Becky Tu-Sekine, Sunghee Jin, Frederick Anokye-Danso, Rexford S Ahima, Todd T Brown, Sangwon F Kim, Dolutegravir Suppresses Thermogenesis via Disrupting Uncoupling Protein 1 Expression and Mitochondrial Function in Brown/Beige Adipocytes in Preclinical Models, The Journal of Infectious Diseases, Volume 226, Issue 9, 1 November 2022, Pages 1626–1636, https://doi.org/10.1093/infdis/jiac175
- Share Icon Share
Abstract
Antiretroviral therapy (ART) containing integrase strand transfer inhibitors (INSTIs) has been associated with weight gain in both ART initiation and switch studies, especially in women, but the underlying mechanisms are unclear.
The effects of dolutegravir (DTG) on food intake, energy expenditure, oxygen consumption in female mice, and gene expression from adipose tissues were assessed. Human and murine preadipocytes were treated with DTG either during differentiation into mature brown/beige adipocytes or postdifferentiation. Lipid accumulation, lipolysis, β-adrenergic response, adipogenic markers, mitochondrial respiration, and insulin response were analyzed.
Two-week administration of DTG to female mice reduced energy expenditure, which was accompanied by decreased uncoupling protein 1 (UCP1) expression in brown/beige adipose tissues. In vitro studies showed that DTG significantly reduced brown adipogenic markers, especially UCP1 in brown and beige adipocytes, whereas drugs from other classes did not. Furthermore, a loss of UCP1 by DTG led to a decrease in mitochondrial complex IV component, followed by a reduction in mitochondrial respiratory capacity and reduced insulin-stimulated glucose uptake.
Our findings show that DTG targets UCP1 and mitochondrial functions in brown and beige adipocytes and disrupts thermogenic functions in preclinical models, providing the potential mechanisms by which DTG suppresses energy expenditure leading to weight gain.
The advent of the integrase strand transfer inhibitor (INSTI) class has revolutionized the treatment of people with human immunodeficiency virus (HIV) due to their high efficacy, greater tolerability, and decreased drug–drug interactions. INSTIs are now recommended components of first-line and drug-switch antiretroviral therapy (ART) formulations [1–3]. However, there is growing evidence that the use of INSTIs can lead to a clinically significant increase in body weight or even clinical obesity that is more pronounced than other antiretroviral classes [4–8]. Moreover, these changes may pose an increased risk of diabetes and cardiovascular diseases. Hence, numerous studies have postulated the potential mechanisms or risk factors associated with ART, especially INSTIs, as African American race, female sex, older age, or backbone drugs, but there is no clear explanation of the cause associated with INSTI-associated weight gain [9–11].
Weight gain is driven by energy imbalance. Excess calories promote energy storage mainly as triglyceride in white adipose tissue (WAT). Concomitantly, the inability to use this energy increases adiposity and body weight [12, 13]. WAT plays an important role in energy storage, but contains few mitochondria and does not contribute significantly to organismal energy expenditure. Alternatively, brown adipose tissue (BAT), which is densely packed with mitochondria, can rapidly and robustly affect whole animal energy expenditure. This is particularly evident in response to a cold challenge, where BAT plays a key role in maintaining body temperature by uncoupling cellular respiration from adenosine triphosphate generation in mitochondria via the uncoupling protein 1 (UCP1) transporter to generate heat [14, 15]. Especially in rodents, it has been shown that fatty acid oxidation is critical for this process, and triglycerides in BAT and WAT are rapidly consumed to maintain thermogenesis [16–18]. Recently a distinct population of adipocytes, named brown-like (beige or brite) adipocytes, have been identified in WAT and shown to share a number of properties previously thought unique to brown adipocytes, including multilocular lipid storage and UCP1 expression [19, 20]. Although their origins are under debate, these cells are functionally thermogenic. The appearance of beige adipocyte in WAT has been termed WAT browning, and is commonly induced by cold exposure or stimulation with a sympathetic agonist such as noradrenaline or isoproterenol [21, 22]. Importantly, BAT-like tissue was discovered in adult humans using positron emission tomography scanning, providing therapeutic potential for the treatment of obesity-associated diseases [23–25].
Here we report that DTG specifically suppresses UCP1 expression in brown and beige adipocytes while marginally enhancing white adipogenesis, leading to reduced thermogenesis and increased lipid storage. Moreover, suppression of UCP1 in brown adipocytes is associated with reduction of mitochondrial respiratory chain complexes, mitochondrial capacity, and insulin-mediated glucose uptake.
METHODS
Animal and Drug Treatment
Experiments were performed in accordance with the Institutional Animal Care and Use Committee guidelines with its approvals. Ten-week-old C57BL6J female mice (Jackson Laboratories) were housed n = 5/cage in a 12-hour light:dark cycle, at ambient temperature of 23°C, and allowed free access to food and water. Groups of mice were fed normal rodent chow (4 kcal% fat; 5001 from Lab Diet). Mice were administered with DTG (10 mg/kg/day, 5% dimetyl sulfone + 30% polyethylene glycol 300 + 10% Tween80 + 55% double distilled water) for 2 weeks subcutaneously. The dosage was calculated via allometric scaling [26, 27], based on an average 20-g mouse.
Adipocyte Differentiation
For white adipocyte differentiation, cells were differentiated to mature adipocytes by treatment with 0.5 mM 3-isobutyl-1-methylxanthin (IBMX), 1 µM dexamethasone, and 167 nM insulin for 2 days, followed by continued culture in 167 nM insulin, which was changed every 2 days thereafter until analysis. For brown/beige adipocyte differentiation, cells were treated with 0.5 mM IBMX, 1 µM dexamethasone, 167 nM insulin, 1 nM T3, 1 uM rosiglitazone, and 125 uM indomethacin for 2 days, followed by continued culture in 167 nM insulin, 1 nM T3, and 1 uM rosiglitazone, which was changed every 2 days with an indicated drug thereafter until analysis. Average percentage of differentiation was >90% (calculated by number of cells containing lipid droplets / all the cells in the field × 100), and a plate of cells of which efficiency was below 75% was excluded.
Statistical Analysis
Results were analyzed using GraphPad Prism version 9.0.0 software for Windows (GraphPad, San Diego, California). We assessed statistical significance by unpaired 2-tailed t tests, 1-way analysis of variance (ANOVA) (Dunnett test), or 2-way ANOVA (Tukey test) for multiple comparisons. The level of significance was set to P < .05.
Detailed methods are available in the Supplementary Materials.
RESULTS
DTG Administration Reduces Thermogenesis in Mice
DTG administration resulted in a significant increase in fat mass (about 15%) while trended to higher body weight (about 5.1%) without statistical significance (Figure 1). Indirect calorimetry revealed that DTG administration led a slight decrease in oxygen consumption (VO2) and energy expenditure without significant changes in food intake, respiratory exchange ratio, or mobility, suggesting reduced thermogenesis (Figure 1; Supplementary Figure 1). Importantly, β-adrenergic agonist (CL316 243; CL, 1 mg/kg)–induced stimulation of VO2 and heat generation were significantly attenuated in the DTG-administered group (Figure 1B). UCP1 expression in intrascapular BAT and inguinal WAT were reduced. Moreover, SREBP, a master regulator for lipid synthesis, was slightly upregulated in inguinal WAT whereas there was no change in intrascapular BAT (Figure 1C). Finally, the direct DTG treatment (2 μM for 2 hours) of intrascapular BAT slices significantly reduced an expression of UCP1 messenger RNA (mRNA) (Figure 1D), suggesting that DTG may interfere adaptive thermogenesis by directly disrupting UCP1 expression.
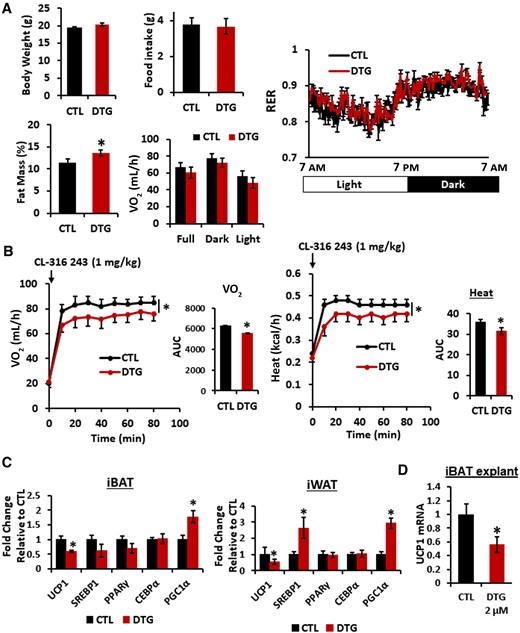
Dolutegravir (DTG) administration reduces uncoupling protein 1 expression in adipose tissues and thermogenesis in mice. Female mice (10 weeks old, n = 9–10) were administered with DTG (15 mg/kg/day) subcutaneously for 2 weeks. A, Body weight. Fat mass was measured by a body composition analyzer. Food intake, oxygen consumption (VO2), and respiratory exchange ratio were measured by Comprehensive Lab Animal Monitoring System. B, VO2 and energy expenditure/heat were measured after administering CL-316 243 (1 mg/kg) interperitoneally. Area under the curves were measured. C, Interscapular BAT and inguinal WAT were isolated and messenger RNA (mRNA) expression was measured by quantitative reverse-transcription polymerase chain reaction (qRT-PCR) (n = 8). D, Interscapular brown adipose tissues were sliced with a tissue chopper and incubated with DTG (2.0 μM) for 4 hours and mRNA expression was measured by qRT-PCR. Control/untreated and DTG-treated conditions were compared (n = 6). *P < .05. Abbreviations: AUC, area under the curve; CEBPα, cyclic adenosine monophosphate response element binding protein α; CTL, control; DTG, dolutegravir; iBAT, interscapular brown adipose tissue; iWAT, inguinal white adipose tissue; mRNA, messenger RNA; PGC1α, peroxisome proliferator-activated receptor-γ coactivator1α; PPARγ, peroxisome proliferator-activated receptor γ; RER, respiratory exchange ratio; SREBP1, sterol regulatory element binding protein 1; UCP1, uncoupling protein 1; VO2, oxygen consumption.
DTG does not affect glucose uptake and insulin signaling in hepatocytes and myocytes.
To better understand the potential target of DTG in metabolic processes, we examined whether DTG directly disrupts the metabolic signaling pathways (eg, pAMPK levels, insulin-mediated Akt activation, or glucose uptake) utilizing the primary murine hepatocytes, and myocytes isolated from C57BL/6 mice. First, DTG has no effect on glucose uptake (Supplementary Figure 2A) nor AMPK, which is a key cellular energy sensor tightly related to glucose level (Supplementary Figure 2B). Moreover, DTG does not have any effect on insulin-mediated Akt activation in primary hepatocytes (Supplementary Figure 2C). Furthermore, we observed similar results from primary myocytes without any disruption in glucose homeostasis or insulin signaling (Supplementary Figure 2D–F), suggesting that DTG is less likely to disrupt the metabolic processes in hepatocytes or myocytes.
DTG Primarily Affects Adipocytes
Stromal vascular fraction (SVF) cells from either mouse intrascapular BAT or inguinal WAT were differentiated into the mature adipocytes [28] with or without DTG. DTG treatment reduced brown adipogenesis with reduced expression of UCP1 mRNA (Figure 2A and 2B). We have confirmed that effects of DTG on brown adipocytes is not due to its cytotoxicity (Supplementary Figure 3). In contrast, white adipocytes treated with DTG showed slight increase in lipid accumulation and gene expression related to lipogenesis in accordance with a previous report [29] (Figure 2C and 2D). Our data show that DTG differentially affects adipocytes during brown and white adipogenesis.
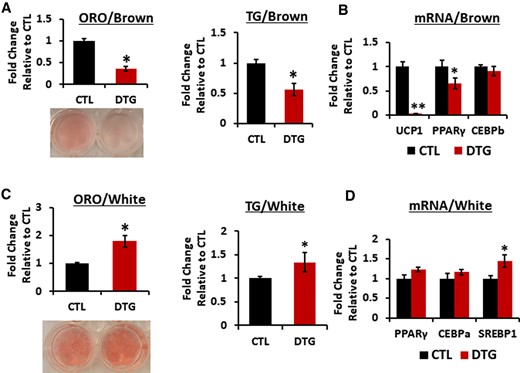
Dolutegravir (DTG) treatment targets uncoupling protein 1 in brown adipocytes in vitro. A and B, Primary murine preadipocytes were isolated from interscapular brown adipose tissue (4- to 6-week-old female mice) and differentiated with or without DTG for 8 days. A, Oil Red O (ORO) staining and quantification (n = 8). A representative image of ORO-stained adipocytes is shown. Triglyceride (TG) detection by Triglyceride-Glo assay kit (n = 8). B, Messenger RNA (mRNA) expression levels by quantitative reverse-transcription polymerase chain reaction (qRT-PCR) (n = 6). C and D, Primary murine preadipocytes were isolated from inguinal white adipose tissue (4- to 6-week-old female mice) and differentiated with or without DTG for 8 days. C, ORO staining and quantification (n = 8) and a representative image of ORO-stained cells are shown. TG detection by Triglyceride-Glo assay kit (n = 8). D, mRNA expression levels by qRT-PCR. (n = 6). *P < .05; **P < .005. Abbreviations: CEBPb, cyclic adenosine monophosphate response element binding protein b; CTL, control; DTG, dolutegravir; mRNA, messenger RNA; ORO, Oil Red O, PPARγ, peroxisome proliferator- activated receptor γ; SREBP1, sterol regulatory element binding protein1; TG, triglyceride; UCP1, uncoupling protein 1.
DTG Suppresses UCP1 Expression and Brown/Beige Adipogenic Markers
Detailed analyses revealed that expression of brown-specific markers such as Dio2 and CIDEA were significantly reduced along with UCP1 in a dose-dependent manner (Figure 3A). Moreover, a decrease in UCP1 mRNA was recapitulated in its protein levels, and yet other mitochondrial proteins such as prohibitin 1 or voltage-dependent ion channel levels were not affected (Figure 3B; Supplementary Figure 4). Notably, UCP1 expression was decreased as soon as 4 hours of DTG treatment, suggesting that the decrease in UCP1 expression is not due to reduced brown adipogenesis (Figure 3C). We further confirmed that DTG reduced UCP1, Dio2, and CIDEA in human brown adipocytes (Figure 3D). Importantly, bictegravir also suppressed UCP1, Dio2, and CIDEA expression in human brown adipocytes while other classes of antiviral drugs such as doravirine and efavirenz had no effect on UCP1 expression in human brown adipocytes. In contrast, DTG treatment slightly increased the white adipogenic marker FABP4 and a master regulator of lipid homeostasis, SREBP1, in human adipocytes (Supplementary Figure 5). In addition, we examined whether DTG has a similar effect on beige adipogenesis utilizing SVF cells isolated from mouse inguinal WAT. We found that DTG treatment resulted in a decrease in UCP1 mRNA as seen in brown adipocytes as well as a reduction on beige adipocyte–specific markers (eg, CITED1, CD137, and TMEM26) (Figure 4). We also found similar results showing reduced UCP1, CITED1, and CD137 mRNA in beige adipocytes differentiated from NIH-3T3 cells (Supplementary Figure 6). These data show that thermogenic process in brown and beige adipocytes is the primary target disrupted by INSTIs.
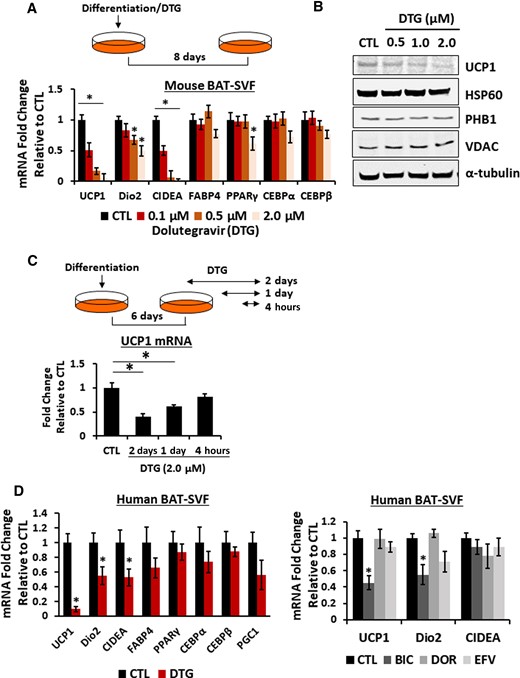
Dolutegravir (DTG) reduces brown adipogenic–specific markers in mouse and human adipocytes. A–C, Primary murine preadipocytes were isolated from interscapular brown adipose tissue (4- to 6-week-old female mice) and differentiated in the presence of DTG as indicated. A, Messenger RNA (mRNA) expression of brown adipocyte markers by quantitative reverse-transcription polymerase chain reaction (qRT-PCR) (n = 8). B, Immunoblotting of mitochondrial proteins in brown adipocytes treated with DTG. A representative image from 4 independent experiments. C, Illustration of DTG treatment and mRNA expression of brown adipocyte markers by qRT-PCR (n = 8). D, mRNA expression in human preadipocytes (A41hBAT-SVF) differentiated into brown adipocytes (21 days) in the presence of DTG (2.5 μM), bictegravir (2.5 μM), doravirine (5 μM), and efavirenz (5 μM) (n = 6). *P < .05. Abbreviations: BAT-SVF, brown adipose tissue-stromal vascular fraction; BIC, bictegravir; CEBP, cyclic adenosine monophosphate response element binding protein; CIDEA, cell eath inducing DFFA like effector a; CTL, control; Dio2, iodothyronine deiodinase 2; DOR, doravirine; DTG, dolutegravir; EFV, efavirenz; FABP4, fatty acid binding protein 4; HSP90, heat shock protein 90; mRNA, messenger RNA; PGC1, peroxisome proliferator-activated receptor-γ coactivator1; PHB1, prohibitin 1; PPARγ, peroxisome proliferator-activated receptor γ; UCP1, uncoupling protein 1; VDAC, voltage-dependent anion channel.
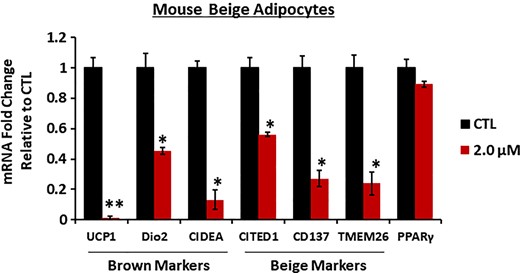
Dolutegravir (DTG) reduces beige adipogenic–specific markers in mouse adipocytes. Primary murine preadipocytes were isolated from inguinal white adipose tissue (4- to 6-week-old female mice) and differentiated to beige adipocytes in the presence of DTG (2.0 μM) for 8 days. Messenger RNA expression levels were measured by quantitative reverse-transcription polymerase chain reaction (n = 6). *P < .05; **P < .005. Abbreviations: CD137, tumor necrosis factor receptor superfamily member 9; CIDEA, cell eath inducing DFFA like effector a; CITED1, Cbp/p300-interacting transactivator 1; CTL, control; Dio2, iodothyronine deiodinase 2; mRNA, messenger RNA; PPARγ, peroxisome proliferator-activated receptor γ; SREBP1, sterol regulatory element binding protein1; TMEM26, transmembrane protein 26; UCP1, uncoupling protein 1.
DTG Does Not Directly Block Adrenergic Signaling
Since UCP1 expression and the subsequent thermogenic response are induced by adrenergic receptor stimulation, we wondered whether the reduced UCP1 expression was linked to a disrupted adrenergic response. We examined UCP1 expression in brown adipocytes treated with DTG for 8 days before and after treatment with the adrenergic agonist isoproterenol for 4 hours. While DTG treatment reduced basal UCP1 expression, isoproterenol treatment resulted in a similar fold increase in UCP1 in both DTG-treated and control brown adipocytes (Figure 5A). Since adrenergic stimulation initiates the canonical Gs-adenylyl cyclase-cAMP-PKA pathway, we also examined isoproterenol-induced cAMP production in brown adipocytes and found that DTG treatment had no effect on cAMP production (Figure 5B), indicating that adrenergic receptor response is intact.
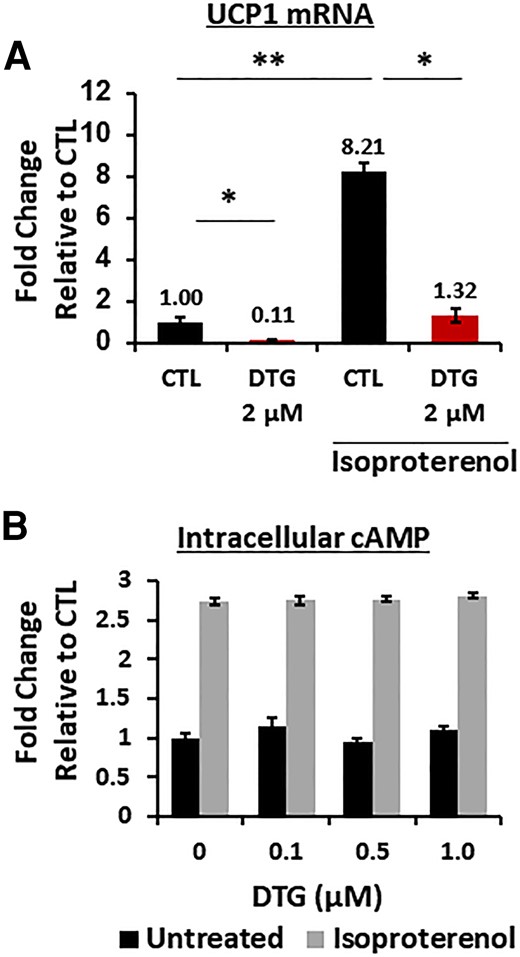
Dolutegravir (DTG) does not affect β3 adrenergic receptor response. A, Messenger RNA expression in brown adipocytes in the presence of DTG during 8 days of differentiation with or without isoproterenol treatment (10 μM) for 4 hours (n = 6). B, Intracellular cAMP measurement in brown adipocytes in the presence of DTG during differentiation with or without isoproterenol (10 μM) for 10 minutes (n = 8). Abbreviations: cAMP, cyclic adenosine monophosphate; CTL, control; DTG, dolutegravir; mRNA, messenger RNA; UCP1, uncoupling protein 1.
DTG Attenuates Lipolysis by Reducing Lipid Droplet Accumulation in Brown Adipocytes
Lipolysis is one of the key mechanisms for the fatty acid–induced activation of UCP1 and subsequent heat production in BAT and beige tissues upon adrenergic stimulation [30, 31]. Hence, we examined whether DTG treatment alters lipolysis by measuring glycerol released into the media following isoproterenol treatment. Glycerol release was decreased in a DTG dose-dependent manner (Figure 6A). Interestingly, triglyceride levels in brown adipocytes were similarly decreased (Figure 6A). Therefore, we performed linear regression to find a best-fit line as well as the correlation analysis showing that a strong correlation (R2 = 0.91, P = .0102) between the observed changes in lipolysis and in triglyceride accumulation (Figure 6B). Moreover, the lipid droplet–associated protein perilipin 1 was decreased in a DTG dose-dependent manner whereas there was no significant change in the levels of lipolysis proteins such as adipose triglyceride lipase (ATGL) or phosphorylated hormone-sensitive lipase (pHSL) (Figure 6C; Supplementary Figure 7). In addition, we further confirmed that there are no differences in expression of mRNA related to lipolysis as well as fatty acid oxidation (Figure 6D). Furthermore, DTG treatment of mature brown adipocytes did not have any effect on lipolysis (Supplementary Figure 8). These data suggest that the observed DTG-dependent decrease in lipolysis is due to a reduction in triglyceride accumulation caused by disrupted brown adipogenesis, and not a direct inhibition of lipolysis.
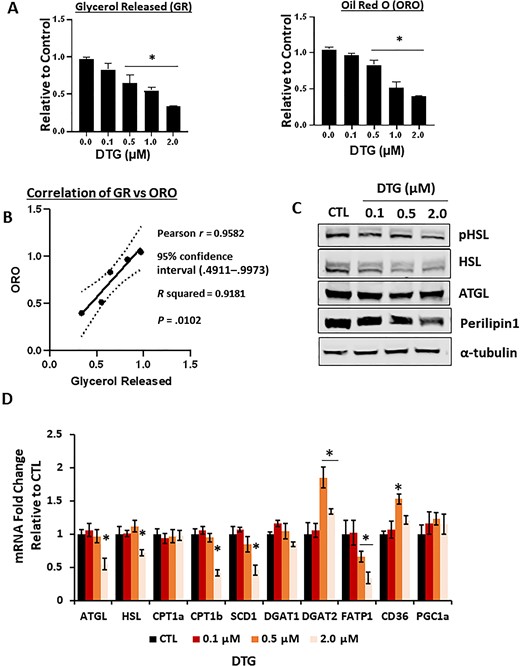
Dolutegravir (DTG) reduces lipolysis by reducing the lipid storage during differentiation. Primary murine preadipocytes were isolated from interscapular brown adipose tissues (4- to 6-week-old female mice) and differentiated in the presence of DTG (8 days). A, Amount of glycerol released upon 10 μM isoproterenol treatment for 1 hour (n = 6). Quantification of Oil Red O (ORO) staining (n = 6). B, Correlation coefficient of ORO vs lipolysis was examined by a linear regression model. — best-fit line, 95% confidence interval (P = .0102). C, Immunoblotting of lipolysis-related protein from brown adipocytes treated with DTG. A representative image from 4 independent experiments. D, Messenger RNA expression of lipolysis and lipid droplet formation–related genes from brown adipocytes treated with DTG by quantitative reverse-transcription polymerase chain reaction (n = 6). *P < .05. Abbreviations: ATGL, adipose triglyceride lipase; CD36, cluster of differentiation 36; CPT, carnitine palmitoyltransferase; CTL, control; DGAT, diacylglycerol O-acyltransferase; DTG, dolutegravir; FATP1, fatty acid transport protein 1; GR, glycerol released; HSL, hormone-sensitive lipase; mRNA, messenger RNA; ORO, Oil Red O; PGC1a, peroxisome proliferator-activated receptor-γ coactivator1; pHSL, phosphorylated hormone-sensitive lipase; SCD1, stearoyl-CoA desaturase1.
DTG Disrupts Mitochondrial Respiratory Chain Proteins and Insulin Functions
UCP1 dissipates the mitochondrial proton motive force generated by the respiratory chain to produce heat and increase thermogenesis [32, 33]. In fact, genetic deletion of UCP1 has been shown to result in the loss of mitochondrial respiratory chain complexes [34]. DTG treatment reduced not only UCP1 protein expression but also the level of components (NDUFB8, MTCO1, and COX IV) of complex I and IV, whereas succinyl dehydrogenase A (a component for complex II) and UQCRC2 (a component for complex III) were not affected (Figure 7A; Supplementary Figure 9). We also confirmed a significant decrease in activity of COXIV (complex IV), which matches with a decrease in protein (Figure 7B). In addition, respiratory capacity was decreased by approximately 2-fold with minimal response to carbonyl cyanide 4-(trifluoromethoxy)phenylhydrazone in DTG-treated cells (Figure 7B). Finally, we examined whether DTG affects insulin response and glucose uptake. We found that DTG-treated brown adipocytes have reduced insulin sensitivity and insulin-mediated glucose uptake (Figure 7C and 7D; Supplementary Figure 10). Hence our data showed that DTG treatment disrupts mitochondrial respiration and insulin-mediated glucose uptake in brown adipocytes.
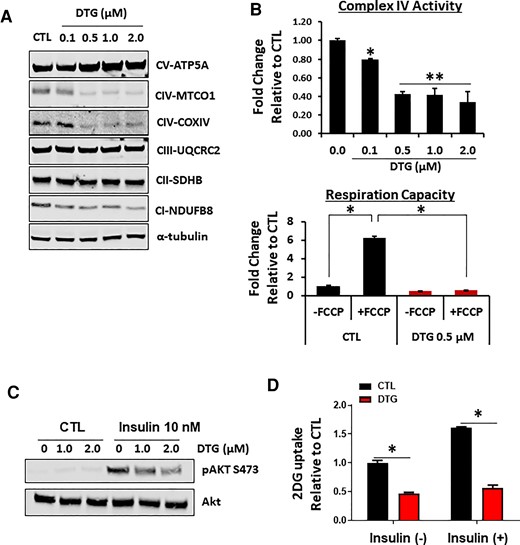
Dolutegravir (DTG) treatment disrupts mitochondrial respiratory complex without affecting mitochondrial biogenesis. A, Immunoblotting of mitochondrial respiratory chain complexes in brown adipocytes treated with DTG during differentiation for 8 days. A representative image from 4 independent experiments. B, Mitochondrial complex IV activity in permeabilized cells (n = 5) and mitochondrial respiratory capacity in intact cells utilizing carbonyl cyanide 4-(trifluoromethoxy)phenylhydrazone, uncoupler of oxidative phosphorylation in mitochondria (3 experiments in triplicate). C, 2DG uptake in primary brown adipocytes treated with DTG with or without 10 nM insulin (n = 6). D, Insulin-mediated Akt activation in primary brown adipocytes treated with DTG. A representative image from 4 independent experiments. *P < .05; **P < .005. Abbreviations: 2DG, 2 deoxy-glucose; CI-NDUFB8, complex I–NADH:uniquinone oxidoreductase subunit B8; CII-SDHB, complex II–succinate dehydrogenase subunit B; CIII-UQCRC2, complex III–uniquinol-cytochrome c reductase core protein 2; CIV-COXIV, complex IV–cytochrome C oxidase subunit IV; CIV-MTCO1, complex IV–mitochondrial complex IV subunit 1; CTL, control; CV-ATP5A, complex V–adenine triphosphate synthase subunit 5A; DTG, dolutegravir; FCCP, carbonyl cyanide 4-(trifluoromethoxy)phenylhydrazone.
DISCUSSION
Here we identified that DTG specifically targets mitochondria in brown and beige adipocytes and potentially disrupts thermogenesis utilizing a virus-free mouse model as well as in vitro human and murine cell culture systems. Our detailed analyses elucidated that DTG reduces brown and beige adipocyte differentiation and is a potent inhibitor of UCP1 expression in mouse as well as human brown adipocytes. Importantly, treatment with bictegravir, another INSTI, also caused a significant decrease in UCP1 in brown adipocytes, whereas drugs such as doravirine or efavirenz from other classes did not. Moreover, this is one of the first studies demonstrating that INSTIs differentially target brown and white adipocytes.
Antiretroviral drugs have been associated with alterations in fat distribution, also known as lipodystrophy [35–37]. It has been long observed that treatment-naive groups always experience an increase in weight, which is usually recognized as a return to health. However, recent observations suggest that INSTIs in particular lead to a general weight gain, which is beyond the return to health phenomenon [4–10]. Weight gain is driven by energy imbalance. Excess calories increase energy storage mainly as triglyceride in WAT, and the inability to use this energy increases adiposity and body weight gain. Our studies utilizing a preclinical model revealed that DTG reduces energy expenditure, in particular thermogenesis, by downregulating UCP1 in brown/beige adipocytes. In fact, previous studies have shown that the duration of combination ART (cART) is negatively related to the expression of brown adipogenic markers in white subcutaneous adipose tissues isolated from 18 people with HIV, and yet individual analyses by subclasses of ART revealed that only the protease inhibitor (PI) class had a statistically significant impact [38]. Moreover, this negative correlation between cART and brown adipose marker expression is primarily detected in a subgroup with lipodystrophy, suggesting that this may be due to lipotoxicity typically associated with the PI class.
We observed that DTG administration in mice led to a reduced energy expenditure and, especially, β3 adrenergic stimulation-mediated thermogenesis or adaptive thermogenesis. The main site for adaptive thermogenesis is brown/beige adipose tissues, and a disruption of these sites can reduce energy expenditure [15]. However, the amount of active BAT in adult humans is highly variable and dependent on many factors (eg, living environment; cold vs warm, sex, body weight/body mass index [BMI], or age) [39, 40]. Interestingly, it has been shown that females have a higher level of adipose tissues including BAT than males [23, 41] and BAT in females has a higher metabolic activity [39]. Hence it is tempting to speculate that this variance in the amount or activity of BAT may contribute to the diverse response to cART (or DTG) with respect to weight gain and especially prevalence of weight gain in females compared with males. On the other hand, it has been shown that BAT and BMI are inversely related, and that BAT activity and BAT mass decline with age in humans while WAT increases [42]. However, it is important to point out that even though BAT plays a critical role in energy expenditure, it relies on the supply of fatty acid from WAT [43, 44]. We and other researchers showed that DTG also affects WAT differentiation and its function [29]. Hence, future studies investigating an interplay between WAT and BAT under different circumstances further clarify how DTG-mediated disruption of adipose tissues collectively affects body weight. UCP1 is a brown/beige-specific protein that plays a key role in adaptive thermogenesis. Reduced expression of UCP1 can result in a decrease in energy expenditure, which has been demonstrated in various animal studies [32, 45]. Our detailed in vitro studies show that UCP1 in brown or beige adipocytes may be a major target of DTG. Adaptive thermogenic responses (eg, upregulation of UCP1 or increased lipolysis) are upregulated by adrenergic stimulation and yet our study confirmed that β3 adrenergic receptor agonist–induced cAMP production was not affected in brown adipocytes, suggesting that adrenergic stimulation is not impaired by DTG. Nevertheless, we showed that lipolysis upon adrenergic stimulation decreased in DTG-treated cells. In addition, this decrease in lipolysis is highly correlated with a decreased amount of lipid droplets as well as perilipin 1 level. Moreover, ATGL or pHSL/HSL protein expression, which is induced by isoproterenol, was not reduced by DTG, suggesting that the lipolysis process itself was not impaired by DTG. These data further support the notion that the adrenergic receptor pathway is not likely to be affected by DTG. UCP1 is stimulated by fatty acids, and mitochondria utilize fatty acids as the main energy source to produce heat. It has been shown that the release of fatty acid can also stimulate UCP1 expression [46]. While we found that β3 adrenergic receptor agonist–mediated cAMP production is not altered by DTG under our conditions, we cannot exclude the possibility that the decrease in UCP1 induction is linked to reduced levels of fatty acid release as shown in glycerol release experiment. Even though UCP1 can utilize other sources such as glucose, our data showed that DTG impaired insulin signaling and insulin-mediated glucose uptake, suggesting that DTG disrupts thermogenic process in brown adipocytes.
UCP1 is the mitochondrial protein primarily responsible for uncoupling oxidative phosphorylation. We found that DTG treatment reduced mitochondrial respiratory capacity in brown/beige adipocytes, which is associated with reduced expression of complex I and IV component proteins. It has been shown that a deletion of UCP1 in brown adipocytes leads to suppression of mitochondrial respiratory proteins [34]. It is prudent to point out that nucleoside reverse transcriptase inhibitors (NRTIs) are known to target the mitochondrial respiratory chain by inhibiting mitochondrial DNA polymerase [47, 48], leading to a reduction of all complexes encoded by mitochondrial DNA such as COX I–III, not including COX IV, suggesting that effect of DTG on mitochondrial protein differs from NRTIs. Interestingly, a recent report also showed that mice without UCP1 in BAT had all the respiratory chain proteins from complex I–V reduced [34], whereas our study showed that DTG-treated cells mainly had a reduced level of complex I and IV, suggesting that DTG effect on mitochondrial respiratory chain may be independent of reduced UCP1 expression. However, this discrepancy between these 2 studies may be caused by differences in duration of UCP1 loss. Nevertheless, we did not find any changes in other mitochondrial proteins, suggesting that overall mitochondrial contents are not altered by DTG treatment. Therefore, further studies are needed to delineate the effects of DTG on UCP1 functions associated with mitochondrial respiratory chain.
Our study has several limitations. Our study was based on virus-free animal and in vitro models. However, DTG has been detected within adipocytes and the stromavascular fraction in adipose tissues of people with HIV, suggesting that it has access to adipose stem cells and adipocytes in vivo [49], and our studies were conducted below maximum concentration value detected in plasma [50]. It has been well known that HIV can affect the fat itself and hence this modification may affect how INSTIs or DTG interact with fat tissues, especially brown adipocytes. Even though weight gain is detected well beyond the point where viral level is strongly suppressed, we cannot exclude a possibility that HIV may have permanently modified fundamental processes that can influence metabolic pathways in adipocytes. Moreover, inflammation is one of the key factors in weight gain, and people with HIV have a compromised immune system. This may further affect the metabolic pathways responding to DTG in people with HIV.
In summary, we showed here for the first time, to the best of our knowledge, that DTG treatment disrupts energy balance by suppressing the thermogenesis in mouse model. Moreover, this change is mediated by a decrease in brown and beige adipogenesis associated with a decrease in UCP1 expression and mitochondrial disruption. Hence, these findings present the potential mechanisms by which DTG suppresses energy expenditure leading to weight gain and may afford a means of managing people with HIV treated by ART containing DTG.
Supplementary Data
Supplementary materials are available at The Journal of Infectious Diseases online (http://jid.oxfordjournals.org/). Supplementary materials consist of data provided by the author that are published to benefit the reader. The posted materials are not copyedited. The contents of all supplementary data are the sole responsibility of the authors. Questions or messages regarding errors should be addressed to the author.
Notes
Author contributions. S. F. K. conceptualized the study. I. J., B. T.-S., and S. F. K. designed the study. I. J., B. T.-S., S. J., F. A.-D., and S. F. K. performed research. I. J., B. T.-S., S. J., F. A.-D., and S. F. K. analyzed data. R. S. A., T. T. B., and S. F. K. wrote the manuscript. All authors approved the final manuscript.
Financial support. This work was supported by the Johns Hopkins Center for AIDS Research Scholar Grant for faculty development (grant number P30AI094189 to S. F. K.) and the National Institutes of Health (grant number K24AI120834 to T. T. B.).
References
Author notes
I. J. and B. T.-S. contributed equally to this work.
Presented in part: Virtual Conference on Retroviruses and Opportunistic Infections, 2021, Abstract F515.
Potential conflicts of interest. All authors: No reported conflicts of interest.
All authors have submitted the ICMJE Form for Disclosure of Potential Conflicts of Interest. Conflicts that the editors consider relevant to the content of the manuscript have been disclosed.